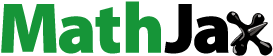
ABSTRACT
Exopolysaccharides (EPSs) are highly heterogeneous natural polymers, can exhibit some unique bio-logical activities, such as anti-oxidation, inflammation regulation, anticancer and anti-viral activities, etc. A new type of EPS was obtained from the fermentation supernatant of Lactiplantibacillus plantarum YT013. However, the low yield of EPS posed a challenge for further research and application. Therefore, in this study, the production process of EPS was optimized, and anti-cancer cells proliferation activity was investigated. Identified the best single-factor conditions for EPS production, including initial pH 6, inoculation amount 5% (v/v), temperature 37°C, cultivation time 36 h, glucose 3% (w/v), soy peptone 1.5% (w/v), KH2PO4 0.3% (w/v). Under these conditions, the maximum production of EPS reached 0.630 mg/mL, which is 1.31 times higher than that before optimization. Concurrently, the nonlinear model was used for fitting, and it was found that the Voigt nonlinear model was in better agreement with the optimization results, which can be used as an effective tool for optimizing EPS production conditions. Furthermore, the anti-proliferative activity of EPS was evaluated using the human gastric cancer AGS cell line in vitro. MTT assay revealed a strong inhibitory effect of EPS on AGS cell proliferation. Additionally, flow cytometry analysis indicated that EPS promoted apoptosis in AGS cells. Lactiplantibacillus plantarum YT013 EPS, as a biodegradable and nontoxic natural active substance, shows potential as a natural antitumor substance.
Introduction
Exopolysaccharides (EPSs) are macromolecular carbohydrates that are secreted outside the cell wall by microorganisms during their growth and metabolism. These compounds have gained significant attention due to their various biological characteristics in recent years.[Citation1,Citation2] EPS primarily consists of carbohydrates, but it may also contain small amounts of other components such as glycolipids and glycoproteins.[Citation3] Based on their chemical composition, EPSs can be categorized into two types: homopolysaccharides (composed of a single type of monosaccharide) and heteropolysaccharides (composed of two or more types of monosaccharides).[Citation4] Homopolysaccharides are typically produced by microorganisms like Lactobacillus, Streptococcus, Wickerella, and Leuconostoc, etc. It is composed of a kind of monosaccharide, namely glucose or fructose, which is called glucans or fructans, respectively.[Citation5] Heteropolysaccharides, on the other hand, are commonly produced by mesophilic and thermophilic lactic acid bacteria (LAB), including species like Leuconostoc lactis, Lacticaseibacillus casei, Lactiplantibacillus plantarum (L. plantarum), Lacticaseibacillus rhamnosus, and Lactobacillus helveticus, etc. LAB, in general, are the preferred choice for EPSs production due to their long history of safe use by humans and their Generally Recognized as Safe (GRAS) status.[Citation6]
LAB EPSs, being biopolymers, have garnered significant attention due to their high safety, ease of use, and unique functionalities. Numerous studies have evaluated the potential of LAB-derived EPS as health care or food additives.[Citation7] In the food industry, they play variously important roles, such as thickeners, gelling agents, emulsifiers, deformers, and stabilizers.[Citation8] Moreover, LAB EPSs exhibit biological activities and stability, making them suitable for medical applications, including anti-radiation, anti-coagulation, hypoglycemic, anti-infective, and anti-tumor activities, etc.[Citation9] Some microbial polysaccharides are also employed in the production of medicines and health products.[Citation10] Additionally, LAB EPSs can also be utilized as bioflocculants for removing heavy metals from wastewater, in packaging materials, and in bio-metallurgy.[Citation11] L. plantarum is a LAB species that is widely present in fermented vegetables, plant surfaces, dairy products, and other traditional fermented foods.[Citation12] Previous studies have demonstrated the antioxidant and anti-proliferative activities of EPS produced by L. plantarum. For instance, EPS produced by L. plantarum MTCC 9510 exhibited anti-proliferative effects on breast cancer MCF-7 cells, as revealed by morphological apoptosis detection and cytotoxicity experiments.[Citation13] Similarly, EPS produced by L. plantarum 70810, referred to as c-EPS, demonstrated inhibition of the in vitro proliferation of three cancer cell lines: HepG-2, BGC-823, and HT-29, as observed in MTT assays.[Citation14] Likewise, EPS produced by L. plantarum WLPL09 displayed a significant concentration-dependent anti-proliferative effect on HepG2 and HCT-8 cells.[Citation15]
LAB-produced EPSs possess favorable biological and physical characteristics, making them suitable for a wide range of industrial applications. However, the low purity and yield of EPSs often restrict their direct utilization, thereby limiting related research and applications. Therefore, optimizing the conditions to improve EPS yield has emerged as an ideal approach.[Citation16] The EPSs yield of LAB were influenced by various factors, including the species of LAB and several culture conditions such as medium composition, carbon source, nitrogen source, culture time, and inoculation amount.[Citation16] In this study, a nonlinear model was established through single-factor conditional optimization analysis to achieve a higher EPS yield from L. plantarum YT013. Additionally, the study aimed to evaluate the anti-proliferative effect of EPS on AGS cells and reveal the potential mechanisms involved.
