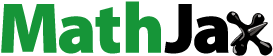
ABSTRACT
The objective of this study was to investigate the effects of aqueous extract of lotus root knot (AELRK) on the gel characteristics of silver carp surimi. Gel strength, storage modulus, and disulfide bond of surimi gels were significantly increased with the addition of the AELRK (p < 0.05), especially at 10% AELRK (p < 0.05), where gel strength and disulfide bond were enhanced by 14.7% and 41.6%, respectively, but decreased in expressible moisture content, ionic bond, hydrogen bond and hydrophobic interaction. Adding 10% AELRK could enhance cross-linking degree surimi gels with the denser and smoother microstructure. There was the reduction in myosin heavy chain but not significant change in actin. More addition of the AELRK significantly decreased in L* and whiteness of surimi gels (p < 0.05) but increased in a * . Therefore, there would be a potential to utilize the phenolic compounds from lotus root to improve the quality of silver carp surimi.
Introduction
Surimi is a type of raw material for aquatic food and has gained widespread attention owing to its unique characteristics, including the high protein, low calorie, low fat, low cholesterol, good gelation, and unique texture.[Citation1] About 1.35 million tons of surimi-based products have been produced in China in 2021.[Citation2] The gelling characteristic is one of the decisive factors in the quality of surimi products and influence the product textural characteristics and consumer experience.[Citation3]
The functional ingredients are added into surimi to improve the gel strength, such as whey protein,[Citation4] konjac oligo-glucomannan,[Citation5] EGCG and TGase,[Citation6] and oil-modified crosslinked tapioca starch.[Citation7] But it is necessary to consider the cost and sources of the additives.[Citation8] Recently, some abundant by-product resources (seeds, peels, husks and other plant wastes) were demonstrated to effectively improve the gel properties of surimi gels, due to their rich in phenolic compounds.[Citation9–12] Plant phenolics could interact with the protein through the non-covalent interaction[Citation13] and induce the protein cross-linking process through the non-covalent and covalent interactions.[Citation14–16] Thus, phenolic compounds could promote the gel properties of surimi. Lotus root (Nelumbo nucifera Gaertn.) has enormous market in China, accompanying with a large amount of waste from lotus root. Lotus root knot (LRK), which is inedible part in lotus root, is normally removed or thrown away during processing. However, the LRK contains the higher phenolics, especially tannin.[Citation17] It is also considered as the most important antioxidative components for phenolic compounds extract from the LRK.[Citation17] There would be a potential to utilize the LRK improve the gel property of surimi-based product and enhance its quality, but there was still limited relevant information about the application of LRK in surimi products. Therefore, the objective of this study was to investigate the effects of the LRK extract on the physicochemical properties of surimi gels from silver carp. It could potentially improve the quality of surimi-based products by the utilization of the LRK.
Materials and methods
Materials
Frozen surimi from silver carp (Hypophthalmichthys molitrix) was Grade AAA purchased from Jingli Fishery Food Co., Ltd (Honghu, Hubei, China). Fresh knot of lotus root (Nelumbo nucifera cv. Elian 9) was obtained from the local market (Wuhan, China). Folin-Ciocalteu reagent and tris-(hydroxymethyl)-aminomethane (Tris) were purchased from Sigma-Aldrich Co., Ltd. (St. Louis, MO, USA), SDS and β-mercaptoethanol (β-Me) were purchased from Shanghai Biological Engineering Co., Ltd. (Shanghai, China). Others chemical regents were purchased from Sinopharm Group Chemical Reagent Co., Ltd. (Shanghai, China). All chemical reagents were analytical grade in the experiment.
Preparation of aqueous extract of lotus root knot
Aqueous extract of lotus root knot (AELRK) was prepared according to the method of Hu and Skibsted[Citation18] with some modification. LRK was cut into pieces and ground into a mashed sample. The ground LRK sample was mixed with 20-time distilled water at 45°C for 30 min under the 100-watt ultrasonic (KQ5200DE, Kunshan Ultrasonic Instrument Co., Ltd., Zhejiang, China). Then the ultrasonic-assisted liquid extract was centrifuged at 4,500 r/min for 10 min at room temperature (Avanti J-E, Beckman, Fullerton, CA, USA). Then the supernatant was evaporated at 45°C using an Eyela rotary evaporator (Tokyo Rikakikai, Co. Ltd., Tokyo, Japan) up to a final volume of 100 mL. The mixture of AELRK was stored at 4°C until use and analysis.
Total phenolic content
Total phenolic content of the AELRK was determined following the method of Zhu et al.[Citation17] with a slight modification. The pH of AELRK was adjusted to 3 and then centrifuged at 4,500 r/min for 10 min. The supernatant (1 mL) was mixed with 1.5 mL Folin-Ciocalteu reagent for 8 min and then added 1.5 mL Na2CO3 (10% w/v) for 30 min at room temperature. The samples were measured the absorbance at 765 nm by a UV-Vis spectrophotometer (UV-1700, Shimadzu Co., Ltd., Kyoto, Japan), while 20 μg/mL gallic acid was used as the standard. The phenolic content was reported as mg tannic acid equivalents per g wet weight of the AELRK. The calculation formula was as follows:
where C is the concentration of gallic acid (μg/mL), V is the final volume of AELRK (mL, 100 mL), V1 is the volume of sample used for the determination (mL), W is the fresh weight of LRK (g), and n is the dilution factor.
