ABSTRACT
Two bioactive peptide fractions (SWP-I and SWP-II) of swim bladder peptides from Atlantic cod (Gadus morhua) were obtained through enzymatic hydrolysis and isolated by gel filtration chromatography. The properties of two fractions were characterized by FT-IR, UV scanning spectroscopy, amino acid composition analysis and molecular weight determination. The results showed that the content of aromatic amino acids in SWP-II was higher than that in SWP-I, and the molecular of SWP-I and SWP-II were 4976 Da and 1960 Da, respectively. The radical scavenging activities showed that SWP-I and SWP-II were effective scavengers of DPPH•, HO•, and O2-•, and demonstrated high Fe2+-chelating activity. The scavenging capacities of SWP-II were stronger than those of SWP-I at the same concentration. Cytoprotective experiment suggested that SWP-I and SWP-II possessed ROS-scavenging activity. Pretreatment with these two peptides increased viability rates, suppressed SA-β-gal activities and inhibited apoptosis rates in hydrogen peroxide (H2O2)-induced premature senescent 2BS cells. In conclusion, the enzymatic peptides from swim bladders of Atlantic cod (Gadus morhua) possessed antioxidant activities and could effectively protect premature cell senescence induced by H2O2.
INTRODUCTION
Aging, also called senescence, refers to an irreversible phenomenon that affects all organisms under normal conditions as their biological development and age increase. Aging affects cellular functions, the stability of their internal environments, and decreases their stress-response abilities, eventually leading to death.[Citation1] Aging is a complex process and the free radical senescence theory proposed by Harman in 1956 is the first and most classical theory about oxidation and aging.[Citation2] In recent years, excessive production of free radicals and reactive oxygen species (ROS) was considered to be the main causes of aging because they could lead to cellular injury of DNA, leading to significant apoptotic and necrotic cell death.[Citation3,Citation4]
Antioxidants play important roles in preventing oxidative stress by safely interacting with free radicals, promoting lower rates of brain aging and longer life spans.[Citation5] Traditionally used antioxidants include synthetic antioxidants such as butylated hydroxyanisole (BHA), butylated hydroxytoluene, tert-butyl-hydroquinone, and propyl gallate.[Citation6] However, the potential hazards of these include liver injury and carcinogenesis, which limit their clinical applications.[Citation7] Recently, novel peptides from marine organisms have been attracting increased attention due to their high antioxidant activities, better biocompatibility, and lower toxicity.[Citation8,Citation9]
The swim bladder is an internal organ of most bony fish which controls their buoyancy.[Citation10] Previous research has shown that the peptides prepared by enzymolysis of swim bladder possessed high antioxidant activities.[Citation11] In order to investigate the antioxidant effects of enzymatic peptides isolated from swim bladders of Atlantic cod (Gadus morhua), their free radical scavenging abilities were assessed in vitro. In addition, an oxidation-induced cell senescence model was established by treating human fetal lung diploid fibroblast cells (2BS) with H2O2, and the cell viability, ROS content, SA-β-gal staining, and apoptosis were analyzed to assess the effects of the peptides on aging.
MATERIALS AND METHODS
Materials and chemicals
Swim bladders of Atlantic cod (Gadus morhua) were supplied by the Oceanese Group Co. (Qingdao, Shandong, China). Human embryonic lung diploid fibroblasts cells (2BS cells) were purchased from Keygen Biotech Co. (Nanjing, Jiangsu, China). Fetal bovine serum (FBS) and minimum essential medium (MEM) were acquired from Thermo Fisher Scientific Co. (Waltham, MA, USA). Sephadex G-15 and the apoptosis detection kit were obtained from Solarbio Co. (Beijing, China). The ROS kit and SA-β-gal staining kit were purchased from Beyotime Biotechnology Co. (Shanghai, China). Other chemicals and reagents used were of analytical grade.
Preparation of enzymatic peptides
Swim bladders were cut into of 0.5 × 0.5 cm chips and dipped in 0.1 M NaOH (1:10 w/v) for 24 h. After being homogenized with distilled water at a ratio of 1:5 (w/v), the homogenate was enzymolyzed by protemax. The hydrolysis process was under the condition of 55°C for 6 h, at pH 7.5, using 200 U/mL of enzyme. After hydrolysis, the mixture heated in a bath at 100°C for 5 min to inactivate the enzyme. Then the product was centrifuged at 4000 × g for 15 min. The supernatants, named SWP, were collected, concentrated with vacuum rotator evaporator, freezing dried, and stored at – 20°C until use.[Citation12]
Isolation of peptides by gel filtration chromatography
Ten milliliters 100 mg/mL SWP were applied to sieve chromatography column (2.6 × 60 cm) loading with Sephadex G-15 gel and eluted with distilled water at a flow rate of 1.5 mL/min. The absorbance was detected at 280 nm. According to the elution curve, the main fractions were collected.[Citation13]
Characterization of peptides
Fourier transforming infrared spectroscopy (FT-IR): FT-IR of two peptides was performed using a Bruker Fourier transform infrared spectrometer (Tensor 27, Bruker, Billerica, MA, USA). The samples of SWP-I and SWP-II were prepared by KBr pellet method and the wavenumber was ranged from 400 to 4000 cm−1.[Citation14]
UV scanning spectroscopy: Each peptide was dissolved phosphate buffer solution and the ultraviolet absorption spectrum was detected using a UV–vis spectrophotometer at room temperature. The wavelength was set to be between 190 nm and 400 nm.[Citation15,Citation16]
Amino acid composition: 500 mg of each fraction was digested with 20 mL of 6 M HCl in sealed ampoules at 110°C for 22 h. One milliliter of hydrolyzate was drain to remove acid in vacuum and redissolved with 0.02 M HCl. After being added with 500 µl of 1 M triethylamine acetonitrile and 500 µl of 0.1 M phenyl acetonitrile isothiocyanate for 1 h at room temperature, 4 ml of n-hexane was added and mixed up. 10 min later, the substratum was filtered through a 0.2 μm membrane filter and subjected to RP-HPLC analysis.[Citation17]
Determination of the molecular weight: The molecular weight distribution profile was determined according to the method of Zhuang,[Citation18] using a gel permeation chromatograph (GPC) system (Viscotek TDA305max, Malvern Instruments Ltd., UK) equipped with an SRT SEC-300 column. 100 µL of sample (1 mg/mL) was loaded and determination was carried out at 30°C with 0.05 M PB and 0.05 M NaCl at a flow rate of 0.5 mL/min. Polyethylene glycol (PEG) with different polymerization degree were used as standards. Empower software was used to calculate the weight-average molecular weight (Mw).