Materials and methods
Materials and instruments
MTT kits was purchased from Solarbio Science & Technology Co., Ltd., Beijing, China. All the other chemicals and nutrients were analytical grade and obtained from commercial sources (Refer to ).
Table 1. The sources of the chemicals and nutrients.
Microorganism and cancer cells
In our previous study, L. plantarum YT013 was isolated from serofluid dish and preserved in the China Center for Type Culture Collection (CCTCC, M2018775). L. plantarum YT013 was cultured in de Man, Rogosa and Sharpe (MRS) broth. The MRS broth (1000 mL) consisted of 20 g glucose, 10 g peptone, 2 g diammonium hydrogen citrate, 5 g yeast extract, 0.5 g magnesium sulfate, 0.2 g manganese sulfate, 2 g dipotassium hydrogen phosphate, 0.5 g sodium acetate, and 1 mL Tween 80.
The human gastric cancer cell line AGS was obtained from the American Type Culture Collection (ATCC, LOT: 70012225). AGS cells were cultured in Roswell Park Memorial Institute (RPMI) 1640 medium. All media were supplemented with 10% fetal bovine serum (Sijiqing Biologic Co. Ltd., Zhejiang, China) and 1% penicillin/streptomycin (Solarbio, Beijing, China), and incubation was carried out at 37°C and 5% CO2.
Preparation of EPS
The preparation of L. plantarum YT013 EPS was referred to previous study.[Citation17] The L. plantarum YT013 strain was activated and inoculated into a conical flask containing MRS medium and cultured at 37°C. After incubation, boiled in a water bath for 10 min, cooled to room temperature, and centrifuged (8,000 ×g, 15 min, 4°C) to collect the supernatant. The collected supernatant was concentrated using a rotary evaporator. Added trichloroacetic acid to the concentrated solution to achieve a final concentration of 4% (v/v). Let stand for 4 h after shaking and then collected the supernatant by centrifugation (12,000 ×g, 15 min, 4°C). Absolute ethanol was added to the supernatant to a final concentration of 75% (v/v) for 12 h and centrifuged (12,000× g, 15 min, 4°C) to collect precipitate. The precipitate was re-dissolved with deionized water, transferred to the 8–14 kDa dialysis bags (Solarbio, Beijing, China) for 72 h, and freeze-dried to obtain EPS.
YT013 optimization of EPS production conditions
The composition and content of media have a crucial impact on the proliferation of microorganisms and the biosynthesis of metabolites.[Citation18] To determine the optimal conditions for the maximum EPS yield from L. plantarum YT013, the fermentation supernatant of L. plantarum YT013 was used as the experimental material, following a previous study.[Citation19] To investigate the effects of various factors on EPS yield, the following parameters were examined: culture time (12, 24, 36, 48, 72 h), temperature (28, 32, 37, 42, 45°C), pH (4, 5, 6, 7, 8), inoculation amount (1, 3, 5, 10, 15%), phosphate (KH2PO4, NaH2PO4, Na2HPO4, K2HPO4), nitrogen source(soy peptone, tryptone, casein peptone, peptone), and carbon source (glucose, sucrose, lactose, starch soluble). All experiments were conducted in triplicate, and the average values were obtained for analysis.
Antiproliferative activity
The MTT (3-(4,5-dimethylthiazol-2-yl)-2,5-diphenyltetrazolium bromide) kit was used to detect the effects of EPS on the proliferation of AGS cells. The assay protocol was adapted from the methods described by Luyao Xiao et al.[Citation20] AGS cells were seeded in 96-well plates and incubated overnight to allow cell attachment and growth. Incubated AGS cells in 96-well plates overnight before treatment with EPS. Cells were treated with 400 μg/mL of EPS and cultured for different time periods, such as 24, 48 and 72 h. EPS was solubilized in RPMI 1640 medium, and the fresh culture medium was used alone as the control. After the respective incubation periods, 10 μL of MTT (5 mg/mL) solution was added to each well, and then continued to culture for 4 h. At last, the culture medium containing MTT solution was carefully removed, and 100 μL of dimethyl sulfoxide (DMSO) was added to each well to dissolve the formazan crystals. and then measured the absorbance at 490 nm using a microplate reader (Perlong New Technology Co., Ltd., Beijing, China). The absorbance at 490 nm is directly proportional to the number of viable cells, allowing the assessment of cell proliferation in response to EPS treatment. The formula for calculating the inhibition rate is as follows:
Inhibitory rate (%)=[1-(Asample-Ablank)/(Acontrol-Ablank)]×100, where Acontrol and Ablank are the absorbance values without EPS or cells, respectively. All results were transformed into percentages based on their respective control.