Surimi gel preparation
Surimi gel was prepared according to the method of Shi et al.[Citation19] with slight modification. Following thawing at 4°C for 12 h, surimi was cut into small pieces, chopped for 1 min, added 2% NaCl, chopped for 2 min, mixed with different additions of the AELRK (0, 1, 5, 10 and 15% based on surimi content, w/w) and chopped for 3 min. The moisture content of surimi paste was adjusted to 80% by ice water. After chopping, surimi paste was stuffed into a casing with a diameter of 33 mm and sealed tightly at both ends. The stuffed samples were heated firstly at 40°C for 60 min and then at 90°C for 30 min. After heating, the gel samples were cooled at iced water for 30 min and then stored at a refrigerator (4°C).
Punch test
After equilibration to room temperature, surimi gels were cut into 20 mm in length. Surimi gels were subjected to the punch test using a Texture Analyzer (TA.XTPlus, Texture Technologies Corp., Scarsdale, NY, USA) equipped with a spherical-ended stainless steel probe (P/0.25S, Ф 5 mm). The penetration speed was set at 1 mm/s. The gel strength (g•mm) was calculated as the product of breaking force (g) and deformation (mm).[Citation20]
Expressible moisture content (EMC)
Expressible moisture content of the gels was measured according to the method of Wang et al.[Citation20] with slight modification. Gel samples were placed between two sheets of filter paper and centrifuged at 4,000×g for 10 min at room temperature. The EMC was expressed as percent water retained in the papers per 100 g water present in the sample.
Color
The color of surimi gels was determined using a HunterLab Ultra Scan XE colorimeter (HunterLab Co., Ltd, Reston, VA, USA). L* (lightness), a* (redness/greenness), b* (yellowness/blueness) of the sample were determined, while the whiteness (W) was calculated according to the following equation[Citation20]:
Dynamic rheological measurements
Dynamic rheological of surimi pastes were analyzed according to the method of Zhang et al.[Citation21] An AR2000ex dynamic rheometer (TA Instrument Ltd., New Castle, Delaware, USA) was used to monitor the storage modulus (G’). The temperature sweep was heated from 20 to 90°C at 1°C/min rate.
Non covalent bonds
Non-covalent bonds (ionic bond, hydrogen bonds and hydrophobic interaction) were determined as described by Gu et al.[Citation22] using 0.05 mol/L NaCl (SA), 0.6 mol/L NaCl (SB), 0.6 mol/L NaCl and 1.5 mol/L urea (SC), and 0.6 mol/L NaCl and 8 mol/L urea (SD). Protein concentration of supernatants was measured by Lowry et al.[Citation23] to determine the ionic bond (difference between protein dissolved in SB and SA), hydrogen bond (difference between protein dissolved in SC and SB) and hydrophobic interaction (difference between protein dissolved in SD and SC).
Disulfide bond content
Free sulfhydryl group (SHF), total sulfhydryl group (SHT) and disulfide bond (S-S) contents of surimi gel were determined following the method of Jia et al.[Citation24] Surimi gel (1.0 g) was homogenized with 10 mL of Tris-Gly Buffer 1 (pH 8.0, 0.086 mol/L Tris, 0.09 mol/L glycine (Gly), 0.004 mol/L ethylenediaminetetraacetic acid (EDTA) and 8 mol/L urea). The homogenate was centrifuged at 10,000 g for 10 min. For the SHF content measurement, supernatant (4 mL) diluted 20 times was mixed with 200 μL Ellman’s reagent (4 mg/mL, 5,5’-dithio-bis-2-nitrobenzoic acid (DTNB), dissolved in Tris-Gly Buffer 1). After mixing for 1 h at room temperature, its absorbance was determined at 420 nm by UV-Vis spectrophotometer (UV-1700, Shimadzu, Shimadzu Co., Ltd., Kyoto, Japan). For the SHT content measurement, the supernatant (1 mL) was mixed with 4 mL Tris-Gly Buffer 2 (pH 8.0, 20 mmol/L Tris, 0.09 mol/L Gly, 15 mg/mL β-Me, 8 mol/L urea, and 5 mol/L guanidine hydrochloride) at 40°C for 1 h, and then the protein was separated by precipitating with 5 mL of 12% trichloroacetic acid (TCA) for 1 h. Centrifuged at 4,000 g for 10 min, the precipitate was collected and rinsed two times with 5 mL TCA (12%) and dissolved in 10 mL Tris-Gly Buffer 1. An aliquot of Ellman reagent (200 μL) was added into the protein solution (4 mL) and the absorbance was measured at 412 nm. The contents of SHF, SHT and S-S were calculated according to the following equation:
where C is the protein concentration (mg/mL), A412 is the absorbance at 412 nm, 13600 is the molar absorptivity.[Citation25]
SDS-polyacrylamide gel electrophoresis (SDS-PAGE)
Protein patterns of surimi gels were analyzed using SDS-PAGE.[Citation26] Surimi gel (2.0 g) was solubilized in 18 mL 5% SDS at 85°C. The protein solutions (3 mg/mL approximately) were mixed with the SDS-PAGE sample buffer (Beijing Xin Xing Tang Biotechnology Co., Ltd., Beijing, China) and β-Me (1 mg/mL) at a volume ratio of 1:1. Samples were heated at 100°C for 5 min and then cooled with the ice. The sample (10 μL) was analyzed using 5% polyacrylamide stacking gel and 10% polyacrylamide running gel. After the separation, the acrylamide gel was fixed and stained with the Coomassie brilliant blue R-250 (Bio-Rad, Richmond, CA, USA).
