ABSTRACT
The ascomycete Cordyceps genus has been used as valued traditional Chinese medicine and functional food. Solid-state fermentation (SSF) of soybean, chickpea, black bean, and mung bean with Cordyceps militaris was performed for 35 days at 22°C, and then the biomass, total phenolic content (TPC), and total flavonoid content (TFC) increased significantly with the extension of fermentation time. The cordycepin content in four SSF-beans was as high as 8.33, 11.12, 10.43, and 6.64 mg/g, respectively. Moreover, the fermentation improved the 1,1′-diphenyl-2- picrylhydrazyl (DPPH) and 2,2′-azino-bis (3-ethylbenzothiazoline-6-sulfonate) (ABTs) radical scavenging capacity, ferric reducing antioxidant power (FRAP) and ACE inhibition ability. Overall, the findings of the study stated clearly that SSF of beans with C. militaris was a potentially promising way to enhance the nutritional, physico-chemical, and biological properties of the substrate. Cordyceps-fermented products may serve as a novel valuable nutritional and functional food in health industry.
Introduction
Leguminosae is one of the most important groups of Dicotyledonae, and the second worldwide food crops, after cereals. As seeds of the plants which have a wide range of adaptability in terms of climate and soil conditions, there are more than 1300 legume species globally, and about 20 are consumed by humans. Soybean is the main legume crop with a production of 33.36 million tons in 2019.[Citation1] While similar with soybean, chickpea, black bean, and mung bean, which are also widely cultivated legumes in the world with high yield, contain elevated levels of healthy functional components and are the main component of vegetarianism and important supplement to meat diet. As the traditional crop with thousands of years of edible history, beans are recognized as nutritious food with health-promoting properties recently due to the consumers’ awareness of the nutritional and functional values. Legumes are unique sources of proteins, low glycemic index (GI), carbohydrates, dietary fiber, minerals, vitamins, phenolic acids, as well as flavonoids, etc.,[Citation2–4] which contribute to balanced daily diets, and possesses some bio-functionalities such as anti-inflammatory, antioxidant, antidiabetic, antihypertensive, and antiproliferative,[Citation5] especially on certain types of cancers,[Citation6] cardiovascular diseases,[Citation7] osteoporosis,[Citation8] and menopausal symptoms.[Citation9] Among the phytochemicals in legumes of greatest interest, the changes of composition and abundance in gut microbiota caused by polyphenols and oligosaccharides are considered to be related to human health.[Citation10]
Solid-state fermentation (SSF) is defined as an economically viable fermentation process in which microorganisms grow on crops to biotransform solid materials for secondary metabolites, for nutritional enrichment, which involves traditionally natural fermentation in limited moisture levels in food products.[Citation11–13] In China, Japan, Indonesia, and other East and Southeast Asian countries, solid-state fermentation technology has long been used in the fermentation of legume products. Famous flavor foods, such as douchi, natto, miso, soy sauce, sufu, tempeh, and so on,[Citation14–16] have a long history of consumption using different types of microorganisms. Bacteria such as Bacillus subtilis, Bacillus natto, and Lactobacillus plantarum, filamentous fungi such as Aspergillus awamori, Aspergillus sojae, Mucor sufu, Aspergillus niger, Monascus purpureus, Rhizopus oligosporus, and Rhizopus nigricans are proved as GRAS (Generally recognized as safe) food-grade microorganism, which have been used in SSF technologies in the production of traditional fermented beans food.[Citation17–20]
Macrofungi, known as mushrooms, have been extensively used as foods, nutraceuticals, and medicines since time immemorial.[Citation21] The edible and medicinal mushrooms are also recognized as one of the most important food supplements. The genus Cordyceps, including more than 500 species, which are distributed in many regions of the world, but mainly found in East Asia, Europe, and North America, belongs to the entomopathogenic fungi, Clavicipitaceae, and Ascomycotina.[Citation22] Cordyceps militaris and Ophiocordyceps sinensis (syn. Cordyceps sinensis) have been used as traditional Chinese medicine (TCM) for hundreds of years, due to the broad spectrum of pharmacological activity.[Citation23] A commercial product (trade name Cikaria), derived from C. cicadae, is sold as health supplement in Sweden.[Citation24,Citation25] Active compounds (e.g., cordycepin, Cordyceps polysaccharides, cordycepic acid, cordymin) isolated from the Cordyceps fungus exhibit a variety of pharmacological effects, including antitumor, antioxidant, anti-inflammatory, immunomodulatory, and anti-microbial activities.[Citation26–28]
As the type species of the genus Cordyceps, C. militaris has been authorized as a new resources food[Citation29] and novel food source.[Citation30] Until now, there is limited information on the use of macrofungi (edible and medicinal mushroom) as a fermentation strain in SSF systems of beans. Therefore, this study sought to evaluate the suitability of soybean, chickpea, black bean, and mung bean as the substrate for SSF on cordycepin, phenolic and flavonoid content, antioxidant activity (specifically DPPH and ABTs radical scavenging effects, ferric reducing antioxidant capacity), and angiotensin I-converting enzyme inhibition with C. militaris. In addition, the aims of the present paper are also to confirm the potential of C. militaris-legumes co-cultures used to be the functional food.
Materials and methods
Microorganism and materials
The anamorph strain JY20 of C. militaris, originally isolated from fresh natural specimen, was confirmed by means of both morphological and molecular methods, and conserved in potato dextrose agar (PDA) slant. The spore suspension (approximately 108–109 spores/ml) was inoculated into 1000 ml PDA liquid medium and incubated at 22°C and 150 rpm for 6 days as seed culture broth. Soybean, chickpea, black bean, and mung bean were purchased from Inzone supermarket of Jinan, Shandong province, China.
Cordycepin, angiotensin I-converting enzyme, and hippuryl-l-histidyl-L-leucine (HHL) were obtained from Sigma Chemical Co. (St. Louis, MO, USA). Total antioxidant capacity assay kits (ABTS method) and (FRAP method) were purchased from Nanjing Jiancheng Bioengineering Institute (Nanjing, Jiangsu province, China). HPLC grade methanol and acetonitrile were procured from Fisher Scientific (Massachusetts, USA). All other chemicals and solvents were of analytical reagent grade and acquired from Sinopharm Chemical Reagent Co., Ltd. (Shanghai, China). High purity deionized water with a resistivity of 18.2 MΩ cm−1was obtained using a Millipore Synergy water purification system with SynergyPak purification cartridges (Billerica, MA, USA).
