ABSTRACT
In this study, sesbania gum (SG) was successfully modified by using H2O2 as the oxidant, CuSO4 as the catalyst, and octenyl succinic anhydride as the esterification agent. The effects of oxidation and esterification on the structure and properties of SG were investigated by Fourier transform infrared spectroscopy (FTIR), X-ray diffraction (XRD), and thermogravimetric analysis (TGA), among other techniques. By FTIR analysis it was found that the oxidation did not introduce new groups, while the ester carbonyl group was successfully introduced into the molecular chain of SG. The oxidation of SG by H2O2 involves the cleavage of long chains, which is also the main cause of its reduction in viscosity. Oxidation reduced the crystallinity of SG, and its crystal structure was found to be severely damaged after esterification. Both oxidation and esterification altered the thermal properties of SG, whereby the thermal stability and freeze-thaw stability of the modified SG were improved and the expansion capacity was effectively reduced.
KEYWORDS:
Introduction
In recent years, with the rapid development of science and technology, there has been increasing research interest in natural polymers.[Citation1,] As a natural alternating copolymer, SG has been established to exhibit a similar performance and have a lower economic cost than gum Arabic and guar gum.
The molecular structure of sesbania gum (SG) is composed of a mannose backbone linked byβ-1,4 glycoside bonds and a galactose side chain connected via α − 1,6 glycosidic bonds at an approximate ratio of 2:1.[Citation3,Citation4]The molecular weight is about one-third that of guar gum.[Citation5] SG extracted from the endosperm of natural plant Sesbania cannabina is insoluble in ethanol, acetone, ether and other organic solvents but soluble in water.[Citation3,Citation6] It can be dispersed in cold water at room temperature, resulting in the formation of a highly viscous hydrosol with flocculation and sedimentation effects, strong crosslinking, extremely low toxicity, and safe to use in the food processing, chemical, petroleum, coal, and paper industries, as well as in environmental protection and other fields.[Citation7–9]
In practical applications, SG possesses the undesirable characteristics of a poor viscosity temperature, high water-insoluble content, poor electrolyte compatibility, and poor high-temperature resistance; furthermore, its swelling, hydration, and viscosity properties do not meet industrial standards.[Citation10] Therefore, it is necessary to modify it by chemical means with the help of abundant hydroxyl groups to make it appropriate for applications for various purposes. The SG is chemically modified to introduce hydrophilic groups into its molecular chains by reacting with reagents, thereby improving its hydrophilicity, accelerating its swelling rate, reducing the content of water-insoluble substances, and weakening the agglomeration between colloidal molecules.[Citation2,Citation11]Currently, the methods used for modification include oxidation,[Citation12] grafting,[Citation10] esterification,[Citation13] etherification,[Citation11] and crosslinking.[Citation14]
As indicated by numerous experiments, compared with other oxidants, the advantage of using hydrogen peroxide as an oxidant is that the generated oxides do not pollute the environment, but the caveat is that its oxidizing ability is weak. Metal ions can be added as catalysts to improve the oxidizing ability of hydrogen peroxide. Such catalysts that are commonly used for this purpose include copper sulfate, ferrous sulfate, nickel chloride, and manganese sulfate.[Citation15,Citation16] Octenyl succinate anhydride (OSA) is the most common starch modifier, containing both hydrophobic and hydrophilic groups, which generate octenyl succinate polysaccharide polymers, which exhibit hydrophilic and hydrophobic properties, and can be used as thickeners, sizing agents and microcapsule wall materials.[Citation17–19] Because SG and starch share similar physicochemical properties, SG can be modified with OSA. At present, the composite modification of oxidation and esterification is more common in starch, and there is no existing report on the composite modification of SG. In this paper, a local Sesbania cannabina in Hainan was selected, from which, SG was extracted by hot water extraction with ethanol precipitation. The SG was modified with reference to the method used for OSA starch. FTIR, XRD, DSC, and TGA were used to study the effects of oxidation and esterification on the structure of SG.
