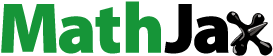
ABSTRACT
Probiotics possess many health-endorsing properties; however, their viability and stability under detrimental condition is uncertain. Encapsulation technology provides protection under various stressed conditions. Furthermore, combination of different wall materials ensures the target delivery of core materials. In the current study, probiotic was encapsulated using solid lipid micro particles (SLMP) that were prepared by high shear homogenization. Whey protein and gum Arabic were used as wall material to encapsulate the probiotics. Obtained micro beads were subjected for size, zeta potential, and encapsulation efficiency measurement. Scanning electron microscopy was used for morphological characterization of the microbeads. Molecular characterization of obtained micro beads was done by Fourier-transform infrared spectroscopy (FTIR) and X-ray diffraction (XRD). Furthermore, the viability and stability were assessed under simulated gastrointestinal conditions. Free and encapsulated probiotics were incorporated in chocolate to evaluate the stability of probiotics. The results in this study indicated that encapsulated probiotics showed significant (P < .05) viability under simulated gastrointestinal and technological conditions compared to free probiotics. A log reduction of 3.54 CFU/mL and 2.52 CFU/mL was detected for SLMW and SLMG after 120 min under simulated gastric condition while 2.42 CFU/mL log reduction and 4.13 log CFU/mL log reduction was detected under intestinal conditions. Likewise, chocolate containing encapsulated probiotics showed better viability at 4°C after 30 days of storage duration and showed significant (p < .05) results. A log reduction of 1.08 log CFU/g was observed in chocolate with Gum Arabic in SLMP as and encapsulating material. In conclusion, solid lipid particles have a strong potential to extend the viability of probiotics under detrimental circumstances.
Introduction
Lactic acid bacteria (LAB) is well known for having a variety of physiological functions that have potential to enhance human health. In order to have such significant effects on human health, beneficial microbes must have the ability to endure and reproduce in the gut of host. Hence, probiotics much have the ability to survive meta-biologically and must reproduce effectively in the large intestine[Citation1]
According to Food and Agriculture Organization – World Health Organization (FAO-WHO, functional food products based on the incorporation of probiotics should contain minimum 106 CFU/mL or g of living probiotic bacteria. However many probiotics including Lactobacilli species do not function well in simulated gastrointestinal tract environments or when heated to high processing.[Citation2] Several researchers investigated bacteria’s resistance to high temperatures in the range of 40–65°C.[Citation3] Some potential health benefits provided by the probiotic bacteria includes endogenous/exogenous infection reduction, lower the chances of inflammatory bowel disease, lactose tolerance improvement, and reduced colon cancer factors, etc. In order to provide maximum health effects to the host, probiotics must remain alive while in the processing, manufacturing and storage of food as well as passage through the gastrointestinal tract.[Citation4] However, the probiotics are vulnerable to the harsh and extreme gastrointestinal conditions that ends in loss of probiotic cells and reduces probiotic efficiency. In order to ensure safe delivery of probiotics in gut to get the claimed health benefits, it is necessary to protect them by covering them with some wall materials (encapsulation).[Citation5] Gum Arabic (GA) is a common polysaccharide that contains glucuronic acid, arabinose, galactose and rhamnose. GA has high solubility and film forming property makes it highly suitable matrix for probiotic encapsulation. GA contains dietary fiber in it that act as a prebiotic matrix and GA can promote the proliferation of beneficial intestinal bacteria.[Citation6]
Many researchers have studied the effects of several encapsulation parameters including the attentiveness of wall material, cell load, carrier size, time of encapsulation, etc., on the viability of probiotic cells under digestive conditions as well as in food products.[Citation7] The choice of encapsulation material, which is dependent on the required chemical and physical qualities, as well as the microcapsule manufacturing process, is a critical aspect in the development of microencapsulated probiotics. The microcapsule should remain stable and intact during its journey through the digestive tract until it reaches its intended destination, where it should break down and release its contents. The bacteria should be retained in the encapsulating substance, which should also prevent the acid wave and digestive enzymes from passing through the microcapsule. Alginate and other carbohydrate polymers have been employed in a variety of dietary applications.[Citation8]
SLMP are molecules made up of a solid lipid shell matrix and have several distinct benefits over micro emulsions and liposomes, including high lipophilic substance encapsulation efficiency, high-scale production capability, slower disintegration rate, and long-term bioactive release.[Citation9] Because of its ability to shield bioactive molecules contained in it from chemical reactions such as oxidation over long periods, SLMP have been used in many food processing areas to improve the shelf-life of food. In addition, SLMP unveil some interesting advantages as compared to solid lipid nanoparticles (SLNs). The SLMP’s micrometric dimensions (that ranges from 20 to 300 m) make it possible to create a stable, solid free-flowing powder that might serve as an intermediary step in the creation of a variety of solid and semisolid pharmaceutical dosage forms. Additionally, their micrometric size is the best suitable for certain applications (such as cutaneous and mucosal distribution) or administration routes (such as nasal and pulmonary). To make SLMP, a wide range of lipophilic excipients can be utilized. When creating these drug delivery systems, selection of appropriate lipid carrier is essential since the excipients chosen will have an impact on its overall qualities.[Citation10] One novel benefit of lipid-based microcapsules over polysaccharide-based carriers is that they can better protect probiotics from severe gastrointestinal conditions because lipases do not present in stomach fluid. Instead, intestinal lipases breakdown lipid-based shells and liberate the core cells near the site of action.[Citation11] The main objective was to evaluate the effectiveness of SLMP encapsulation on the viability and stability of probiotics under simulated gastrointestinal and technological conditions. Furthermore, the viability of free and SLMP were probed in chocolate for.
