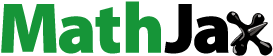
ABSTRACT
Oats are among the most valued cereal commodities in the food market and are considered an exceptional grain. This study aimed focuses on assessing the impact of starter cultures, including lactic acid bacteria (i.e. Streptococcus thermophilus and Lactobacillus delbrueckii subsp. bulgaricus), Bifidobacterium animalis subsp. lactis Bb-12, and Propionibacterium freudenreichii subsp. shermanii PS-4, on selected quality parameters of yogurt-type fermented oat drinks. These drinks were produced by aqueous extraction of commercial oat flakes in drinking water. Acidification curves were determined during fermentation, and the oat drinks were subsequently stored at 6°C for 35 days. Throughout the study, acidity (measured by pH values), populations of lactic acid bacteria, bifidobacteria, and propionibacteria, as well as texture attributes (hardness and adhesiveness), water-holding capacity, and selected carbohydrate content were examined at the end of fermentation and every 7 days during storage. Results indicated that the addition of bifidobacteria and/or propionic acid bacteria in oat drink cultures expedited and improved the acidification process, resulting in a drink with a lower pH. Furthermore, the incorporation of propionic acid bacteria enhanced the water-holding capacity of the drink. Although the fermentation process had no significant impact on the texture of the oat drinks, the introduction of bifidobacteria reduced the adhesiveness of the final product. Carbohydrate content in the oat drinks diminished during fermentation, with sucrose experiencing the most substantial reduction. In conclusion, the study suggests that supplementing oat drink cultures with bifidobacteria and/or propionic acid bacteria proves to be an effective strategy for enhancing both the quality and shelf life of the final product.
Introduction
Fermentation is a biological process where microorganisms such as bacteria or yeast metabolize nutrients in raw foods, transforming them into new substances.[Citation1] Fermented products contribute essential microflora to the digestive system, featuring bacteria from genera such as Lactobacillus, Bifidobacterium, Lactococcus, Pediococcus, and Propionibacterium. In dairy products, fermentation is well known for yogurt and kefir, where lactic acid bacteria are used to ferment lactose. According to the definitions provided by the Food and Agriculture Organization of the United Nations Food and Agriculture Organization and the World Health Organization (FAO/WHO), as well as the International Dairy Federation (IDF), fermented milk is described as “products made from whole, partially skimmed, or fully skimmed milk, condensed milk, or reconstituted milk powder, fermented by specific microorganisms.” These microorganisms lower the milk’s pH by fermenting lactose, causing it to coagulate. This microflora must remain alive, active, and numerous in the final product until its last day of shelf life.[Citation2] Given these attributes, such products can be classified as functional foods due to their particularly positive physiological effects on the body. The growing popularity and variety of fermented milk beverages are primarily attributable to the health-promoting benefits they offer. These benefits are delivered by probiotics. Etymologically, the term “probiotic” is of Greek origin, and it means “for life.” Probiotics are carefully selected, live or dormant, active cultures used in the technological process. They must meet specific requirements, including the ability to improve the microbial balance of the gut.[Citation3]
However, fermentation is not limited to dairy products alone.[Citation4–7] One of the main reasons for its increasing popularity is the public’s diverse dietary needs and preferences. Many people face challenges such as lactose intolerance or milk protein allergies, while a plant-based diet is becoming popular for ethical and environmental reasons. The long-standing trend of reducing or eliminating meat consumption has resulted in a wide range of plant-based foods on the market. Increasing interest in plant-based nutrition has led to the development of innovative fermentation techniques specifically for plant-based products.[Citation8] The fermentation of plant-based drinks, including those made from nuts, cereal grains, or legumes, provides a viable alternative for people seeking not only flavor variety but also the health and functional benefits of the process. Proper bacterial culture selection for these products takes into account both the temperature of the fermentation process and the expected properties of the resulting product.[Citation4,Citation9,Citation10]
Oat grain stands out as one of the most valued cereal commodities in the food market and is considered an exceptional grain. Compared to other cereals, oats are distinguished by their higher content of protein, fat, and dietary fiber, as well as a significant content of vitamins and minerals.[Citation7,Citation11–14] The distinctive health-promoting properties of oats reinforce their uniqueness among cereals. β-glucans are mainly responsible for these benefits, although they are most effective when oat products are consumed regularly over a long period and the amount in the diet exceeds certain thresholds established for this polysaccharide.[Citation15,Citation16] In response to the growing interest in plant-based diets and consumer expectations, the food industry is increasingly offering a wide variety of fermented plant-based drinks such as yogurts. By utilizing a variety of raw materials, manufacturers can produce drinks with interesting flavors and textures, as well as confer beneficial health properties.[Citation16] In the present study, the aim was to evaluate the impact of starter cultures of lactic acid bacteria, bifidobacteria, and propionibacteria on selected quality parameters of yogurt-type fermented oat drinks. Currently, the growing interest in fermented oat drinks prompted us to conduct research in which we assessed the impact of fermentation on selected quality parameters of these products. Unlike studies cited in the literature, our research focused on the use of Bifidobacterium and Propionibacterium bacterial strains.
Materials and methods
Materials
The materials used for the experiments described below included three starter cultures obtained from Chr. Hansen (Chr. Hansen Poland, Czosnów, Poland): YC-X16 (comprising Streptococcus thermophilus and Lactobacillus delbrueckii subsp. bulgaricus), BB12 (composed of Bifidobacterium animalis subsp. lactis Bb-12), and Propionibacterium PS-4 (comprising Propionibacterium freudenreichii subsp. shermanii PS-4). These starter cultures were strategically selected based on their availability in the market, ensuring the feasibility of future industrial-scale production of fermented oat drinks using these readily available probiotics. These starter cultures were stored in a freezer at − 18°C until utilized in the experiments. They were used for fermenting oat drinks made from commercial oat flakes (Kupiec Sp. z o. o., Krzymów, Poland). According to the nutritional information provided on the packaging, the oat flakes had the following values per 100 g of the product: energy—418.00 kcal, fat—7.60 g (of which saturated fatty acids constituted 1.40 g), carbohydrates—69.00 g (including sugars—1.30 g), fibre—9.00 g, protein—14.00 g, and salt—0.02 g.
Analysis design and treatments
The oat drink was obtained under laboratory conditions using aqueous extraction of 60.0 g of commercial oat flakes in 700 mL of drinking water. The oat flakes, once soaked in water, were brought to a boil and the mixture was then simmered for 20 min before being blended. The resulting liquid was filtered through a sieve with a 0.1 mm mesh size and subsequently homogenized using a laboratory homogenizer L4R (Silverson, Chesham, UK). Portions of the oat drink, measured at 180 mL each and placed in glass jars, were sterilized at 121°C for 15 min. The day after sterilization, these portions were inoculated with bacteria. Inoculums were prepared by rehydrating the freeze-dried starter cultures in a sterile saline solution. The oat drink portions were then inoculated with the starter cultures to achieve an initial cell density of approximately 6–7 log CFU/mL and incubated at 38°C for 20 h. Different combinations of starter cultures were used in the fermentation of the oat drinks:
YC-X16 starter culture (samples coded as YC).
YC-X16 + BB12 starter cultures (samples coded as YC-BB).
YC-X16 + Propionibacterium PS-4 starter cultures (samples coded as YC-PS); and
YC-X16 + BB12 + Propionibacterium PS-4 starter cultures (samples coded as YC-BB-PS).
After fermentation, samples of the fermented oat drink were refrigerated at 6°C and stored at this temperature for 35 days. The entire experiment was conducted twice for verification. During the fermentation process, acidification curves were determined by measuring the pH value at 2 h. Immediate completion of fermentation, and at 7-day intervals during refrigerated storage, the fermented oat drink samples were examined for various parameters. These included acidity (measured by pH value), populations of lactic acid bacteria, bifidobacteria, and propionibacteria, as well as texture attributes like hardness and adhesiveness. Additionally, water-holding capacity (WHC) and carbohydrate content were assessed. The entire experiment was repeated twice.