SSF of beans and biomass measurement
The dry beans (15 g) were soaked with distilled water at 1:2 solid-to-liquid ratio in 500-mL glass jars for 24 h at room temperature. After soaking, the legumes were sterilized by autoclaving at 121°C for 20 min. The cooled steamed beans were inoculated with seed culture broth of C. militaris (5 mL for every 15 g dry legumes) and incubated at 22°C for 35 days. The same procedure was applied to a control test without fungal inoculation. For further analysis, fermented and non-fermented beans were lyophilized and shattered to powder (60 meshes), sealed and stored at −20°C.
The biomass of C. militaris during the fermentation process was indirectly estimated by the glucosamine content referring to the method of [Citation11]. The glucosamine content was assayed spectrophotometrically with Ehrlich's reagent at 530 nm, using a standard curve of glucosamine in water (0.1–0.4 mg/mL). The amount of glucosamine in fermented legumes was subtracted from that of the unfermented legumes, and the biomass of the fermented legumes was expressed as mg glucosamine/g legumes in dry base.
Ethanol extraction
The extraction of lyophilized SSF powder (1 g) was carried out three times, each time with 10-fold solvents of ethanol (80%) under ultrasound at 50°C for 30 min. The extract was centrifuged at 5,000 rpm for 20 min at 4°C. The combined supernatants were evaporated at 50°C under reduced pressure to remove ethanol, and then re-dissolved in 10 mL of 80% ethanol, and stored in the dark at 4°C for further analysis. All the extractions were performed in triplicate.
Total phenolic content (TPC)
TPC in ethanol extracts was determined using the Folin–Ciocalteu method as described by Liu et al.[Citation31] with minor modifications. Briefly, 200 μL of extracts were mixed with 2.3 mL of deionized water, 0.5 mL of Folin-Ciocalteu’s reagent, and 2 mL of 7.5% sodium carbonate solution. Samples were mixed and then incubated in darkness at room temperature for 2 h. The absorbance of the mixtures at 760 nm was recorded. A standard curve was plotted using gallic acid (40–200 μg·mL−1) as a standard. Samples were independently analyzed in triplicate, and results were expressed as milligrams of gallic acid equivalents per gram of dry weight (mg GAE/g d.w.).
Total flavonoid content (TFC)
Total flavonoid content was measured referring to the method of Juan and Chou.[Citation17] Extracts or standard solutions (250 μL) were mixed with distilled water (1.25 mL) and 5% NaNO2 solution (75 μL). After standing for 6 min, the mixture was combined with 10% Al(NO3)3 solution (150 μL) for 5 min, and then 0.5 mL of NaOH (1 M) and 275 μL distilled water was added to the mixture. The absorbance of the solutions was measured at 510 nm after reaction for 15 min. Rutin was used to calculate the total flavonoid content, the determined results were expressed as milligrams of rutin equivalent per gram of dry weight (mg RUT/g d.w.).
Content of cordycepin
High performance liquid chromatography (HPLC) was employed to determine the content changes of cordycepin in SSF of legumes. The 80% ethanol extracts were filtered through a 0.22 μm nylon membrane and analyzed by Agilent 1100 HPLC system (Agilent, Santa Clara, CA, USA) with a Agilent TC-C18 column (250 mm × 4.6 mm i.d., 5 μm) at 260 nm and at a column temperature of 25°C. The mobile phase, a solution of H2O (containing 0.1% trifluoroacetic acid): acetonitrile = 92:8 (v/v), was eluted at a flow rate of 0.8 mL/min. Identification of the target compound cordycepin was based on comparison with the standard. The determined results of cordycepin were expressed as milligrams per gram of dry weight legumes (mg/g d.w.)
Antioxidant activities
The capacity of SSF ethanol extracts to scavenge the DPPH stable free radical was measured using the modification method of Blois.[Citation32] 0.05 mL sample solution was mixed with equivalent 50% ethanol containing 0.1 mM DPPH. The mixture was shaken vigorously and incubated in dark at room temperature for 30 min, and the absorbance at 517 nm was measured using the Model 550 Microplate Reader (Bio-Rad, Hercules, CA, USA). Vitamin C (Vc) was used as a positive control. The DPPH scavenging rate (R) was calculated as the following formula, R (%) = [1-(As-Ai)/A0] ×100. Where A0 is the absorbance of the incubation DPPH solution without the tested sample; As is the absorbance of the incubation mixture containing both the sample and DPPH, and sample reference solution, recorded as Ai, is the absorbance of the blank solution without DPPH. All tests were performed in triplicate, and the mean of Abs was used in the equation above.
According to the product operation manual, ABTS radical cation (ABTS·+) scavenging activity of SSF sample was evaluated with a total antioxidant capacity assay kit (ABTS method) with minor alteration. A 10 μL of SSF-bean samples were added in a 96-well plate to react with 190 μL of the ABTS·+ work solution (containing 20 μL peroxidase solution) at room temperature for 6 min, and then the absorbance was recorded at 405 nm with an Enspire multilabel Reader (PerkinElmer, Turku, Finland). Vc and water were used as positive control and blank control. The calculation formula of scavenging rate (R) was the same as that of DPPH method.
The ferric-reducing power of antioxidants against oxidative effects was measured with a total antioxidant capacity assay kit (FRAP method) with minor modification. A 5 μL of SSF-bean samples were allowed to react with 180 μL of the freshly prepared FRAP work solution in a 96-well plate at 37°C for 3–5 min in the dark. Colorimetric readings of the product Ferrous tripyridyltriazine complex were measured at 593 nm using an Enspire multilabel Reader (PerkinElmer, Turku, Finland). A higher absorbance indicates a higher ferric reducing power. Experiments were performed in triplicate and compared with the positive Vc control. The measurements were compared with a calibration curve of ferrous sulfate solution, and the final results were expressed as millimoles of Fe (II) equivalents per milliliter (mmol Fe (II)/ml).
ACE inhibition activity
One gram lyophilized SSF flour was mixed with 40 folds of Na2CO3 buffer (0.05 M, pH 10). The mixture was homogenized for 1 h at room temperature and centrifuged at 10,000 g for 10 min. The supernatant was filtered through 0.22 μm filter, lyophilized, and dissolved in 2 ml 0.1 M boric acid buffer (containing 0.3 M NaCl, pH 8.3) for the following analysis.