Materials and methods
The freeze-dried culture of probiotic strain Lactobacillus rhamnosus GG was attained from National Institute of Food Science and Technology (NIFSAT), University of Agriculture Faisalabad. The hydrogenated palm oil used for the construction of SLMP was acquired from the local market of Faisalabad. The chemicals used in this study were obtained from sigma Aldrich (USA) and the research was conducted in different laboratories of Department of Food Science, Government College University Faisalabad, Pakistan.
Bacterial culture preparation
The freeze-dried cell culture was activated by incubating the cells of. L. rhamnosus GG in 100 mL of Man, Rogosa, and Sharpe (MRS) broth for 24 hours at 37°C. Afterward, it was centrifuged (Thermo Fisher Scientific Inc. USA, 750286 EA) at a revolution of 2500 g for 10 minutes and washed with saline sol. (0.85%). Afterward, the cells were suspended in a freshly prepared MRS medium and incubated (Incubator, Memmert BE−400, Germany) for an additional 20 hours at 37°C. The cells were extracted, weighed, and the results were recorded. The concentration of the cells was set to 109 CFU/g.
Preparation of solid lipid microparticles (SLMP)
SLMP were prepared by following the method of Kouider Amar et al.,[Citation12] with some minor modifications in the process. Two different wall materials (Whey protein isolate and Gum Arabic) were used in this study as an encapsulating matrix and hydrogenated palm oil (MP 42°C) was used as a lipid carrier for SLMP production. The encapsulating materials were taken in separate beakers (5% w/w) and the lipid carrier (hydrogenated palm oil) was added in aqueous form. Mixtures were stirred and L. rhamnosus GG in concentration of 109 log CFU/g was added in the beakers separately. Suspensions of the mixtures (lipid matrix + probiotic + wall material) were produced using homogenizer (FSH−2A, China) at 6800 rpm for 1.5 min at an ambient temperature. The prepared suspensions were automized using spray chiller at 10°C and using 4.8 bar pressure for the production of solid lipid microparticles. The obtained liposomes were termed as SLMW (L. rhamnosus GG coated with Whey protein isolate), SLMG (L. rhamnosus GG coated with Gum Arabic).
Experimental study plan for the encapsulation
Characterization of SLMP
The particle size of the prepared solid lipid microparticles were calculated using laser diffraction technique by following the method of de Souza et al.,[Citation13] with few changes in the process. The equipment used for measuring the size was Mastersizer 2000 (Malvern, UK). Before the test, the SLMP were dispersed in ethyl alcohol for 5 min and allowed to settle.
Encapsulation efficiency
Using the method of Cedran et al.,[Citation14] the encapsulation efficiency or entrapment efficiency was estimated. The prepared solid lipid particles were taken, and the particles were disintegrated using a stomacher and the cells were incubated (Incubator, Memmert BE−400, Germany) using pour plate procedure, with the number of cells liberated being measured. The outcome was stated as colony forming units (CFU/mL). The importance of encapsulating effectiveness was assessed using the following formulas:
Encapsulation Efficiency = EE =
Assume that, N is the number of live encapsulated cells that have been discharged from the beads, N0 is before encapsulation, the number of free cells introduced.
Zeta potential
The zeta potential of the prepared solid lipid microparticles were determined using the method of Gunasangkaran et al.,[Citation15] with slight modifications. Zeta potentiometer (Zetasizer ZSU002, Malvern, Worcestershire, UK) was used for the analysis of prepared microparticles after its dispersion in distilled water.