Materials and methods
Materials
Sesbania cannabina; Mass fraction 30% hydrogen peroxide, ethanol, hydrochloric acid, sodium hydroxide, copper sulfate, anhydrous sodium sulfite, sodium bisulfite are all analyzed and pure; Mass fraction of 30% hydrogen peroxide, ethanol, copper sulfate, anhydrous sodium sulfite and sodium hydroxide were purchased from Xilong Science Co., Ltd.; Ocenyl succinate anhydride purchased from Guangzhou Guangya Chemical Co., Ltd.; Sodium bisulfite was purchased from Shanghai Macklin Biochemical Co., Ltd.
Extraction of SG
SG was extracted using a slight modification of the method described by Pan Dong et al.[Citation20,Citation21] A specific amount of S. cannabina was weighed and soaked with an appropriate amount of 0.01% NaHSO3 solution for 1 h, followed by washing. The treated S. cannabina was added to distilled water in a 1:35 ratio and mashed thoroughly, followed by incubation in a 70°C underwater bath for 3 h and centrifugation at 10000 rpm for 15 min. The filtrate was concentrated at 60°C under reduced pressure to half of the original volume and supplemented with 4 times the volume of absolute ethanol, followed by refrigeration at 4°C for 12 h, and centrifugation at 80000 rpm for 10 min. SG was extracted from hot water after re-dissolution, alcohol washing, and freeze drying.
Preparation of oxidative sesbania gum (OSG)
SG (dry base) 4 g was treated and evenly dispersed with an appropriate amount of absolute ethanol, followed by the addition of 200 mL of distilled water to obtain SG slurry with a mass score of 2%. Then SG was then stirred with a magnetic stirrer until it became a homogeneous slurry. It was then heated to 40°C in a water bath. The mixture was treated with 400 µL of 2.5% CuSO4 used as a catalyst. The system pH was adjusted to 8 with a mass fraction of 5% NaOH or 5% HCl solution. Next, 2 mL of H2O2 solution was added, and the reaction was carried out in a constant temperature water bath at 40°C for 2 h. Hydrogen peroxide release was tested with potassium iodide dipsticks for complete response, to ensure the absence of reduced H2O2 treated with sodium sulfite with a mass fraction of 10%. After the reaction, the sample was precipitated with an aqueous dilute ethanol solution of 60%, alcohol-washed, freeze-dried, and crushed to produce OSG.[Citation1,Citation22]
Preparation of oxidative octenyl succinated composite-modified sesbania gum (OOSSG)
OSG (3 g) was treated with an appropriate amount of absolute ethanol in a beaker to disperse the oxide field elite glue evenly, followed by the addition of 150 mL of distilled water to obtain OSG slurry with a mass score of 2%. The resulting slurry was then stirred with a magnetic stirrer to a homogeneous slurry and heated to 40°C in the water bath. The addition of a 5% NaOH solution to adjust the system pH to 8.5. A 2 mL aliquot of OSA was added repeatedly dropwise within 2 h and the reaction was carried out in a constant temperature water bath for 4 h. During the reaction, the lye was replenished in time to maintain a constant pH of the reaction system. The reaction was neutralized and terminated with 5% HCl after a set time. The samples were precipitated with an aqueous solution of dilute ethanol at a concentration of 60%, followed by alcohol washing, freeze-drying and crushing to obtain OOSSG.[Citation23,Citation24]
Determination of freeze-thaw stability
SG and modified SG (absolutely dry) were accurately weighed to 1.0 g in a three-mouth flask, and dispersed with a small amount of ethanol, followed by the addition of distilled water to obtain a mass fraction of 1.0% at room temperature. The product was stirred with a magnetic stirrer for 30 min to ensure uniform mixture. The paste was transferred into a pre-weighed 10 mL centrifuge tube and the total mass of the paste and the centrifuge tube was weighed accurately after the addition. The mixture was frozen in a refrigerator at about −20°C. The centrifuge tube was removed after 24 h and thawed at room temperature. After 5 repetitions of freezing and thawing, the solution was centrifuged at 3000 rpm for 30 min. The supernatant was discarded and the precipitate weighed again to calculate the water evolution rate. The greater the water evolution rate, the worse is the freeze-thaw stability.[Citation8,Citation25] The water absorption rate was calculated as shown below:
water absorption rate = (G1-G2)/(G1-G0)*100%
where G0 is centrifuge barrel mass (g), G1 is sample and centrifuge mass before freezing (g), G2 is discarded supernatant after the precipitate and the centrifuge barrel mass (g).