Apoptosis analysis
The apoptosis was detected by fluorescent cell counts using the Annexin V Alexa Fluor 488/PI kit (Solarbio, Beijing, China) according to our previous method.[Citation21] Briefly, the AGS cells were cultured in 6-well culture plates at 8 × 105 cells/well and treated with EPS (400 μg/mL) for 48 h. After incubation, the cells were treated with trypsin without EDTA and washed twice with precooled PBS. The cells were treated with the Annexin V Alexa Fluor 488/PI kit. Flow cytometry (FACSCalibur, BD Biosciences, Mountain View, CA, USA) was used to analyze the samples to detect Annexin V- and PI-positive subsets. Further analysis was performed with Flow Jo V10 software.
Statistical analysis
All experiments were repeated three times independently, and the average value was taken for analysis. The nonlinear mathematical model embedded in OriginPro 2021 was used to analyze and plot the experimental data.
Results and discussion
Single factor optimization
Effect of culture conditions on PES yield
The pH of fermentation medium plays a crucial role in microbial growth and metabolism, because it affects biosynthesis by affecting transport across cell membranes.[Citation22] As shown in , the highest EPS yield of 0.574 mg/mL was observed when the initial pH of the culture medium was around 6. Deviating from this optimal pH value, either toward acidity or alkalinity, resulted in a decrease in EPS yield. According to relevant studies, most LAB species thrive in weakly acidic conditions, which provide a favorable growth environment.[Citation23,Citation24] However, extreme pH values can disrupt enzyme activity and cell membrane permeability in microorganisms.[Citation25] The effect of the pH on EPS yield was various in different species.[Citation26] Studies have shown that controlling the pH during fermentation can significantly increase the yield of EPS,[Citation27] with pH-controlled conditions leading to more than three times higher EPS yield compared to uncontrolled pH conditions.[Citation28]
Figure 1. The influence of culture conditions. Effect of (a) pH, (b) inoculation volume, (c) time, (d) temperature on EPS production.
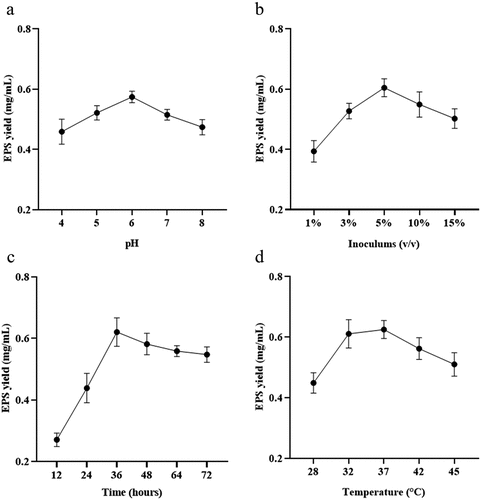
The inoculation amount is a critical factor in fermentation. demonstrated that the EPS yield initially increased and then decreased as the inoculation amount varies from 1% to 15%. The maximum yield of EPS (0.605 mg/mL) was observed at an inoculation amount of 5%. Exceeding the optimal inoculation amount can accelerate growth rate and nutrient consumption, leading to decreased yield.[Citation29] Higher inoculation amounts may result in the strain reaching saturation quickly, entering the decay phase, and experiencing bacterial autolysis, all of which negatively impact EPS yield.
Another important factor is the incubation time of microorganisms. Sufficient supply of nutrients and other growth factors in the early stage, depletion of nutrients and accumulation of toxic substances in the later stage, resulting in unfavorable conditions.[Citation30] As can be seen from , during the rapid growth phase, EPS yield increased as nutrients in the medium were utilized for bacterial propagation.[Citation31] However, as the culture time reached 36 h, EPS yield started to decline due to nutrient depletion, accumulation of toxic substances, and the transition of the strain from the stable phase to the decay phase. This transition is characterized by a decrease in the number of viable cells of the strain, swelling, and autolysis, all of which impede the strain’s ability to produce EPS.
The optimum temperature of different microorganisms is unique, which affects the growth, metabolism and enzyme activity of cells. showed that in the range of 32°C to 42°C, EPS production first increased and then decreased with increasing temperature, with the highest relative maximum EPS production (0.625 mg/mL) was observed at 37°C. Studies have shown that the optimal deproteinization range of LAB fermentation between 30 and 40°C.[Citation32] Relevant studies have shown that while an increase in temperature can enhance bacterial activity, excessively high temperatures can cause irreversible denaturation of proteins and nucleic acids in microorganisms, negatively affecting strain activity.[Citation33]
Effect of culture medium on EPS yield
Carbon and nitrogen sources are crucial for bacterial reproduction and polysaccharide biosynthesis.[Citation34] In terms of carbon sources, previous studies have shown that different carbon sources can influence EPS yield due to variations in EPS synthesis pathways[Citation31] and the production of specific enzymes[Citation35] in different species. In the analysis of different carbon sources, one study found that lactose increased the yield of EPS significantly, followed by glucose.[Citation36] In the study, glucose was found to be the most favorable carbon source for EPS production in L. plantarum YT013, followed by starch (. The strain showed enhanced growth and metabolism when glucose was used as the carbon source. Additionally, the highest EPS yield (0.584 mg/mL) was obtained with a glucose content of 3% (.