Scanning electron microscopy (SEM)
Surimi gels were prepared by the CO2 critical point drying method according to the method of Oujifard et al.[Citation27] The gels were dehydrated in ethanol with serial concentrations of 30, 50, 70, 90 and 100% (v/v). Dried samples were sputter-coated with the gold. The specimens were observed in a JSM-6390 scanning electron microscope (JEOL, Tokyo, Japan) at an acceleration voltage of 20 kV.
Statistical analysis
Analysis of variance (ANOVA) was tested and performed using a SPSS package (SPSS 26.0 for Windows, SPSS Inc, Chicago, IL, USA). Data with p < 0.05 were used as criterion for statistical significance. All samples’ conditions were replicated in triplicates with three repeat measurements for each replication.
Results and discussion
Total phenolic content
The total phenolic content of the AELRK was 3.75 ± 0.06 mg/g, so the LRK might be a practical source of phenolic compounds. The yield of polyphenols was related to the raw material of extraction. It was 5.43 ± 1.28 g GAE/100 g dry basis sample for the content of total polyphenols in residues of coconut extracted by aqueous solution. The total phenolic content of lotus rhizome knot and lotus leaf aqueous extracts were 17.1 ± 0.4, 34.9 ± 0.4 g GAE/100 g dry basis sample, respectively.[Citation28] The extraction method had a significant influence on the phenolic compounds of polyphenols and allowed phenolic yield up to 4.08% from rhizome knot by the combination of enzyme-assisted extraction and ultrafiltration,[Citation17] which was higher than the yield of aqueous extraction. The extraction rate of phenols was significantly related to the extraction solvent.[Citation29–31] Thus, the extraction rate of polyphenols was related to the type of raw material, the state of the raw material, and the extraction method. Results showed that the water could effectively extract phenolic compounds of the LRK, which could serve as the potential additive to improve the quality of surimi-based products.
Gel strength
Gel strength is one of the important indicators of surimi gel properties. shows the effects of the AELRK on gel strength of surimi gels from silver carp. With the increase of the AELRK, breaking force of surimi gels increased firstly and then decreased, while there was significantly higher breaking force of surimi gels with 5% and 10% AELRK than that with other additions (p < 0.05). However, there was no significant difference in the deformation of surimi gels with the addition of AELRK (p > 0.05). Gel strength was increased in surimi gels with the addition of AELRK increased to 10% and then decreased with more addition (15%). The gel strength was enhanced by 14.7% at 10% AELRK. There was no significant difference between surimi gels with 1% AELRK and the control (p > 0.05). It indicated that polyphenol substance in the AELRK (5–10%) might induce protein cross-linking in surimi gels. The ethanolic extract of coconut husk (0.5 mg/g) was able to improve the gel strength of sardine surimi.[Citation32] The decrease in gel strength might probably be caused by the rapid aggregation of proteins due to excessive cross-linking with more phenolic extracts. Gels with rapid and random aggregation usually exhibited poor mechanical properties.[Citation6] The extreme cross-linking might lead to the coagulation of proteins as the increase of ethanolic coconut husk extracts levels, resulting in the formation of disorder gel network.[Citation33] Therefore, the addition of 10% AELRK could effectively improve the gel strength of surimi gel from silver carp.
Expressible moisture content
The expressible moisture content (EMC) reflected the changes of protein–water interactions and gel structure.[Citation34] presents the expressible moisture content of surimi gels with different concentrations of the AELRK. The EMC decreased firstly and then increased with the increase of the AELRK. As the EGCG content increased from 0 to 0.12%, the EMC of silver carp protein gel decreased firstly and then increased.[Citation6] Compared with the control, the EMC was significantly decreased in surimi gels with 5 and 10% AELRK (p < 0.05), which decreased by 15% and 29.5%, respectively, while there was the lowest EMC in surimi gel with 10% AELRK. A lower EMC indicated that more water was entrapped within the gel network, reflecting a higher WHC.[Citation35] There was no significant difference for the EMC between surimi gels with 1 or 15% AELRK and the control (p > 0.05). The EMC was opposite to the gel strength of surimi gels with the addition of the AELRK (). Higher AELRK or phenolics could produce the excessive coagulation or precipitation of proteins in surimi gels, resulting in lower water-holding capacity.[Citation16,Citation36] In addition, the EMC is negatively correlated with the network structure of surimi gels, while the lower EMC implied more uniform density of surimi gel network structure.[Citation4] Thus, 5 and 10% AELRK could promote protein cross-linking and form the denser gel structure in surimi gel.
Color
shows color of surimi gels with different concentrations of the AELRK. There were significantly decreased (p < 0.05) in L* and W values of surimi gels with more addition of the AELRK, while a* value was significantly increased (p < 0.05). Compared with the control, b* value was decreased in surimi gels with increasing AELPK upto 5%, but significantly increased with 10 and 15% AELRK (p < 0.05). The color of surimi gels was related to the color of the AELRK. It might darken the water extract with prolonged high temperature evaporation of aqueous extracts, because there were polyphenols, sugar and other organic compounds in the extracts.[Citation10,Citation37,Citation38] The color of the AELRK could potentially decreased whiteness of the surimi gels (). Whiteness of surimi gel was reduced by 8.7% by the addition of AELRK to 15%. Results showed that the whiteness of surimi gels decreased with the increase of the AELRK. It should be carefully considered for its application, due to its influence of the AELRK on the color of surimi-based products.