ACE inhibition activity assay was performed according to the method of,[Citation33]by monitoring the formation of hippuric acid (HA) from Hippuryl-His-Leu (HHL) as substrate using HPLC. In brief, 80 μL sample solution of different concentrations was added to 200 μL containing 5 mM HHL, and then pre-incubated for 5 min at 37°C. HHL were prepared in 100 mM borate buffer, pH 8.3, containing 300 mM NaCl. The reactions were then initiated by adding 20 μL of 0.1 U/mL ACE prepared in the same buffer and incubated for 30 min at 37°C. The enzymatic reaction was terminated by the addition of 250 μL of 1.0 M HCl, and then HA peak was detected at 228 nm and at a column temperature of 20°C. The mobile phase, a solution of methanol: water = 50:50 (v/v) with 0.1% TFA, was eluted at a flow rate of 0.8 mL/min. ACE-inhibitory activity was calculated using the following equation: ACE inhibition (%) = [(B-A)/(B-C)] ×100. Where B was the absorbance of the control in which 80 μL of borate buffer was added instead of the sample, A was the absorbance of the test sample, and C was the absorbance of the blank which was absent of ACE.
Results and discussion
Biomass, content of total phenolic and flavonoids
Due to the difficulty of separating fungal cells from the substrate, it is hard and complicated to assess fungal biomass. As an alternative, the determination of ergosterol[Citation34] and/or NAGA[Citation35] was used as an indirect means of fungus hyphal growth. The glucosamine could be produced as a reducing sugar by enzymatic hydrolysis, so fungal biomass was estimated by measuring glucosamine in the solid substrate and recalculated to dry biomass concentration. During the fermentation process, the mycelium of C. militaris began to expand on the second day, and the whole culture medium was almost full on the fifth to sixth day. After a week of fermentation, the mycelia began to turn yellow under the stimulation of light. With the increase of fermentation time, the color of mycelia gradually darkened, and the thickness of aerial mycelia also increased. At about the 4th week of fermentation, small amount of Cordyceps stroma began to sprout on legume culture media. The growth of C. militaris for 35 days was determined by measuring the content of glucosamine in SSF of legumes. As shown in , all legume medium of SSF represented the same trend in biomass formation. In the early stage of fermentation, the mycelium biomass increased rapidly, and after 15 days, glucosamine content steadily increased during the SSF. At the end of fermentation, the net increment of glucosamine in medium of black soybean, soybean, chickpea, and mung bean were 19.08, 26.84, 22.30, and 25.30 mg/g, respectively.
Figure 1. The total phenolic content and biomass of SSF-beans. (A) Black bean; (B) soybean; (C) chickpea; (D) mung bean, N represented non-fermented beans. Values are means ± SD (n = 3).
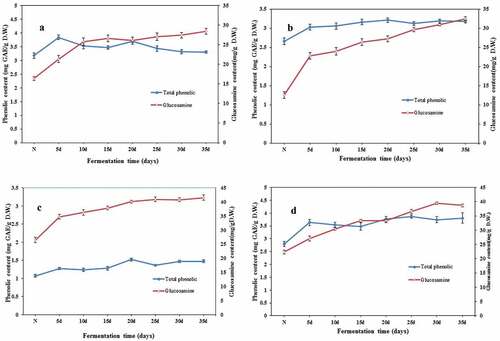
Correspondingly, the TPC content of legumes fermented with C. militaris increased in the first 5 days and tended to be stable with the extension of time, showing the final values of 4.08 percentage point increase for black bean (from 3.18 mg/g to 3.31 mg/g DW), 20.00 percentage point increase for soybean (from 2.65 mg/g to 3.18 mg/g DW), 37.38 percentage point increase for chickpea (from 1.07 mg/g to 1.47 mg/g DW), and 36.07 percentage point increase for mung bean (from 2.80 mg/g to 3.81 mg/g DW) in relation to unfermented substrates. As one kind of secondary metabolites, phenolic compounds are generally categorized as phenolic acids, flavonoids, et al.[Citation36] Phenolic acids exist in three forms: soluble free acids, soluble conjugated, and insoluble bound states that are covalently bound (ester- and etherified) to cell wall structural component. Previous studies reported that SSF served as a good strategy to assist in converting bound to free phenolics, thus increasing their bioavailability.[Citation37] The microorganism-derived enzymes play an important role in the mobilization of phenolic compounds.[Citation38–41] The present results show that TPC increased with time of fermentation during fungal bio-processing. This may be due to the proteolytic activities of C. militaris contributing to the release of bonds to free TPC. Generally speaking, SSF is a viable technique useful for enhancing the nutrient quality of agro-industrial by-products and for producing bioactive products. The liquid fermentation process of macrofungi was short (3–7 days), only mycelia were formed, and therefore, there were fewer secondary metabolites. The period of solid-state fermentation was relatively long (15 days to several months), and fruiting bodies were produced, resulting in a large number of polyphenols. In our follow-up experiments to study the effect of Cordyceps genus and fermentation conditions, the improvement of individual phenolic compounds by SSF (data not shown) also confirmed the above finding, which was consistent with the conclusion of previous papers.
Flavonoids have been proposed to act as beneficial agents in sub-health and multiple disease states.[Citation42,Citation43] Generally speaking, no single solvent can extract all the active components with different polarity and solubility, and in most cases, different organic solvents are used to extract flavonoids and phenolic acid compounds in plant materials.[Citation28,Citation44] In the present study, the TFC in SSF of beans extracted with 80% ethanol reveal a similar tendency with regard to the TPC. As shown in and , after 5 days of SSF, both fermented black bean and mung bean showed a substantial increase in flavonoid contents until the maximum productions were reached of 1.44 and 1.51 mg/g, respectively. However, TFC of soybean reached the highest yield of 0.67 mg/g after 15 days of fermentation, from the initial level of 0.35 mg/g ( (A)). After that, the amount of flavonoids began to decline, at the end of SSF, TFC of black bean and mung bean were almost equivalent to the initial levels of non-fermented controls, and the flavonoid content of soybean remained at a high level showing 47.22 percentage point increase from 0.36 to 0.53 mg/g DW. It is speculated that the flavonoids produced during the fermentation of C. militaris may only be intermediate products of metabolic pathway, and then converted to other substances.
Figure 2. Evaluation of total flavonoids content and biomass of SSF-beans. (A) Black bean; (B) soybean; (C) mung bean, N represented non-fermented beans. Values are means ± SD (n = 3).
