ABSTRACT
Staphylococcus aureus is a gram-positive, coccus-shaped facultative anaerobe and a member of the Staphylococcaceae family. In recent years, alimentary toxicosis caused by S. aureus is a very serious problem worldwide, which constitutes a great threat to public health. In this review, we tried to summarize the conventional methods and newly developed rapid detection techniques of S. aureus (traditional detection method, biochemical detection, immunology method, molecular biology, and biosensor method) for their principles, advantages, disadvantages, and applications. Furthermore, the future perspectives of S. aureus detection methods were forecasted at last.
Introduction
Staphylococcus aureus is facultative anaerobic gram-positive cocci which occur in the form of grape-like microscopic clusters. S. aureus is nonmotile, non-spore forming, catalase and coagulase positive. It was discovered in the 1880s.[Citation1] Since then, it was proved to be a potential pathogenic gram-positive bacterium that has a strong resistance. Numerous diseases in animals and humans can be attributed to S. aureus. It is responsible for the majority of cases of alimentary toxicosis, which is prevalent worldwide.[Citation2] Recently, S. aureus has been the centre of attention due to the increased incidence of alimentary toxicosis.[Citation3] According to the US Center for Disease Control, alimentary toxicosis caused by S. aureus ranked in the second place, where in the USA and in Canada were 33% and 45%, respectively.[Citation4] In China, alimentary toxicosis is prevalent every year, even though the important issue in the world.[Citation5] Therefore, food safety remains one of the most important global health issues and foodborne diseases caused by microbes are a widespread public health problem. Hence, in order to reduce foodborne disease occurrence, development of a rapid, sensitive, cost-effective, and easy-operating method for S. aureus detection is of the utmost importance.
At present, the detection methods of S. aureus are traditional biochemical identification methods and rapid detection methods.[Citation6] According to the main principle, these rapid detection methods can be classified into three categories: biochemical detection, immunological detection, and molecular biological detection. The aim of this paper is to review such rapid methods of detection and identification and to discuss some of the more recent and novel detection methods of S. aureus.
Conventional methods
The conventional detection methods of S. aureus are the three methods in the national standard GB4789.10-2010 of the People's Republic of China: S. aureus qualitative test, S. aureus Baird-Parker (B-P) plate count, and S. aureus most probable number count. The methods for the detection of S. aureus mainly involve the following basic steps: pre-enrichment, selective enrichment, selective plating, and biochemical screening and serological confirmation. These methods, generally regarded as gold standard, would take about three to seven days to obtain a confirmed result.[Citation7] These methods are sensitive, inexpensive, and can give both qualitative and quantitative information on the number and the nature of micro-organisms present in the food sample.[Citation8] However, it can no longer meet the needs of the rapid detection of pathogens in food, because of the complicated operation and time consumption.[Citation9]
Rapid detection methods
Biochemical detection
Biochemical detection is based on the biochemical reaction in the cell, according to microbial metabolism of enzymes combining with the corresponding colour substrate show a specific colour to distinguish the target bacteria. Compared with the traditional culture detection, biochemical detection can be more rapid and efficient.