Figure 2. The influence of medium composition. Effect of (a) carbon source, (b) glucose concentration, (c) nitrogen source, (d) soy peptone concentration, (e) phosphate, (f) KH2PO4 concentration on EPS production.
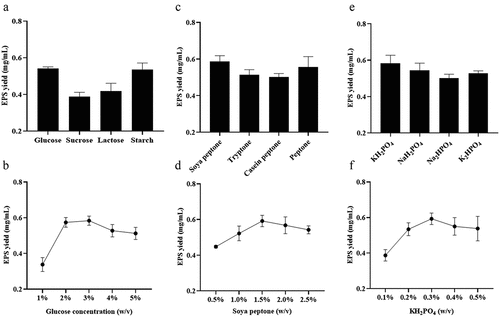
Nitrogen sources not only serve as precursors for cells component synthesis but can also affect the pH of the fermentation medium and influence enzyme activity and stability.[Citation37] In this study, different nitrogen sources, including tryptone, casein peptone, soy peptone, and protein, were analyzed for their impact on EPS yield. It was found that soy peptone resulted in the highest EPS yield (0.586 mg/mL) (. Furthermore, the increase in soy peptone concentration initially led to an increase in EPS production ( which is consistent with previous reports suggesting that higher carbon content and limited nitrogen concentration can greatly increase EPS yield. Studies have shown that the composition of organic nitrogen sources can improve the ability of L. plantarum MTCC9510 to EPS-producing, significantly.[Citation38]
Inorganic salts, even at low concentrations, can also affect EPS production by influencing the metabolic pathways of microorganisms.[Citation37] Among the studied inorganic salts (KH2PO4, NaH2PO4, Na2HPO4, K2HPO4), KH2PO4 had the greatest impact on EPS production (. The highest EPS yield (0.593 mg/mL) was achieved with a KH2PO4 concentration of 0.3% (. The addition of KH2PO4 can buffer the pH of the medium, which may be conducive to EPS production. Low concentrations of mineral elements are also essential factors in EPS production.[Citation18] The role of K+ in EPS production might vary among different strains, explaining the discrepancy with previous studies.[Citation39]
Nonlinear model for the EPS yield of YT013
The analysis of EPS yield over time was fitted using a nonlinear model equation. The model is the Voigt function, and the equation is as follows:
where y0 is the offset; A is the peak area; WL, WG and XC are the Lorentz width, Gaussian width and center of the parabola, respectively. The best fit of the nonlinear equation curve and correlation coefficient are listed in , which quantifies the deviation between the actual observed values and the inferred values based on the model. The simplified chi-square of the nonlinear model was 0.00269, the adjusted R2 value was 0.88476, and the fitting status code was successful (100). These results showed that the Voigt function could successfully fit the relationship between EPS yield and culture time.
Table 2. Best-fit nonlinear model equation and correlation factors (R-square) for EPS production.
From it is evident that the maximum EPS yield was obtained at 40 h under the optimal single-factor conditions, followed by a stability period. The fermentation time served as the independent variable in this analysis. When examining which represents the Voigt function, it can be observed that EPS production in L. plantarum YT013 initially increased and then stabilized over time. This observation aligns with the optimal results, indicating that the Voigt nonlinear model fits well with the data.
Figure 3. Fitting results under the best single factor condition. (a) EPS yield of different fermentation time under the best single factor condition; (b) Best-fit nonlinear model equation for EPS production.
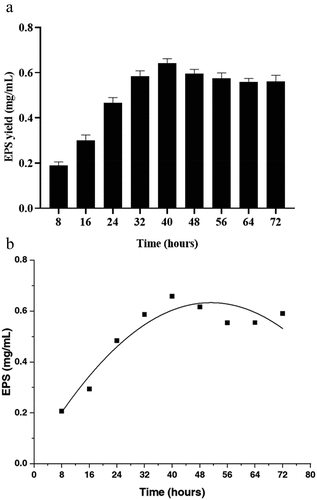
Overall, the Voigt function effectively captures the trend of EPS yield over time, with an initial increase followed by a stable period, as demonstrated by the successful fitting and agreement with the optimal results.