Analyses
For measuring the pH value, a mixed sample of the fermented oat drink was prepared. A pH meter electrode and a temperature sensor were inserted into the sample.[Citation17] Duplicate measurements were conducted to ensure accuracy. The maximum acidification rate (Vmax) was determined using EquationEquation (1)(1)
(1) as proposed by Bezerra et al.[Citation18]
To determine the populations of lactic acid bacteria, bifidobacteria, and propionibacteria, the traditional plate method was employed following the ISO 6887–5:2020 standard.[Citation20] The De Man, Rogosa, and Sharpe agar medium (MRS agar; Merck, Darmstadt, Germany) was used to determine the lactobacilli population, while the M17 agar medium (M17 agar; Merck, Darmstadt, Germany) was used for streptococci. The Bifidus Selective Medium (BSM) agar (Merck, Darmstadt, Germany) was used to determine the population of B. animalis subsp. lactis Bb-12. Plates with MRS and BSM agars were incubated at 37°C for 72 h under anaerobic conditions, using Anaerocult jars (Merck, Darmstadt, Germany) and DeltaChrom™ A (Merck, Darmstadt, Germany) for anaerobic cultures. M17 agar plates were incubated under aerobic conditions at 37°C for 72 h. For the population of P. freudenreichii subsp. shermanii, the medium contained yeast extract (10 g/L), Na2HPO4 × H2O (3 g/L), KH2PO4 (1 g/L), sodium lactate (40 g/L), and agar – agar (15.0 g/L).[Citation17] The pH of this medium was set between 7.0 and 7.2 at 25°C. These agar plates were incubated at 37°C for 7 days under anaerobic conditions. After incubation, the number of colonies that had grown was counted and converted to colony-forming units per millilitre of sample (CFU/mL). The results were expressed as the mean values of the decadic logarithm of the total cell count. Duplicate incubations were performed to ensure accuracy. The presence of contaminating microflora was concurrently assessed. The total numbers of moulds and yeasts were determined using YGC medium (Merck, Darmstadt, Germany), with plates incubated aerobically at 25°C for 5 days. The total number of Enterobacteriaceae was ascertained using the VRBG medium (Merck, Darmstadt, Germany). These plates were overlaid on the same medium and incubated anaerobically at 37°C for 24 h. All media were prepared according to the manufacturer’s instructions.
Texture analysis of the fermented oat drink samples involved measuring hardness and adhesiveness as delineated in the procedure by Ziarno and Zaręba.[Citation17] For this purpose, a Texture Analyzer CT3 10 K (Brookfield Engineering Laboratories Inc., Middleborough, MA, USA) was employed, using the TexturePro CT V 1.4 Build 17 software (Brookfield Engineering Laboratories Inc., Middleborough, MA, USA). The test type used was TPA (Texture Profile Analysis). The measurements were carried out using the TA4/1000 probe, which is cylindrical, with a diameter of 38.1 mm and a height of 20 mm. The tests were performed at a temperature of 6°C. During the testing, a force of 0.04 N was applied to the sample. The probe moved toward the sample at a speed of 2 mm/s and retracted at a speed of 4.5 mm/s. The measurement was performed in duplicates. The WHC of fermented oat drink samples was determined following the method described by Ziarno and Zaręba.[Citation17] Briefly, 40.00 g of sample was centrifuged at 3,250 × g at 4°C for 20 min. After centrifugation, the supernatant was decanted and weighed. The measurement was repeated twice. WHC (%) was calculated according to the following formula (EquationEquation (2)(2)
(2) ):
The carbohydrate content of the fermented oat drink samples was determined using high-performance liquid chromatography (HPLC), following the method described by Ziarno et al..[Citation21] Briefly, carbohydrates were extracted from the samples by mixing 8.00 g of the fermented oat drink with 32.00 g of methanol (Avantor Performance Materials Poland, Gliwice, Poland). The mixtures were then intensively mixed, placed in an ultrasonic bath at 30°C for 30 min, and centrifuged using an MPW-350 R centrifuge (MPW Med. Instruments, Warsaw, Poland) at 16,000 × g for 30 min at 4°C. Next, the mixtures were filtered through a 0.45 µm syringe filter (Merck, Darmstadt, Germany), and the supernatants were analyzed using HPLC. The analytes were separated using an HPLC kit equipped with DeltaChrom™ pumps (Sykam, Fürstenfeldbruck, Germany), a Needle Injection Valve dosing loop (0.01 mL; S6020; Sykam), a DeltaChrom™ Temperature Control Unit column temperature controller (Sykam), and a 05397–51 Cosmosil Sugar-D column (4.6 ID × 250 mm, Cosmosil, Nacalai Tesque, Kyoto, Japan), which was secured by a Pre-column 05394–81 Cosmosil Guard Column Sugar-D (4.6 ID × 10 mm, Cosmosil). The analytes were detected using a refractive index detector (S3580 RI; Sykam). The mobile phase used was a mixture of acetonitrile (Avantor) and deionized water at a ratio of 4:1. Analyses were performed using isocratic elution. Each fermented oat drink sample was analyzed for 30 min. The peaks were identified by comparing the obtained retention times to those of selected carbohydrate standards, including fructose, glucose, maltose, sucrose, raffinose, and stachyose (Merck, Darmstadt, Germany). The content of selected carbohydrates in the fermented oat drink samples was calculated based on the area under the peak of the identified carbohydrate, considering the concentration degree of the initial sample. Each fermented oat drink sample was analyzed twice
A two-factor analysis of variance (MANOVA) was used for the statistical analysis. This MANOVA analysis was carried out using the Statgraphics Centurion XVII program (Statgraphics Technologies, Inc., The Plains, VA, USA). In conjunction with the MANOVA, Tukey’s test and a significance coefficient α of 0.05 were used.
Results and discussion
Acidification curve
The acidification curve of plant-based drinks represents the graphical representation of pH changes during the fermentation process (). Acidification curves help to understand how the production of acids by starter microorganisms affects the pH of these drinks. Statistical analysis showed that the final pH of the drink is determined by the type of plant-based drink, the starter culture used, and the fermentation conditions. Studies have shown that the microbiological composition of starter cultures plays a statistically significant role in pH reduction. The choice of starter cultures emerges as a statistically significant determinant of the final pH. The most substantial drops in pH in the fermented samples occurred during the initial 8–10 h. Notably, the addition of bifidobacteria, either alone or in combination with propionic acid bacteria, considerably statistically accelerates the rate of acidification in oat drink samples. This synergistic effect of bifidobacteria and propionic acid bacteria is clearly illustrated in the calculated Tmax and Te values (). In oat drink samples fermented with a mixture of yogurt bacteria and bifidobacteria and/or propionic acid bacteria, a significantly shorter fermentation process was observed compared to samples fermented with only yogurt bacteria.
Figure 1. Acidification curve of oat drink by selected combinations of starter cultures. a,b,c,d same lowercase letters indicate no statistically significant difference (α = 0.05; n = 4). Legend: YC – oat drink samples fermented with a starter culture YC-X16; YC-BB – oat drink samples fermented by the starter culture YC-X16 and Bifidobacterium animalis subsp. lactis BB-12; YC-PS – oat drink samples fermented with the starter culture YC-X16 and Propionibacterium PS-4; and YC-BB-PS – oat drink samples fermented with a starter culture YC-X16, Bifidobacterium animalis subsp. lactis BB-12 and Propionibacterium PS-4.
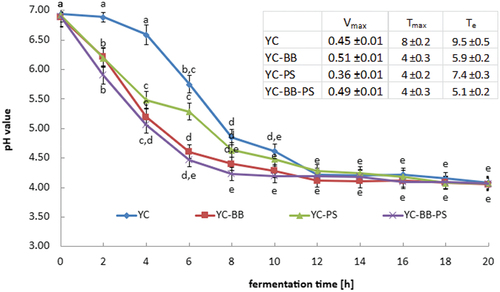
The optimal fermentation processes are a crucial consideration in drink manufacturing. The strategic inclusion of specific microbial strains could prove instrumental in achieving desired pH levels efficiently. In particular, manufacturers can leverage our insights to tailor their processes. Significant falls in pH value within the initial 8–10 h of the fermentation process are in agreement with the results reported by other researchers.[Citation22,Citation23] The Vmax values calculated in these studies are the same level as the results obtained by Luana et al..[Citation24] Quoted researchers determined the Vmax of drinks made with oat flakes fermented with L. plantarum LP09, without or with enzymatic oat treatment. The obtained Vmax values were 0.30 ± 0.02 and 0.76 ± 0.01, respectively. Meanwhile, Coda et al.[Citation25] calculated a Vmax of 0.15–0.22 for oat drinks fermented by various strains of L. plantarum. The oat drink samples were made from a mixture of 6% oat flour, 10% concentrated red grape must, 12% rice flour, and 72% tap water, which can explain the lower Vmax values calculated for these samples. According to Lazaridou et al.[Citation26] not only are the fermenting microorganisms crucial for acidification parameters and acidification kinetics, but also the composition of the drink, as well as the temperature and time of the fermentation process, are also key. Quoted researchers have proved that the addition of β-glucan addition increases the value of acidification parameters and acidification kinetics of fermented milk. While our study offers valuable insights, it is essential to acknowledge its limitations. Future research could delve deeper into understanding the interplay of additional variables, such as temperature and specific strain variations, to refine our comprehension of the acidification process.