Chromogenic medium
Chromogenic medium is a kind of rapid detection method, which is the new type of culture medium used to determine and identify bacteria with the enzyme and the corresponding colour substrate reaction. Currently, the optimum selective media for isolation of S. aureus are: SaSelect (Bio-Rad, Hercules, CA, USA), CHROMagar S. aureus (CHROMagar Microbiology, Paris, France), S. aureus ID (bioMerieux, France), mannitol salt agar (MSA), Baird-Parker agar (BPA), B-P supplemented with rabbit plasma fibrinogen (BPA+RPF), and Petrifilm Staph Express count system (Petrifilm). While B-P agar medium is currently specified for isolation of S. aureus in the united states food and drug administration and standard methods for the examination of dairy products.[Citation10,Citation11]
Petrifilm method
Hirvonen et al. demonstrated the sensitivity of some media for detecting S. aureus were high (100.0%) after 24 h of incubation.[Citation12] However, their specificities varied at 93.3% (95% confidence interval CI, 86.0% to 100.0%) (CHROMagar S. aureus), 97.8% (95% CI, 93.5% to 100.0%) (S. aureus ID), and 100.0% (SaSelect). While other researchers found that sensitivity of BPA+RPF, CSA, Petrifilm, MSA, and BPA were 100%, 100%, 100%, 90.5%, and 90.5%, respectively. In addition, the specificity of BPA+RPF, CSA, MSA, BPA, and Petrifilm were 100%, 100%, 84.6%, 75.0%, and 67.3%, respectively.[Citation13] Schoeller et al. compared the B-P agar with 3M (TM) Petrifilm (TM) rapid S. aureus count plate methods (PFRSA) for detection and enumeration of S. aureus. They discovered that the PFRSA method was suitable alternative to B-P for detecting and enumerating S. aureus.[Citation14]
Immunological methods
Immunological detection methods are the use of antibodies with the corresponding antigen-specific binding methods for the realization of the theoretical basis of samples tested. Currently, immunological methods are used to detect S. aureus, including immuno-labelling methods, enzyme-linked immunosorbent assay, immunofluorescence assay, immune agar diffusion method, and immunomagnetic separation (IMS) method. These methods consist of antigen-antibody reaction with a toxin produced by S. aureus, which can effectively avoid the interference of impurities in the complex sample, simplifying the purification steps before processing, simple operation, high sensitivity and specificity, and other characteristics.
Immuno-labelling methods
Immuno-labelling methods are the combination between antigen-antibody reactions and labelling techniques. In essence, it is a combined method for detection of antigen or antibody. The tags, such as fluorescein, enzymes, radioisotopes, biotin-avidin, chemiluminescent agents, and colloidal labeled antibody, are treated as tracer with the aid of fluorescence microscopy, ELISA detector, radiation meter, electron microscope, and other sophisticated chemiluminescence immunoassay analyser instrument, which improves its sensitivity and detection speed. According to the different tags, it can be divided into immune fluorescence, radio immunity assay, immunoassay, and chemiluminescence immunoassay. These methods not only detect antigen or antibody qualitatively and quantitatively, but also with high sensitivity and specificity, fast response, wide range of applications, and easy operation.
Enzyme-linked immunosorbent assay
Enzyme-linked immunosorbent assay (ELISA) is the most commonly used method in the diagnosis of immune diseases, and widely used in the diagnosis of infectious diseases, parasitic diseases, and non-infectious diseases caused by many pathogenic micro-organisms. The principle is to combine the enzyme molecules with antibodies (antigens) to form an enzyme labelled molecule, which has both the immune activity and the activity of the enzyme. The usual steps are as follows: enzyme-labelled molecules and the corresponding antigen or antibody binding to the solid phase immune adsorbent, the formation of the enzyme antigen antibody conjugate, the catalytic addition of the substrate to produce coloured products, can be based on the colour reaction of the depth of detection. Poli et al. presented the simple, sensitive, and accurate colorimetric capture ELISAs for staphylococcal enterotoxins A and B. The results showed that toxins could be accurately measured at 0.5 ng/mL in assay buffer or 0.1 ng/mL in human urine.[Citation15] This method is simple, rapid, sensitive, and specific, can be directly from the poisoning of enterotoxins, while complexity is not necessary for the extraction, concentration, and other means, and easy to automate.
Immunofluorescence assay
Immunofluorescence assay is used to detect the antibody with fluorescein (it is labeled) and the corresponding antigen by fluorescence microscope. The method is divided into direct and indirect methods. The direct method is to detect the sample directly with a specific fluorescent labelled antiserum. The results are observed using fluorescence microscope after washing. Indirect method and direct method are different, the fluorescent labelling antiserum and more than one anti-molecular or antigen molecules are combined. The detection limit is greatly improved; the sensitivity is greatly enhanced by the detection of various antibodies. Rowe et al. used immunofluorescence method in mixed samples were detected in the high molecular weight of S. aureus enterotoxin and low molecular weight of S. aureus enterotoxin.[Citation16]
Immune agar diffusion method
Agar diffusion is a reaction by soluble antigen and its corresponding antibody, which produce a white precipitation line, as a variation of the immunological method used in the detection of enterotoxin. Originally, this method is adopted to detect enterotoxin, which mainly has simple agar diffusion and agar diffusion test. The difference between them is that both the antigen and the antibody are spread separately, or both at the same time. Unidirectional diffusion is a quantitative test, which can be used for the determination of the content of immune proteins, while two-way diffusion is more used for qualitative testing. The detection of S. aureus has low sensitivity, and the increasing bacterial process is time-consuming, and needs a lot of time to achieve full detection with complicated operations. Nowadays, it is seldom used in the rapid detection of S. aureus.