Swelling power
A 0.25 g of the absolutely dry sample was accurately weighed in an Erlenmeyer flask, dispersed with 2 mL absolute ethanol, followed by addition of an appropriate amount of distilled water to obtain a mass fraction of 1% (calculated on a dry basis) of the paste. The mixture was agitated at 25°C for 30 min and then centrifuged at 3000 r/min for 30 min. The precipitate in the tube represents an expanded sample. The supernatant was distilled dry in a vapor bath and dried at 105°C to a constant weight for about 3 h. The water-soluble sample was obtained and weighed. The solubility and swelling power were calculated as follows.[Citation10,Citation26]
S = A/W*100
Swelling power (%) = P*100/W(100-S)
where S is the solubility (%), A is mass of the supernatant after distillation and constant weight (g), W is absolute dry sample mass (g), and P is mass of precipitate after centrifugation (g).
Characterization
FTIR spectra in the range of 4000–400 cm−1 were recorded with an IR Prestige-21 infrared spectrometer with a sample/KBr ratio of 1:150 (Shimadzu Corporation, Japan).[Citation27] XRD analysis of SG and modified SG was performed with X’Pert Pro powder X-ray diffractometer (PANalytical Co.Ltd., the Netherlands) under the following test conditions: working voltage of 40 kV, working current of 30 mA, scan speed of 8°/min, and scan range (2θ) of 5°-45°.[Citation28] The thermal analysis of SG and modified SG was carried out in a nitrogen environment using TGA Q50 V20.10 Build36 thermogravimetric analyzer under test conditions: heating rate of 10°C/min, temperature range of 20–800°C, and 60 mL/min of nitrogen.[Citation29] DSC Q20 V24.4 Build116 (TA Instruments, US) was used to perform differential scanning calorimetry at a temperature range of 10–250°C, heating rate of 30°C/min, and 50 mL/min of nitrogen in an aluminum pan.[Citation30]
Statistical analysis
Experiments were performed in triplicate, and the results were expressed as means standard deviation. The graphs were generated using OriginPro 9.5, Adobe Illustrator CS6, MetaboAnalyst 3.0, and PathVisio 3.3.0. Statistical analysis was performed using SPSS 21.0 software (IBM, Armonk, NY, USA), and the data were expressed as the mean values ± standard deviation (± s). ANOVA with a Tukey post hoc test was employed to assess the multiple comparisons.
Results and discussion
Effects of oxidation and esterification on freeze-thaw stability of SG
The effect of oxidation and esterification on the freeze-thaw stability of SG is shown in . The smaller the water absorption rate, the better the freeze-thaw stability. As can be seen from the table, the water absorption rate of SG increased after its oxidation, which indicates that oxidation reduced its freeze-thaw stability. The water absorption rate of SG was significantly reduced after esterification, which indicates that esterification can significantly improve its freeze-thaw stability. This occurs because oxidation destroys the molecular structure of SG, breaks its constituent macromolecules, and separates water molecules, thereby resulting in a decrease in the freeze-thaw stability of SG. Esterification caused a large number of octenyl long chain and ester hydrophobic groups to block the hydrogen bond formation between the natural polymer macromolecules and increases the spatial barrier, thereby trapping the water in the colloidal well and enhancing the freeze-thaw stability. The freeze-thaw stability decreased in the following order: OSSG > OOSSG > SG > OSG.
Table 1. Freeze-thaw stability of SG and modified SG.