Antioxidant activity in vitro
DPPH• scavenging activity: The radical scavenging activities on DPPH• of two peptides were detected by the method of Barkia.[Citation19] The concentrations of SWP-I and SWP-II were designed to be 0.25, 1.0 5.0, 10.0, and 20.0 mg/mL. Besides, the same concentrations of glutathione (GSH) were detected as positive controls.
HO• scavenging activity: Fenton’s reaction was used to estimate the scavenging ability against HO• in accordance with the method of Liang.[Citation20] The concentrations of peptides and positive control (GSH) were set as above.
O2-• scavenging activity: O2-• scavenging activity was assayed by the method as reported.[Citation21] The concentrations of peptides and positive control (GSH) were set as above.
Fe2+ chelating ability: The abilities of two peptides to chelate Fe2+ were determined according to the method of Gao.[Citation22] The concentrations of peptides were set as above and the same concentrations of edetate disodium (EDTA-Na2) were detected as positive controls.
Anti-aging activity on 2BS induced by H2O2
Cell culture: 2BS cells were cultured in a humidified atmosphere of 5% CO2 at 37°C in MEM NEAA medium containing 10% fetal bovine serum (FBS), 1% penicillin G and 1% streptomycin. The experimental cells were at passage numbers between ten and twenty. Cells with healthy morphology and high growth potential with approximately 70–80% confluence were used for the experiments.
Cell viability: Cck-8 (Cell Counting Kit-8) assay was used to measure the cell viability. 2BS cells were seeded into 96-well plates at a density of 2 × 105 cells/mL and then grouped for 24 h. After being subjected to different treatments, 10 µL of cck-8 was added and the cells were incubated for 120 min at 37°C. The absorbance was detected with an enzyme calibrator at 450 nm. Cell viability = (A sample/A blank) × 100%. Four wells were used for each concentration.[Citation23]
Intracellular ROS content: Cells were treated as above and then grouped for 24 h after the addition of different concentrations of SWP-I and SWP-II (100 µg/mL, 400 µg/mL). Meanwhile, the normal blank group and aging control group were set as control. The cells were washed twice with PBS, and 10 µL of fluorescent probe supporting liquid 2,7-dichlorodihydrofluorescein diacetate (DCFH-DA, 10 mM) was then added. After incubation at 37°C for 30 min, intracellular ROS was measured using an automated live cell imager[Citation24] (Biotek, Winooski, VT, USA) and the excitation and emission wavelengths were set of 485 and 528 nm. The fluorescence images were observed and captured.
Senescence-associated beta-galactosidase (SA-β-gal) staining: Cells were pretreated as above. Firstly the cells were fixed with 3.7% paraformaldehyde to for 15 min at room temperature and then stained with X-gal solution for 48 h at 37°C. The cells were washed with PBS and observed with standard light microscopy (Nikon, Tokyo, Japan). The senescent cells were stained blue and the results were presented as percentage of positively stained cells in five random fields.[Citation25]
Apoptosis analysis: After being washed with PBS, the cells were digested with trypsin enzyme and collected by centrifuging for 5 min at 1000 × g. Then the cells were suspended at a density of 1 × 106 cells/mL and stained with Annexin V and PI (propidium iodide) for 10 min in the dark. The apoptosis rates were detected using a flow cytometer[Citation26] (Beckman Cyto FLEX Flow cytometer, Beckman Coulter, Brea, CA, USA).
Statistical analysis
The data were performed as mean ± SD and statistically analyzed using a paired T-test.
RESULTS AND DISCUSSION
Isolation and purification of peptides
Gel filtration chromatography is a traditional and effective method to isolate different macromolecules according to their size, based on the sequence of their elution sequence.[Citation27] This method also allows salts, which may have been added during pretreatment, to be removed. After enzymatic hydrolysis of protemax, the protein hydrolyzates are mainly composed of undigested proteins.[Citation28] The isolation of crude protein hydrolyzates on Sephadex G-15 is shown in . The SWPs were separated into four fractions and two major parts, named SWP-I and SWP-II, which were separately collected and freeze-dried to be used in the following research.
Figure 1. Elution profile of SWP on the G-15. The column was eluted with distilled water at a flow rate of 1.2 mL/min.