Antiproliferative activity
The antitumor effects of LAB EPSs have been reported in various studies, demonstrating their potential in inhibiting tumor cell proliferation. For example, when HepG-2 cells were treated with EPS at a concentration of 400 μg/mL for 48 h, a significant inhibition rate of 61.2% was observed.[Citation40] Another study revealed that EPS exhibited pronounced antitumor proliferative activity against A549 and BGC-823 cells.[Citation41] In the research, the effect of EPS derived from L. plantarum YT013 on the proliferation of AGS cells was investigated. From it can be observed that treating AGS cells with EPS at a concentration of 400 μg/mL for 24, 48, and 72 h resulted in the highest inhibitory effect at the 48-h time point. However, no statistically significant differences were observed in the inhibitory effects of EPS obtained from different fermentation periods on AGS cell proliferation. Previous studies have indicated that the composition of carbohydrates in the medium can affect EPS yield, but it may not alter its chemical structure.[Citation42] Furthermore, the effect of EPS on the apoptosis of AGS cells was evaluated by flow cytometry. The results shown in indicated that EPS could promote the apoptosis of AGS cells. This suggests that EPS may inhibit the proliferation of AGS cells by inducing apoptosis.
Figure 4. EPS sample and its inhibitory effect on AGS cell proliferation. (a) Fluffy and light-yellow EPS samples were obtained by dialysis and freeze-drying. (b) Cytotoxic effects of crude EPS (400 μg/mL) extracted at different culture times on the human AGS cancer cells. Inhibition effects of crude EPS on the human AGS cancer cells for 24, 48 and 72 h, respectively. (c) control group, (d) treated with EPS (400 μg/mL).
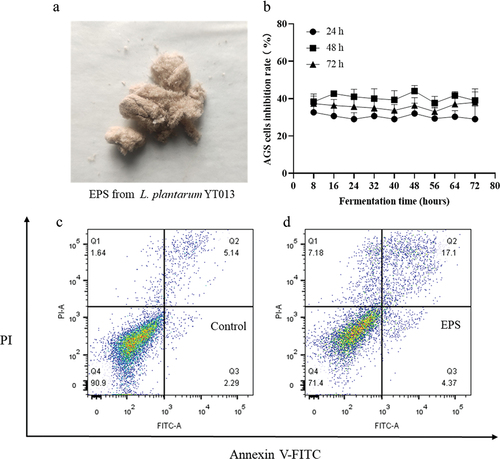
In summary, the findings suggest that EPS derived from L. plantarum YT013 has the potential to inhibit the proliferation of AGS cells, potentially through the promotion of apoptosis. These results contribute to the understanding of the antitumor effects of LAB EPS and support further exploration of their potential therapeutic applications.
Conclusion
In this study, the effects of different carbon and nitrogen sources, as well as growth conditions, on the production of EPS by L. plantarum YT013 were investigated. The results revealed that the optimal single-factor conditions for EPS production were an initial pH 6, inoculation amount 5% (v/v), temperature 37°C, cultivation time 36 h, glucose 3% (w/v), soy peptone 1.5% (w/v), KH2PO4 0.3% (w/v). The nonlinear model fitted well with the experimental data and could be used as an effective tool for the optimal conditions of EPS yield. Under these conditions, the EPS yield reached 0.630 mg/mL, which was 1.31 times higher than the baseline yield. Furthermore, the study demonstrated that the EPS produced by L. plantarum YT013 exhibited inhibitory effects on the proliferation of AGS cells, a type of tumor cell, and promoted apoptosis in these cells. These findings suggest that the EPS obtained from L. plantarum YT013 has potential as a natural antitumor drug.
Overall, this study enhanced our understanding of the characteristics of EPS derived from L. plantarum YT013 and highlighted its potential as a promising natural agent for combating tumors.
Ethical approval
The article does not include research involving humans or animals.
Author contributions
PC and RZ contributed to the study design and drafted the manuscript. ZZ, YM, KD and HT collected, analyzed and interpreted the data. XJ, LT and JL contributed to the conception and design of the current study. HZ, MS and YN helped perform the analysis with constructive discussions. All authors read and approved the final manuscript.
Disclosure statement
No potential conflict of interest was reported by the author(s).
Data availability statement
The authors confirm that the data supporting the findings of this study are available within the article.
Additional information
Funding
References
- Cerning, J. Exocellular Polysaccharides Produced by Lactic Acid Bacteria. FEMS Microbiol. Rev. 1990, 87(1–2), 113–130. https://doi.org/10.1111/j.1574-6968.1990.tb04883.x.
- Yang, Z.; Li, S.; Zhang, X.; Zeng, X.; Li, D.; Zhao, Y.; Zhang, J. Capsular and Slime-Polysaccharide Production by Lactobacillus Rhamnosus JAAS8 Isolated from Chinese Sauerkraut: Potential Application in Fermented Milk Products. J. Biosci. Bioeng. 2010, 110(1), 53–57. DOI: 10.1016/j.jbiosc.2009.12.010.