Effects of oxidation and esterification on SG swelling power
The effects of oxidation and esterification on the swelling power of SG are shown in . It can be seen from the table that both oxidation and esterification reduced the swelling power of SG, and the swelling power of SG decreased significantly after esterification. This is attributable to oxidation destroying the molecular structure of SG and weakening its swelling capacity. The bond energy of the ester bond is stronger than that of the hydrogen bond in SG, such that the former exists between SG particles and decreases its capacity to well. The swelling power was in the following order: SG > OSG > OSSG > OOSSG.
Table 2. Swelling power of SG and modified SG.
FTIR analysis
The FTIR of SG and SG derivatives are shown in , the wave number is 3100 ~ 3500 cm−1 with a wide and strong telescopic vibration peak of hydroxyl group (-OH), which indicates widespread hydrogen bonding between molecules in the molecular structure of SG. The absence of any new functional groups were introduced into SG after oxidation. The decrease in viscosity was attributed to the rupture of the polymer chain, that is, the decrease in molecular weight. Esterification and oxidation of modified octenyl succinate ester composite resulted in new characteristic absorption peaks at 1728 cm−1 and 1573 cm−1. The absorption peaks at 1728 cm−1 and 1572 cm−1 are generated by carbon-oxygen double bond and carboxylate vibrations, which also indicates successful oxidation of the SG molecule, and introduction of ester carbonyl groups via esterification, resulting in the synthesis of a double composite-modified SG.
Effects of oxidation and esterification on the crystalline structure of SG
XRD is an effective method for detecting and analyzing the crystallinity of polysaccharide substances. The crystalline structure of the SG can be determined by observing and analyzing the morphology of the characteristic peaks. Polymer crystallization results in ordered configuration, and the greater the degree of polymer crystallinity, the more orderly is the molecular chain arrangement during the crystallization. As shown in , the peak position of SG after oxidation remained unaltered, whereas the crystallinity decreased due to oxidation following disruption of the β-1,4 glycoside bond on the SG backbone, which destroyed the structure of the SG particles in the crystalline region. Thus, the area of the amorphous region of the SG particles increased, which exposed additional hydroxyl groups and introduced disorder into the SG molecules. Therefore, oxidation reduces the crystallinity of SG. The diffraction patterns revealed spike diffraction peaks corresponding to crystalline structures, while the diffusion diffraction peaks correspond to amorphous structures. The esterification reaction seriously altered the crystalline structure of SG into an amorphous polymer.
Effect of oxidation and esterification on thermal properties of SG
The DSC curves for SG, OSG, and OOSSG are shown in . Oxidation and esterification of SG led to significant changes in the starting temperature, peak temperature, end temperature and enthalpy changes of the endothermic peak, indicating that oxidation and esterification altered the thermal properties of SG. From , after the oxidation of SG, the DSC curve shifted to the right markedly and the endothermic peaks of both OSG and OOSSG were significantly enhanced after SG was modified. The thermodynamic data for the DSC curve are shown in .
Table 3. DSC thermodynamic data of SG, OSG and OOSSG.
Oxidation of SG increased the onset, peak and end temperature, and increased enthalpy. Esterification of the OSG decreased its onset and peak temperature, and increased the end temperature and enthalpy. The larger the value of enthalpy, the greater is the energy required by the altered SG structure. This demonstrates that SG is more stable after its modification. Such a result is attributable to oxidation destroying the structure of SG molecules. However, the short-chain molecules are stabler and their structural disruption requires additional energy. Esterification enhances the thermal stability of SG and the enthalpy since the ester bonds are stronger than the hydrogen bonds.
The TGA curves for SG, OSG, and OOSSG are shown in . The decomposition temperature and weight loss rate of OSG and OOSSG changed compared with SG, so oxidation and esterification affect the thermodynamic properties of SG. The thermal decomposition of SG, OSG and OOSSG can be divided into water loss and decomposition stages. The thermodynamic data of the TGA curves of SG, OSG, and OOSSG are shown in .