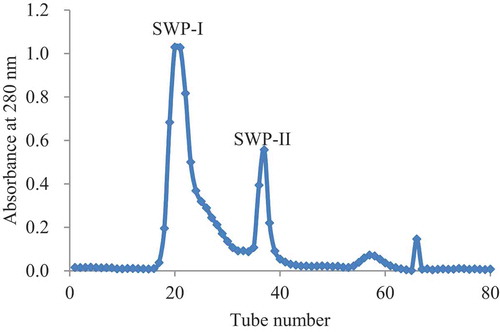
Characterization of peptides
Fourier transforming infrared spectroscopy (FT-IR): The FT-IR spectra of SWP-I and SWP-II were measured to characterize the peptide fractions and the results re shown in . The FT-IR spectra of SWP-I and SWP-II were distinguishable with each other in the intensity of bonds. The strong absorption peaks of the two peptides at 3312 cm−1 were ascribed to the stretching vibrations of the O-H and N-H bonds. The commonly absorptive peak at 2924 cm−1 was attributed the asymmetrical stretch of CH2.[Citation29]The characteristic peaks at 2359 cm−1 and 2341 cm−1 indicate the presence of S-H, due to the amino acid methionine. The strong stretching vibration at 1661 cm−1 contributed to the carboxylic acid groups.[Citation30] The stretching vibrations at 1080 cm−1 corresponded to absorption of C-OH side groups, indicating a hexatomic ring unit. Compared with SWP-I, SWP-II lacked an absorptive peak at 3069 cm−1, 1544 cm−1, and 1456 cm−1 which indicated the stretching vibrations of = C-H bonds, N-O bonds, and C-H bonds. Meanwhile, the absorptive stretching at 1032 cm−1 of SWP-II, corresponded to the side groups of C-OH was stronger than that of SWP-I.[Citation31] Therefore, the two fractions showed some differences in their structures.
Figure 2. FT-IR spectrum of SWP-I and SWP-II. SWP-I and SWP-II were mixed with KBr powder, ground thoroughly and then pressed into a 1 mm pellet for FT-IR measurement.
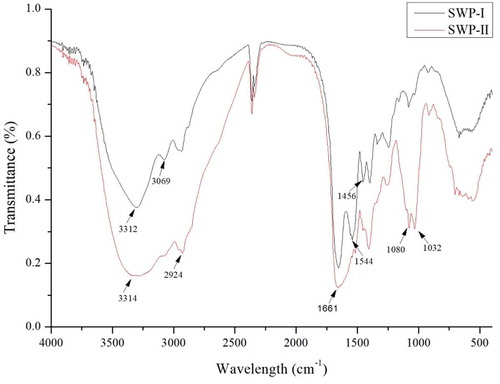
UV scanning spectroscopy: A property of protein is that it absorbs ultraviolet light over a range of wavelengths due to aromatic amino acids producing different UV spectra with their different chromophores.[Citation32] The structure of peptide linkage leads to absorption at 200–220 nm, while the presence of the conjugate double bonds of tryptophan, tyrosine, and other aromatic amino acids, leads to absorption at 280 nm.[Citation33] The UV light absorption spectrum of peptides is shown in . This showed that SWP-I and SWP-II displayed strong absorption at 220 nm, which was consistent with the typical properties of protein. The absorption at 280 nm of SWP-II was stronger than of SWP-I, indicating that the content of aromatic amino acids in SWP-II was higher than in SWP-I.
Figure 3. UV light absorption spectrum of SWP-I and SWP-II between 190 nm and 400 nm on a UV spectrophotometer.
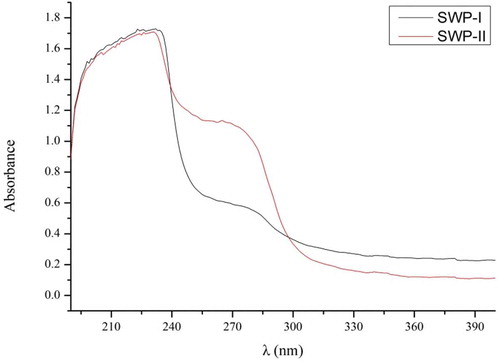
Amino acid composition: The bioactivities of peptides were mainly related to the composition of its amino acids and their R groups. Previous study showed that the presence of aromatic amino acids including phenylalanine and tyrosine played important roles in the potency of antioxidant peptides, as tyrosine is thought to act as a hydrogen donor.[Citation34] In addition, histidine has been shown to possess strong radical scavenging properties as a result of chelating, lipid trapping, and decomposition of the imidazole ring.[Citation35] Accordingly, the amino acid profiles of the fractions were detected. The results in showed that although the two fractions mainly consisted of Asp, Glu, Gly, Ala, and Pro, but there were still some differences in amino acid compositions between them, for example the content of hydrophobic amino acids in SWP-II were higher than that in SWP-I. Consistent with the results of UV light absorption spectroscopy, the content of aromatic amino acids, including Tyr and Phe in SWP-II were far higher than those in SWP-I. The differences on amino acids composition between these peptides might contribute to the differences in their antioxidant activities.
Table 1. Amino acid composition of SWP-I and SWP-II (g/100 g).
Molecular weight: GPC has been an effective method to detect the molecular weight of peptide.[Citation36] Different molecular weights of the samples in the column were detected according to different retention time. The results of two parts in showed that SWP-I possessed wider peak than SWP-II and the dispersion indexes of them were 1.70 and 1.11 separately. It also showed that SWP-II had higher purity than SWP-I. The retention time of the two components was 18.87 min and 20.82 min, and the weight-average molecular weight of SWP-I and SWP-II were calculated to be 4976 Da and 1960 Da, respectively.
Antioxidant activity in vitro
To assess the antioxidant activities of two fractions, the scavenging rates of DPPH•, HO•, and O2-• and chelating rate of Fe2+ were detected through chemical methods. DPPH• is a kind of stable free radicals with a nitrogen center which has maximum absorption occurs at 517 nm and a noticeable color change means that it can be detected visually during the reaction process to obtain the required initial amount of free radicals.[Citation37] The results in A showed that at a concentration of 5 mg/mL, both peptides possessed DPPH• scavenging activities in a dose-dependent manner and their radical scavenging activities were 54.69% and 83.05%, respectively. Compared with these two fractions, the positive control of GSH showed stronger DPPH• scavenging activity and its scavenging rate was 97.17% when the concentration was as low as 1 mg/mL.