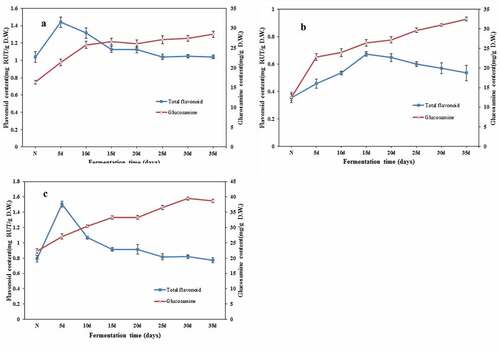
It should be pointed out that the content of flavonoids in chickpea or chickpea ferment extracted by ethanol was very low, even could not be detected by colorimetric method. The results indicated that fermentation of C. militaris may have no effect on the polarity of flavonoids in chickpea. It may suggest us that in the follow-up study, we should consider different fermentation fungi or replacing extraction solvents. Comprehensive consideration to the changes of biomass, TPC, and TFC, the fermentation end point for the subsequent determination of cordycepin content, antioxidant activity, and ACE inhibition was 30 days.
Content of cordycepin
Cordycepin (3´-deoxyadenosine), a metabolic product isolated from C. militaris,[Citation45] is the main functional component and has been reported to exert anti-tumor activity in a broad spectrum of cancer types. Anticancer mechanism of cordycepin has been found to inhibit cell growth by inducing apoptosis and autophagy,[Citation46] it is also reported that cordycepin restrains transcriptional factor nuclear factor kappa B (NF-κB) activity and mammalian target of rapamycin (mTOR) signaling.[Citation47] Cordycepin also has a wide range of other physiological functions, including inhibition of vascular endothelial smooth muscle cell proliferation,[Citation48] anti-ischemia-reperfusion injury,[Citation49] anti-platelet aggregation,[Citation50] et al.
Due to the potential medicinal usage and commercial value of cordycepin, many attempts, such as biosynthetic pathway analysis,[Citation51,Citation52] microbial breeding,[Citation53] medium optimization, and growth kinetics [Citation5,Citation54] have been made to develop the efficient cultivation process for improving the biomass of fruiting body or mycelia and cordycepin production by C. militaris and C. kyushuensis. shows that after 30 days of fermentation, the amount of cordycepin in four SSF-beans (black bean, soybean, chickpea, and mung bean) were 8.33, 11.12, 10.43, and 6.64 mg/g, respectively, while no cordycepin was detected in unfermented beans. In general, most of the commercial fruiting body of C. militaris was obtained by culturing with rice or wheat for about 2 months, and cordycepin content was less than 1 mg/g, while cordycepin in the mycelia cultured by liquid submerged fermentation was even lower. The cordycepin quantity of bean samples were effectively increased by fermentation, SSF of soybean showed the highest cordycepin content (11.12 mg/g), even the lowest yield of mung bean products, was still more than six times the commercial Cordyceps after only 30 days. The finding demonstrated that it was a feasible and successful method to obtain cordycepin from SSF of beans by the fungus C. militaris.
Antioxidant activities
DPPH radical scavenging activity: DPPH radical methods were often conducted to evaluate the free radical scavenging ability of natural compounds.[Citation55]As a stable free radical, DPPH showed the maximum absorption at 517 nm with violet color due to its odd electron. When DPPH encountered antioxidant scavengers, the resulting decolorization was stoichiometric with respect to the ability to bleach the DPPH radical. The antioxidant activities by DPPH of SSF-beans with C. militaris are presented in . The scavenging effect increased with increasing fermentation time, ranged approximately from 4.59% to 25.56%, 2.33% to 27.52%, 1.50% to 47.14%, and 3.53% to 36.32% in SSF of black bean, soybean, chickpea, and mung bean, respectively (). The highest scavenging activities of the samples were obtained when fermentation was carried out for 30 days.
Figure 4. Evaluation of DPPH radical scavenging activity of SSF-beans. (A) With different fermentation time, N represented non-fermented beans; (B) with different concentration. Values are means ± SD (n = 3).
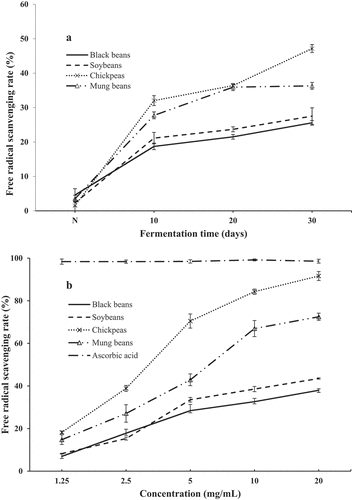
The bean ferments of the 30th day were collected and extracted by 80% ethanol to detect the antioxidant activity at different final concentrations (1.25, 2.5, 5, 10, and 20 mg/mL). As the results shown in , all the samples showed a good scavenging effect against DPPH radical in a dose-dependent manner at each concentration level. The scavenging ratios at the highest concentration of chickpea and mung bean were 91.64% and 71.50%, respectively. The present study demonstrated that the antioxidants in SSF of beans could inhibit the formation of various radicals or react with the radicals to turn them into less detrimental or harmless products, thereby breaking the radical chain reaction. Meanwhile, an interesting fact was that TPC of chickpea was the lowest among the four fermented legumes, and its TFC could not even be detected, but chickpea ferment had the highest antioxidant activity, which was almost similar to that of the positive control Vc at the concentration of 20 mg/mL. There was no significant correlation between phenolic (flavonoid) content and antioxidant activity in fermented chickpea. Wade and Tucker [Citation56] reported that some amino acids, such as valine, lysine, glycine, and histidine, could show antioxidative activity. Wali et al.[Citation57] found a novel antioxidant peptide from chickpea sprout protein hydrolysates, which exhibited high DPPH and hydroxyl radical scavenging activities.,[Citation58]reported that SSF process by using Bacillus subtilis improved the proteolysis and the antioxidative activity (DPPH) of chickpeas. Our research corresponded with the previous studies and proved that the fungal fermentation is indeed a complex metabolic process, the higher antioxidant activities in chickpea fermented by C. militaris may be attributed to the nature of proteolytic products released and to other non-phenolic compounds with antioxidant activities.
ABTS radical cation scavenging activity: The ABTS solution possesses a distinctly blue green color, which disappears quickly in the presence of antioxidants. The antioxidant activities of fermented legumes with different fermentation time and product concentration were detected by ABTS method, and the results are represented in. The scavenging effect increased steadily with the extension of fermentation time, the clearance rate of four kinds of bean ferments was similar after 20 days, and reached the maximum of approximately 84% on the 30th day of fermentation ().
Figure 5. Evaluation of ABTS radical scavenging activity of SSF-beans. (A) With different fermentation time, N represented non-fermented beans; (B) with different concentration. Values are means ± SD (n = 3).