Figure 4. TGA curves of SG, OSG and OOSSG.
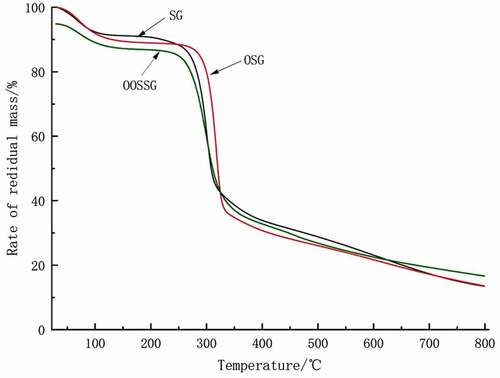
Table 4. TGA thermodynamic data of SG, OSG and OOSSG.
The weight loss rate of SG is reduced after oxidation, and the end decomposition and onset decomposition temperature are increased, indicating increased stability of the modified SG sample after oxidation .The onset decomposition temperature and weight loss rate of SG are reduced after esterification, and the end decomposition temperature is increased, indicating increased stability of the modified SG sample after oxidation esterification. The order of thermal stability decreased in the following order: OOSSG > OSG > SG.
Conclusion
Both oxidation and esterification affect the freeze-thaw stability and expansion capacity of SG. Oxidation decreases the freeze-thaw stability of SG. During esterification, a large number of octenyl long chains and ester hydrophobic groups were introduced, which improved the freeze-thaw stability of SG. Modification of the compound significantly enhanced the freeze-thaw stability of SG. Oxidation and esterification disrupted the molecular structure of SG and attenuated its expansion. Increased frequency of modifications reduced the water solubility and expansion. FTIR analysis revealed no new functional groups following H2O2 oxidation. Esterification and composite modification resulted in new characteristic absorption peaks at 1728 cm−1 and 1573 cm−1 compared with SG, which demonstrates the successful introduction of new groups on the SG molecular chain. Oxidation reduced the crystallinity of SG, and esterification seriously damaged the crystal structure of SG. Oxidation and esterification altered the thermal properties of SG. The thermal stability of the sample was improved after compound modification.
Acknowledgments
This study was jointly supported by China Agriculture Research System of MOF and MARA (CARS-22).
Disclosure statement
No potential conflict of interest was reported by the author(s).
Correction Statement
This article has been republished with minor changes. These changes do not impact the academic content of the article.
References
- Baran, T.; Menteş, A.; Arslan, H. Synthesis and Characterization of Water Soluble O-carboxymethyl Chitosan Schiff Bases and Cu (II) Complexes. Int. J. Biol. Macromol. 2015, 72, 94–103. DOI: 10.1016/j.ijbiomac.2014.07.029.
- Tang, H.; Gao, S.; Li, Y.; Dong, S. Modification Mechanism of Sesbania Gum, and Preparation, Property, Adsorption of Dialdehyde cross-linked Sesbania Gum. Carbohydr. Polym. 2016, 149, 151–162.
- Hongbo, T.; Yao, Y.; Yanping, L.; Siqing, D. Effect of cross-linking and Oxidization on Structure and Properties of Sesbania Gum. Int. J. Biol. Macromol. 2018, 114, 640–648. DOI: 10.1016/j.ijbiomac.2018.03.144.
- Patel, G. C.; Patel, M. M. Preliminary Evaluation of Sesbania Seed Gum Mucilage as Gelling Agent. Int. J. Pharm Tech Res. 2009, 1, 840–843.
- Li, R.; Cheng, Y.; Tang, N.; Wu, L.; Nirasawa, S.; Jia, X.; Cao, W. Rheological, Structural and Physicochemical Characteristics of heat-induced Egg albumin/sesbania Gum Mixed Gels. Int. J. Biol. Macromol. 2020, 163, 87–95. DOI: 10.1016/j.ijbiomac.2020.06.172.