Figure 5. (A) DPPH•, (B) HO•, (C) O2-• scavenging activity, and (D) Fe2+ chelating ability of SWP-I and SWP-II. Data are presented as mean ± SEM n = 3.
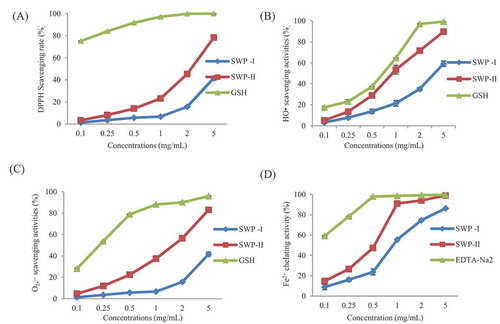
Among all of the reactive oxygen species, the hydrogen radical causes the strongest oxidation and causes enormous biological damage by reacting with amino acids, DNA, and membrane components. However, the hydrogen radical has a high reactivity but a very short life span. The detection of the scavenging activities of HO• is based on the principle of the Fenton reaction.[Citation38] The scavenging rates of different concentrations of two fractions are shown in B. It can be seen that both of two peptides exhibited a concentration-dependent hydroxyl radical scavenging activity. At the same concentration, the scavenging ability of SWP-II appeared to be much higher than of SWP-I and was close to that of GSH. The scavenging rate of SWP-II was 53.6% at a concentration of 1 mg/mL and was 21.4% of SWP-I at the same concentration. The scavenging ability of HO• was consistent with that of DPPH• and the results showed that scavenging activities on free radicals in vitro were related to the molecular weights of peptides.
Superoxide radicals can be generated through numerous biological reactions. If not cleared in time, redundant radicals could be accumulated and cause damage to the organism.[Citation39] The results in C showed that both swim bladder hydrolyzates possessed antioxidant activities for O2-•. At a concentration of 2 mg/mL, the scavenging rate of SWP-II was 56.5%, which was almost four times than that of SWP-I. As a positive control, GSH could scavenge O2-• effectively and the scavenging rate was 78.8% at a concentration of 0.5 mg/mL.
The increasing amount of ferrous ion could accelerate the formation of superoxide anions and hydroxyl groups. Therefore, the ferrous ion chelating agent possessed the ability to prevent or reduce the negative effects of Fe2+, and slow down the oxidation process.[Citation34] The chelating effects on Fe2+ are presented in D. The relationship between chelating ability and concentration was shown to be dose-dependent. The Fe2+ chelating rates of SWP-I, SWP-II, and EDTA-Na2 were 23.8%, 47.5%, and 98.0%, respectively, at a concentration of 0.5 mg/mL. Due to the presence of the imidazole group, the histidine at the N-terminus played an important role in metal ion chelation.[Citation40] Similar results were reported that peptides of lower molecular weight possessed stronger abilities on scavenging free radicals and chelating metal ions than those of a higher molecular weight.[Citation9] The reason for this could be that peptides with lower molecular weight might have more effective interactions with radicals relating to the oxidization process.[Citation41]
Cell viability
It has been reported that cells induced with H2O2 undergo premature senescence.[Citation42] This leads to reduced cell viability and cell damage. It has been previously shown that human embryonic lung diploid fibroblasts cells can be used to establish models of stress-induced premature senescence.[Citation43] In order to select the proper concentration of H2O2, the viabilities of cells added with different concentrations of H2O2 (0, 0.1, 0.2, 0.5, 1.0, and 2.0 mmol/L) were detected. The histogram in showed that cell viability was 80.1% and 59.9% at H2O2 concentrations of 0.2 mM and 0.5 mM, respectively. Therefore, 0.2 mM of H2O2 was used to establish the premature senescence model. The results also showed that hydrogen peroxide may have bidirectional effects: promoting growth at low concentrations and inhibiting proliferation at high concentrations. A similar phenomenon was observed by Wang,[Citation44] who showed that the damage caused by hydrogen peroxide to cells depends on the type of cells and the induction dose.
Cells were cultured with different concentrations of SWP-I and SWP-II (100 µg/mL and 400 µg/mL). 2 h after being induced with 0.2 mM H2O2 (except in the normal control group), the cells were replaced with normal culture medium. Results in showed that the viability of cells stimulated by H2O2 (control group) decreased significantly (p < .01) versus normal cells (blank group). Compared with cells of control group, treatment of 400 µg/mL of SWP-I and 100 µg/mL of SWP-II provided remarkable protection on cell viability (p < .05). The results revealed that SWP-I and SWP-II reduced cytotoxicity and exhibited protective effects against H2O2-induced oxidative stress.
Figure 7. Effects of SWP-I and SWP-II on cell viability. Data are shown as mean± SEM, n = 4. ** p < .01 versus the blank group, ## p < .01 versus the control group.
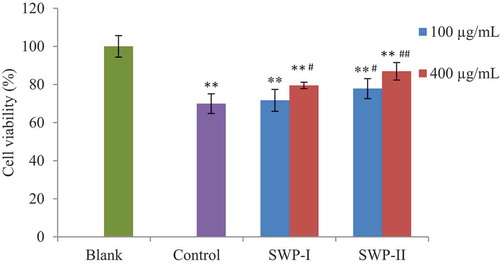
Intracellular ROS content
Intracellular generation of ROS was detected as previously described, by labeling with the fluorescence probe, DCFH-DA. Nonpolar DCFH-DA could cross the cell membrane and the more polar DCFH is formed after the probe being hydrolyzed by cellular esterase. Then the fluorescent DCF was generated by oxidation of intracellular free radicals.[Citation34] To confirm whether the peptides fractions could reduce oxidative stress, the fluorescence intensities of DCF were detected. As shown in (a), ROS generation increased significantly in 2BS cells after treatment with H2O2. In the fluorescence images of (b), it was obvious that in H2O2-treated control group, the amounts of fluorescent cells and their fluorescence intensities were enhanced, while pretreatment with both peptides produced a rapid decrease in intracellular oxidant levels. At a concentration of 100 µg/mL, SWP-II strongly decreased the fluorescent intensity to 7344 (p< .01), which was better than SWP-I at the same concentration (p< .05). Treatment with SWP-I and SWP-II of a higher concentration (400 µg/mL) exerted considerable radical scavenging abilities (p< .01, p< .01). A Similar study reported that natural compounds confer protection on oxidative stress-induced fibroblasts.[Citation45] Our results showed that both fractions of cod swim bladder might suppress oxidative stress induced by H2O2 through scavenging reactive oxygen radicals.