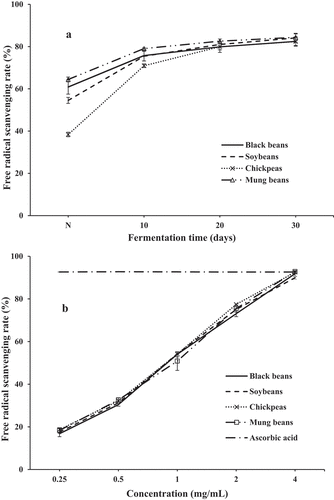
The bean ferments of the 30th day were extracted by 80% ethanol to assay the scavenging activity of ABTS radical at different final concentrations (0.25, 0.5, 1, 2, and 4 mg/mL). As shown in , similar to DPPH radical scavenging activity, all the samples showed a good scavenging effect in a dose-dependent manner. The highest ABTS scavenging activity was observed at the concentration of 4 mg/mL, ranged of 91.83%, 89.94%, 92.53%, and 92.65% in SSF of black bean, soybean, chickpea, and mung bean, respectively. The highest antioxidant activities of all four bean ferments were almost correspond to that of the positive reference of Vc. The fact clearly demonstrated that SSF with C. militaris enhanced the ABTS scavenging activity of beans.
Ferric-reducing antioxidant power: In the FRAP assay, the antioxidants present in extracts acted as oxidants and were estimated from the ability to reduce 2,4,6-tripyridyl-s-triazine (TPTZ)-Fe3+ to TPTZ-Fe2+ complex. The reduction formed an intense blue color, which could be measured at wavelength of 593 nm. The absorbance clearly increased due to formation of the Fe2+-TPTZ complex, indicating a higher ferric reducing power. The trends for the antioxidant activity of SSF-beans extracts by FRAP method are shown in . At the 30th day of fermentation, the reducing power of chickpea ferment was 3.89 mM/mL, whereas those of black bean, soybean, and mung bean were 2.81, 2.28, and 3.39 mM/mL, respectively (). reveals the dose-response curves for the reducing powers. The ferric reducing capacity was effective in the order: chickpea > mung bean > black bean > soybean at 20 mg/mL. Vc at 1.25 mg/mL showed a high reducing ability of 11.00 mM/mL Fe2+ concentration. The results demonstrated that the extracts possessed hydrogen-donating capacity indicating the significant reducing power of SSF of legumes.
ACE inhibition activity
As a dipeptidyl carboxypeptidase, angiotensin I-converting enzyme plays an important role in human Renin-Angiotensin-Aldosterone System and converts angiotensin I (decapeptide) into angiotensin II (octapeptide).[Citation59] Due to the important function in regulation of blood pressure, the inhibition of ACE had been widely used in scientific research and clinical treatment of hypertension. ACE-inhibition activity is an in vitro indicator of the antihypertensive properties of functional food products.[Citation60] The content of HA released from HHL by the action of ACE was measured to evaluate the ACE inhibition[Citation61] activity of the test sample. Lower content of liberated HA signified a stronger ACE inhibition. Several reports had documented ACE inhibition peptides derived from SSF of beans. The ACE inhibitory activities of Monascus fermented soybean,[Citation62] Bacillus subtilis fermented lentil,[Citation63] Bacillus spp. fermented black soybeans,[Citation64] Cordyceps militaris fermented red bean [Citation64]), and Rhizopus oligosporus fermented black common bean seeds[Citation65] were reported, and the results showed that the ACE inhibitory effect was different for the variant materials and microorganisms. Fermented bean products, such as natto (B. subtilis fermented soybean) and Monascus-fermented soybean, are used as traditional functional food and regarded as the important source of ACE inhibition peptides.
The fermentation process markedly increased the ACE inhibition of all legumes. SSF of legumes with C. militaris showed 2.14–4.22 folds inhibitory activity after different ferment time when compared with the non-fermented beans (). SSF of black bean had the best effect, followed by soybean and chickpea, mung beans showed the lowest effect. It was worth noted that the activity of 10-day ferment was almost the same as that of 20-day and 30-day ferment. The results suggested that short-term fermentation of beans with C. militaris may achieve satisfactory effects considering the cost-effectiveness ratio. Further investigations are needed to characterize the bioactive compounds in fermented legumes that show ACE-inhibitory action.
Table 1. The ACE inhibition rate of SSF-beans with different fermentation time, N represented non-fermented beans. Values are means ± SD (n = 3)
Conclusion
SSF was regarded as the effective bioprocess to enhance the antioxidant activities and ACE inhibition activity with beans as the substrate by C. militaris, a food-grade fungus. The subsequent fermentation further increased the contents of cordycepin, TPC, and TFC. Based on the present findings, SSF of beans with C. militaris was expected to broaden our understanding to be potential antioxidants and nutraceuticals as well as functional foods. Overall, the data from the study may provide useful information on the utilization of macrofungus fermentation to enhance the bio-functional activity of various bean by-products.
Disclosure statement
No potential conflict of interest was reported by the author(s).
Additional information
Funding
References
- FAOSTAT (2021). Retrieve from Feb 17, 2021: http://www.fao.org/faostat/en/#data/QC.
- Hall, C.; Hillen, C.; Garden Robinson, J. Composition, Nutritional Value, and Health Benefits of Pulses. Cereal Chem. 2017, 94, 11–31. DOI: 10.1094/CCHEM-03-16-0069-FI.
- Kutoš, T.; Golob, T.; Kač, M.; Plestenjak, A. Dietary Fibre Content of Dry and Processed Beans. Food Chem. 2003, 80(2), 231–235. DOI: 10.1016/S0308-8146(02)00258-3.
- Yu, Z.; Fan, Y. S.; Wang, X. W.; Xia, M.; Cai, Y. In Vitro and in Vivo Digestibility of Pea and Chickpea Powder Prepared by Cooking and Drying Treatment. Int. J. Food Prop. 2020, 23(1), 1187–1199. DOI: 10.1080/10942912.2020.1792925.
- Tang, D.; Dong, Y.; Guo, N.; Li, L.; Ren, H. Metabolomic Analysis of the Polyphenols in Germinating Mung Beans (Vigna radiata) Seeds and Sprouts. J. Agric. Food Chem. 2014, 94, 1639–1647. DOI: 10.1002/jsfa.6471.
- Nagata, C.; Mizoue, T.; Tanaka, K.; Tsuji, I.; Tamakoshi, A.; Matsuo, K.; Wakai, K.; Inoue, M.; Tsugane, S.; Sasazuki, S., et al. Soy Intake and Breast Cancer Risk: An Evaluation Based on a Systematic Review of Epidemiologic Evidence among the Japanese Population. Japanese J. Clin. Oncol. 2014, 44(3), 282–295. DOI: 10.1093/jjco/hyt203.