Change in pH during refrigerated storage of fermented oat drinks
The initial pH value of the obtained fermented oat drink samples ranged from 4.11 to 4.14, statistically regardless of the type of starter cultures used in production (). No statistically significant differences in pH values were observed during the refrigerated storage of these fermented oat drinks. This low pH proves that oat drinks provide a suitable matrix for fermentation by lactic acid bacteria, whether or not bifidobacteria or propionic acid bacteria are included. No statistically significant differences were discerned, underscoring the robust nature of the fermentation process and the resilience of the oat drink matrix. The sustained low pH values observed during storage have significant implications for quality assurance. A stable pH is indicative of a well-controlled fermentation process. This aspect contributes to the overall quality of the final products, aligning with industry standards.
Table 1. Change in pH during refrigerated storage of fermented oat drinks (average values and standard deviations).
Low pH results in oat-based matrices fermented with selected lactic acid bacteria – such as S. thermophilus, L. acidophilus, L. delbrueckii subsp. bulgaricus, L. delbrueckii subsp. lactis, L. plantarum, L. casei, and L. paracasei strains – as well as bifidobacteria, have also been reported by other researchers.[Citation24,Citation27–34] They observed a decline in pH under refrigerated storage conditions, attributing it to the metabolic activity of the starter and probiotic bacteria used. These observations have been corroborated by additional research focused on oat-based fermented drinks.[Citation29,Citation30] Meanwhile, Part et al.[Citation35] examined the acidity of commercially available plant-based yogurt alternatives, reporting a pH range of 3.8–4.4 for oat-based samples.
The stability in pH values observed in our study carries practical implications for the shelf life of fermented oat drinks. Understanding how pH remains constant under refrigerated conditions is crucial information to ensure optimal product storage and longevity. Unlike this research, many researchers have noted a significant decrease in pH value during refrigeration tests. This decline is typically attributed to the potential production of lactic acid by lactic acid bacteria during storage, a phenomenon known as “cold postacidification.”[Citation31,Citation36,Citation37] However, research findings also exist that indicate a lack of significant pH changes during the refrigeration of oat-based drink samples.[Citation29] In conclusion, our analysis of pH changes during refrigerated storage not only emphasizes the reliability of the fermentation process but also sheds light on the multiple factors that contribute to the quality and consumer appeal of fermented oat drinks. Investigating the effects of different storage conditions or examining microbial dynamics long-term storage provide additional insights into the long-term stability of fermented oat drinks.
Change in bacterial cell population during refrigerated storage of fermented oat drinks
Our investigation into the microbial dynamics during refrigerated storage provides valuable insights into the changes in bacterial cell populations, encompassing lactic acid bacteria, bifidobacteria, and propionic acid bacteria. The initial population of lactic acid bacteria, bifidobacteria, and propionic acid bacteria exceeded 7 log (CFU/g). The refrigeration period led to a statistically significant reduction in the population of live cells for all types of bacteria studied, although the degree of their extinction depended on the time and type of starter culture (). The strongest extinction was observed in bifidobacteria and propionic acid bacteria, while the best survival rates were observed for S. thermophilus. A notable aspect of our results is the possible connection between the reduction in bacterial cell population and the stability of pH values observed during refrigerated storage. Investigating these relationships provides a more comprehensive understanding of the microbial ecology in fermented oat drinks.
Table 2. Change in bacterial cell population [log(CFU/g)] during refrigerated storage of fermented oat drinks (average values and standard deviations).
Concurrently, the presence of unwanted microorganisms – namely the combined counts of molds and yeasts, as well as the Enterobacteriaceae population – was assessed. The results indicated that molds, yeast, and Enterobacteriaceae were not detected in any of the refrigerated storage samples of fermented oat drinks.
The different levels of extinction observed in different bacteria species led to an investigation into contributing factors. According to the Codex Alimentarius Standard for Milk and Milk Products (Codex Alimentarius, 2010), a minimum dose of 7 log(CFU/g) for microorganisms constituting the starter culture and a minimum dose of 6 log(CFU/g) for labeled microorganisms in yogurt are recommended.[Citation38] The viability of this starter or labeled microorganisms can be affected by various factors, such as chemical composition of the raw material used, organic acids produced at the end of fermentation, initial and subsequent pH reduction during storage, fermentable sugar composition, and metabolites such as bacteriocins or hydrogen peroxide produced by lactic acid bacteria.[Citation13,Citation16,Citation37,Citation39–45] Considering possible microbial interactions during refrigerated storage revealed interesting findings. Interactions between different bacterial strains and their response to environmental factors may contribute to the extinction patterns observed. Numerous researchers have noted a reduction in the microflora involved in the fermentation of plant-based drinks during refrigeration.[Citation30,Citation32,Citation39,Citation42,Citation46–48] Understanding potential microbial behavior deepens our understanding of microbial behavior in fermented oat drinks. Based on the findings of this study, the S. thermophilus species, which is part of the classical yogurt starter culture, appeared to be the most promising. This may shed light on the observations regarding the microflora composition of commercially available plant-based yogurt alternatives.[Citation35,Citation39] Low storage temperatures can affect the survival of thermophilic bacteria such as bifidobacteria and propionic acid bacteria. Although Zhang et al.[Citation16] prove that the oat phenolic compound group could promote the viability of lactobacilli and bifidobacteria, a meta-analysis of literature data generally suggests that the viability of lactic acid bacteria and bifidobacteria was genus-dependent.[Citation39] Probably the same conclusions can be applied to propionic acid bacteria. Various types of interactions, including symbiotic relationships, between traditional yogurt bacteria and additional microorganisms including bifidobacteria and propionic acid bacteria, also play a critical role.[Citation33,Citation39,Citation49–52] Therefore, to produce a functional product with a sufficient number of live cells to achieve the desired health effects – specifically, exceeding 6 log (CFU/g) – it is crucial to carefully select the starter bacteria. Moreover, their initial population should be properly managed, considering the rate at which these microorganisms decline during the refrigeration storage of fermented oat drinks.
Change in hardness and adhesiveness during refrigerated storage of fermented oat drinks
Immediately after fermentation, the hardness and adhesiveness of the fermented oat drinks were consistent, statistically regardless of the type of starter culture used. However, these parameters evolved during refrigerated storage, depending on both the type of starter culture and the storage time (). A statistically significant decrease in hardness was only observed in samples of fermented oat drinks made without the addition of propionic acid bacteria; notably, these changes became significant only on the 28th day of storage. Meanwhile, statistically significant changes in adhesiveness were evident as early as the 7th day of refrigerated storage. Interestingly, for oat drink samples fermented with the addition of bifidobacteria, a gradual reduction in adhesiveness was observed. In contrast, other oat drink samples showed a gradual increase in this parameter.
Figure 2. Change in hardness and adhesiveness during refrigerated storage of fermented oat drinks (average values and standard deviations).
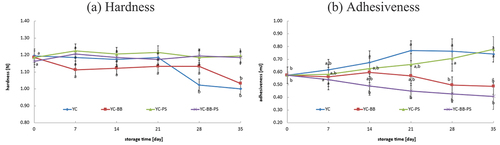
Figure 3. Change in sugar content during refrigerated storage of fermented oat drinks (a) YC, (b) YC-BB, (c) YC-PS, and (d) YC-BB-PS.