Figure 8. Effects of SWP-I and SWP-II on H2O2-induced ROS generation of 2BS cells. (a) Fluorescence intensities of DCF due to oxidation of DCFH by cellular ROS were detected (Ex = 485 nm, Em = 535 nm). Data are shown as mean± SEM, n = 4. ** p < .01 versus the blank group, ## p < .01 versus the control group. (b) Representative fluorescence images illustrating the increasing fluorescence intensity of DCF produced by ROS in H2O2-treated cells and ROS scavenging abilities of the two fractions.
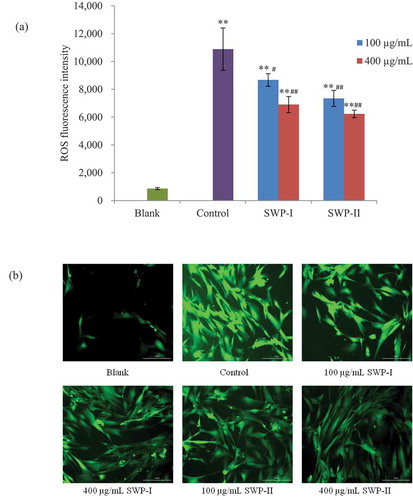
Senescence-associated beta-galactosidase (SA-β-gal) staining
The presence of SA-β-gal activity is a common biomarker to assess cellular senescence.[Citation46] Staining with X-Gal, a blue product is produced after being catalyzed by galactosidase, allowing easy identification of the affected cells using an optical microscope. In our study, this assay is used to detect and quantify the SA-β-gal present in stress-induced senescent cells.[Citation47] To determine the effects of SWP-I and SWP-II on H2O2-induced 2BS cells, the phenotypic observations associated with senescence were investigated and the ratios of SA-β-gal positive cells were calculated. The results in showed that stimulation with 0.2 mM H2O2 induced SA-β-gal activity and that the percentage of senescent cells increased to 54.8%. The cells pretreated with the two peptides exhibited reductions in positive cells to some degree when compared with cells of control group. Pretreatments with 400 µg/mL of SWP-I and both concentrations of SWP-II significantly inhibited SA-β-gal activity in H2O2-induced 2BS cells (p< .01). These results demonstrated that SWP-I and SWP-II exerted effective inhibition of premature senescence induced by hydrogen peroxide.
Figure 9. Inhibitory effects of SWP-I and SWP-II on oxidative stress-induced premature senescence in 2BS cells. (a) The ratios of SA-β-gal positive cells were assessed using a paired T-test. Data are shown as mean± SEM, n = 4. ** p < .01 versus the blank group, ## p < .01 versus the control group. (b) Morphological changes of 2BS cells after staining with SA-β- Gal were observed by optical microscopy. Magnification: 100 ×.
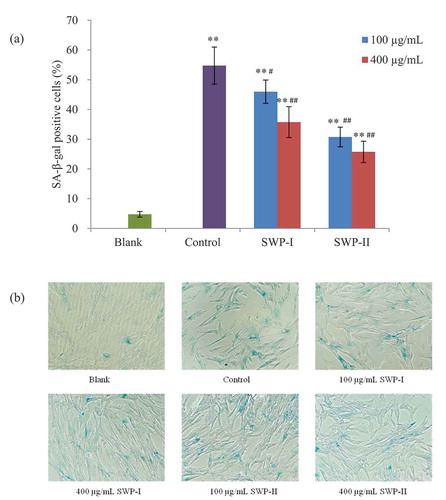
Apoptosis analysis
Apoptosis is a mechanism of physiological cell death which is essential to development and tissue homeostasis in multicellular organisms.[Citation48] Recently, resistance to cell apoptosis has been described in cellular senescence.[Citation49] The most common method for detecting the apoptosis rate of cells is to stain with fluorescent dyes such as acridine orange (AO) and sodium iodide (PI), which can distinguish and identify the viable, apoptotic, and necrotic cell populations. Annexin V (AV) is a natural phospholipid with a high affinity for phosphatidylserine, which is transferred from the inner surface of the cell membrane to the outer surface during the early phase of apoptosis.[Citation50] Therefore, the combination of PI and FITC-AV could be used to induce red fluorescence in the membranes of damaged cells to identify living cells (Q1-LL in ), necrotic cells (Q1-UL in ), late apoptotic cells (Q1-UR in ), and early apoptotic cells (Q1-LR in ). In this study, apoptotic cells staining with annexin V and PI were detected by flow cytometry to investigate whether the two fractions have cytoprotective effects against H2O2-induced cell damage. It was clear that the apoptosis rate of cells (the sum of cells in early and late apoptosis) stimulated with H2O2 increased significantly from 3.37% to 20.39% (p < .01). However, pretreatment with the two peptides inhibited H2O2-induced cell apoptosis. In addition, the percentage of viable cells decreased in a concentration-dependent manner. A conclusion could be drawn that the two novel peptides might alleviated oxidative damage and protect 2BS cells against H2O2.