Immunomagnetic beads separation method
IMS method, a technology that utilizes immunomagnetic beads as capturing reagents, has been developed for S. aureus isolation and identification.[Citation17] Deng et al. showed that S. aureus immune magnetic ball on target bacteria capture rate was more than 80% when the pure bacteria concentration was from 101 to 104 CFU/ml level.[Citation18] However, there are some limitations in IMS, such as high price and cross reaction, so other technical ways are needed to assist the detection.
Molecular biology methods
Polymerase chain reaction detection
The polymerase chain reaction (PCR) is the most well-known and established nucleic acid amplification technique for detecting pathogenic micro-organisms [Citation19]. This detection method is a specific enzymatic in vitro amplification of DNA by Mullis et al.[Citation20] PCR amplification can produce approximately 100 billion copies of one molecule of DNA in a few hours.[Citation21] In the method, a specific DNA fragment is amplified during a cyclic three-step process including: the target DNA is denatured at high temperature, two synthetic oligonucleotides (primers) one annealed to opposite strands at a temperature that only allows hybridization with correct target ().[Citation21] Polymerization is performed with the oligonucleotide as primers for the enzymes and the target DNA as template.[Citation22] The PCR technique has the distinct advantages of rapidity, specificity, sensitivity, efficiently, and less samples over culture-based methods, many PCR assays for the detection and validation of foodborne bacteria and viruses in food have been developed and applied in food samples. Nakano et al. developed a PCR assay for the detection of S. aureus targeting the 16S rRNA gene in various foods. The study found that the PCR assay detected the bacteria, during the inoculation (S. aureus) of 10–90 CFU/g into noodle foods containing endogenous microflora (103 to 105 CFU/g) followed by a 6-h enrichment culture step.[Citation23] PCR is a powerful technology, but the limitations of the PCR amplification technique include difficulty in obtaining a DNA preparation suitable for PCR amplification and the procedures are rather complicated with equipment and clean environment required. Furthermore, PCR cannot distinguish between live and dead cells and hence providing more false negative results.[Citation24]
Real-time fluorescence quantitative PCR
This technique is a refinement of the original PCR developed by Kary Mullis and coworkers.[Citation25] In real-time PCR, the amount of product formed is monitored during the course of the reaction by monitoring the fluorescence of dyes or probes introduced into the reaction that is proportional to the amount of product formed. The number of amplification cycles required to obtain a particular amount of DNA molecules is registered. Real-time PCR needs a fluorescent reporter that binds to the product formed and reports its presence by fluorescence. This limitation was resolved in 1992 with the development of real-time PCR by Higuchi et al.[Citation26] According to the real-time changes of fluorescence intensity can be detected, the copy number of the sample can be measured; it has the advantages of rapid, high specificity and high sensitivity. And real-time quantitative PCR is extremely accurate and less labour-intensive than current qualitative PCR methods.[Citation27] Hein et al. described two different real-time quantitative PCR approaches applied for PCR-based quantification of S. aureus cells by targeting the thermonuclease (nuc) gene. The results showed that application of the RT-PCR assay to quantify S.aureus cells in artificially contaminated cheeses of different types achieved sensitivities from 1.5 × 102 to 6.4 × 102 copies of the nuc gene/2 g, depending on the cheese matrix.[Citation28] Hence, the technology has also been widely used in molecular diagnostics, molecular biology research, animal and plant quarantine and food safety testing, and so on.