- Li, S. H.; Liu, X. X.; Bai, Y. Y.; Wang, X. J.; Sun, K.;Chen, J. Z.; Hui, R.T. Effect of Oral Isoflavone Supplementation on Vascular Endothelial Function in Postmenopausal Women: A Meta-analysis of Randomized Placebo-controlled Trials. Am. J. Clin. Nutr. 2010, 91(2), 480–486. DOI: 10.3945/ajcn.2009.28203.
- Alekel, D. L.; Van Loan, M. D.; Koehler, K. J.; Hanson, L. N.; Stewart, J. W.; Hanson, K. B., Kurzer, M.S.; Peterson, C.T. The Soy Isoflavones for Reducing Bone Loss (SIRBL) Study: A 3-y Randomized Controlled Trial in Postmenopausal Women. Am. J. Clin. Nutr. 2010, 91(1), 218–230. DOI: 10.3945/ajcn.2009.28306.
- Taku, K.; Melby, M. K.; Kronenberg, F.; Kurzer, M. S.; Messina, M. Extracted or Synthesized Soybean Isoflavones Reduce Menopausal Hot Flash Frequency and Severity: Systematic Review and Meta-analysis of Randomized Controlled Trials. Menopause. 2012, 19(7), 776–790. DOI: 10.1097/gme.0b013e3182410159.
- Ma, G.; Chen, Y. Polyphenol Supplementation Benefits Human Health via Gut Microbiota: A Systematic Review via Meta-analysis. J. Funct. Foods. 2020, 66, 103829. DOI: 10.1016/j.jff.2020.103829.
- Krishna, C.;. Solid-state Fermentation Systems-an Overview. Crit. Rev. Biotechnol. 2005, 25(1–2), 1–30. DOI: 10.1080/07388550590925383.
- Olukomaiya, O. O.; Adiamo, O. Q.; Fernando, W. C.; Mereddy, R.; Li, X. H.; Sultanbawa, Y. Effect of Solid-state Fermentation on Proximate Composition, Anti-nutritional Factor, Microbiological and Functional Properties of Lupin Flour. Food Chem. 2020a, 315, 126238. DOI: 10.1016/j.food.chem.2020.126238.
- Olukomaiya, O. O.; Fernando, W. C.; Mereddy, R.; Li, X. H.; Sultanbawa, Y. Physicochemical, Microbiological and Functional Properties of Camelina Meal Fermented in Solid-state Using Food Grade Aspergillus Fungi. Fermentation. 2020b, 6, 44–59. DOI: 10.3390/fermentation6020044.
- Lan, G. Q.; Li, C. Q.; He, L. P.; Zeng, X. F.; Zhu, Q. J. Effects of Different Strains and Fermentation Method on Nattokinase Activity, Biogenic Amines, and Sensory Characteristics of Natto. J. Food Sci. Technol. 2020, 57, 4414–4423. DOI: 10.1007/s13197-020-04478-3.
- Sharma, A.;. A Review on Traditional Technology and Safety Challenges with Regard to Antinutrients in Legume Foods. J. Food Sci. Technol. 2020. DOI: 10.1007/s13197-020-04883-8.
- Wang, H. L.;. Tofu and Tempeh as Potential Protein Sources in the Western Diet. J. Am. Oil Chem. Soc. 1984, 61, 528–534. DOI: 10.1007/BF02677023.
- Juan, M. Y.; Chou, C. C. Enhancement of Antioxidant Activity, Total Phenolic and Flavonoid Content of Black Soybeans by Solid State Fermentation with Bacillus subtilis BCRC 14715. Food Microbiol. 2010, 27(5), 586–591. DOI: 10.1016/j.fm.2009.11.002.
- Polanowska, K.; Grygier, A.; Kuligowski, M.; Rudzińska, M.; Nowak, J. Effect of Tempe Fermentation by Three Different Strains of Rhizopus oligosporus on Nutritional Characteristics of Faba Beans. LWT. 2020, 122, 109024. DOI: 10.1016/j.lwt.2020.109024.
- Reyes-Bastidas, M.; Reyes-Fernandez, E. Z.; Lopez-Cervantes, J.; Milan-Carrillo, J.; Loarca-Pina, G. F.; Reyes-Moreno, C. Physicochemical, Nutritional and Antioxidant Properties of Tempeh Flour from Common Bean (Phaseolus vulgaris L.). Food Sci. Technol. Int. 2010, 16, 427–434. DOI: 10.1177/1082013210367559.
- Rui, X.; Wen, D.; Li, W.; Chen, X.; Jiang, M.; Dong, M. Enrichment of ACE Inhibitory Peptides in Navy Bean (Phaseolus vulgaris) Using Lactic Acid Bacteria. Food Funct. 2015, 6, 622–629. DOI: 10.1039/c4fo00730a.
- Friedman, M.;. Mushroom Polysaccharides: Chemistry and Antiobesity, Antidiabetes, Anticancer, and Antibiotic Properties in Cells, Rodents, and Humans. Foods. 2016, 5(4), 80–119. DOI: 10.3390/foods5040080.
- Tang, W.; Eisenbrand, G. Chinese Drugs of Plant Origin: Chemistry, Pharmacology and Use in Traditional and Modern Medicine; Springer-Verlag: Berlin Heidelberg, 1992.
- Su, J. J.; Sun, J.; Jian, T. T.; Zhang, G. Y.; Ling, J. Y. Immunomodulatory and Antioxidant Effects of Polysaccharides from the Parasitic Fungus Cordyceps kyushuensis. Biomed Res. Int. 2020, 20, 8257847. DOI: 10.1155/2020/8257847.
- Nxumalo, W.; Elateeq, A. A.; Sun, Y. F. Can Cordyceps cicadae Be Used as an Alternative to Cordyceps militaris and Cordyceps sinensis? - a Review. J. Ethnopharmacol. 2020, 257, 112879–112887. DOI: 10.1016/j.jep.2020.112879.
- Scanafarma. (2021). http://www.scanfarma.se/ciakria.html. accessed February/17/2021.
- Ahn, Y. J.; Park, S. J.; Lee, S. G.; Shin, S. C.; Choi, D. H. Cordycepin: Selective Growth Inhibitor Derived from Liquid Culture of Cordyceps militaris against Clostridium Spp. J. Agric. Food Chem. 2000, 48(7), 2744–2748. DOI: 10.1021/jf990862n.