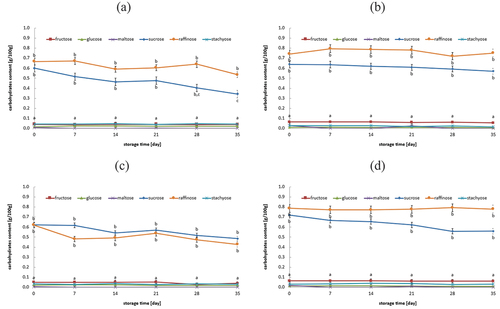
A fascinating line of exploration is the potential correlation between changes in textural properties and the observed pH dynamics. The reductions in hardness was not related to the specific pH stability during refrigerated storage. Understanding the relationships between pH value and structural changes enriches our understanding of the complexity of the fermentation process.
Changes in hardness and adhesiveness can significantly impact the sensory properties of fermented drinks, including fermented oat drinks.[Citation53,Citation54] The rate of these changes depends on various factors such as the drink’s recipe, the type of bacteria used for fermentation, storage temperature, and the length of storage time.[Citation37,Citation54–57] Generally, the changes are more pronounced at lower temperatures and over longer storage times. Investigating how components in the oat matrix affect changes in hardness and adhesiveness provides valuable insights. Oats contain fibers, proteins, and lipids that may play different roles in shaping the developing texture of the final product. Oat drinks have strong emulsifying and gel-forming properties, resulting in firm gels with good hardness and water retention.[Citation15,Citation58,Citation59] This is likely due to the presence of β-glucan, a fraction of soluble dietary fiber that readily absorbs water and contributes to strong gel formation.[Citation37] According to this research, it is important to choose the composition of microorganisms involved in the fermentation of oat drinks for the values of hardness and adhesion. Dong et al.[Citation60] showed that oat β-glucans significantly promote the growth of Lactobacillus and Bifidobacterium. Other studies have also demonstrated the ability of Bifidobacterium animalis subsp. lactis Bb-12 to ferment β-glucans.[Citation61] This unique capability of bifidobacteria to selectively ferment β-glucan may explain the observed changes in adhesiveness values. It is worth noting, however, that these changes did not influence other analyzed physical parameters, such as pH, hardness, and WHC. In the oat drink samples studied here, it appears that only the enzymatic activity of the bacteria under discussion was observed. The activity might have been so subtle that no significant changes in adhesiveness were detected in samples containing lactobacilli without the addition of bifidobacteria. However, measurable changes were evident in samples containing both lactobacilli and bifidobacteria, potentially due to the release of intracellular enzymes during the gradual decline of bacterial cell populations.
Change in water-holding capacity during refrigerated storage of fermented oat drinks
Understanding the water-holding capacity (WHC) of fermented oat drinks is crucial for elucidating the textural dynamics and stability of these products. In this study, the WHC value of fermented oat drinks is determined by the starter cultures and the storage time of fermented oat drink samples () of a food product is crucial for its texture and stability. For fermented oat drinks, maintaining optimal WHC is critical to prevent the product from becoming watery and unstable, which directly impacts consumer satisfaction. The change in WHC during refrigerated storage of fermented oat drinks is an important factor to consider in both product development and storage. The WHC of fermented oat drinks statistically significant increases during refrigerated storage. Key to the initial (first day of analysis) and final (on the 35th day of refrigeration) WHC values was the use of propionic acid bacteria, this addition determined the differentiation of samples in terms of measured WHC values.
Table 3. Change in water-holding capacity during refrigerated storage of fermented oat drinks (average values and standard deviations).
WHC refers to the ability of a gel to retain its liquid component. Exploring the changes in WHC during refrigerated storage provides valuable insights for product development. This is often the converse of syneresis, a process in which the gel network shrinks and releases liquid because it cannot effectively hold the liquid within its structure.[Citation62] In the context of fermented milk, the WHC often decreases during refrigerated storage due to the destabilization of the protein network in the drink caused by protein network breakdown. The pH of the drink also influences the WHC, with lower pH values typically leading to a lower WHC.[Citation63] Syneresis – defined as the separation of whey from the curd – increases in fermented milk during storage. Contrastingly, fermented oat drinks, which rely on a carbohydrate network instead of a protein network, experience a decrease in syneresis during storage.[Citation31,Citation37] These drinks are composed of oats and water and are fermented with lactic acid bacteria. The bacteria can break down carbohydrates in the oat drink, including starch and soluble fiber fractions like β-glucans, into simpler sugars that are then fermented to lactic acid.[Citation15,Citation55] Unlike in milk fermentation, the acidity in the oat drink does not significantly denature proteins.[Citation64] Consequently, the starch network is more stable and does not degrade during later storage, leading to lower syneresis. This mechanism is likely relevant for oat drinks where starch and soluble fiber fractions have not been enzymatically broken down during early production stages.[Citation56] If enzymatic processing is used, an increase in syneresis is observed in the fermented oat drink during refrigeration. In these studies, the oat drink was prepared using a water extract of oats without thermal treatment, and the oat-to-water ratio was significantly adjusted to produce drinks with dense consistency. Previous research suggests that an increase in the total dry matter content, including fibers, can improve water retention in the gel network and increase WHC.[Citation65,Citation66] This may explain the WHC values observed in our study and the direction of changes during cooling.[Citation37,Citation67,Citation68]
Generally, the WHC value is influenced by the microbial composition of the starter culture used, likely due to the acidifying properties of the bacteria used.[Citation69,Citation70] In the present studies, no significant changes in pH value were observed during storage, nor were there notable differences in pH across the fermented oat drink samples. This suggests that pH was not the driving factor behind the WHC changes recorded in this case. Previous research indicates that propionibacteria produce an extracellular exopolysaccharide in liquid cultures that increases viscosity.[Citation71–73] This could explain why oat-based drinks fermented with the addition of propionibacteria statistically exhibited higher WHC values compared to those made without them.
An intriguing aspect of our study is the investigation of possible correlation between changes in WHC and the observed hardness and adhesiveness changes during refrigerated storage. Understanding how hardness and adhesiveness changes affect WHC contributes to a more nuanced understanding of the fermentation process and its impact on the properties of final product. Changes in WHC have direct impact on consumer’s perception and acceptance. To counter the decrease in WHC of fermented milk during refrigerated storage, several strategies can be employed. One approach is to add stabilizers to the drink; another is to utilize a starter culture with low proteolytic activity.[Citation43,Citation74,Citation75] For fermented oat drinks specifically, texture-stabilizing additives could be introduced. This would allow for the modification and control of syneresis in the final product.[Citation37]
Change in sugar content during refrigerated storage of fermented oat drinks
The initial content of selected carbohydrates (fructose, glucose, maltose, sucrose, raffinose, and stachyose) in the analyzed oat drinks before fermentation averaged 0.10 ± 0.01, 0.00 ± 0.00, 0.09 ± 0.02, 0.87 ± 0.03, 0.92 ± 0.01, and 0.01 ± 0.00 g/100 g, respectively. The fermentation process has reduced the amount of these carbohydrates in oat drinks. There is also starch in oat drinks, which in the designation was not the subject of the analysis, but it should be assumed that during the preparation and oat fermentation of drinks, its content could be changed. Changes in the content of selected carbohydrates in oat drinks fermented with lactic acid bacteria, bifidobacteria, and Propionibacterium were statistically minimal (). Across all samples of fermented oat drinks, the levels of fructose, glucose, maltose, and stachyose remained very low and showed no statistically significant changes during 35 days of refrigerated storage. Sucrose and raffinose were the only carbohydrates found at levels above 0.1 g/100 g. A statistically significant reduction in sucrose levels was observed only in oat drinks fermented with YC starter culture during refrigerated storage (). In the remaining samples of fermented oat drinks, no changes in the content of selected carbohydrates were observed during refrigerated storage.
Carbohydrates play an important role in influencing taste, consistency, and nutritional value. They serve as the primary substrate for the fermenting microorganisms. Carbohydrates can either come from natural sources or be added to the product in forms like sweeteners, such as beetroot sugar, or flavor additives such as fruit preparations. Importantly, no additional carbon sources were introduced in the studies for the tested starter cultures.