Loop-mediated isothermal amplification
Loop-mediated isothermal amplification (LAMP) was first developed in 2000 by the Japanese scientist Notomi et al.[Citation29,Citation30] This method relies on an auto-cycling strand displacement DNA synthesis performed by the Bst DNA polymerase enzyme at a constant temperature around 60 °C.[Citation31,Citation32] The mechanism of the LAMP reaction can be explained in three steps, an initial step, a cycling amplification step, and an elongation step, which is different from PCR in that four or six primers perform the amplification of the target gene ().[Citation30] It needs four (B3, F3, FIP, and BIP) or six primers (B3, F3, FIP, BIP, loop F, and loop R) to perform the target gene amplification without thermal cycling with high specificity.[Citation33,Citation34] Furthermore, the entire process, from bio-sample pre-treatment to optical detection, can be automatically completed within 60 min.[Citation35] The reaction time of the LAMP assay is shortened by using the loop primers. LAMP reactions usually result in about 103-fold or higher levels of amplification product with stem-loop DNAs in 60 min than with conventional PCR.[Citation36]
Figure 2. Schematic diagram of LAMP primers. In detail, eight distinct regions on every strand were used to design LAMP primers. For inner primers, forward inner primer (FIP) consisted of the complementary sequence of F1 (F1c), a T-T-T-T linker and F2, backward inner primer (BIP) consisted of complementary sequence of B1 (B1c), a T-T-T-T linker and B2. Outer primers F3 and B3 located outside of the F2 and B2 regions, with loop primers LF and LB located between F2 and F1 or B1 and B2.
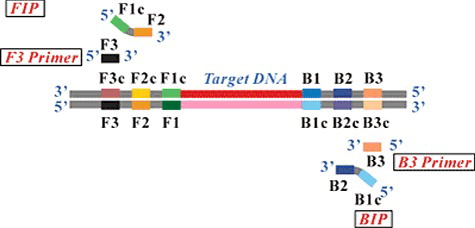
The magnesioum ions react with pyrophosphate ions from the dNTP release and a precipitate is formed. Therefore, it is easily to identify whether the amplification occurred or not without the need for cumbersome electrophoresis and ultraviolet observation process. Recently, Tomita et al. have developed an effective endpoint detection method using calcein, whose fluorescence is quenched by the binding of manganese ion.[Citation37] Yang et al. compared LAMP with PCR for detecting S. aureus, then concluded that detection limit of LAMP was 100 times lower than PCR.[Citation38] The technology has the characteristics of high specificity and sensitivity, simple operation, no special equipment, time-saving, and high efficiency. It can be widely used in many fields for resource-limited laboratories in rural areas of developing countries.[Citation30] However, the limitation of LAMP is that it needs high requirements of prime design, and easy to lead false positive result without rigorous experiment environments.
Gene chip detection
DNA microarray technology is a kind of oligonucleotide probe for detecting bacteria. An array of oligonucleotides are synthesized directly on a glass chip and then exposed to labelled target DNA. Genomic hybridization on whole genome arrays detects the presence or absence of similar DNA regions in sufficiently related micro-organisms, allowing genome-wide comparison of their genetic contents. A whole genome array is based on a sequenced bacterial isolate, as a collection of DNA probes fixed on a solid support. In a single hybridization experiment, the absence/presence status of all genes of the sequenced microbe in the queried isolate can be examined.[Citation39] DNA chip technology makes it possible to detect diverse individual sequences in complex DNA samples.[Citation40] Compared with conventional microbiological detection method, it is characterized by its outstanding variety of target genes that can be simultaneously detected and identified with high throughput detection, simple, rapid, high sensitivity, automation, etc. However, with complex protocol and high price, gene chip method is seldom used in the detection of S. aureus in practical application.
Nucleic acid probe technique
Nucleic acid probe technology is based on nucleic acid hybridization. The principle is the two-base complementary DNA chain under the appropriate conditions, so that with a specific identification tag (as a marker, biotin labeled) DNA fragments and the subject of the specimen can be detected in the DNA fragments hybridized to determine whether the sample contains target bacteria. Shin et al. described a real-time isothermal target and probe amplification (iTPA) method that allowed the rapid and simultaneous amplification of the S. aureus nuc gene and a fluorescence resonance energy transfer-based signal probe under 61 °C isothermal conditions or detection of S. aureus in real time.[Citation41] The results showed that the assay was able to specifically detect all 91 S. aureus strains tested without nonspecific detection of 51 non–S. aureus strains, and detect S. aureus at an initial level of 101 CFU in overnight cultures of pre-enriched food samples.