Figure 10. Protective effects of SWP-I and SWP-II on H2O2-induced cell apoptosis. (a) Cell apoptosis rates of 2BS were assessed by annexin V and PI double staining and flow cytometry. Data are shown as mean± SEM, n = 4. ** p < .01 versus the blank group, ## p < .01 versus the control group. (b) Scatter diagram of cell apoptosis by flow cytometry.
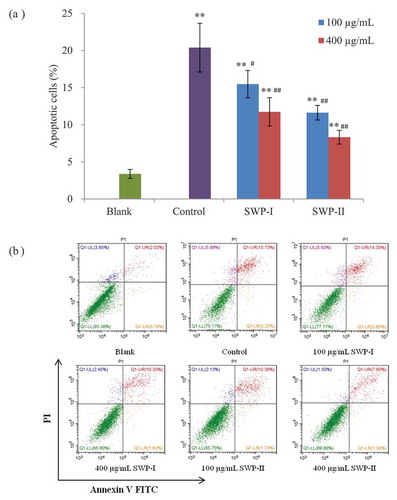
CONCLUSION
Two bioactive peptides, SWP-I and SWP-II, were isolated through the enzymatic hydrolysis of swim bladders from Atlantic cod (Gadus morhua). The characterization of the two peptides demonstrated the differences between them. In vitro assays of their antioxidant activities revealed that SWP-I and SWP-II have the ability to scavenge DPPH•, HO•, and O2-•, and have the ability to chelate metal ions. In addition, both peptides reduced H2O2-induced cellular damage and intracellular reactive oxygen species production in 2BS cells. In conclusion, the study provides evidence that enzymatic peptides of swim bladders from Atlantic cod (Gadus morhua) have potent antioxidant and anti-aging activities and could serve as potential ingredients in the food industry for attenuating aging and preventing age-related diseases in humans.
Disclosure statement
We declare that we have no financial or personal relationships with other people or organizations that would inappropriately influence our work.
Additional information
Funding
References
- Tong, T. J.; Zhang, Z. Y. Mechanisms of Aging and Its Theories. Progress in Physiological Sciences. 2007, 38(1), 14–18.
- Denham, H.;. Aging: A Theory Based on Free Radical and Radiation Chemistry. J. Geront.. 1956, 11, 298–300.
- Forman, H. J.;. Redox Signaling: An Evolution from Free Radicals to Aging. Free Radical Biol. Med. 2016, 97, 398–407.
- Zhu, S. Y.; Jiang, N.; Tu, J.; Yang, J.; Zhou, Y. Antioxidant and Anti-aging Activities of Silybum Marianum Protein Hydrolysate in Mice Treated with D-galactose. Biomed. Environ. Sci. 2017, 30(9), 623–631.
- Li, S. S.; Liu, M.; Zhang, C.; Tian, C. Y.; Wang, X. X.; Song, X. L.; … Jia, L. Purification, in Vitro Antioxidant and in Vivo Anti-aging Activities of Soluble Polysaccharides by Enzyme-assisted Extraction from Agaricus Bisporus. Int. J. Biol. Macromol. 2018, 109, 457–466.
- Sun, Y. X.; Kennedy, J. F. Antioxidant Activities of Different Polysaccharide Conjugates (Crps) Isolated from the Fruiting Bodies of Chroogomphis Rutilus (Schaeff.: Fr.) O. K. Miller. Carbohydr. Polym. 2010, 82(2), 510–514.
- Ding, Q. Y.; Yang, D.; Zhang, W. N.; Lu, Y. M.; Zhang, M. Z.; Wang, L. M.; … Chen, Y. Antioxidant and Anti-aging Activities of the Polysaccharide TLH-3 from Tricholoma Lobayense. Int. J. Biol. Macromol. 2016, 85, 133–140.
- Fan, L. H.; Zou, S. Q.; Ge, H. Y.; Xiao, Y.; Wen, H. G.; Feng, H.; … Nie, M. Preparation and Characterization of Hydroxypropyl Chitosan Modified with Collagen Peptide. Int. J. Biol. Macromol. 2016, 93, 636–643.
- Chi, C. F.; Wang, B.; Hu, F. Y.; Wang, Y. M.; Zhang, B.; Deng, S. G.; Wu, C. W. Purification and Identification of Three Novel Antioxidant Peptides from Protein Hydrolysate of Bluefin Leatherjacket (Navodon Septentrionalis) Skin. Food Res. Int. 2015, 73, 124–129.
- Kanwate, B. W.; Ballari, R. V.; Kudre, T. G. Influence of Spray-drying, Freeze-drying and Vacuum-drying on Physicochemical and Functional Properties of Gelatin from Labeo Rohita Swim Bladder. Int. J. Biol. Macromol. 2018. DOI: 10.1016/j.ijbiomac.2018.10.015.
- Chang, H.; Duan, Z. H.; Cheng, Z. Y.; Yang, Y. Study on Antioxidant Peptide Prepared by Enzymolysis of Swim Bladder Protein. J. Anhui Agri Sci. 2012, 40(6), 3389–3391.
- Shavandi, A.; Hu, Z. H.; Teh, S. S.; Zhao, J.; Carne, A.; Bechit, A.; Bechit, A. E. A. Antioxidant and Functional Properties of Protein Hydrolysates Obtained from Squid Pen Chitosan Extraction Effluent. Food Chem. 2017, 227, 194–201.
- Song, R.; Zhang, K. Q.; Wei, R. B. In Vitro Antioxidative Activities of Squid (Ommastrephes Bartrami) Viscera Autolysates and Identification of Active Peptides. Process Biochem. 2016, 51, 1674–1682.