Table 1. The color of surimi gels with different concentrations of the AELRK.
Dynamic rheological analysis
shows the storage modulus (G’) of surimi with various concentrations of the AELRK during heating from 20 to 90°C. An increase G,’ which represented energy restored during the deformation, indicated an increase in elastic properties of the sample.[Citation39] All G’ values slightly increased from 20 to 24°C. The initial increase might be related to the interactions of protein chains, indicating the initial formation of the gel network structure.[Citation21] The first decrease in G’ from 24 to 42°C suggested weak chemical bonds decreased gradually, such as hydrogen bond.[Citation40] G’ rapidly decreased as the temperature continued to rise to 48°C, due to the helix-to-coil transformation of myosin.[Citation41] G’ was increased for the second time at the temperature of 48–74°C, because of the aggregation of unfolded protein and the formation of irreversible gel network.[Citation42] Unfolded proteins might favor the aggregation via reactive groups or domains. Hydrophobic domains plausibly underwent hydrophobic interactions, while sulfhydryl group was oxidized to form a disulfide bond.[Citation12] Further heating broke hydrogen bond, resulting in the decrease of G.’[Citation43]
There was the highest G’ in surimi with 10% AELRK, which was correlated to the highest gel strength of surimi gel (). It might probably be due to that the addition of 10% AELRK caused the higher cross-linking among fish proteins during heating, because polyphenols could increase protein cross-linking and promote the stability of protein gel structure.[Citation44] Currently, a higher G’ value corresponded to a higher gel strength. Then, G’ value of surimi with the AELRK was followed the order: 10% > 15% > 0% > 5% > 1%. Thus, the addition of 10% AELRK could enhance more cross-linkings and form higher viscoelasticity in surimi.
Chemical interactions
Chemical interactions of protein molecular mainly include ionic bond, hydrogen bond, hydrophobic interaction and disulfide bond, which maintain three-dimensional network structure during the formation of surimi gels.[Citation45] presents four chemical interactions of surimi gels with different concentrations of the AELRK. Compared to the control, ionic bond, hydrogen bond and hydrophobic interaction of surimi gels were significantly reduced (p < 0.05) with the addition of the AELRK. It was higher ionic and hydrogen bonds of surimi gel with 10% AELRK than that other concentrations of the AELRK. Hydrogen bond was considered to be the most critical force in stabilizing the secondary structure of proteins.[Citation34] The initial increase in hydrogen bonds of surimi gels with 1–10% AELRK might be due to the interactions between hydroxyl groups of phenolic compounds and hydrogen acceptors.[Citation15] With more addition of the AELRK (10–15%), the reduction of hydrogen bonds might be caused by the breakdown between carbon groups and amino groups in protein chains during heating.[Citation46] Hydrophobic interactions were decreased in surimi gels with more addition of the AELRK. It should be caused by the excessive unfolding of myofibrillar protein after the addition of phenolic compounds, leading to the aggregation of myofibrillar protein. The unfolded proteins were partially shielded, resulting in the reduction of hydrophobic groups.[Citation47] Hydrophobic interaction of surimi gel was much higher than ionic and hydrogen bonds, because hydrophobic interaction was a major chemical interaction in surimi gel.[Citation34] The addition of the AELRK into surimi gels weakened the influences of ionic bond, hydrogen bond and hydrophobic interaction on the gel structure of surimi gel.
Figure 4. Effects of AELRK on chemical interactions of surimi gels.
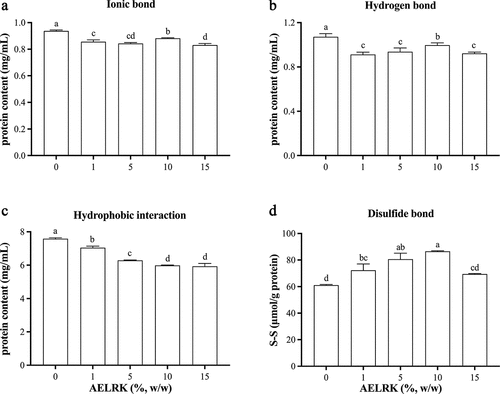
Disulfide bond (S-S) was the major chemical interaction contributed to the formation of the gel network.[Citation16] Surimi gels with the AELRK had more disulfide bond than the control, indicating that the AELRK caused an increase in the formation of S-S cross-linkings in the gel network. Disulfide bond was increased in surimi gels with the increase of AELRK to 10% and then decreased with more addition of the AELRK. Disulfide bond was enhanced by 41.6% at 10% AELRK. The changes of disulfide bond were almost in accordance with gel strength () and G’ () but opposite to the EMC (). The AELRK might probably alter the conformation of the protein, expose more easily oxidized SH groups and form S-S crosslinks in the gel system, because phenolics could change the structure of myofibrillar protein and affect its comprehensive properties, such as gel strength.[Citation48] Overall, 10% AELRK could improve the texture of surimi gels by inducing disulfide bond cross-links. Results suggested that the AELRK could significantly influence chemical interactions of surimi gels, while 10% AELRK had the higher ionic bond, hydrogen bond and disulfide bond in surimi gel.