- Castillo Pedraza, M. C.; Novais, T. F.; Faustoferri, R. C.; Quivey, R. G.; Terekhov, A.; Hamaker, B. R.; Klein, M. I. Extracellular DNA and Lipoteichoic Acids Interact with Exopolysaccharides in the Extracellular Matrix of Streptococcus Mutans Biofilms. Biofouling. 2017, 33(9), 722–740. DOI: 10.1080/08927014.2017.1361412.
- Bajpai, V. K.; Rather, I. A.; Park, Y. H. Partially Purified Exo-Polysaccharide from Lactobacillus Sakei Probio 65 with Antioxidant, α-Glucosidase and Tyrosinase Inhibitory Potential. J. Food Biochem. 2016, 40(3), 264–274. DOI: 10.1111/jfbc.12230.
- Lynch, K. M.; Zannini, E.; Coffey, A.; Arendt, E. K. Lactic Acid Bacteria Exopolysaccharides in Foods and Beverages: Isolation, Properties, Characterization, and Health Benefits. Annu. Rev. Food Sci. Technol. 2018, 9(1), 155–176. https://doi.org/10.1146/annurev-food-030117-012537.
- Urshev, Z. L.; Dimitrov, Z. P.; Fatchikova, N. S.; Petrova, I. G.; Ishlimova, D. I. Partial Characterization and Dynamics of Synthesis of High Molecular Mass Exopolysaccharides from Lactobacillus delbrueckii Ssp. Bulgaricus and Streptococcus Thermophilus Bulgaricus and Streptococcus thermophilus. World J. Microb Biot. 2007, 24(2), 171–179. DOI: 10.1007/s11274-007-9453-0.
- Zannini, E.; Waters, D. M.; Coffey, A.; Arendt, E. K. Production, Properties, and Industrial Food Application of Lactic Acid Bacteria-Derived Exopolysaccharides. Appl. Microbiol. Biotechnol. 2016, 100(3), 1121–1135. DOI: 10.1007/s00253-015-7172-2.
- Prajapati, V. D.; Jani, G. K.; Khanda, S. M. Pullulan: An Exopolysaccharide and Its Various Applications. Carbohydr. Polym. 2013, 95(1), 540–549. DOI: 10.1016/j.carbpol.2013.02.082.
- Zhou, Y.; Cui, Y.; Qu, X. Exopolysaccharides of Lactic Acid Bacteria: Structure, Bioactivity and Associations: A Review. Carbohydr. Polym. 2019, 207, 317–332. DOI: 10.1016/j.carbpol.2018.11.093.
- Nwodo, U.; Green, E.; Okoh, A. Bacterial Exopolysaccharides: Functionality and Prospects. Int. J. Mol. Sci. 2012, 1(12), 14002–14015. DOI: 10.3390/ijms131114002.
- Liang, T. W.; Wang, S. L. Recent Advances in Exopolysaccharides from Paenibacillus Spp.: Production, Isolation, Structure, and Bioactivities. Mar. Drugs. 2015, 13(4), 1847–1863. DOI: 10.3390/md13041847.
- Wang, J.; Zhao, X.; Yang, Y.; Zhao, A.; Yang, Z. Characterization and Bioactivities of an Exopolysaccharide Produced by Lactobacillus Plantarum YW32. Int. J. Biol Macromol. 2015, 74, 119–126. DOI: 10.1016/j.ijbiomac.2014.12.006.
- Ismail, B.; Nampoothiri, K. M. Exposition of Antitumour Activity of a Chemically Characterized Exopolysaccharide from a Probiotic Lactobacillus Plantarum MTCC 9510. Biologia. (Bratisl). 2013, 68(6), 1041–1047. DOI: 10.2478/s11756-013-0275-2.
- Wang, K.; Li, W.; Rui, X.; Chen, X.; Jiang, M.; Dong, M. Characterization of a Novel Exopolysaccharide with Antitumor Activity from Lactobacillus Plantarum 70810. Int. J. Biol Macromol. 2014, 63, 133–139. DOI: 10.1016/j.ijbiomac.2013.10.036.
- Jiang, B.; Tian, L.; Huang, X.; Liu, Z.; Jia, K.; Wei, H.; Tao, X. Characterization and Antitumor Activity of Novel Exopolysaccharide APS of Lactobacillus Plantarum WLPL09 from Human Breast Milk. Int. J .Biol Macromol. 2020, 163, 985–995. DOI: 10.1016/j.ijbiomac.2020.06.277.
- Adesulu-Dahunsi, A. T.; Sanni, A. I.; Jeyaram, K.; Ojediran, J. O.; Ogunsakin, A. O.; Banwo, K. Extracellular Polysaccharide from Weissella confusa OF126: Production, Optimization, and Characterization. Int. J. Biol Macromol. 2018, 111, 514–525. DOI: 10.1016/j.ijbiomac.2018.01.060.