- Li, N.; Shen, X. R.; Liu, Y. M.; Zhang, J. L.; He, Y.; Liu, Q.; … Li, K. X. Isolation, Characterization, and Radiation Protection of Sipunculus Nudus L. Polysaccharide. Int. J. Biol. Macromol. 2016, 83, 288–296.
- Ye, M.; Guo, G. Y.; Lu, Y.; Song, S.; Wang, H. Y.; Yang, L. Purification, Structure and Anti-radiation Activity of Melanin from Lachnum YM404. Int. J. Biol. Macromol. 2018, 63, 170–176.
- Xiong, Q. P.; Li, X.; Zhou, R. Z.; Hao, H. R.; Li, S. L.; Jing, Y.; … Shi, Y. Y. Extraction, Characterization and Antioxidant Activities of Polysaccharides from E. Corneum Gigeriae Galli. Carbohydr. Polym. 2014, 108, 247–256.
- Zou, Z. P.; Wang, M. J.; Wang, Z. G.; Aluko, R. E.; He, R. Antihypertensive and Antioxidant Activities of Enzymatic Wheat Bran Protein Hydrolysates. J. Food Biochem. 2019. DOI: 10.1111/jfbc.13090.
- Zhuang, Y. L.; Sun, L. P.; Zhao, X.; Wu, J. F.; Hou, H.; Li, B. F. Antioxidant and Melanogenesis-inhibitory Activities of Collagen Peptide from Jellyfish (Rhopilema Esculentum). J. Sci. Food Agric. 2009, 89, 1722–1727.
- Barkia, I.; Al-Haj, L.; Hamid, A. A.; Zakaria, M.; Saari, N.; Zadjali, F. Indigenous Marine Diatoms as Novel Sources of Bioactive Peptides with Antihypertensive and Antioxidant Properties. Int. J. Biol. Macromol. 2019, 54, 1514–1522.
- Liang, L. L.; Wang, C.; Li, S. G.; Chu, X. M.; Sun, K. L. Nutritional Compositions of Indian Moringa Oleifera Seed and Antioxidant Activity of Its Polypeptides. Food Sci. Nutr. 2019. DOI: 10.1002/fsn3.1015.
- Chen, Y. X.; Liu, X. Y.; Wu, L. X.; Tong, A. J.; Zhao, L. N.; Liu, B.; Zhao, C. Physicochemical Characterization of Polysaccharides from Chlorella Pyrenoidosa and Its Anti-ageing Effects in Drosophila melanogaster[J]. Carbohydr. Polym. 2018, 185, 120–126.
- Gao, J.; Zhang, T.; Jin, Z. Y.; Xu, X. M.; Wang, J. H.; Zha, X. Q.; Chen, H. Q. Structural Characterisation, Physicochemical Properties and Antioxidant Activity of Polysaccharide from Lilium Lancifolium Thunb. Food Chem. 2015, 169, 430–438.
- Zhou, Z. D.; Huang, Y.; Liang, J. T.; Ou, M. L.; Chen, J. J.; Li, G. Y. Extraction, Purification and Anti-radiation Activity of Persimmon Tannin from Diospyros Kaki L.f. J. Environ. Radioact. 2016, 162-163, 182–188.
- Chatzigeorgiou, S.; Thai, Q. D.; Tchoumtchoua, J.; Tallas, K.; Tsakiri, E. N.; Papassoderi, I.; … Trougakos, I. P. Isolation of Natural Products with Anti-ageing Activity from the Fruits of Platanus Orientalis. Phytomedicine. 2017, 33, 53–61.
- Kim, D. B.; Shin, G. H.; Kim, J. M.; Kim, Y. H.; Lee, J. H.; Lee, J. S.; … Lee, O. H. Antioxidant and Anti-ageing Activities of Citrus-based Juice Mixture. Food Chem. 2016, 194, 920–927.
- Han, L. Z.; Tang, L.; Jiang, Z.; Jiang, Y. Q. Enhanced Radiosensitization of Human Glioblastoma Multiforme Cells with Phosphorylated Peptides Derived from Gli2. Neuropeptides. 2018. DOI: 10.1016/j.npep.2018.05.009.
- You, L. J.; Zhao, M. M.; Regenstein, J. M.; Ren, J. Y. Purification and Identification of Antioxidative Peptides from Loach (Misgurnus Anguillicaudatus) Protein Hydrolysate by Consecutive Chromatography and Electrospray Ionization-mass Spectrometry. Food Res. Int. 2010, 43, 1167–1173.
- Chalamaiah, M.; Jyothirmayi, T.; Bhaskachary, K.; Vajreswari, A.; Hemalatha, R.; Kumar, B. D. Chemical Composition, Molecular Mass Distribution and Antioxidant Capacity of Rohu (Labeo Rohita) Roe (Egg) Protein Hydrolysates Prepared by Gastrointestinal Proteases. Food Res. Int. 2013, 52(1), 221–229.
- Muyonga, J. H.; Cole, C. G. B.; Duodu, K. G. Characterisation of Acid Soluble Collagen from Skins of Young and Adult Nile Perch (Lates Niloticus). Food Chem. 2004, 85, 81–89.
- Wang, X. M.; Zhang, Z. S.; Zhao, M. X. Carboxymethylation of Polysaccharides from Tremella Fuciformis for Antioxidant and Moisture-preserving Activities. Int. J. Biol. Macromol. 2015, 72, 526–530.
- Zhao, T.; Mao, G. H.; Feng, W. W.; Mao, R. W.; Gu, X. Y.; Li, T.; … Wu, X. Y. Isolation, Characterization and Antioxidant Activity of Polysaccharide from Schisandra sphenanthera[J]. Carbohydr. Polym. 2014, 105, 26–33.