Scanning electron microscopy
The morphology of SLMP was examined using scanning electron microscopy (SEM) following the method of Qi et al..[Citation16] Ted Pella, Inc. double-faced carbon tape was used to attach the SLMP to aluminum stubs (Redding, USA). The pictures were captured using 5 kV voltage and a 1.750 mA current. To examine the internal morphologies of SLMP, they were frozen in liquid N2 solution and sectioned. The materials were examined using SEM with a 63×/1.4 NA oil plan apochromatic DIC objective lens (Emcraft cubeseries, South Korea). Excitation was accomplished with 605 nm laser lines, and pinholes were adjusted at 1 airy unit per channel, yielding pictures in the 1024 1024 format. To stain the bacteria, SYTO 9, a component of the BacLight bacterial viability kit LIVE/DEADs, was employed (Molecular Probes, Eugene, Oregon USA).
Fourier-transform infrared spectroscopy (FTIR)
To assess the presence of different functional groups, Fourier-transform infrared spectroscopy (FTIR) spectroscopy was used by adopting the methods of Mahmud et al.,[Citation17,Citation18] to characterize the SLMP in the 4000–600 cm1 region, handling a Spectrum Two Perkin Elmer FTIR spectrometer (Massachusetts/USA) present at the National Textile Research Centre NTRC spectroscopy lab in National Textile University Faisalabad. The probiotic solution was hanging in a 2% sodium citrate solution.
X-ray diffraction (XRD)
The X-ray diffraction technique (XRD) was carried out using AXS Analytical X-Ray Systems Siemens (D 5005 Germany) diffractometer that exists at Central Hi-tech lab, Government College University Faisalabad was used to detect crystallinity and amorphousness of the internal structure with a voltage of 40 kV and a current of 40 mA. Scanning angles varied from 3 to 90 degrees, with rates of 2 degrees per minute and 0.033 degrees per second.
In vitro gastrointestinal analysis
Cell viability under simulated gastric and intestinal conditions was evaluated by following the method of Rodrigues et al.[Citation19] For the reason, the simulated gastric fluid was prepared taking 2 g of the sample in a test tube having 20 mL of artificial gastric solution that contains 1 M HCl and Pespin 3 g/L in 1% saline solution. The pH of the solution was adjusted to 2.0 and the viability was detected after every 30 min.
Similarly, for simulated intestinal fluid, the solution was prepared having an alkaline condition with bovine salt (2 g), 0.2 M NaOH solution (150 ml), trypsin (20 g) and di-potassium hydrogen (13.6 g) were mixed in 1 L distilled water. By using NaOH, the pH of the simulated intestinal fluid was raised to 7.0 (0.2 M). the free cells and encapsulated cells were added in simulated stomach and intestinal fluid separately and incubated at 37°C. Samples from each treatment were taken and counted for viable after regular intervals of 0, 30, 60, 90, and 120 minutes
Analysis of micro particles under heat treatment
For determining the viability of SLMP, the protocol of Feng et al.[Citation19] was slightly modified and used. In a test tube having 9 ml of normal saline solution (0.9% w/v), free cells and encapsulated microbeads (SLMP) (109 CFU/ml) were inoculated. The test tubes were then incubated for 5 min in a water bath at varied temperatures (40°, 60°, and 75°C). Aliquots were taken out and poured in MRS broth for incubation 37°C for 24 h and the results were noted.
Viability of encapsulated micro particle under refrigeration storage
For the storage stability determination of encapsulated and free probiotic bacteria, the method of Kim et al.[Citation20] was adopted with some minor changes in the process. The SLMP and free cells were stored at 4°C for up to 4 weeks and after every week the cells were harvested for the probiotic enumeration.
Product development
Probiotic chocolate was prepared by following the method of Erdem et al.,[Citation21] by incorporation some modifications in the production process. Cocoa powder (50 g/100 g) was used for the production of chocolate. Free and encapsulated probiotics were added at 3% concentration in a range of 108 log CFU/g during chocolate solidification process. Three different chocolate treatment were prepared Ch-C (control treatment/without probiotics), ChF (Chocolate with probiotics), ChW (chocolate having probiotics encapsulated with whey protein isolate), ChG (chocolate having probiotics encapsulated with Gum Arabic). All the prepared treatments were stored at refrigeration temperature 4°C in aseptic packaging and was stored for four up to 30 days at 20°C.
Probiotic enumeration in Chocolate
Probiotic viability in chocolate will be determined by following the method of Mirković et al.[Citation22] with some modifications. Standard plate count method was for probiotic ant the results were expressed as CFU/g of chocolate. Chocolate was firstly homogenized in a stomacher bag (Stomacher 40, Seward, West Sussex, UK), with saline solution (1% NaCl) and serial dilutions were made. Afterwards, pouring was done in plated on MRS agar (Merck, Germany). The plates were incubated in an incubator (memmert GmbH, Germany). Probiotic colonies were evaluated and counted after 48 h and results were noted.
Statistical analysis
The data obtained from all the experiments were analyzed by applying a two-way analysis of variance along with standard deviation and least significant difference (LSD) was applied on it using Statistix 8.1 software.