- Zhang, S.; Hao, A. J.; Zhao, Y. X.; Zhang, X. Y.; Zhang, Y. J. Comparative Mitochondrial Genomics toward Exploring Molecular Markers in the Medicinal Fungus Cordyceps militaris. Sci. Rep. 2017, 7, 40219. DOI: 10.1038/srep40219.
- Zhao, X.; Zhang, G. Y.; Li, C. Y.; Ling, J. Y. Cordycepin and Pentostatin Biosynthesis Gene Identified through Transcriptome and Proteomics Analysis of Cordyceps kyushuensis Kob. Microbiol. Res. 2019, 218, 12–21. DOI: 10.1016/j.micres.2018.09.005.
- Ministry of Health of the People’s Republic of China. (2009). http://www.gov.cn:8080/gzdt/2009-03/18/content_1262018.htm.
- National Health Commission of the People’s Republic of China. (2014). http://www.nhc.gov.cn/sps/s7890/201406/8268613682e44b1cb2098e0b9c9143d7.
- Liu, R. H.;. Whole Grain Phytochemicals and Health. J. Cereal Sci. 2007, 46(3), 207–219. DOI: 10.1016/j.jcs.2007.06.010.
- Blois, M. S.;. Antioxidant Determinations by the Use of a Stable Free Radical. Nature. 1958, 181(4617), 1199–1200. DOI: 10.1038/1811199a0.
- Sahingil, D.; Hayaloglu, A. A.; Kirmaci, A. H.; Ozer, B.; Simsek, O. Changes of Proteolysis and angiotensin-I Converting Enzyme-inhibitory Activity in White-brined Cheese as Affected by Adjunct Culture and Ripening Temperature. J. Dairy Res. 2014, 81, 394–402. DOI: 10.1017/S0022029914000326.
- Bjurman, J.;. Ergosterol as an Indicator of Mold Growth on Wood in Relation to Culture Age. Int. Biodeterior. Biodegrad. 1994, 33(4), 355–368. DOI: 10.1016/0964-8305(94)90013-2.
- Montoya Barreto, S.; Orrego Alzate, C. E.; Levin, L. Modeling Grifola frondosa Fungal Growth during Solid-state Fermentation. Eng. Life Sci. 2011, 11(3), 316–321. DOI: 10.1002/elsc.201000087.
- Masisi, K.; Beta, T.; Moghadasian, M. H. Antioxidant Properties of Diverse Cereal Grains: A Review on in Vitro and in Vivo Studies. Food Chem. 2016, 196, 90–97. DOI: 10.1016/j.foodchem.2015.09.021.
- Dey, T. B.; Chakraborty, S.; Jain, K. K.; Sharma, A.; Kuhad, R. C. Antioxidant Phenolics and Their Microbial Production by Submerged and Solid State Fermentation Process: A Review. Trends Food Sci. Technol. 2016, 53, 60–74. DOI: 10.1016/j.tifs.2016.04.007.
- Martins, E. S.; Silva, D.; Da Silva, R.; Gomes, E. Solid State Production of Thermostable Pectinases from Thermophilic Thermoascus aurantiacus. Process Biochem. 2002, 37(9), 949–954. DOI: 10.1016/S0032-9592(01)00300-4.
- Razak, D. L. A.; Rashid, N. Y. A.; Jamaluddin, A.; Sharifudin, S. A.; Long, K. Enhancement of Phenolic Acid Content and Antioxidant Activity of Rice Bran Fermented with Rhizopus oligosporus and Monascus purpureus. Biocatal Agric. Biotechnol. 2015, 4(1), 33–38. DOI: 10.1016/j.bcab.2014.11.003.
- Schmidt, C. G.; Gonçalves, L. M.; Prietto, L.; Hackbart, H. S.; Furlong, E. B. Antioxidant Activity and Enzyme Inhibition of Phenolic Acids from Fermented Rice Bran with Fungus Rizhopus oryzae. Food Chem. 2014, 146, 371–377. DOI: 10.1016/j.foodchem.2013.09.101.
- Wu, G.; Fang, Z.; Johnson, S. K. CHAPTER 16: Sorghum Non-extractable Polyphenols: Chemistry, Extraction and Bioactivity. In Non-extractable Polyphenols and Carotenoids: Importance in Human Nutrition and Health; Eds. Saura-Calixto, F., Pérez-Jiménez, J. Roal Society of Chemistry: London, UK, 2018; pp 326–344. DOI: 10.1039/9781788013208-00326.
- Spencer, J. P. E.; Mohsen, M. M. A. E.; Rice-Evans, C. Cellular Uptake and Metabolism of Flavonoids and Their Metabolites: Implications for Their Bioactivity. Arch. Biochem. Biophys. 2004, 423(1), 148–161. DOI: 10.1016/j.abb.2003.11.010.
- Velioglu, Y. S.; Mazza, G.; Gao, L.; Oomah, B. D. Antioxidant Activity and Total Phenolics in Selected Fruits, Vegetables, and Grain Products. J. Agric. Food Chem. 1998, 46(10), 4113–4117. DOI: 10.1021/jf9801973.
- Sun, T.; Ho, C. T. Antioxidant Activities of Buckwheat Extracts. Food Chem. 2005, 90(4), 743–749. DOI: 10.1016/j.foodchem.2004.04.035.
- Cunningham, K. G.; Manson, W.; Spring, F. S.; Hutchinson, S. A. Cordycepin, a Metabolic Product Isolated from Cultures of Cordyceps militaris (Linn.) Link. Nature. 1950, 166, 949. DOI: 10.1038/166949a0.
- Yu, X. H.; Ling, J. Y.; Liu, X. F.; Guo, S.; Lin, Y. D.; Liu, X. G.;Su, L. Cordycepin Induces Autophagy-mediated c-FLIPL Degradation and Leads to Apoptosis in Human Non-small Cell Lung Cancer Cells. Oncotarget.2017, 8(4), 6691–6699. DOI: 10.18632/oncotarget.14262.
- Oh, J.; Yoon, D. H.; Shrestha, B.; Choi, H. K.; Sung, G. H. Metabolomic Profiling Reveals Enrichment of Cordycepin in Senescence Process of Cordyceps militaris Fruit Bodies. J. Microbiol. 2019, 57(1), 54–63. DOI: 10.1007/s12275-019-8486-z.
- Chang, W.; Lim, S.; Song, H.; Song, B. W.; Kim, H. J.; Cha, M. J., Sung, J.M., Kim, T.W.; Hwang, K.C. Cordycepin Inhibits Vascular Smooth Muscle Cell Proliferation. Eur. J. Pharmacol. 2008, 597(1–3), 64–69. DOI: 10.1016/j.ejphar.2008.08.030.