Biosensor technology
Bio-sensing science is a multidisciplinary area, which is developed by the mutual infiltration of biological engineering and various technical disciplines. Biosensor is an analytical device based on biochemical and sensing technology, which converts a biological response into an electrical signal. It consists of following components: a bioreceptor or biorecognition element, which recognizes the target analyte and a transducer, for converting the recognition event into a measurable electrical signal.[Citation42] Schematic diagram of a biosensor is shown in [Citation43] The potential application of biosensor technology to food testing offers several attractive features. Depending on the biorecognition element employed, the biosensors can be divided into enzyme sensor, microbial sensor, issue sensor, organelle sensor, antibody sensor nucleic acid sensor, biomimic sensor, etc.[Citation43,Citation44] In food microbiology detection, biosensor is mainly employed in toxins and genes detection. Ritche et al. found that it was interesting to note that a majority of biosensors used antibody for capture and detection of the target analyte.[Citation45] S. aureus cells were detected in pure culture at concentrations as low as 1000 cells/mL in a relatively short assay time of 30 min by the immunosensor.[Citation46] Biosensor detection method is fast and can be used for the detection of many substances. However, many of them are still in the research stage at present with high cost, which is not conducive to the promotion of the technology.
Conclusions
Food safety is a global public health problem, with the continuous development of economy and society. How to detect the foodborne pathogenic bacteria using a fast and effective method is of great significance. The traditional method of microbial culture has the advantages of simple operation, low cost, but the step of pre-enrichment, selective enrichment, selective plating, biochemical screening, and serological confirmation are so complicated that cannot meet the detection of on-site implementation. Chromomeric medium has the presence of interference reaction of false positive and false-negative strains, and should be associated with other methods to improve the detection accuracy. Fluorescent quantitative PCR is more sensitive and with higher specificity than conventional PCR, but is still limited in laboratory work due to the high cost. LAMP is a rapid, flexible, and simple tool with high specificity and sensitivity for the identifications of S. aureus comparable to the Chinese National Standard method. Only simple equipment, as a water bath, is required for the operation of LAMP assay, which is also costless. For the issue of foodborne pathogen detection, a single detection technology has failed to meet the test requirements at this stage. The combination of several main methods is also a hot spot (for example, ELISA-PCR method, immuno-PCR, DNA biosensor combined with PCR technology, LAMP combined with biological fluorescent real-time detection technology) to greatly improve the sensitivity and efficiency. The brief comparison of various detection methods of S. aureus are shown in . In summary, there are a host of promising applications in the field of rapid and automated detection methods for S. aureus. Given the broad applicability and the great potential of such methods, there is still a great chance for further developments in the near future. In these situations, S. aureus detection will be toward the low detection limit and cost, high specificity and sensitivity, simplicity, and automation.
Table 1. Comparison of various detection methods of Staphylococcus aureus.
Disclosure statement
No potential conflict of interest was reported by the authors.
Additional information
Funding
References
- Deurenberg RH, Stobberingh EE. The evolution of Staphylococcus aureus. Infect Genet Evol. 2008;8(6):747–763.
- Shylaja R, Thakasi DKK, Murali HS, et al. Application of a chimeric protein construct having enterotoxin B and toxic shock syndrome toxin domains of S. aureus in immunodiagnostics. Indian J Microbiol. 2012;52(3):449–455.
- Tayel AA, El-tras WF. Anticandidal activity of pomegranate peel extract aerosol as an applicable sanitizing method. Mycoses. 2010;53(2):117–122.
- Ying Y, Xiaoyan M, Wei Z. Detection of Staphylococcus aureus in milk using real-time fluorescence loop-mediated isothermal amplification. Adv J Food Sci Techn. 2015;8(9):678–684.
- Yan L, Orhan S, Lei D, et al. Development of a loop-mediated isothermal amplification assay for rapid, sensitive and specific detection of a Campylobacter jejuni clone. J Vet Med Sci. 2012;74(5):591–596.