Part et al.[Citation35] explored the content of selected sugars in commercial samples of plant-based yogurt alternatives, including fermented oat drinks, from retail stores in Estonia, Finland, and Germany. Oat drinks fermented solely by S. thermophilus and L. bulgaricus contained 0.018 g/100 mL of fructose, 2.133 g/100 mL of glucose, 1.280 g/100 mL of maltose, and 0.098 g/100 mL of sucrose. Notably, these samples predominantly contained S. thermophilus, with only traces of L. bulgaricus. Meanwhile, oat drinks fermented with a selected yogurt starter culture alongside live cultures of L. acidophilus and bifidobacteria had 0.029 g/100 mL of fructose, 0.001 g/100 mL of glucose, 0.028 g/100 mL of maltose, and 1.072 g/100 mL of sucrose. It is important to note that in the oat drink samples examined, S. thermophilus was the predominant bacteria, with only trace amounts of the declared L. acidophilus and B. lactis. Unfortunately, the authors of the cited studies did not provide information on whether the oat drinks they tested contained any added sugars. Despite this, they indicate that oat-based drink samples were most likely enzymatically treated to improve the texture by degrading the starch and releasing mono and disaccharides.[Citation64] This finding is also confirmed in other publications.[Citation4,Citation31,Citation33] Such procedures were not carried out during the preparation of oat drink samples in the present study, which may explain the dissimilarity of the results obtained.
Glucose is well-known as the fundamental carbon source for living microorganisms, and other sugars like fructose or lactose are necessary for the growth of specific lactic acid bacteria.[Citation4] In this study, the immediate postfermentation content of fructose, glucose, maltose, and stachyose was found to be minimal, suggesting that these carbohydrates did not serve as significant carbon sources for the starter cultures under investigation. The steady levels of raffinose further support the idea that this particular oligosaccharide was also not used as a carbon source by the tested starter cultures. Interestingly, changes in sucrose levels were observed solely in oat drinks fermented with the YC starter culture. In other samples, no such changes in sucrose levels were noted, implying that sucrose only served as a carbon source for bacteria when yogurt bacteria were not accompanied by other starter cultures. Regrettably, there is a dearth of literature data concerning fluctuations in the levels of the selected carbohydrates (fructose, glucose, maltose, sucrose, raffinose, and stachyose) in fermented oat drink samples, either during fermentation or subsequent storage. However, Bernat et al.[Citation55] checked the possibility of oat drink fermentation and assessed the quality of the resulting products. One aspect of their analysis included examining the concentrations of select carbohydrates. The highest contents of fructose, mannitol, and glucose were recorded in their samples. Following 21 days of refrigerated storage, the content of these sugars decreased.
The discrepancies between the findings of our study and the existing literature may result from the unique formulation of the oat drink samples used for fermentation in each case, as well as differences in the characteristics of the starter cultures used for the fermentation process. Vénica et al.[Citation76] checked the impact of carbohydrate composition on the metabolic activity of starter cultures in yogurt. They analyzed four types of yogurt: traditional and lactose-free, each with and without added sucrose. In lactose-free yogurts supplemented with sucrose, there were no discernible changes in the amount of lactose, glucose, or galactose. This suggests that sucrose functioned as the primary carbon source. Likewise, traditional yogurt sweetened with sucrose showed reduced lactose consumption compared to its unsweetened counterpart. According to Anbukkarasi et al.,[Citation77] most yogurt starter cultures lack the capability to ferment galactose. Zhang et al.[Citation78] conducted research on the lactose and galactose metabolism capabilities of Lactiplantibacillus plantarum. This study is noteworthy because common yogurt starter bacteria like S. thermophilus and L. delbrueckii subsp. bulgaricus can metabolize only the glucose component of lactose. As a result, substantial amounts of residual galactose and nonhydrolysed lactose remain in the yogurt. Experiments involving these bacteria and L. plantarum, grown on lactose-based substrates or in skimmed milk, revealed that lactose was entirely hydrolyzed in samples with L. plantarum, leaving negligible galactose content. Independent culturing of the starter bacteria showed that S. thermophilus strains were able to metabolize between 90% and 95% of lactose, while L. delbrueckii subsp. bulgaricus managed only 77% to 79%. When all these bacteria were combined and incubated together, lactose content was substantially reduced. However, galactose content likely increased, which could be attributed to the type of milk used in the experiment.
Wu et al.[Citation79] conducted research in which they checked changes in the content of carbohydrates during the cow’s and soy drink fermentation process by bacteria from the types of Propionibacterium and Bifidobacterium. Quoted researchers also observed that the sucrose, raffinose, and stachyose contained in the soy drink remained at the same level throughout the entire fermentation process. The same relationship was also observed in these studies conducted based on soy drink samples.
Conclusion
In this study, we investigated the effects of fermentation on the quality parameters of oat drinks. Our findings indicate that introducing bifidobacteria and/or propionic acid bacteria to oat drink cultures expedites the acidification process, resulting in a drink with a lower pH. The choice of starter culture can have a significant impact on the final pH of the fermented product. Adding bifidobacteria, either alone or in conjunction with propionic acid bacteria, notably speeds up the acidification of oat drink samples. The oat drink proved to be a favorable substrate for fermentation by lactic acid bacteria, irrespective of the presence of other bacteria like bifidobacteria or propionic acid bacteria. Furthermore, the fermentation process remained stable, and the pH was not influenced by storage conditions. The fermentation process results in a significant reduction in the carbohydrate content in oat drinks, with the most significant reduction being in the amount of sucrose. The type of bacteria used can affect the amount of carbohydrates reduced during fermentation. Adding propionic acid bacteria to the starter culture for fermented oat drinks can improve the WHC of the drink and extend its shelf life. Interestingly, the fermentation process had little impact on the texture of the drink. The hardness of the fermented oat drinks decreased during refrigeration, but this was only observed in samples without propionic acid bacteria. In the fermented oat drink samples, the initial population of lactic acid bacteria, bifidobacteria, and propionic acid bacteria was notably high, exceeding 7 log(CFU/g). This confirms the success of the fermentation process, indicating that the starter cultures thrived and proliferated in the oat drink environment. Upon refrigeration, there was a significant decline in the population of live bacterial cells across all types studied. Bifidobacteria and propionic acid bacteria were found to be more susceptible to refrigeration compared to lactic acid bacteria. Therefore, it is crucial to select a starter culture that is compatible with the intended food product and its storage conditions. WHC of fermented oat drinks is determined by both the starter cultures used and the storage time. By adding propionic acid bacteria to the oat drink culture, the WHC of the drink is significantly increased. Conversely, the adhesiveness of the fermented oat drinks increased during refrigerated storage, but adding bifidobacteria to a fermented food product can help reduce the adhesiveness of the product. The changes in carbohydrates content during refrigerated storage were negligible.
In summary, our study suggests that incorporating bifidobacteria and/or propionic acid bacteria to oat drink cultures can enhance both the quality and shelf life of the final product. It is important to note, however, that this study was specific to a certain type of oat milk and starter cultures. The study explored a novel approach to enhancing oat drink quality and shelf life using fermentation with specific bacteria strains. The results may not be generalizable to other types of oat milk or starter cultures. Additionally, the study was conducted over a short period, so the long-term effects of fermentation on oat drink quality remain unexplored. The study focused on a specific type of oat milk and starter cultures, which may have limited the applicability of the results to other oat milk formulations or starter culture combinations. This study also did not assess the sensory properties of the fermented oat drinks, such as taste, smell, and appearance. Further research is needed to confirm these preliminary findings, assess sensory qualities, and explore the potential health benefits of fermented oat drinks.
Author Contributions
Conceptualization, M.Z., D.Z., I.Ś., and M.K.; methodology, M.Z., D.Z., I.Ś., and M.K.; investigation, M.Z., I.Ś., and M.K.; data curation, M.Z., I.Ś., and M.K.; writing – M.Z., D.Z., I.Ś., and M.K.; writing – review and editing, M.Z., and D.Z.; project administration, M.Z. All authors have read and agreed to the published version of the manuscript.
Institutional Review Board statement
This study did not involve humans or animals.
Informed consent statement
This study did not involve humans.
Acknowledgments
The authors gratefully acknowledge the Institute of Food Sciences of Warsaw University of Life Sciences WULS–SGGW for supporting and providing necessary infrastructure and research stuff.
Disclosure statement
No potential conflict of interest was reported by the author(s).
Data availability statement
The data that support the findings of this study are available from the corresponding author (M.Z.) upon reasonable request.
Additional information
Funding
References
- Motarjemi, Y. Impact of Small Scale Fermentation Technology on Food Safety in Developing Countries. Int. J. Food Microbiol. 2002, 75(3), 213–229. DOI: 10.1016/S0168-1605(01)00709-7.