- Zhou, X.; Qi, W.; Hong, T.; Xiong, T.; Gong, D.; Xie, M.; Nie, S. Exopolysaccharides from Lactobacillus Plantarum NCU116 Regulate Intestinal Barrier Function via STAT3 Signaling Pathway. J. Agric. Food. Chem. 2018, 66(37), 9719–9727. DOI: 10.1021/acs.jafc.8b03340.
- Wu, Z.; Lu, J.; Wang, X.; Hu, B.; Ye, H.; Fan, J.; Abid, M.; Zeng, X. Optimization for Production of Exopolysaccharides with Antitumor Activity in vitro from Paecilomyces Hepiali. Carbohydr. Polym. 2014, 99, 226–234. DOI: 10.1016/j.carbpol.2013.08.010.
- Wang, X.; Shao, C.; Liu, L.; Guo, X.; Xu, Y.; Lü, X. Optimization, Partial Characterization and Antioxidant Activity of an Exopolysaccharide from Lactobacillus Plantarum KX041. Int. J. Biol Macromol. 2017, 103, 1173–1184. DOI: 10.1016/j.ijbiomac.2017.05.118.
- Xiao, L.; Ge, X.; Yang, L.; Chen, X.; Xu, Q.; Rui, X.; Fan, X.; Feng, L.; Zhang, Q.; Dong, M., et al. Anticancer Potential of an Exopolysaccharide from Lactobacillus helveticus MB2-1 on Human Colon Cancer HT-29 Cells via Apoptosis Induction. Food. Funct. 2020, 11(11), 10170–10181. DOI: 10.1039/d0fo01345e.
- Zhang, R.; Zhou, Z.; Ma, Y.; Du, K.; Sun, M.; Zhang, H.; Tu, H.; Jiang, X.; Lu, J.; Tu, L., et al. Anti-Gastric Cancer Activity of the Cell-Free Culture Supernatant of Serofluid Dish and Lactiplantibacillus plantarum YT013. Front. Bioeng. Biotechnol. 2022, 10, 10. DOI: 10.3389/fbioe.2022.898240.
- Suberu, Y.; Akande, I.; Samuel, T.; Lawal, A.; Olaniran, A. Optimization of Protease Production in Indigenous Bacillus Species Isolated from Soil Samples in Lagos, Nigeria Using Response Surface Methodology. Biocatal Agric. Biotechnol. 2019, 18, 101011. DOI: 10.1016/j.bcab.2019.01.049.
- Adamberg, K. The Effect of Temperature and pH on the Growth of Lactic Acid Bacteria: A Ph-Auxostat Study. Int. J. Food Microbiol. 2003, 85(1–2), 171–183. DOI: 10.1016/s0168-1605(02)00537-8.
- Rhee, S. K.; Pack, M. Y. Effect of Environmental pH on Fermentation Balance of Lactobacillus Bulgaricus. J. Bacteriol. 1980, 144(1), 217–221. DOI: 10.1128/jb.144.1.217-221.1980.
- Bhunia, B.; Basak, B.; Mandal, T.; Bhattacharya, P.; Dey, A. Effect of pH and Temperature on Stability and Kinetics of Novel Extracellular Serine Alkaline Protease (70 kDa). Int. J. Biol. Macromol. 2013, 54, 1–8. DOI: 10.1016/j.ijbiomac.2012.11.024.
- Li, W.; Ji, J.; Chen, X.; Jiang, M.; Rui, X.; Dong, M. Structural Elucidation and Antioxidant Activities of Exopolysaccharides from Lactobacillus helveticus MB2-1. Carbohydr. Polym. 2014, 102, 351–359. DOI: 10.1016/j.carbpol.2013.11.053.
- Raza, W.; Makeen, K.; Wang, Y.; Xu, Y.; Qirong, S. Optimization, Purification, Characterization and Antioxidant Activity of an Extracellular Polysaccharide Produced by Paenibacillus polymyxa SQR-21. Bioresources. Technol. 2011, 102(10), 6095–6103. DOI: 10.1016/j.biortech.2011.02.033.
- Ding, X.; Hou, Y. L.; Hou, W. R. Structure Elucidation and Antioxidant Activity of a Novel Polysaccharide Isolated from Boletus Speciosus Forst. Int. J. Biol Macromol. 2012, 50(3), 613–618. DOI: 10.1016/j.ijbiomac.2012.01.021.
- Abusham, R. A.; Rahman, R. N. Z. R.; Salleh, A. B.; Basri, M. Optimization of Physical Factors Affecting the Production of Thermo-Stable Organic Solvent-Tolerant Protease from a Newly Isolated Halo Tolerant Bacillus subtilis Strain Rand. Microb. Cell Fact. 2009, 8(1), 20. DOI: 10.1186/1475-2859-8-20.