- Cai, X. X.; Lin, J. P.; Wang, S. Y. Novel Peptide with Specific Calcium-Binding Capacity from Schizochytrium Sp. Protein Hydrolysates and Calcium Bioavailability in Caco-2 Cells. Marine.Drugs. 2017, 15(3). DOI: 10.3390/md15010003.
- Aitken, A.; Learmonth, M. Protein Determination by UV Absorption. In The Protein Protocols Handbook; Walker, J. M., Ed.; Humana Press: Totowa, NJ, USA, 1996; pp 3–6.
- Shi, Y. N.; Kovacs-Nolan, J.; Jiang, B.; Tsao, R.; Mine, Y. Antioxidant Activity of Enzymatic Hydrolysates from Eggshell Membrane Proteins and Its Protective Capacity in Human Intestinal Epithelial Caco-2 Cells. J. Funct. Foods. 2014, 10, 35–45.
- Farvin, K. H. S.; Andersen, L. L.; Otte, J.; Nielsen, H. H.; Jessen, F.; Jacobsen, C. Antioxidant Activity of Cod (Gadus Morhua) Protein Hydrolysates: Fractionation and Characterization of Peptide Fractions. Food Chem. 2016, 204, 409–419.
- Li, J. E.; Nie, S. P.; Xie, M. Y.; Li, C. Isolation and Partial Characterization of a Neutral Polysaccharide from Mosla Chinensis Maxim. Cv. Jiangxiangru and Its Antioxidant and Immunomodulatory Activities. J. Funct. Foods. 2014, 6, 410–418.
- Saidi, S.; Deratani, A.; Belleville, M. P.; Amar, R. B. Antioxidant Properties of Peptide Fractions from Tuna Dark Muscle Protein By-product Hydrolysate Produced by Membrane Fractionation Process. Food Res. Int. 2014, 65, 329–336.
- Chung, S. K.; Osawa, T.; Kawakishi, S. Hydroxyl Radical Scavenging Effects of Spices and Scavengers from Brown Mustard (Brassica Nigra). Biosci. Biotechnol. Biochem. 1997, 61(1), 118–123.
- Rossi, M., .; Caruso, F.; Antonioletti, R.; Viglianti, A.; Traversi, G.; Leone, S.; … Cozzi, R. Scavenging of Hydroxyl Radical by Resveratrol and Related Natural Stilbenes after Hydrogen Peroxide Attack on DNA. Chem.-Biol. Interact. 2013, 206, 175–185.
- Rajapakse, N.; Mendis, E.; Jung, W.-Y.; Je, J.-Y.; Kim, S.-K. Purification of a Radical Scavenging Peptide from Fermented Mussel Sauce and Its Antioxidant Properties. Food Res. Int. 2005, 38, 175–182.
- He, R.; Girgih, A. T.; Malomo, S. A.; Ju, X.; Aluko, R. E. Antioxidant Activities of Enzymatic Rapeseed Protein Hydrolysates and the Membrane Ultrafiltration Fractions. J. Funct. Foods. 2013, 5(1), 219–227.
- Zhang, Z. S.; Wang, X. M.; Su, H. L.; Pan, Y. L.; Han, J. F.; Zhang, T. S.; Mao, G. X. Effect of Sulfated Galactan from Porphyra Haitanensis on H2O2-induced Premature Senescence in WI-38 Cells. Int. J. Biol. Macromol. 2018, 106, 1235–1239.
- Tang, W. Z.; Wang, Y. A.; Gao, T. Y.; Wang, X. J.; Zhao, Y. X. Identification of C-geranylated Flavonoids from Paulownia Atalpifolia Gong Tong Fruits by HPLC-DAD-ESI-MS/MS and Their Anti-aging Effects on 2BS Cells Induced by H2O2. Chin. J. Nat. Med. 2017, 15(5), 0384–0391.
- Wang, C.; Tang, X. L.; Chen, K. M.; Zhang, L.; Liu, N. W.; Zhao, J. The Effect of Puerarin on the Damage of Osteoblasts Induced by Hydrogen Peroxide. Chin. J. Osteo. 2017, 23(11), 1442–1450.
- Kutuk, O.; Adli, M.; Poli, G. Resveratrol Protects against 4-HNE Induced Oxidative Stress and Apoptosis in Swiss 3T3 Fibroblasts. Biofactors. 2004, 20, 1–10.
- Storer, M.; Mas, A.; Robert-Moreno, A.; Pecoraro, M.; Ortells, M. C.; Giacomo, V. D.; … Keyes, W. M. Senescence Is a Developmental Mechanism that Contributes to Embryonic Growth and Patterning. Cell. 2013, 155, 1119–1130.
- Bassaneze, V.; Miyakawa, A. A.; Krieger, J. E. A Quantitative Chemiluminescent Method for Studying Replicative and Stress-induced Premature Senescence in Cell Cultures. Anal. Biochem. 2008, 372, 198–203.
- Arur, S.; Uche, U. E.; Rezaul, K.; Fong, M.; Scranton, V.; Cowan, A. E.; … Han, D. K. Annexin I Is an Endogenous Ligand that Mediates Apoptotic Cell Engulfment. Dev. Cell. 2003, 4, 587–598.
- Nguyen, H. Q.; To, N. H.; Zadigue, P.; Kerbrat, S.; Taille, A. D. L.; Gouvello, S. L.; Belacemi, Y. Ionizing Radiation-induced Cellular Senescence Promotes Tissue Fibrosis after Radiotherapy. A review[J]. Crit. Rev. Oncol./hematol. 2018, 129, 13–26.
- Ishaque, A.; Al-Rubeai, M. Use of Intracellular pH and annexin-V Flow Cytometric Assays to Monitor Apoptosis and Its Suppression by Bcl-2 Over-expression in Hybridoma Cell Culture. J. Immunol. Methods. 1998, 22, 43–57.