SDS-PAGE
demonstrates the effects of different concentration AELRK on protein patterns of surimi gels. Myosin heavy chain (MHC, 220 kDa) and actin (AC, 43 kDa) were two major bands in the SDS-PAGE of surimi gels. Compared to MHC, surimi gels had generally intenser AC band, which did not change significantly. However, MHC was lower intensity in surimi gels with the AELRK, while there were more high molecular weight protein aggregates (P) in the upper gel electrophoresis. High molecular weight protein aggregates might be derived from myosin and actin.[Citation49] There was the formation of cross-linkings through disulfide and nondisulfide covalent bonds in surimi gels with the addition of the AELRK, resulting in the decrease of the MHC intensity.[Citation50] Phenolics might undergo the autoxidation during extracting or mixing with surimi paste. Those oxidized phenolic compounds, electrophilic in nature, could induce the formation of nondisulfide covalent bonds between proteins.[Citation12] Moreover, compounds, such as monosaccharaides and oligosaccharides present in AELRK, might help in the polymerization of proteins.[Citation33] The addition of oxidized tannic acid resulted in a higher degree of nondisulfide covalent bond formation in surimi gel of mackerel.[Citation51] However, high molecular weight protein aggregates could not enter the stacking gel, due to their large size.[Citation35] The formed phenol oxygens, such as quinine, induced the cross-linkings between amino groups and attached to proteins via covalent bond, resulting in the reduction of protein bands.[Citation9,Citation32,Citation52] Thus, results suggested that the AELRK enhanced the cross-linking of surimi proteins through covalent and nondisulfide covalent bonds.
Microstructure
displays the microstructure of surimi gels with the AELRK. Surimi gel without the AELRK (the control) had large and uneven holes in the network structure, which was consistent with the lower gel strength () and higher EMC (). Protein network had higher density and smaller void in surimi gels with the AELRK, indicating that AELRK could promote the surimi gels forming ordered network structures. Polyphenols could assist the cross-linkings between proteins and themselves.[Citation53] The pores of gel network were decreased in surimi gels with the AELRK. Phenolics in the AELRK could induce the unfolding of proteins and promote the interactions between proteins, such as hydrogen bond, which was conducive to the formation of denser and ordered network structure.[Citation54] The denser and smoother surface protein was formed in the network of surimi gel with 10% AELRK. Normally, the gels microstructure was positively associated with the water-holding capacity (WHC), so surimi gel with 10% AELRK showed higher gel strength () and lower EMC (). Therefore, results suggested that there were much denser network structure and smaller pores in surimi gel with 10% AELRK.
Conclusion
In summary, the total phenolic content of the AELRK was 3.75 ± 0.06 mg/g, which could affect the gel properties of silver carp surimi. The AELRK (especially 10%) significantly improved the gel structure with the increases of gel strength and G’ and the decrease of expressible moisture content. The AELRK effectively induced cross-linkings of gel proteins by increasing disulfide bond and nondisulfide covalent bond. The AELRK could influence the gelation properties of surimi gels from silver carp, while the extraction process of polyphenols from lotus root knot would be further optimized to improve its extraction rate. The addition of AELRK affected the color of surimi gel, which might influence the consumer acceptance. Thus, the AELRK could serve as a natural additive to promote the proteins cross-linking and improve the gel characteristics of surimi gels.
CRediT authorship contribution statement
Yudong Wang: Conceptualization, Methodology, Validation, Formal analysis, Writing – Original Draft. Xiaoyan Wang: Investigation, Software, Data Curation. Hong Yang: Funding acquisition, Conceptualization, Investigation, Methodology, Resources, Supervision, Validation, Visualization, Writing – Review & Editing.
Acknowledgments
This work was financially supported by the National Key R&D Program of China (2018YFD0901003).
Disclosure statement
The authors declare that they have no known competing financial interests or personal relationships that could have appeared to influence the work reported in this paper.
References
- Wang, Y.; Zhuang, Y.; Yan, H.; Lu, Y.; Deng, X.; Hu, Y.; Xiong, S.; Yang, H. The Influence of pH and Monovalent/Divalent Cations on the Structural and Physicochemical Properties of Myofibrillar Protein from Silver Carp. Food. Chem. 2023, 404, 134519. DOI: 10.1016/j.foodchem.2022.134519.
- China fy. Beijing, China: China Agricultural Press, (in Chinese). 2022.
- Ding, J.; Zhao, X.; Li, X.; Huang, Q. Effects of Different Recovered Sarcoplasmic Proteins on the Gel Performance, Water Distribution and Network Structure of Silver Carp Surimi. Food. Hydroco. 2022, 131, 107835. DOI: 10.1016/j.foodhyd.2022.107835.
- Rawdkuen, S.; Benjakul, S. Whey Protein Concentrate: Autolysis Inhibition and Effects on the Gel Properties of Surimi Prepared from Tropical Fish. Food. Chem. 2008, 106(3), 1077–1084. DOI: 10.1016/j.foodchem.2007.07.028.
- Liu, J.; Fang, C.; Luo, Y.; Ding, Y.; Liu, S. Effects of Konjac Oligo-Glucomannan on the Physicochemical Properties of Frozen Surimi from Red Gurnard (Aspitrigla cuculus). Food. Hydroco. 2019, 89, 668–673. DOI: 10.1016/j.foodhyd.2018.10.056.
- Li, J.; Munir, S.; Yu, X.; Yin, T.; You, J.; Liu, R.; Xiong, S.; Hu, Y. Double-Crosslinked Effect of TGase and EGCG on Myofibrillar Proteins Gel Based on Physicochemical Properties and Molecular Docking. Food. Chem. 2021, 345, 128655. DOI: 10.1016/j.foodchem.2020.128655.