Results and discussions
Morphological characterization
depicts the mean significant results (P < .05) for the size, zeta potential, and encapsulation yield of solid lipid microparticles. The average particle size of the samples differed greatly, and this was due to the different wall materials used for its preparation. The particle size of SLMP having whey protein as a wall material was 414 ± 3 mm, while SLMP with Gum Arabic showed 386.28 ± 5.18 mm bead size. Cai et al. (2014) suggested that the particle size may differ due to water solubility difference between the wall materials with the solution, hence resulted in different sizes. Sharifi et al.[Citation23] also suggest the similar results for encapsulation of probiotics.
Table 1. Mean values for encapsulation efficiency, size of SLMP and zeta potential. The values are represented as mean ± SD followed by least significant difference that is expressed in lower case letters.
The Z-potential values of all SLMs fell, regardless of oil content. SLMs have a z-potential of −10.4 ± 0.6 to −16.7 ± 0.6 mV, indicating the presence of more stable droplets. Colloidal particles have a high zeta potential, which improves the electrostatic repulsion force and the system’s physical stability. pH, ionic strength, polysaccharide type and concentration, and protein macromolecules used all influence the degree of surface charge and zeta potential.[Citation24]
The SLMP with whey protein isolate and Gum Arabic were evaluated for the yield efficiency and results are shown in . The initial concentration of the L. rhamnosus GG was 9.5 log CFU/mL, which showed a significant (P < .05) decreasing trend after disintegration process in the stomacher. The mean results obtained after incubation showed that SLMP having Gum Arabic as wall material showed better resistance and releases less probiotics in the surrounding environment. However, Whey protein isolate SLMP showed lower resistance when compared with SLMP with Gum Arabic as wall material. The final yield (%) obtained for SLMG, solid lipid microparticles with gum Arabic was 91.79 ± 1.14% while, SLMW showed 88.22 ± 2.03% yield.
Scanning electron microscopy (SEM)
Solid lipid microparticles encapsulated by whey protein and Gum Arabic containing probiotic bacteria were examined using scanning electron microscopy. shows the SEM images of prepared SLMP, respectively. The SLMPs had a smooth surface, a spherical shape, and crystallized material with variable diameters. Similar morphological images were also reported by Okuro et al.,[Citation25] as well as Pedroso et al.[Citation26] Despite variances in the vegetable oil employed to protect the probiotics, the morphology of the particles produced by high shear homogenization is typical. In this regard, Pelissari et al.[Citation27] produced SLMP loaded with lycopene and soybean protein hydrolyzate, respectively, and reported a particle morphology that was very similar to the particles produced in this study. They found similar results for SLMP generated by spray cooling cinnamon extract and utilizing the same type of vegetable fat soybean oil, with the average diameter of SLMP ranging between 79.2 and 83.2 µm.
Fourier transforms infrared spectrometry (FTIR) of probiotics loaded micro particles
Compounds or potential charges produced during the encapsulation process can be determined using Fourier Transform Infrared Spectroscopy (FTIR). Between the encapsulated particles and the carrier, the spectra were obtained. shows the FTIR spectra of probiotics loaded polymers micro particles. The maximum value of SLMW was 3351.31 cm−1 intensity calculated at 50.0% transmittance and the Lowest Value was measured 926.76 cm−1 intensity, and transmittance at 97.83%. The calculated values 2135.36 cm−1 at 97.61% transmittance, 1632.04 cm−1 at 64.95%, 1363.80 cm−1 at 90.24% Transmittance, 1255.81 cm−1 at 94.23% transmittance, 1064.84 cm−1 at 80.54% transmittance, respectively. The intensities and groupings discovered are consistent with previous research that looked at the main bands of the spectrum for SLM encapsulated with sodium alginate.[Citation28]
Figure 2. FTIR spectra of L. rhamnosus GG coated in (A) whey protein isolate based SLMP and (B) Gum Arabic based SLMP.
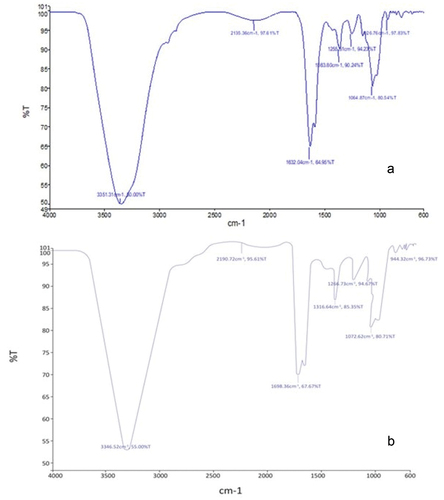
The maximum value of SLMG was 33,320.41 cm−1 intensity calculated at 63.11% transmittance and the Lowest Value was measured 976.22 cm−1 intensity, and transmittance at 98.78%. In between this, we calculated the values 2312.57 cm−1 at 92.27% transmittance, 1769.10 cm−1 at 72.33%, 1450.12 cm−1 at 92.28% Transmittance, 1290.61 cm−1 at 96.91% transmittance, 1095.63 cm−1 at 81.78% transmittance, respectively. Obtained results from the present research correlated with the finding of Holkem et al.,[Citation29] the infrared spectra of the SLMP revealed that none of the peaks indicative of the encapsulated substance were altered, implying that no major interactions occurred.