- Tang, M.; Hong, Y.; Gu, Z.; Zhang, Y.; Cai, X. The Effect of Xanthan on Short and Long‐term Retrogradation of Rice Starch. Starch‐Stärke. 2013, 65(7–8), 702–708. DOI: 10.1002/star.201200170.
- Tian, J.; Tang, X.; Yin, J.; Chen, J.; Luo, X.; Rao, G. Enhanced Leachability of a Lean Weathered Crust elution-deposited rare-earth Ore: Effects of Sesbania Gum filter-aid Reagent. Metallurgical and Materials Transactions B. 2013, 44(5), 1070–1077. DOI: 10.1007/s11663-013-9871-3.
- Ponzini, E.; Natalello, A.; Usai, F.; Bechmann, M.; Peri, F.; Müller, N.; Grandori, R. Structural Characterization of Aerogels Derived from Enzymatically Oxidized Galactomannans of Fenugreek, Sesbania and Guar Gums. Carbohydr. Polym. 2019, 207, 510–520. DOI: 10.1016/j.carbpol.2018.11.100.
- Tian, J.; Yin, J.; Tang, X.; Chen, J.; Luo, X.; Rao, G. Enhanced Leaching Process of a low-grade Weathered Crust elution-deposited Rare Earth Ore with Carboxymethyl Sesbania Gum. Hydrometallurgy. 2013, 139, 124–131. DOI: 10.1016/j.hydromet.2013.08.001.
- Huijuan, L.; Lin, G.; Man, X.; Ding, S.; Yuanchen, C. Grafting Modification of Sapura Gum and Its Application in Textile. Sizing J Textile. 2012, 33, 60–64.
- Tang, H.; Liu, Y.; Li, Y.; Li, Q.; Liu, X. Hydroxypropylation of cross-linked Sesbania Gum, Characterization and Properties. Int. J. Biol. Macromol. 2020, 152, 1010–1019. DOI: 10.1016/j.ijbiomac.2019.10.188.
- Shen, D.; Xue, M.; Zhang, L.; Liu, H.; Gao, L.; Cui, Y. Preparation and Characterization of Oxidized Sesbania Gum and Evaluation of Its Warp Sizing Performance for Fine Cotton Yarns. Polym. Degrad. Stab. 2011, 96, 2181–2188.
- D’Melo, D.; Sabnis, A.; Shenoy, M.; Kathalewar, M. Preparation of Acetylated Guar Gum–Unsaturated Polyester Composites & Effect of Water on Their Properties. Curr Chem Lett. 2012, 1(4), 147–156. DOI: 10.5267/j.ccl.2012.9.001.
- Yanping, L.; Hongbo, T.; Qiumei, H.; Xiaoyang, D. Cross-linking of Partially Hydrolyzed Sesbania Gum, Property and Characterization. Int. J. Biol. Macromol. 2020, 159, 11–17. DOI: 10.1016/j.ijbiomac.2020.04.164.
- Sondari, D.; Iltizam, I. Effect of Hydrogen Peroxide on Edible Film from Cassava starch[C]//AIP Conference Proceedings. AIP Publishing LLC 2018, Selangor, Malaysia, 2026, 020083.
- Can, L.; Jiyou, G.; Yanhua, Z. Compare with the Different Catalysts on Conversion and Crystallinity of Hydrogen Peroxide Oxidized Starch. J Northeast Forestry Univ. 2012, 40, 15–147.
- Gahruie, H. H.; Eskandari, M. H.; Van der Meeren, P.; Hosseini, S. M. H. Study on Hydrophobic Modification of Basil Seed gum-based (BSG) Films by Octenyl Succinate Anhydride (OSA). Carbohydr. Polym. 2019, 219, 155–161. DOI: 10.1016/j.carbpol.2019.05.024.
- Mora, C. P.; Martinez-Alejo, J. M.; Roman, L.; Martinez, M. M.; Carvajal, T.; Pinal, R.; Mora-Huertas, C. E. Molecular and Physical Characterization of Octenyl Succinic anhydride-modified Starches with Potential Applications in Pharmaceutics. Int. J. Pharmaceutics. 2020, 579, 119163. DOI: 10.1016/j.ijpharm.2020.119163.