- Roberfroid, M. B. What is Beneficial for Health? The Concept of Functional Food. Food. Chem. Toxicol. 1999, 37(9), 1039–1041. DOI: 10.1016/S0278-6915(99)00080-0.
- Salminen, S.; Ouwehand, A.; Benno, Y.; Lee, Y. K. Probiotics: How Should They Be Defined? Trends Food Sci. Technol. 1999, 10(3), 107–110. DOI: 10.1016/S0924-2244(99)00027-8.
- Ziarno, M.; Cichońska, P. Lactic Acid Bacteria-Fermentable Cereal- and Pseudocereal-Based Beverages. Microorganisms. 2021, 9(12), 2532. DOI: 10.3390/microorganisms9122532.
- Cichońska, P.; Ziarno, M. Legumes and Legume-Based Beverages Fermented with Lactic Acid Bacteria as a Potential Carrier of Probiotics and Prebiotics. Microorganisms. 2022, 10(1), 91. DOI: 10.3390/microorganisms10010091.
- Cichońska, P.; Kowalska, E.; Ziarno, M. Fermentation of Plant-Based Beverages Using Lactic Acid Bacteria – a Review. Postępy Tech. Przetwórstwa Spoż. 2022, 86–97.
- Cui, L.; Jia, Q.; Zhao, J.; Hou, D.; Zhou, S. A Comprehensive Review on Oat Milk: From Oat Nutrients and Phytochemicals to Its Processing Technologies, Product Features, and Potential Applications. Food Funct. 2023, 14(13), 5858–5869. DOI: 10.1039/D3FO00893B.
- Fehér, A.; Gazdecki, M.; Véha, M.; Szakály, M.; Szakály, Z. A Comprehensive Review of the Benefits of and the Barriers to the Switch to a Plant-Based Diet. Sustainability. 2020, 12(10), 4136. DOI: 10.3390/su12104136.
- Ziarno, M.; Zaręba, D. Substancje dodatkowe stosowane w serowarstwie. Przem. Spoż. 2007, T. 61(10), 34–38.
- Parrish, C. R.; Over, M.-O. Cow’s Milk: The Rise of Plant-Based Dairy Alternatives. Pract. Gastroenterol. 2018, 7, 20–27.
- Ibrahim, M. S.; Ahmad, A.; Sohail, A.; Asad, M. J. Nutritional and Functional Characterization of Different Oat (Avena Sativa L.) Cultivars. Int. J. Food. Prop. 2020, 23(1), 1373–1385. DOI: 10.1080/10942912.2020.1806297.
- Sangwan, S.; Singh, R.; Tomar, S. Nutritional and Functional Properties of Oats: An Update. 2014.
- Savas, B. S.; Akan, E. Oat Bran Fortified Raspberry Probiotic Dairy Drinks: Physicochemical, Textural, Microbiologic Properties, in vitro Bioaccessibility of Antioxidants and Polyphenols. Food Biosci. 2021, 43, 101223. DOI: 10.1016/j.fbio.2021.101223.
- Ullah, H.; Hussain, Y.; Santarcangelo, C.; Baldi, A.; Di Minno, A.; Khan, H.; Xiao, J.; Daglia, M. Natural Polyphenols for the Preservation of Meat and Dairy Products. Molecules. 2022, 27(6), 1906. DOI: 10.3390/molecules27061906.
- Butt, M. S.; Tahir-Nadeem, M.; Khan, M. K. I.; Shabir, R.; Butt, M. S. Oat: Unique Among the Cereals. Eur. J. Nutr. 2008, 47(2), 68–79. DOI: 10.1007/s00394-008-0698-7.
- Zhang, Y.; Li, Y.; Ren, X.; Zhang, X.; Wu, Z.; Liu, L. The Positive Correlation of Antioxidant Activity and Prebiotic Effect About Oat Phenolic Compounds. Food Chem. 2023, 402, 134231. DOI: 10.1016/j.foodchem.2022.134231.
- Ziarno, M.; Zaręba, D. The Effect of the Addition of Microbial Transglutaminase Before the Fermentation Process on the Quality Characteristics of Three Types of Yogurt. Food Sci. Biotechnol. 2020, 29(1), 109–119. DOI: 10.1007/s10068-019-00640-6.
- Bezerra, M. F.; Souza, D. F. S.; Correia, R. T. P. Acidification Kinetics, Physicochemical Properties and Sensory Attributes of Yoghurts Prepared from Mixtures of Goat and Buffalo Milks. Int. J Dairy Technol. 2012, 65(3), 437–443. DOI: 10.1111/j.1471-0307.2012.00845.x.
- Varghese, K.; Mishra, S.; N, H. Modelling of Acidification Kinetics and Textural Properties in Dahi (Indian Yogurt) Made from Buffalo Milk Using Response Surface Methodology. Int. J Dairy Technol. 2008, 61(3), 284–289. DOI: 10.1111/j.1471-0307.2008.00411.x.
- International Organization for Standardization. ISO 6887-5:2020. Microbiology of the Food Chain—Preparation of Test Samples, Initial Suspension and Decimal Dilutions for Microbiological Examination—Part 5: Specific Rules for the Preparation of Milk and Milk Products; 2020.
- Ziarno, M.; Derewiaka, D.; Dytrych, M.; Stawińska, E.; Zaręba, D. Effects of Fat Content on Selected Qualitative Parameters of a Fermented Coconut “Milk” Beverage. J. Food Nutr. Res. 2020, 59(2), 155–162.
- Helland, M. H.; Wicklund, T.; Narvhus, J. A. Growth and Metabolism of Selected Strains of Probiotic Bacteria, in Maize Porridge with Added Malted Barley. Int. J. Food Microbiol. 2004, 91(3), 305–313. DOI: 10.1016/j.ijfoodmicro.2003.07.007.
- Salmerón, I.; Thomas, K.; Pandiella, S. S. Effect of Substrate Composition and Inoculum on the Fermentation Kinetics and Flavour Compound Profiles of Potentially Non-Dairy Probiotic Formulations. LWT - Food Sci. Technol. 2014, 55(1), 240–247. DOI: 10.1016/j.lwt.2013.07.008.
- Luana, N.; Rossana, C.; Curiel, J. A.; Kaisa, P.; Marco, G.; Rizzello, C. G. Manufacture and Characterization of a Yogurt-Like Beverage Made with Oat Flakes Fermented by Selected Lactic Acid Bacteria. Int. J. Food Microbiol. 2014, 185, 17–26. DOI: 10.1016/j.ijfoodmicro.2014.05.004.
- Coda, R.; Lanera, A.; Trani, A.; Gobbetti, M.; Di Cagno, R. Yogurt-Like Beverages Made of a Mixture of Cereals, Soy and Grape Must: Microbiology, Texture, Nutritional and Sensory Properties. Int. J. Food Microbiol. 2012, 155(3), 120–127. DOI: 10.1016/j.ijfoodmicro.2012.01.016.
- Lazaridou, A.; Serafeimidou, A.; Biliaderis, C. G.; Moschakis, T.; Tzanetakis, N. Structure Development and Acidification Kinetics in Fermented Milk Containing Oat β-Glucan, a Yogurt Culture and a Probiotic Strain. Food Hydrocoll. 2014, 39, 204–214. DOI: 10.1016/j.foodhyd.2014.01.015.
- Mårtensson, O.; Andersson, C.; Andersson, K.; Öste, R.; Holst, O. Formulation of an Oat-Based Fermented Product and Its Comparison with Yoghurt. J. Sci. Food Agric. 2001, 81(14), 1314–1321. DOI: 10.1002/jsfa.947.
- Angelov, A.; Gotcheva, V.; Kuncheva, R.; Hristozova, T. Development of a New Oat-Based Probiotic Drink. Int. J. Food Microbiol. 2006, 112(1), 75–80. DOI: 10.1016/j.ijfoodmicro.2006.05.015.
- Gupta, S.; Cox, S.; Abu-Ghannam, N. Process Optimization for the Development of a Functional Beverage Based on Lactic Acid Fermentation of Oats. Biochem. Eng. J. 2010, 52(2), 199–204. DOI: 10.1016/j.bej.2010.08.008.
- Russo, P.; de Chiara, M. L. V.; Capozzi, V.; Arena, M. P.; Amodio, M. L.; Rascón, A.; Dueñas, M. T.; López, P.; Spano, G. Lactobacillus Plantarum Strains for Multifunctional Oat-Based Foods. LWT - Food Sci. Technol. 2016, 68, 288–294. DOI: 10.1016/j.lwt.2015.12.040.