X-ray diffraction
X-ray powder diffraction was used to get further information on the solid-state architectures of SLMs. Examining the parameters for encapsulation and structure of materials, X-ray diffraction analyses are used (crystalline or amorphous). The diffraction profiles (diffractograms) of the four formulations examined are shown in . Because SLMs have a lipid matrix, a tendency for polymorphic reorganization for more energetically advantageous levels should be noted, and this behavior may result in the expulsion of the bioactive ingredient when it achieves a more crystalline arrangement.[Citation30]
Figure 3. The diffraction profiles (diffractograms) of (a) whey protein isolate based SLMP and (b) Gum Arabic based SLMP.
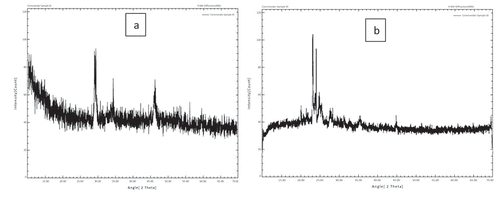
The oil layer and polymers all display similar diffractograms with different peaks, which are features of the fat’s B′ type crystal structure. Fat crystals of the B′ type may go through a phase transition to a kind that is more stable and has larger crystals. The probiotics may be ejected as a result of this rearrangement; however, because these lipid particles have a second cover that is made up of polymer complexes, the probiotics will be well protected under the microcapsules. The development of’ crystals during manufacture is what gives the interesterified fat features like fluidity and various melting and frying properties. The SLM diffraction patterns are highly similar, showing that no polymorphic alterations have occurred. The findings were compared to those of Gamboa et al.[Citation31] who researched the behavior of lipid materials and found that the behavior observed in this study was similar to that reported in the literature, which is often associated with a polymorphic form of triacylglycerol and fatty acids.
Simulated gastric and intestinal study
The bacteria must transit through the host’s digestive tract and colonize there in order to have a favorable effect on the host. shows the significant results (P < .05) for the survival of free and SLMP encapsulated probiotics under simulated gastrointestinal environment. From the mean results, it can be noted that the overall cell viability decreases during the gastric and intestinal analysis. The extreme acidic environment of the stomach was responsible for cell death during the gastric transit of free and encapsulated probiotics (Prestes et al., 2021). When observed precisely, a sharp decline in the viability of free probiotics was observed during gastric conditions and a reduction of 9.86 log CFU/mL was noted. However, a log reduction of 3.54 CFU/mL and 2.52 CFU/mL was calculated for SLMW and SLMG after 120 min. Saha et al.[Citation32] recommended that gums are compatible wall materials for the encapsulation of probiotics as they maintain the probiotic survival and enhance the chances of probiotic viability.
Table 2. Mean values for simulated gastric conditions (GC) and intestinal conditions (IC). Values are expressed as mean ± SD followed by least significant difference that is expressed in upper and lower case letters.
A similar study was also conducted to analyze the probiotic viability under simulated intestinal conditions as well. The initial cell was adjusted in the range of 9.97–9.99 log CFU/mL and cell viability was detected after every 30 min (0, 30, 60, 90, and 120 min) interval. Free probiotics showed a rapid fall in its population with and no cell was detected after 120 min. However, a log reduction was observed for SLMW and SLMG. The lowest reduction in bacterial cell population was evaluated in SLMG with a log reduction of 2.42 CFU/mL, while SLMW showed 4.13 log CFU/mL reduction. Shinde et al.[Citation33] also analyze a decline in the probiotic cell growth under simulated intestinal conditions due to difference in the pH and intestinal salts that may cause unfavorable conditions for the normal growth of probiotics.
Probiotic enumeration in chocolate
The mean results regarding enumeration of probiotics in chocolate predicted a significant impact that can be observed in . A positive result was observed for ChW and ChG as both treatments showed the probiotics in the recommended levels. A significant (p < .05) decrease in the probiotic growth was observed in ChF as it has free probiotics. However, the highest probiotic counts were determined in ChG with the least log reduction of 1.08 log CFU/g. These results indicated that the formulation of SLMP added chocolate treatment showed a sufficient levels of probiotics even after storage for 30 days due to the presence of protective barrier against probiotics and hence the consumption of SLMP added chocolate may provide the recommended probiotics to the consumer as recommended by International Dairy Federation.[Citation34]
Table 3. Mean values for probiotic viability during in chocolate during storage interval of 30 days (0, 10th, 20th and 30th days). Values are expressed as mean ± SD followed by least significant difference that is expressed in upper and lower case letters.