- Zhao, X.; Wang, X.; Li, X.; Zeng, L.; Huang, J.; Huang, Q.; Zhang, B. Effect of Oil Modification on the Multiscale Structure and Gelatinization Properties of Crosslinked Starch and Their Relationship with the Texture and Microstructure of Surimi/Starch Composite Gels. Food. Chem. 2022, 391, 133236. DOI: 10.1016/j.foodchem.2022.133236.
- Park, J. W. Surimi and Surimi Seafood. 3rd ed.; CRC Press: Boca Raton, Florida, 2014.
- Prodpran, T.; Benjakul, S.; Phatcharat, S. Effect of Phenolic Compounds on Protein Cross-Linking and Properties of Film from Fish Myofibrillar Protein. Int. J. Biol. Macromol. 2012, 51(5), 774–782. DOI: 10.1016/j.ijbiomac.2012.07.010.
- Shitole, S. S.; Balange, A. K.; Gangan, S. S. Use of Seaweed (Sargassum tenerrimum) Extract as Gel Enhancer for Lesser Sardine (Sardinella brachiosoma) Surimi. Int. Aquat. Res. 2014, 6(1), 55. DOI: 10.1007/s40071-014-0055-9.
- Buamard, N.; Benjakul, S. Cross-Linking Activity of Ethanolic Coconut Husk Extract Toward Sardine (Sardinella albella) Muscle Proteins. J. Food Biochem. 2017, 41(2), e12283. DOI: 10.1111/jfbc.12283.
- Buamard, N.; Benjakul, S. Improvement of Gel Properties of Sardine (Sardinella albella) Surimi Using Coconut Husk Extracts. Food. Hydroco. 2015, 51, 146–155. DOI: 10.1016/j.foodhyd.2015.05.011.
- Ozdal, T.; Capanoglu, E.; Altay, F. A Review on Protein–Phenolic Interactions and Associated Changes. Food Res. Int. 2013, 51(2), 954–970. DOI: 10.1016/j.foodres.2013.02.009.
- Munir, S.; Hu, Y.; Liu, Y.; Xiong, S. Enhanced Properties of Silver Carp Surimi-Based Edible Films Incorporated with Pomegranate Peel and Grape Seed Extracts Under Acidic Condition. Food Pack. Shelf Life. 2019, 19, 114–120. DOI: 10.1016/j.fpsl.2018.12.001.
- Sharma, S.; Majumdar, R. K.; Mehta, N. K. Gelling Properties and Microstructure of the Silver Carp Surimi Treated with Pomegranate (Punica Granatum L.) Peel Extract. J. Food Sci. Technol. 2022, 59, 4210–4220. DOI: 10.1007/s13197-022-05478-1.
- Balange, A.; Benjakul, S. Enhancement of Gel Strength of Bigeye Snapper (Priacanthus tayenus) Surimi Using Oxidised Phenolic Compounds. Food. Chem. 2009, 113(1), 61–70. DOI: 10.1016/j.foodchem.2008.07.039.
- Zhu, Z.; Li, S.; He, J.; Thirumdas, R.; Montesano, D.; Barba, F. J. Enzyme-Assisted Extraction of Polyphenol from Edible Lotus (Nelumbo nucifera) Rhizome Knot: Ultra-Filtration Performance and HPLC-MS2 Profile. Food Res. Int. 2018, 111, 291–298. DOI: 10.1016/j.foodres.2018.05.047.
- Hu, M.; Skibsted, L. H. Antioxidative Capacity of Rhizome Extract and Rhizome Knot Extract of Edible Lotus (Nelumbo nuficera). Food. Chem. 2002, 76(3), 327–333. DOI: 10.1016/S0308-8146(01)00280-1.
- Shi, L.; Wang, X.; Chang, T.; Wang, C.; Yang, H.; Cui, M. Effects of Vegetable Oils on Gel Properties of Surimi Gels. LWT - Food Sci. Technol. 2014, 57(2), 586–593. DOI: 10.1016/j.lwt.2014.02.003.
- Wang, Y.; Tu, X.; Shi, L.; Yang, H. Quality Characteristics of Silver Carp Surimi Gels as Affected by Okara. Int. J. Food Prop. 2023, 26(1), 49–64. DOI: 10.1080/10942912.2022.2153863.
- Zhang, F.; Fang, L.; Wang, C.; Shi, L.; Chang, T.; Yang, H.; Cui, M. Effects of Starches on the Textural, Rheological, and Color Properties of Surimi–Beef Gels with Microbial Tranglutaminase. Meat. Sci. 2013, 93(3), 533–537. DOI: 10.1016/j.meatsci.2012.11.013.
- Gu, R.; Xiao, X.; Sun, J.; Shi, L.; Yang, H. Effects of Rice Residue on Physicochemical Properties of Silver Carp Surimi Gels. Int. J. Food Prop. 2018, 21(1), 1743–1754. DOI: 10.1080/10942912.2016.1214146.
- Lowry, O.; Rosebrough, N.; Farr, A. L.; Randall, R. Protein Measurement with the Folin Phenol Reagent. J. Biol. Chem. 1951, 193(1), 265–275. DOI: 10.1016/S0021-9258(19)52451-6.