- Shi, Y.; Li, C.; Zhang, L.; Huang, T.; Ma, D.; Tu, Z. C.; Ouyang, B. L.; Xie, H.; Zhang, N.-H.; Ouyang, B.-L. Characterization and Emulsifying Properties of Octenyl Succinate Anhydride Modified Acacia Seyal Gum (Gum Arabic). Food Hydrocolloids. 2017, 65, 10–16. DOI: 10.1016/j.foodhyd.2016.10.043.
- Safdar, B.; Pang, Z.; Liu, X.; Jatoi, M. A.; Mehmood, A.; Rashid, M. T.; Naveed, M.; Naveed, M. Flaxseed Gum: Extraction, Bioactive Composition, Structural Characterization, and Its Potential Antioxidant Activity. J. Food Biochem. 2019, 43(11), e13014. DOI: 10.1111/jfbc.13014.
- Dong, P.; Shiying, Z. Study on the Extraction Method of Sesbania Gum and Its Properties. J Wuxi Light Industry College. 1988, 46–53.
- Liang, S.; Liao, W.; Ma, X.; Li, X.; Wang, Y. H2O2 Oxidative Preparation, Characterization and Antiradical Activity of a Novel Oligosaccharide Derived from Flaxseed Gum. Food Chem. 2017, 230, 135–144. DOI: 10.1016/j.foodchem.2017.03.029.
- Tang, H.; Liu, Y.; Li, Y.; Liu, X. Octenyl Succinate Acidolysis Carboxymethyl Sesbania Gum with High Esterification Degree: Preparation, Characterization and Performance. Polym. Bull. 2022, 35, 1–23.
- Omar-Aziz, M.; Yarmand, M. S.; Khodaiyan, F.; Mousavi, M.; Gharaghani, M.; Kennedy, J. F.; Hosseini, S. S. Chemical Modification of Pullulan Exopolysaccharide by Octenyl Succinic Anhydride: Optimization, Physicochemical, Structural and Functional Properties. Int. J. Biol. Macromol. 2020, 164, 3485–3495. DOI: 10.1016/j.ijbiomac.2020.08.158.
- Liu, Y.; Gao, J.; Wu, H.; Gou, M.; Jing, L.; Zhao, K.; Li, W.; Zhang, G.; Li, W. Molecular, Crystal and Physicochemical Properties of Granular Waxy Corn Starch after Repeated freeze-thaw Cycles at Different Freezing Temperatures. Int. J. Biol. Macromol. 2019, 133, 346–353. DOI: 10.1016/j.ijbiomac.2019.04.111.
- Wang, S.; Copeland, L. New Insights into Loss of Swelling Power and Pasting Profiles of Acid Hydrolyzed Starch Granules. Starch - Stärke. 2012, 64(7), 538–544. DOI: 10.1002/star.201100186.
- Bai, Y.; Shi, Y.; Wetzel, D. Novel FT-IR Microspectroscopic Census of Simple Starch Granules for Octenyl Succinate Ester Modification. J. Agric. Food Chem. 2009, 57, 35-49.
- KarmvIr, G.; Ritika, B. Y.; Baljeet, S. Y.; Roshanlal, Y. Physico-chemical, Textural and Crystallinity Properties of Oxidized, cross-linked and dual-modified White Sorghum Starch. Int. Food Res. J. 2018, 25, 2104–2111.
- Prachayawarakorn, J.; Kansanthia, P. Characterization and Properties of Singly and Dually Modified Hydrogen Peroxide Oxidized and Glutaraldehyde Crosslinked Biodegradable Starch Films. Int. J. Biol. Macromol. 2022, 194, 331–337.
- Das, A.; Sit, N. Modification of Taro Starch and Starch Nanoparticles by Various Physical Methods and Their Characterization. Starch‐Stärke. 2021, 73(5–6), 2000227. DOI: 10.1002/star.202000227.