Conclusion
The present study was planned to encapsulate the probiotic bacteria in SLMP having Gum Arabic and Whey protein isolate as a wall material. The prepared SLMP were evaluated successfully using various technological parameters, i.e., SEM, FTIR, and XRD that showed the morphological and structural characteristics of the prepared beads, which undergone simulated gastric and intestinal analysis to determine the probiotic viability. The simulated studies showed SLMG reported better stability under adverse digestive environment. Moreover, the encapsulated cells were incorporated in chocolate to ensure the probiotic stability during storage.
Acknowledgments
The authors are thankful to the Department of Food Science, Government College University Faisalabad for providing lab facilities for the current study.
Disclosure statement
The authors declare no potential conflict of interest.
Data availability statement
The data has been provided in the form of tables and figures. However, more details will be provided on request basis.
References
- Peng, M.; Tabashsum, Z.; Anderson, M.; Truong, A.; Houser, A. K.; Padilla, J.; Biswas, D.; Bhatti, J.; Rahaman, S. O.; Biswas, D. Effectiveness of Probiotics, Prebiotics, and Prebiotic‐Like Components in Common Functional Foods. Compr. Rev. Food Sci. Food Saf. 2020, 19(4), 1908–1933. DOI: 10.1111/1541-4337.12565.
- Altamirano‐Ríos, A. V.; Guadarrama‐Lezama, A. Y.; Arroyo‐Maya, I. J.; Hernández‐Álvarez, A. J.; Orozco‐Villafuerte, J. Effect of Encapsulation Methods and Materials on the Survival and Viability of Lactobacillus acidophilus: A Review. Int J. Food Sci. Tech. 2022, 57(7), 4027–4040. DOI: 10.1111/ijfs.15779.
- Zupanc, M.; Pandur, Ž.; Perdih, T. S.; Stopar, D.; Petkovšek, M.; Dular, M. Effects of Cavitation on Different Microorganisms: The Current Understanding of the Mechanisms Taking Place Behind the Phenomenon. A Review and Proposals for Further Research. Ultrason. Sonochem. 2019, 57, 147–165. DOI: 10.1016/j.ultsonch.2019.05.009.
- Palanivelu, J.; Thanigaivel, S.; Vickram, S.; Dey, N.; Mihaylova, D.; Desseva, I. Probiotics in Functional Foods: Survival Assessment and Approaches for Improved Viability. Appl. Sci. 2022, 12(1), 455. DOI: 10.3390/app12010455.
- Ma, J.; Li, T.; Wang, Q.; Xu, C.; Yu, W.; Yu, H.; Jiang, Z.; Feng, Z.; Chen, L.; Hou, J. Enhanced Viability of Probiotics Encapsulated within Synthetic/Natural Biopolymers by the Addition of Gum Arabic via Electrohydrodynamic Processing. Food Chem. 2023, 413, 135680. DOI: 10.1016/j.foodchem.2023.135680.
- Xu, C.; Ma, J.; Liu, Z.; Wang, W.; Liu, X.; Qian, S.; Jiang, Z.; Gu, L.; Sun, C.; Hou, J. Preparation of Shell-Core Fiber-Encapsulated Lactobacillus Rhamnosus 1.0320 Using Coaxial Electrospinning. Food Chem. 2023, 402, 134253. DOI: 10.1016/j.foodchem.2022.134253.
- Rashidinejad, A.; Bahrami, A.; Rehman, A.; Rezaei, A.; Babazadeh, A.; Singh, H.; Jafari, S. M. Co-Encapsulation of Probiotics with Prebiotics and Their Application in Functional/Synbiotic Dairy Products. Critical Reviews In Food Sci. And Nutr. 2022, 62(9), 2470–2494. DOI: 10.1080/10408398.2020.1854169.
- Allan-Wojtas, P.; Hansen, L. T.; Paulson, A. T. Microstructural Studies of Probiotic Bacteria-Loaded Alginate Microcapsules Using Standard Electron Microscopy Techniques and Anhydrous Fixation. LWT-Food Sci. Tech. 2008, 41(1), 101–108. DOI: 10.1016/j.lwt.2007.02.003.
- Nahum, V.; Domb, A. J. Recent Developments in Solid Lipid Microparticles for Food Ingredients Delivery. Foods. 2021, 10(2), 400. DOI: 10.3390/foods10020400.