- Hwang, I. K.; Lim, S. S.; Yoo, K. Y.; Lee, Y. S.; Kim, H. G.; Kang, I. J.; Kwon, H.J.;Park, J.; Choi, S.Y.; Won, M.H. A Phytochemically Characterized Extract of Cordyceps militaris and Cordycepin Protect Hippocampal Neurons from Ischemic Injury in Gerbils. J. Med. Plant Nat. Prod. Res. 2008, 74(2), 114–119. DOI: 10.1055/s-2008-1034277.
- Cho, H. J.; Cho, J. Y.; Rhee, M. H.; Lim, C. R.; Park, H. J. Cordycepin (3’-deoxyadenosine) Inhibits Human Platelet Aggregation Induced by U46619, a TXA2 Analogue. J. Pharm. Pharmacol. 2006, 58(12), 1677–1682. DOI: 10.1211/jpp.58.12.0016.
- Vongsangnak, W.; Raethong, N.; Mujchariyakul, W.; Nguyen, N. N.; Leong, H. W.; Laoteng, K. Genome-scale Metabolic Network of Cordyceps militaris Useful for Comparative Analysis of Entomopathogenic Fungi. Gene. 2017, 626, 132–139. DOI: 10.1016/j.gene.2017.05.027.
- Xia, Y. L.; Luo, F. F.; Shang, Y. F.; Chen, P. L.; Lu, Y. Z.; Wang, C. S. Fungal Cordycepin Biosynthesis Is Coupled with the Production of the Safeguard Molecule Pentostatin. Cell. Chem. Biol. 2017, 24, 1479–1489. DOI: 10.1016/j.chembiol.2017.09.001.
- Wang, Y. M.; Zhang, G. Y.; Zhao, X.; Ling, J. Y. Genome Shuffling Improved the Nucleosides Production in Cordyceps kyushuensis. J. Biotechnol. 2017, 260, 42–47. DOI: 10.1016/j.jbiotec.2017.08.021.
- Wongsa, B.; Raethong, N.; Chumnanpuen, P.; Wong-ekkabut, J.; Laotenge, K.; Vongsangnak, W. Alternative Metabolic Routes in Channeling Xylose to Cordycepin Production of Cordyceps militaris Identified by Comparative Transcriptome Analysis. Genomics. 2020, 112, 629–636. DOI: 10.1016/j.ygeno.2019.04.015.
- Tang, J. P.; Liu, Y. T.; Li, Z. Optimization of Fermentation Conditions and Purification of Cordycepin from Cordyceps militaris. Prep Biochem Biotechnol. 2014, 44(1), 90–106. DOI: 10.1080/10826068.2013.833111.
- Amarowicz, R.; Pegg, R. B.; Rahimi-Moghaddam, P.; Barl, B., and Weil, J. A. Free-radical Scavenging Capacity and Antioxidant Activity of Selected Plant Species from the Canadian prairies. Food Chem. 2004, 84(4), 551–562. DOI: 10.1016/S0308-8146(03)00278-4.
- Wade, A. M.; Tucker, H. N. Antioxidant Characteristics of L-histidine. J. Nutr. Biochem. 1998, 9(6), 308–315. DOI: 10.1016/S0955-2863(98)00022-9.
- Wali, A.; Mijiti, Y.; Gao, Y. H.; Abulimiti, Y.; Haji, A. A.; Adilijiang, K. Isolation and Identification of a Novel Antioxidant Peptide from Chickpea (Cicer arietinum L.) Sprout Protein Hydrolysates. Int. J. Pept. Res. Ther. 2021, 27, 219–227. DOI: 10.1007/s10989-020-10070-2.
- Li, W.; Wang, T. Effect of Solid-state Fermentation with Bacillus subtilis Lwo on the Proteolysis and the Antioxidative Properties of Chickpeas. Int. J. Food Microbiol. 2021, 338, 108988. DOI: 10.1016/j.ijfoodmicro.2020.108988.
- Daskaya-Dikmen, C.; Yucetepe, A.; Karbancioglu-Guler, F.; Daskaya, H.; Ozcelik, B. Angiotensin-I-converting Enzyme (Ace)-inhibitory Peptides from Plants. Nutrients. 2017, 9, 316. DOI: 10.3390/nu9040316.
- Ayyash, M.; Johnson, S. K.; Liu, S. Q.; Mesmari, N.; Dahmani, S.; Dhaheri, A. S. A.; Kizhakkayil, J. In Vitro Investigation of Bioactivities of Solid-state Fermented Lupin, Quinoa and Wheat Using Lactobacillus Spp. Food Chem. 2019, 275, 50–58. DOI: 10.1016/j.foodchem.2018.09.031.
- Bei, Q.; Liu, Y.; Wang, L.; Chen, G.; Wu, Z. Q. Improving Free, Conjugated, and Bound Phenolic Fractions in Fermented Oats (Avena sativa L.) With Monascus anka and Their Antioxidant Activity. J. Funct. Foods. 2017, 32, 185–194. DOI: 10.1016/j.jff.2017.02.028.
- Pyo, Y. H.; Lee, T. C. The Potential Antioxidant Capacity and Angiotensin I-converting Enzyme Inhibitory Activity of Monascus-fermented Soybean Extracts: Evaluation of Monascus- Fermented Soybean Extracts as Multifunctional Food Additives. J. Food Sci. 2007, 72(3), S218–S223. DOI: 10.1111/j.1750-3841.2007.00312.x.
- Juan, M. Y.; Wu, C. H.; Chou, C. C. Fermentation with Bacillus Spp. A Bioprocess to Enhance Anthocyanin Content, the Angiotensin Converting Enzyme Inhibitory Effect, and the Reducing Activity of Black Soybeans. Food Microbiol. 2010, 27(7), 918–923. DOI: 10.1016/j.fm.2010.05.009.
- Rochіn-Medina, J. J.; Gutierrez-Dorado, R.; Sanchez-Magana, L. M.; Milan-Carrillo, J.; Cuevas- Rodriguez, E. O.; Mora-Rochin, S.; Valdez-Ortiz, A.;Reyes-Moreno, C. Enhancement of Nutritional Properties, and Antioxidant and Antihypertensive Potential of Black Common Bean Seeds by Optimizing the Solid-state Bioconversion Process. Int. J. Food Sci. Nutr. 2015, 66, 498–504. DOI: 10.3109/09637486.2015.1052377.