- Moreira A, Conceição F, Conceição RC, et al. Detection of Salmonella typhimurium in raw meats using in-house prepared monoclonal antibody coated magnetic beads and PCR assay of the fimA gene. J Immunoassay Immunoch. 2008;29(1):58–69.
- Zhao X, Li Y, Wang L, et al. Development and application of a loop-mediated isothermal amplification method on rapid detection Escherichia coli O157 strains from food samples. Mol Biol Rep. 2010;37(5):2183–2188.
- Doyle MP, Robert L. Food microbiology: fundamentals and frontiers. Washington, DC: American Society for Microbiology Press; 2012.
- Mandal P, Biswas A, Choi K, et al. Methods for rapid detection of foodborne pathogens: an overview. Am J Food Techn. 2011;6(2):87–102.
- Malorny B, Tassios PT, Rådström P, et al. Standardization of diagnostic PCR for the detection of foodborne pathogens. Int J Food Microbiol. 2003;83(1):39–48.
- Wehr H, Frank J. Standard methods for the examination of dairy products. Washington, DC: American Public Health Association; 2004.
- Hirvonen JJ, Kerttula AM, Kaukoranta SS. Performance of saselect, a chromogenic medium for detection of Staphylococci in clinical specimens. J Clin Microbiol. 2014;52(4):1041–1044.
- Kim SR, Lee SH, Seo MK, et al. Evaluation of selective media for isolation of Staphylococcus aureus from agricultural products. J Food Hygiene Safety. 2012;27(2):169–175.
- Schoeller NP, Ingham SC. Comparison of the baird–parker agar and 3M™ petrifilm™ rapid S.aureus count plate methods for detection and enumeration of Staphylococcus aureus. Food Microbiol. 2001;18(6):581–587.
- Poli MA, Rivera VR, Neal D. Sensitive and specific colorimetric ELISAs for Staphylococcus aureus enterotoxins A and B in urine and buffer. Toxicon. 2002;40(40):1723–1726.
- Rowe MT. Predictive microbiology: uses for assessing quality and safety of dairy products. J Ind Microbiol Biot. 1993;12(12):330–336.
- Sung YJ, Suk HJ, Sung HY, et al. Novel antibody/gold nanoparticle/magnetic nanoparticle nanocomposites for immunomagnetic separation and rapid colorimetric detection of Staphylococcus aureus in milk. Biosens Bioelectron. 2013;43(1):432–439.
- Deng Y, Xu D, Linna Z, et al. Study on the immune magnetic bead capture-PCR detection of Staphylococcus aureus in milk. Food Ind Techn. 2015;36(7):304–313.
- Zhao X, Lin CW, Wang J, et al. Advances in rapid detection methods for foodborne pathogens. J Microbiol Biotechn. 2014;24(3):297–312.
- Mullis K, Faloona F, Scharf S, et al. Specific enzymatic amplification of DNA in vitro: the polymerase chain reaction. Cold Spring Harb Symp Quant Biol. 1986;51(1):263–273.
- Templeton NS. The polymerase chain reaction history methods, and applications. Diagn Mol Pathol. 1992;1(1):58–72.
- Kubista M, Andrade JM, Bengtsson M, et al. The real-time polymerase chain reaction. Mol Aspects Med. 2006;27(2):95–125.
- Nakano S, Kobayashi T, Funabiki K, et al. PCR detection of Bacillus and Staphylococcus in various foods. J Food Protect. 2004;67(6):1271–1277.
- Zhao X, Wang J, Forghani F, et al. Rapid detection of viable Escherichia coli O157 by coupling propidium monoazide with loop-mediated isothermal amplification. J Microbiol Biotechnol. 2013;23(12):1708–1716.
- Saiki RK, Scharf S, Faloona F, et al. Enzymatic amplification of beta-globin genomic sequences and restriction site analysis for diagnosis of sickle cell anemia. Science. 1985;230(4732):1350–1354.
- Higuchi R, Dollinger G, Walsh PS, et al. Simultaneous amplification and detection of specific DNA sequences. Bio/technology. 1992;10(4):413–417.