- Scalia, S.; Young, P. M; Traini, D. Solid Lipid Microparticles as an Approach to Drug Delivery. Expert Opin. Drug Delivery. 2015, 12(4), 583–599. DOI: 10.1517/17425247.2015.980812.
- Matos-Jr, F. E.; Di Sabatino, M.; Passerini, N.; Favaro-Trindade, C. S.; Albertini, B. Development and Characterization of Solid Lipid Microparticles Loaded with Ascorbic Acid and Produced by Spray Congealing. Food Res. Int. 2015, 67, 52–59. DOI: 10.1016/j.foodres.2014.11.002.
- Kouider Amar, M.; Rahal, S.; Laidi, M.; Rebhi, R.; Hentabli, M.; Hanini, S.; Hamadache, M. Rheological and Structural Study of Solid Lipid Microstructures Stabilized within a Lamellar Gel Network. J. Pharm. Innovation. 2022, 17(4), 1434–1450. DOI: 10.1007/s12247-022-09642-0.
- de Souza Queirós, M.; Viriato, R. L. S.; Ribeiro, A. P. B.; Gigante, M. L. Dairy-Based Solid Lipid Microparticles: A Novel Approach. Food Res. Int. 2020, 131, 109009. DOI: 10.1016/j.foodres.2020.109009.
- Cedran, M. F.; Rodrigues, F. J.; Bicas, J. L. Encapsulation of Bifidobacterium BB12® in Alginate-Jaboticaba Peel Blend Increases Encapsulation Efficiency and Bacterial Survival Under Adverse Conditions. Appl. Microbiol. Biotechnol. 2021, 105(1), 119–127. DOI: 10.1007/s00253-020-11025-9.
- Gunasangkaran, G.; Ravi, A. K.; Arumugam, V. A.; Muthukrishnan, S. Preparation Characterization, and Anticancer Efficacy of Chitosan, Chitosan Encapsulated Piperine and Probiotics (Lactobacillus Plantarum (MTCC-1407), and Lactobacillus Rhamnosus (MTCC-1423) Nanoparticles. J. Bionanosci. 2022, 12(2), 527–539.
- Qi, W.; Liang, X.; Yun, T.; Guo, W. Growth and Survival of Microencapsulated Probiotics Prepared by Emulsion and Internal Gelation. J. Food Sci. Technol. 2019, 56(3), 1398–1404. DOI: 10.1007/s13197-019-03616-w.
- Mahmud, S.; Khan, S.; Khan, M. R.; Islam, J.; Sarker, U. K.; Hasan, G. A.; Ahmed, M. Viability and Stability of Microencapsulated Probiotic Bacteria by Freeze‐Drying Under in vitro Gastrointestinal Conditions. Food Process. Preserv. 2022, 46(11), 17123. DOI: 10.1111/jfpp.17123.
- Rodrigues, F. J.; Cedran, M. F.; Garcia, S. Influence of Linseed Mucilage Incorporated into an Alginate-Base Edible Coating Containing Probiotic Bacteria on Shelf-Life of Fresh-Cut Yacon (Smallanthus Sonchifolius). Food. Bioprocess Techy. 2018, 11, 1605–1614.
- Feng, K.; Zhai, M. Y.; Zhang, Y.; Linhardt, R. J.; Zong, M. H.; Li, L.; Wu, H. Improved Viability and Thermal Stability of the Probiotics Encapsulated in a Novel Electrospun Fiber Mat. J. Agri. Food Chem. 2018, 66(41), 10890–10897. DOI: 10.1021/acs.jafc.8b02644.
- Kim, J. U.; Kim, B.; Shahbaz, H. M.; Lee, S. H.; Park, D.; Park, J. Encapsulation of Probiotic Lactobacillus acidophilus by Ionic Gelation with Electrostatic Extrusion for Enhancement of Survival Under Simulated Gastric Conditions and During Refrigerated Storage. Int J. Food Sci. Tech. 2017, 52(2), 519–530. DOI: 10.1111/ijfs.13308.
- Erdem, Ö.; Gültekin-Özgüven, M.; Berktaş, I.; Erşan, S.; Tuna, H. E.; Karadağ, A.; Özçelik, B.; Güneş, G.; Cutting, S. M. Development of a Novel Synbiotic Dark Chocolate Enriched with Bacillus Indicus HU36, Maltodextrin and Lemon Fiber: Optimization by Response Surface Methodology. LWT Food Sci. Technol. 2014, 56(1), 187–193. DOI: 10.1016/j.lwt.2013.10.020.
- Mirković, M.; Seratlić, S.; Kilcawley, K.; Mannion, D.; Mirković, N.; Radulović, Z. The Sensory Quality and Volatile Profile of Dark Chocolate Enriched with Encapsulated Probiotic Lactobacillus Plantarum Bacteria. Sensors. 2018, 18(8), 2570. DOI: 10.3390/s18082570.