- Walsh, H.; Ross, J.; Hendricks, G.; Guo, M. Physico-Chemical Properties, Probiotic Survivability, Microstructure, and Acceptability of a Yogurt-Like Symbiotic Oats-Based Product Using Pre-Polymerized Whey Protein as a Gelation Agent. J. Food Sci. 2010, 75(5), M327–M337. DOI: 10.1111/j.1750-3841.2010.01637.x.
- Raikos, V.; Juskaite, L.; Vas, F.; Hayes, H. E. Physicochemical Properties, Texture, and Probiotic Survivability of Oat-Based Yogurt Using Aquafaba as a Gelling Agent. Food Sci. Nutr. 2020, 8(12), 6426–6432. DOI: 10.1002/fsn3.1932.
- Kütt, M.-L.; Orgusaar, K.; Stulova, I.; Priidik, R.; Pismennõi, D.; Vaikma, H.; Kallastu, A.; Zhogoleva, A.; Morell, I.; Kriščiunaite, T. Starter Culture Growth Dynamics and Sensory Properties of Fermented Oat Drink. Heliyon. 2023, 9(5), e15627. DOI: 10.1016/j.heliyon.2023.e15627.
- Akan, E.; Karakaya, S.; Eda Eker Özkacar, M.; Kinik, Ö. Effect of Food Matrix and Fermentation on Angiotensin-Converting Enzyme Inhibitory Activity and β-Glucan Release After in vitro Digestion in Oat-Based Products. Food Res. Int. Ott. Ont. 2023, 165, 112508. DOI: 10.1016/j.foodres.2023.112508.
- Part, N.; Kazantseva, J.; Rosenvald, S.; Kallastu, A.; Vaikma, H.; Kriščiunaite, T.; Pismennõi, D.; Viiard, E. M. Chemical, and Sensorial Characterisation of Commercially Available Plant-Based Yoghurt Alternatives. Fut. Foods. 2023, 7, 100212. DOI: 10.1016/j.fufo.2022.100212.
- Shah, N. P.; Lankaputhra, W. E. V.; Britz, M. L.; Kyle, W. S. A. Survival of Lactobacillus Acidophilus and Bifidobacterium Bifidum in Commercial Yoghurt During Refrigerated Storage. Int. Dairy. J. 1995, 5(5), 515–521. DOI: 10.1016/0958-6946(95)00028-2.
- Dhakal, D.; Younas, T.; Bhusal, R. P.; Devkota, L.; Henry, C. J.; Dhital, S. Design Rules of Plant-Based Yoghurt-Mimic: Formulation, Functionality, Sensory Profile and Nutritional Value. Food Hydrocoll. 2023, 142, 108786. DOI: 10.1016/j.foodhyd.2023.108786.
- Codex Alimentarius. Milk and Milk Products, 2nd ed.; United Nations, Rome: World Health Organization, Food and Agriculture Organization, 2010.
- Soto, L. P.; Sirini, N. E.; Frizzo, L. S.; Zbrun, M. V.; Zimmermann, J. A.; Ruiz, M. J.; Rosmini, M. R.; Sequeira, G. J.; Miotti, C.; Signorini, M. L. Lactic Acid Bacteria Viability in Different Refrigerated Food Matrices: A Systematic Review and Meta‑Analysis. Crit. Rev. Food Sci. Nutr. 2022, 1–29. DOI: 10.1080/10408398.2022.2099807.
- Angelov, A.; Gotcheva, V.; Hristozova, T.; Gargova, S. Application of Pure and Mixed Probiotic Lactic Acid Bacteria and Yeast Cultures for Oat Fermentation. J. Sci. Food Agric. 2005, 85(12), 2134–2141. DOI: 10.1002/jsfa.2223.
- Shah, N. P. Probiotic Bacteria: Selective Enumeration and Survival in Dairy Foods. J. Dairy. Sci. 2000, 83(4), 894–907. DOI: 10.3168/jds.S0022-0302(00)74953-8.
- Charalampopoulos, D.; Pandiella, S. S.; Webb, C. Growth Studies of Potentially Probiotic Lactic Acid Bacteria in Cereal‐Based Substrates. J. Appl. Microbiol. 2002, 92(5), 851–859. DOI: 10.1046/j.1365-2672.2002.01592.x.
- Li, J.; Guo, M. Effects of Polymerized Whey Proteins on Consistency and Water-Holding Properties of Goat’s Milk Yogurt. J. Food Sci. 2006, 71(1), C34–C38. DOI: 10.1111/j.1365-2621.2006.tb12385.x.
- Talwalkar, A.; Kailasapathy, K. The Role of Oxygen in the Viability of Probiotic Bacteria with Reference to L. Acidophilus and Bifidobacterium Spp. Curr. Issues Intest. Microbiol. 2004, 5(1), 1–8.
- Talwalkar, A.; Miller, C. W.; Kailasapathy, K.; Nguyen, M. H. Effect of Packaging Materials and Dissolved Oxygen on the Survival of Probiotic Bacteria in Yoghurt. Int. J. Food Sci. Technol. 2004, 39(6), 605–611. DOI: 10.1111/j.1365-2621.2004.00820.x.
- Ziarno, M.; Zaręba, D.; Dryzek, W.; Hassaliu, R.; Florowski, T. Effect of the Addition of Soy Beverage and Propionic Bacteria on Selected Quality Characteristics of Cow’s Milk Yoghurt Products. Appl. Sci. 2022, 12(24), 12603. DOI: 10.3390/app122412603.
- Shori, A. B.; Baba, A. S. Viability of Lactic Acid Bacteria and Sensory Evaluation in Cinnamomum Verum and Allium Sativum-Bio-Yogurts Made from Camel and Cow Milk. J. Assoc. Arab Univ. Basic Appl. Sci. 2012, 11(1), 50–55. DOI: 10.1016/j.jaubas.2011.11.001.
- Cichońska, P.; Ziębicka, A.; Ziarno, M. Properties of Rice-Based Beverages Fermented with Lactic Acid Bacteria and Propionibacterium. Molecules. 2022, 27(8), 2558. DOI: 10.3390/molecules27082558.
- Tarnaud, F.; Gaucher, F.; Do Carmo, F. L. R.; Illikoud, N.; Jardin, J.; Briard-Bion, V.; Guyomarc’h, F.; Gagnaire, V.; Jan, G. Differential Adaptation of Propionibacterium Freudenreichii CIRM-BIA129 to Cow’s Milk versus Soymilk Environments Modulates Its Stress Tolerance and Proteome. Front. Microbiol. 2020, 11, 11. DOI: 10.3389/fmicb.2020.549027.
- Gagnaire, V.; Thierry, A.; Léonil, J. Propionibacteria and Facultatively Heterofermentative Lactobacilli Weakly Contribute to Secondary Proteolysis of Emmental Cheese. Le Lait. 2001, 81(3), 339–353. DOI: 10.1051/lait:2001136.
- Weber, M. H. W.; Marahiel, M. A. Bacterial Cold Shock Responses. Sci. Prog. 2003, 86(1–2), 9–75. DOI: 10.3184/003685003783238707.
- Loux, V.; Mariadassou, M.; Almeida, S.; Chiapello, H.; Hammani, A.; Buratti, J.; Gendrault, A.; Barbe, V.; Aury, J.-M.; Deutsch, S.-M., et al. Mutations and Genomic Islands Can Explain the Strain Dependency of Sugar Utilization in 21 Strains of Propionibacterium Freudenreichii. BMC Genom. 2015, 16(1), 296. DOI: 10.1186/s12864-015-1467-7.
- Mudgil, D.; Barak, S.; Khatkar, B. S. Texture Profile Analysis of Yogurt as Influenced by Partially Hydrolyzed Guar Gum and Process Variables. J. Food Sci. Technol. 2017, 54(12), 3810–3817. DOI: 10.1007/s13197-017-2779-1.
- Demir, H.; Aydemir, L. Y.; Özel, M. Ş.; Koca, E.; Şimşek Aslanoğlu, M. Application of Plant-Based Proteins for Fortification of Oat Yogurt Storage Stability and Bioactivity. J. Food Sci. 2023, 88(10), 4079–4096. DOI: 10.1111/1750-3841.16729.