- Jia, D.; Huang, Q.; Xiong, S. Chemical Interactions and Gel Properties of Black Carp Actomyosin Affected by MTGase and Their Relationships. Food. Chem. 2016, 196, 1180–1187. DOI: 10.1016/j.foodchem.2015.10.030.
- Ellman, G. L. Tissue Sulfhydryl Groups. Archiv. of Biochem & Biophys. 1959, 82(1), 70–77. DOI: 10.1016/0003-9861(59)90090-6.
- Chang, T.; Wang, C.; Yang, H.; Xiong, S.; Liu, Y.; Liu, R. Comparison of Conventional Washing Processing and pH Shift Processing on Gelation Characteristics of Bighead Carp (Aristichthys nobilis) Muscle Proteins. J. Aquat. Food Prod. Technol. 2017, 26(1), 103–114. DOI: 10.1080/10498850.2015.1099067.
- Oujifard, A.; Benjakul, S.; Ahmad, M.; Seyfabadi, J. Effect of Bambara Groundnut Protein Isolate on Autolysis and Gel Properties of Surimi from Threadfin Bream (Nemipterus bleekeri). LWT - Food Sci. Technol. 2012, 47(2), 261–266. DOI: 10.1016/j.lwt.2012.01.016.
- Huang, B.; He, J.; Ban, X.; Zeng, H.; Yao, X.; Wang, Y. Antioxidant Activity of Bovine and Porcine Meat Treated with Extracts from Edible Lotus (Nelumbo Nucifera) Rhizome Knot and Leaf. Meat. Sci. 2011, 87(1), 46–53. DOI: 10.1016/j.meatsci.2010.09.001.
- Zhu, Z.; Guan, Q.; Koubaa, M.; Barba, F. J.; Roohinejad, S.; Cravotto, G.; Yang, X.; Li, S.; He, J. HPLC-DAD-ESI-MS2 Analytical Profile of Extracts Obtained from Purple Sweet Potato After Green Ultrasound-Assisted Extraction. Food. Chem. 2017, 215, 391–400. DOI: 10.1016/j.foodchem.2016.07.157.
- Fattore, M.; Montesano, D.; Pagano, E.; Teta, R.; Borrelli, F.; Mangoni, A.; Seccia, S.; Albrizio, S. Carotenoid and Flavonoid Profile and Antioxidant Activity in “Pomodorino Vesuviano” Tomatoes. J. Food Compost. Anal. 2016, 53, 61–68. DOI: 10.1016/j.jfca.2016.08.008.
- Rocchetti, G.; Lucini, L.; Chiodelli, G.; Giuberti, G.; Montesano, D.; Masoero, F.; Trevisan, M. Impact of Boiling on Free and Bound Phenolic Profile and Antioxidant Activity of Commercial Gluten-Free Pasta. Food Res. Int. 2017, 100, 69–77. DOI: 10.1016/j.foodres.2017.08.031.
- Kaewdang, O.; Benjakul, S. Effect of Ethanolic Extract of Coconut Husk on Gel Properties of Gelatin from Swim Bladder of Yellowfin Tuna. LWT - Food Sci. Technol. 2015, 62(2), 955–961. DOI: 10.1016/j.lwt.2015.02.006.
- Singh, A.; Prabowo, F. F.; Benjakul, S.; Pranoto, Y.; Chantakun, K. Combined Effect of Microbial Transglutaminase and Ethanolic Coconut Husk Extract on the Gel Properties and in-Vitro Digestibility of Spotted Golden Goatfish (Parupeneus heptacanthus) Surimi Gel. Food. Hydroco. 2020, 109, 106107. DOI: 10.1016/j.foodhyd.2020.106107.
- Zhang, L.; Li, Q.; Shi, J.; Zhu, B.; Luo, Y. Changes in Chemical Interactions and Gel Properties of Heat-Induced Surimi Gels from Silver Carp (Hypophthalmichthys molitrix) Fillets During Setting and Heating: Effects of Different Washing Solutions. Food. Hydroco. 2018, 75, 116–124. DOI: 10.1016/j.foodhyd.2017.09.007.
- Wasinnitiwong, N.; Benjakul, S.; Hong, H. Effects of κ-Carrageenan on Gel Quality of Threadfin Bream (Nemipterus spp.) Surimi Containing Salted Duck Egg White Powder. Int. J. Biol. Macromol. 2022, 221, 61–70. DOI: 10.1016/j.ijbiomac.2022.08.200.
- Ali Arfat, Y.; Benjakul, S. Gel Strengthening Effect of Zinc Salts in Surimi from Yellow Stripe Trevally. Food. Biosci. 2013, 3, 1–9. DOI: 10.1016/j.fbio.2013.04.009.
- Pansera, M. R.; Iob, G. A.; Atti-Santos, A. C.; Rossato, M.; Atti-Serafini, L.; Cassel, E. Extraction of Tannin by Acacia Mearnsii with Supercritical Fluids. Braz. Arch. Biol. Technol. 2004, 47, 995–998. DOI: 10.1590/S1516-89132004000600019.
- Buamard, N.; Benjakul, S.; Konno, K. Improvement of Gel Quality of Sardine Surimi with Low Setting Phenomenon by Ethanolic Coconut Husk Extract. J. Texture Stud. 2017, 48(1), 47–56. DOI: 10.1111/jtxs.12207.