- Sharifi, S.; Rezazad-Bari, M.; Alizadeh, M.; Almasi, H.; Amiri, S. Use of Whey Protein Isolate and Gum Arabic for the Co-Encapsulation of Probiotic Lactobacillus Plantarum and Phytosterols by Complex Coacervation: Enhanced Viability of Probiotic in Iranian White Cheese. Food Hydrocoll. 2021, 113, 106496. DOI: 10.1016/j.foodhyd.2020.106496.
- Khorshidi, M.; Heshmati, A.; Taheri, M.; Karami, M.; Mahjub, R. Effect of Whey Protein‐And Xanthan‐Based Coating on the Viability of Microencapsulated Lactobacillus acidophilus and Physiochemical, Textural, and Sensorial Properties of Yogurt. Food Sci. Nutr. 2021, 9(7), 3942–3953. DOI: 10.1002/fsn3.2398.
- Okuro, P. K.; Thomazini, M.; Balieiro, J. C.; Liberal, R. D.; Fávaro-Trindade, C. S. Co-Encapsulation of Lactobacillus acidophilus with Inulin or Polydextrose in Solid Lipid Microparticles Provides Protection and Improves Stability. Food Res. Int. 2013, 53(1), 96–103. DOI: 10.1016/j.foodres.2013.03.042.
- de Lara Pedroso, D.; Thomazini, M.; Heinemann, R. J. B.; Favaro-Trindade, C. S. Protection of Bifidobacterium Lactis and Lactobacillus acidophilus by Microencapsulation Using Spray-Chilling. Int. Dairy. J. 2012, 26(2), 127–132. DOI: 10.1016/j.idairyj.2012.04.008.
- Pelissari, J. R.; Souza, V. B.; Pigoso, A. A.; Tulini, F. L.; Thomazini, M.; Rodrigues, C. E.; Favaro-Trindade, C. S.; Favaro-Trindade, C. S. Production of Solid Lipid Microparticles Loaded with Lycopene by Spray Chilling: Structural Characteristics of Particles and Lycopene Stability. Food Bioprod. Process. 2016, 98, 86–94. DOI: 10.1016/j.fbp.2015.12.006.
- Zhang, Z. H.; Zhang, Y. L.; Zhou, J. P.; Lv, H. X., Xu, X-R., Zhou, J-P., Huo, W. Solid Lipid Nanoparticles Modified with Stearic Acid–Octaarginine for Oral Administration of Insulin. IJN. 2012, 3333–3339. DOI: 10.2147/IJN.S31711.
- Holkem, A. T.; Favaro-Trindade, C. S. Potential of Solid Lipid Microparticles Covered by the Protein-Polysaccharide Complex for Protection of Probiotics and Proanthocyanidin-Rich Cinnamon Extract. Food Res. Int. 2020, 136, 109520. DOI: 10.1016/j.foodres.2020.109520.
- Tulini, F. L.; Souza, V. B.; Echalar-Barrientos, M. A.; Thomazini, M.; Pallone, E. M.; Favaro-Trindade, C. S. Development of Solid Lipid Microparticles Loaded with a Proanthocyanidin-Rich Cinnamon Extract (Cinnamomum Zeylanicum): Potential for Increasing Antioxidant Content in Functional Foods for Diabetic Population. Food Res. Int. 2016, 85, 10–18. DOI: 10.1016/j.foodres.2016.04.006.
- Gamboa, O. D.; Gonçalves, L. G.; Grosso, C. F. Microencapsulation of Tocopherols in Lipid Matrix by Spray Chilling Method. Procedia Food Sci. 2011, 1, 1732–1739. DOI: 10.1016/j.profoo.2011.09.255.
- Saha, D.; Bhattacharya, S. Hydrocolloids as Thickening and Gelling Agents in Food: A Critical Review. J. Food Sci. Technol. 2010, 47(6), 587–597. DOI: 10.1007/s13197-010-0162-6.
- Shinde, T.; Vemuri, R.; Shastri, M. D.; Perera, A. P.; Tristram, S.; Stanley, R.; Eri, R. Probiotic Bacillus Coagulans MTCC 5856 Spores Exhibit Excellent in-Vitro Functional Efficacy in Simulated Gastric Survival, Mucosal Adhesion and Immunomodulation. J. Funct. Foods. 2019, 52, 100–108. DOI: 10.1016/j.jff.2018.10.031.
- Dong, Q. Y.; Chen, M. Y.; Xin, Y.; Qin, X. Y.; Cheng, Z.; Shi, L. E.; Tang, Z. X. Alginate-based and protein-based materials for Probiotics Encapsulation: A Review. Int J. Food Sci. Tech. 2013, 48(7), 1339–1351. DOI: 10.1111/ijfs.12078.