- Bernat, N.; Cháfer, M.; González-Martínez, C.; Rodríguez-García, J.; Chiralt, A. Optimisation of Oat Milk Formulation to Obtain Fermented Derivatives by Using Probiotic Lactobacillus Reuteri Microorganisms. Food Sci. Technol. Int. 2015, 21(2), 145–157. DOI: 10.1177/1082013213518936.
- Deswal, A.; Deora, N. S.; Mishra, H. N. Optimization of Enzymatic Production Process of Oat Milk Using Response Surface Methodology. Food Bioprocess. Technol. 2014, 7(2), 610–618. DOI: 10.1007/s11947-013-1144-2.
- Yang, Z.; Xie, C.; Bao, Y.; Liu, F.; Wang, H.; Wang, Y. Oat: Current State and Challenges in Plant-Based Food Applications. Trends Food Sci. Technol. 2023, 134, 56–71. DOI: 10.1016/j.tifs.2023.02.017.
- Demir, H.; Simsek, M.; Yıldırım, G. Effect of Oat Milk Pasteurization Type on the Characteristics of Yogurt. LWT. 2021, 135, 110271. DOI: 10.1016/j.lwt.2020.110271.
- Sethi, S.; Tyagi, S. K.; Anurag, R. K. Plant-Based Milk Alternatives an Emerging Segment of Functional Beverages: A Review. J. Food Sci. Technol. 2016, 53(9), 3408–3423. DOI: 10.1007/s13197-016-2328-3.
- Dong, J.; Yang, M.; Zhu, Y.; Shen, R.; Zhang, K. Comparative Study of Thermal Processing on the Physicochemical Properties and Prebiotic Effects of the Oat β-Glucan by in vitro Human Fecal Microbiota Fermentation. Food. Res. Int. 2020, 138, 109818. DOI: 10.1016/j.foodres.2020.109818.
- Moghadam, B. E.; Hasebi, Z.; Seyfzadeh, S.; Talebi, V.; Keivaninahr, F.; Fouladi, M.; Mokarram, R. R. Barley β-Glucan for Conjugated Linoleic Acid (CLA) Production by Bifidobacterium Animalis Subsp. Lactis: Fatty Acid Variation and Bacterial Viability Study. Bioact. Carbohydr. Diet. Fibre. 2022, 28, 100321. DOI: 10.1016/j.bcdf.2022.100321.
- Aryana, K. J.; Olson, D. W. A 100-Year Review: Yogurt and Other Cultured Dairy Products. J. Dairy. Sci. 2017, 100(12), 9987–10013. DOI: 10.3168/jds.2017-12981.
- Gumus, C. E.; Gharibzahedi, S. M. T. Yogurts Supplemented with Lipid Emulsions Rich in Omega-3 Fatty Acids: New Insights into the Fortification, Microencapsulation, Quality Properties, and Health-Promoting Effects. Trends Food Sci. Technol. 2021, 110, 267–279. DOI: 10.1016/j.tifs.2021.02.016.
- Patra, T.; Axel, C.; Rinnan, Å.; Olsen, K. The Physicochemical Stability of Oat-Based Drinks. J. Cereal Sci. 2022, 104, 103422. DOI: 10.1016/j.jcs.2022.103422.
- McCann, T. H.; Fabre, F.; Day, L. M. Rheology and Storage Stability of Low-Fat Yoghurt Structured by Carrot Cell Wall Particles. Food. Res. Int. 2011, 44(4), 884–892. DOI: 10.1016/j.foodres.2011.01.045.
- Rinaldoni, A. N.; Campderrós, M. E.; Pérez Padilla, A. Physico-Chemical and Sensory Properties of Yogurt from Ultrafiltreted Soy Milk Concentrate Added with Inulin. LWT - Food Sci. Technol. 2012, 45(2), 142–147. DOI: 10.1016/j.lwt.2011.09.009.
- Pang, Z.; Xu, R.; Luo, T.; Che, X.; Bansal, N.; Liu, X. Physiochemical Properties of Modified Starch Under Yogurt Manufacturing Conditions and Its Relation to the Properties of Yogurt. J. Food Eng. 2019, 245, 11–17. DOI: 10.1016/j.jfoodeng.2018.10.003.
- Lobato-Calleros, C.; Ramírez-Santiago, C.; Vernon-Carter, E. J.; Alvarez-Ramirez, J. Impact of Native and Chemically Modified Starches Addition as Fat Replacers in the Viscoelasticity of Reduced-Fat Stirred Yogurt. J. Food Eng. 2014, 131, 110–115. DOI: 10.1016/j.jfoodeng.2014.01.019.
- Domagała, J.; Wszołek, M. Effect of Concentration Method and Starter Culture Type on the Texture and Susceptibility to Syneresis of Yoghurt and Bio-Yoghurts Made of Goat’s Milk | Polish Society of Food Technologists. Żywność Nauk Technol. Jakość. 2008, 6: 118–126.
- Torre, L. L.; Tamime, A. Y.; Muir, D. D. Rheology and Sensory Profiling of Set-Type Fermented Milks Made with Different Commercial Probiotic and Yoghurt Starter Cultures. Int. J Dairy Technol. 2003, 56(3), 163–170. DOI: 10.1046/j.1471-0307.2003.00098.x.
- Skogen, L. O.; Reinbold, G. W.; Vedamuthu, E. R. CAPSULATION of PROPIONIBACTERIUM1. J. Food Prot. 1974, 37(6), 314–321. DOI: 10.4315/0022-2747-37.6.314.
- Ekinci, F. Y.; Barefoot, S. F. Fed-Batch Enhancement of Jenseniin G, a Bacteriocin Produced by Propionibacterium Thoenii (Jensenii) P126. Food Microbiol. 2006, 23(4), 325–330. DOI: 10.1016/j.fm.2005.05.012.
- Ekinci, F. Y.; Gurel, M. Effect of Using Propionic Acid Bacteria as an Adjunct Culture in Yogurt Production. J. Dairy. Sci. 2008, 91(3), 892–899. DOI: 10.3168/jds.2007-0244.
- Ares, G.; Gonçalvez, D.; Pérez, C.; Reolón, G.; Segura, N.; Lema, P.; Gámbaro, A. Influence of Gelatin and Starch on the Instrumental and Sensory Texture of Stirred Yogurt. Int. J Dairy Technol. 2007, 60(4), 263–269. DOI: 10.1111/j.1471-0307.2007.00346.x.
- Considine, T.; Noisuwan, A.; Hemar, Y.; Wilkinson, B.; Bronlund, J.; Kasapis, S. Rheological Investigations of the Interactions Between Starch and Milk Proteins in Model Dairy Systems: A Review. Food Hydrocoll. 2011, 25(8), 2008–2017. DOI: 10.1016/j.foodhyd.2010.09.023.
- Vénica, C. I.; Wolf, I. V.; Suárez, V. B.; Bergamini, C. V.; Perotti, M. C. Effect of the Carbohydrates Composition on Physicochemical Parameters and Metabolic Activity of Starter Culture in Yogurts. LWT. 2018, 94, 163–171. DOI: 10.1016/j.lwt.2018.04.034.
- Anbukkarasi, K.; UmaMaheswari, T.; Hemalatha, T.; Nanda, D. K.; Singh, P.; Singh, R. Preparation of Low Galactose Yogurt Using Cultures of Gal+streptococcus Thermophilus in Combination with Lactobacillus Delbrueckii Ssp. Bulgaricus. J. Food Sci. Technol. 2014, 51(9), 2183–2189. DOI: 10.1007/s13197-014-1262-5.
- Zhang, S. S.; Xu, Z. S.; Qin, L. H.; Kong, J. Low-Sugar Yogurt Making by the Co-Cultivation of Lactobacillus Plantarum WCFS1 with Yogurt Starter Cultures. J. Dairy. Sci. 2020, 103(4), 3045–3054. DOI: 10.3168/jds.2019-17347.
- Wu, Q. Q.; You, H. J.; Ahn, H. J.; Kwon, B.; Ji, G. E. Changes in Growth and Survival of Bifidobacterium by Coculture with Propionibacterium in Soy Milk, Cow’s Milk, and Modified MRS Medium. Int. J. Food Microbiol. 2012, 157(1), 65–72. DOI: 10.1016/j.ijfoodmicro.2012.04.013.