- Kim, B.; Park, J.; Yoon, W. Rheology and Texture Properties of Surimi Gels. In Surimi and Surimi Seafood, 2nd ed.; CRC Press: Boca Raton, Florida, 2005; pp. 491–582. DOI: 10.1201/9781420028041.ch11.
- Chen, J.; Dickinson, E.; Edwards, M. Rheology of Acid-Induced Sodium Caseinate Stabilized Emulsion Gels. J. Texture Stud. 1999, 30(4), 377–396. DOI: 10.1111/j.1745-4603.1999.tb00226.x.
- Yin, T.; Park, J. W. Textural and Rheological Properties of Pacific Whiting Surimi as Affected by Nano-Scaled Fish Bone and Heating Rates. Food. Chem. 2015, 180, 42–47. DOI: 10.1016/j.foodchem.2015.02.021.
- Lou, X.; Wang, C.; Xiong, Y. L.; Wang, B.; Mims, S. D. Gelation Characteristics of Paddlefish (Polyodon spathula) Surimi Under Different Heating Conditions. J. Food Sci. 2000, 65(3), 394–398. DOI: 10.1111/j.1365-2621.2000.tb16014.x.
- Petcharat, T.; Benjakul, S. Effect of Gellan Incorporation on Gel Properties of Bigeye Snapper Surimi. Food. Hydroco. 2018, 77, 746–753. DOI: 10.1016/j.foodhyd.2017.11.016.
- Xue, H.; Zhang, G.; Han, T.; Li, R.; Liu, H.; Gao, B.; Tu, Y.; Zhao, Y. Improvement of Gel Properties and Digestibility of the Water-Soluble Polymer of Tea Polyphenol-Egg White Under Thermal Treatment. Food. Chem. 2022, 372, 131319. DOI: 10.1016/j.foodchem.2021.131319.
- Lanier, T.; Yongsawatdigul, J.; Carvajal-Rondanelli, P. Surimi Gelation Chem. In Surimi and Surimi Seafood; CRC Press: Boca Raton, Florida, 2013; pp. 101–140. DOI: 10.1201/b16009-6.
- Zhou, X.; Jiang, S.; Zhao, D.; Zhang, J.; Gu, S.; Pan, Z.; Ding, Y. Changes in Physicochemical Properties and Protein Structure of Surimi Enhanced with Camellia Tea Oil. LWT - Food Sci. Technol. 2017, 84, 562–571. DOI: 10.1016/j.lwt.2017.03.026.
- Zhang, Y.; Lv, Y.; Chen, L.; Wu, H.; Zhang, Y.; Suo, Z.; Wang, S.; Liang, Y.; Xu, X.; Zhou, G., et al. Inhibition of Epigallocatechin-3-Gallate/protein Interaction by Methyl-β-Cyclodextrin in Myofibrillar Protein Emulsion Gels Under Oxidative Stress. J. Agric. Food Chem. 2018, 66(30), 8094–8103. DOI: 10.1021/acs.jafc.8b00275.
- Q-D, X.; Z-L, Y.; Zeng, W. -C. Structural and Functional Modifications of Myofibrillar Protein by Natural Phenolic Compounds and Their Application in Pork Meatball. Food Res. Int. 2021, 148, 110593. DOI: 10.1016/j.foodres.2021.110593.
- Cao, Y.; True, A. D.; Chen, J.; Xiong, Y. L. Dual Role (Anti- and Pro-Oxidant) of Gallic Acid in Mediating Myofibrillar Protein Gelation and Gel in vitro Digestion. J. Agric. Food Chem. 2016, 64(15), 3054–3061. DOI: 10.1021/acs.jafc.6b00314.
- Lu, H.; Zhang, L.; Li, Q.; Luo, Y. Comparison of Gel Properties and Biochemical Characteristics of Myofibrillar Protein from Bighead Carp (Aristichthys nobilis) Affected by Frozen Storage and a Hydroxyl Radical-Generation Oxidizing System. Food. Chem. 2017, 223, 96–103. DOI: 10.1016/j.foodchem.2016.11.143.
- Balange, A. K.; Benjakul, S. Effect of Oxidised Tannic Acid on the Gel Properties of Mackerel (Rastrelliger kanagurta) Mince and Surimi Prepared by Different Washing Processes. Food. Hydroco. 2009, 23(7), 1693–1701. DOI: 10.1016/j.foodhyd.2009.01.007.
- Temdee, W.; Benjakul, S. Effect of Oxidized Kiam Wood and Cashew Bark Extracts on Gel Properties of Gelatin from Cuttlefish Skins. Food. Biosci. 2014, 7, 95–104. DOI: 10.1016/j.fbio.2014.07.001.
- Roy, V. C.; Shiran Chamika, W. A.; Park, J. -S.; Ho, T. C.; Khan, F.; Kim, Y. -M.; Chun, B. -S. Preparation of Bio-Functional Surimi Gel Incorporation of Fish Oil and Green Tea Extracts: Physico-Chemical Activities, in-Vitro Digestibility, and Bacteriostatic Properties. Food. Control. 2021, 130, 108402. DOI: 10.1016/j.foodcont.2021.108402.
- Zhang, D.; Yang, X.; Wang, Y.; Wang, B.; Wang, S.; Chang, J.; Liu, S.; Wang, H. Proanthocyanidin B2 and Transglutaminase Synergistically Improves Gel Properties of Oxidized Myofibrillar Proteins. Food. Chem. 2022, 391, 133262. DOI: 10.1016/j.foodchem.2022.133262.