- Banerjee, S.; Maiti, T. K.; Roy, R. N. Protease Production by Thermo-Alkaliphilic Novel Gut Isolate Kitasatospora Cheerisanensis GAP 12.4 from Gryllotalpa Africana. Biocatal. Biotransform. 2017, 35(3), 168–176. DOI: 10.1080/10242422.2017.1306739.
- Imran, M. Y.; Reehana, N.; Jayaraj, K. A.; Ahamed, A. A. P.; Dhanasekaran, D.; Thajuddin, N.; Alharbi, N. S.; Muralitharan, G. Statistical Optimization of Exopolysaccharide Production by Lactobacillus Plantarum NTMI05 and NTMI20. Int. J. Biol Macromol. 2016, 93, 731–745. DOI: 10.1016/j.ijbiomac.2016.09.007.
- Pacheco, N.; Garnica-Gonzalez, M.; Ramirez-Hernandez, J. Y.; Flores-Albino, B.; Gimeno, M.; Bárzana, E.; Shirai, K. Effect of Temperature on Chitin and Astaxanthin Recoveries from Shrimp Waste Using Lactic Acid Bacteria. Bioresources. Technol. 2009, 100(11), 2849–2854. DOI: 10.1016/j.biortech.2009.01.019.
- Mehta, A.; Bodh, U.; Gupta, R. Isolation of a Novel Lipase Producing Fungal Isolate Aspergillus Fumigatus and Production Optimization of Enzyme. Biocatal. Biotransformation. 2018, 36(6), 450–457. DOI: 10.1080/10242422.2018.1447565.
- Gandhi, H. P.; Ray, R. M.; Patel, R. M. Exopolymer Production by Bacillus Species. Carbohydr. Polym. 1997, 34(4), 323–327. DOI: 10.1016/S0144-8617(97)00132-X.
- Taneja, K.; Kumar Bajaj, B.; Kumar, S.; Dilbaghi, N. Process Optimization for Production and Purification of Novel Fibrinolytic Enzyme from Stenotrophomonas sp. KG-16-3. Biocatal. Biotransformation. 2018, 37(2), 124–138. DOI: 10.1080/10242422.2018.1504925.
- Petry, S.; Furlan, S.; Crepeau, M. J.; Cerning, J.; Desmazeaud, M. Factors Affecting Exocellular Polysaccharide Production by Lactobacillus delbrueckii Subsp. Bulgaricus Grown in a Chemically Defined Medium. Appl. Environ. Microbiol. 2000, 66(8), 3427–3431. DOI: 10.1128/AEM.66.8.3427-3431.2000.
- Li, Q. M.; Shi, Z.; Xiong, X. Y.; Wen, Q.; Hu, Q.-L.; Su, X.-J. Ethanol Production from Xylose by Fusarium oxysporum and the Optimization of Culture Conditions. Biocatal. Biotransformation. 2016, 34(3), 110–118. DOI: 10.1080/10242422.2016.1212848.
- Zou, X. Optimization of Nutritional Factors for Exopolysaccharide Production by Submerged Cultivation of the Medicinal Mushroom Oudemansiella Radicata. World J. Microb. Bio. 2005, 21(6–7), 1267–1271. DOI: 10.1007/s11274-005-1941-5.
- Cui, J. D.; Zhang, Y. N. Evaluation of Metal Ions and Surfactants Effect on Cell Growth and Exopolysaccharide Production in Two-Stage Submerged Culture of Cordyceps Militaris. Appl. Biochem. Biotechnol. 2012, 168(6), 1394–1404. DOI: 10.1007/s12010-012-9865-7.
- Xue, Q.; Sun, J.; Zhao, M.; Zhang, K.; Lai, R. Immunostimulatory and Anti-Tumor Activity of a Water-Soluble Polysaccharide from Phellinus Baumii Mycelia. World J. Microb. Bio. 2010, 27(5), 1017–1023. DOI: 10.1007/s11274-010-0545-x.
- Wei, D.; Wei, Y.; Cheng, W.; Zhang, L. Sulfated Modification, Characterization and Antitumor Activities of Radix Hedysari Polysaccharide. Int. J. Biol. Macromol. 2012, 51(4), 471–476. DOI: 10.1016/j.ijbiomac.2012.06.004.
- Sirajunnisa, A. R.; Vijayagopal, V.; Sivaprakash, B.; Viruthagiri, T.; Surendhiran, D. Optimization, Kinetics and Antioxidant Activity of Exopolysaccharide Produced from Rhizosphere Isolate, Pseudomonas fluorescens CrN6. Carbohydr. Polym. 2016, 135, 35–43. DOI: 10.1016/j.carbpol.2015.08.080.