- Gibson U, Heid CA, Williams PM. A novel method for real time quantitative RT-PCR. Genome Res. 1996;6(10):995–1001.
- Hein I, Lehner A, Rieck P, et al. Comparison of different approaches to quantify Staphylococcus aureus cells by real-time quantitative PCR and application of this technique for examination of cheese. Appl Environ Microb. 2001;67(7):3122–3126.
- Mori Y, Kanda H, Notomi T. Loop-mediated isothermal amplification (LAMP): recent progress in research and development. J Infect Chemother. 2013;19(3):404–411.
- Mori Y, Notomi T. Loop-mediated isothermal amplification (LAMP): a rapid, accurate, and cost-effective diagnostic method for infectious diseases. J Infect Chemother. 2009;15(2):62–69.
- Zhao X, Chen X, Zhang Y, et al. Development and evaluation of reverse-transcription loop-mediated isothermal amplification for rapid detection of human immunodeficiency virus type 1. Indian J Med Microbiol. 2012;30(4):391–396.
- Zhao X, Wang L, Chu J, et al. Rapid detection of Vibrio parahaemolyticus strains and virulent factors by loop-mediated isothermal amplification assays. Food Sci Biotechnol. 2010;19(5):1191–1197.
- Zhao X, Wang L, Chu J, et al. Development and application of a rapid and simple loop- mediated isothermal amplification method for food-borne Salmonella detection. Food Sci Biotechnol. 2010;19(6):1655–1659.
- Zhao X, Li Y, Park M, et al. Loop-mediated isothermal amplification assay targeting the femA gene for rapid detection of Staphylococcus aureus from clinical and food samples. J Microbiol Biotechnol. 2013;23(2):246–250.
- Wang CH, Lien KY, Wu JJ, et al. A magnetic bead-based assay for the rapid detection of methicillin-resistant Staphylococcus aureus by using a microfluidic system with integrated loop-mediated isothermal amplification. Lab Chip. 2011;11(8):1521–1531.
- Wang XR, Wu LF, Wang Y, et al. Rapid detection of Staphylococcus aureus by loop-mediated isothermal amplification. Appl Biochem Biotechnol. 2015;175(2):882–891.
- Mori Y, Nagamine K, Tomita N, et al. Detection of loop-mediated isothermal amplification reaction by turbidity derived from magnesium pyrophosphate formation. Biochem Biophys Res Commun. 2001;289(1):150–154.
- Yang H, Ma X, Zhang X, et al. Development and evaluation of a loop-mediated isothermal amplification assay for the rapid detection of Staphylococcus aureus in food. Eur Food Res Technol. 2011;232(5):769–776.
- Garaizar J, Rementeria A, Porwollik S. DNA microarray technology: a new tool for the epidemiological typing of bacterial pathogens? FEMS Immunol Med Microbiol. 2006;47(2):178–189.
- Pitcher D, Saunders N, Owen R. Rapid extraction of bacterial genomic DNA with guanidium thiocyanate. Lett Appl Microbiol. 1989;8(4):151–156.
- Shin H, Kim M, Yoon E, et al. Isothermal target and probe amplification assay for the real-time rapid detection of Staphylococcus aureus. J Food Protect. 2015;78(4):723–727.
- Chuang TL, Chang CC, Yu CS, et al. Disposable surface plasmon resonance aptasensor with membrane-based sample handling design for quantitative interferon-gamma detection. Lab Chip. 2014;14(16):2968–2977.
- Velusamy V, Arshak K, Korostynska O, et al. An overview of foodborne pathogen detection: in the perspective of biosensors. Biotechnol Adv. 2010;28(2):232–254.
- Arora P, Sindhu A, Kaur H, et al. An overview of transducers as platform for the rapid detection of foodborne pathogens. Appl Microbiol Biotechnol. 2013;97(5):1829–1840.
- Richter ER. Biosensors: applications for dairy food industry. J Dairy Sci. 1993;76(10):3114–3117.
- Koydemir HC, Kulah H, Alp A, et al. A fully microfabricated electrochemical sensor and its implementation for detection of methicillin resistance in Staphylococcus aureus. IEEE Sens J. 2014;14(6):1844–1851.