Abstract
In an attempt to synthesise new tyrosinase inhibitors, we designed and synthesised a series of chalcone-hydroxypyridinone hybrids as potential tyrosinase inhibitors adopting strategic modifications of kojic acid. All the newly synthesised compounds were characterised by NMR and mass spectrometry. Initial screening of the target compounds demonstrated that compounds 1a, 1d, and 1n had relatively strong inhibitory activities against tyrosinase monophenolase, with IC50 values of 3.07 ± 0.85, 2.25 ± 0.8 and 2.75 ± 1.19 μM, respectively. The inhibitory activity against monophenolase was 6- to 8-fold higher than that of kojic acid. Compounds 1a, 1d, and 1n also showed inhibition of diphenolase, with IC50 values of 17.05 ± 0.07, 11.70 ± 0.03 and 19.3 ± 0.28 μM, respectively. The inhibition kinetics of diphenolase indicates that compounds 1a and 1d induce reversible inhibition on tyrosinase. Finally, we found that copper coordination should be one of the important inhibitory mechanism of these compounds in tyrosinase.
1. Introduction
Tyrosinase is a copper-containing metalloenzyme widely distributed in animals, plants, and microorganisms and is a key and rate-limiting enzyme for melanogenesisCitation1. It is isolated from diverse resources and its structural properties are well documentedCitation2. A di-copper centre is the common structural unit of the active site which is found in all species. Each copper ion is coordinated by three histidine residues. Further, antiferromagnetic coupling of the two copper ions renders the active site EPR inactiveCitation3. During the tyrosinase-catalysed oxidation process, the binuclear metal centre activates an O2 molecule and subsequently oxidises substrates such as l-tyrosine and l-DOPA to catecholates and benzoquinones, respectivelyCitation4. As tyrosinase plays a fundamental role in the melanogenesis process, the abnormal function of this enzyme poses challenges in a range of socio-economic areas such as agriculture, food, and the pharmaceutical industryCitation5. Tyrosinase regulates pigmentation, and abnormal expression can lead to various pigmented diseases such as freckles and age spotsCitation6. If the expression is insufficient, skin diseases such as those associated with albinism and vitiligo will occur. In fruits and vegetables, tyrosinase causes browning during post-harvest processing, transportation, and storage, affecting its quality and commercial valueCitation7.
There are many tyrosinase inhibitors originating from both natural sources and chemical synthesisCitation8. Kojic acid, vitamin C, cysteine, and arbutin are inhibitors isolated from natural sources. Although ideal in many respects, kojic acid has not found wide application due to its metabolic instabilityCitation9. Benzaldehyde, 4-halo-benzoic acids, and 4-chlorosalicylic acid are tyrosinase inhibitors of synthetic originCitation10. In addition, extracts from animals and fish, collagen peptides, sorghum peptides have also been found to possess inhibitory effects on tyrosinaseCitation11,Citation12.
Development of useful tyrosinase inhibitors, possessing potent commercial utility has been limited to date due to poor solubility, low shelf-life, and safety concernsCitation13. Kojic acid, a secondary metabolite produced by Aspergillus and Penicillium moulds, provides a promising starting point for the synthesis of new tyrosinase inhibitors; the α-hydroxy ketone functionality plays an important role in tyrosinase inhibitionCitation14,Citation15. Based on previous results, relating to hydroxypyridinonesCitation16–19, we have functionalised kojic acid to form new derivatives in attempt to identify more potent tyrosinase inhibitors.
As many chalcones possess tyrosinase inhibitory activities (IC50 value for tyrosinase inhibition of 2′,4′,4-trihydroxychalcone is 8.1 μM)Citation20,Citation21, it was decided to investigate the properties of chalcone analogues (1) which contain either a pyranone or pyridinone ring ().
2. Materials and methods
2.1. Chemistry
1H NMR and 13 C NMR spectra were recorded on a Bruker Avance 400 spectrometer (Bruker Corp., Karlsruhe, Germany) with TMS as an internal standard. Electrospray ionisation (ESI) mass spectra were obtained by infusing samples into an LCQ Deca XP ion trap instrument (ThermoFinnigan, SanJose, CA). High resolution mass spectra (HRMS) were determined on Waters QTOF micro (Waters, USA). Lyophilised powder of mushroom tyrosinase (≥1000 U/mg) was purchased from Sigma. Kojic acid, l-tyrosine, and l-DOPA of analytical grade were purchased from Aladdin chemicals, Shanghai, China. All other chemicals were of AR grade and used without any further purification. 5-Benzyloxy-2-hydroxymethyl-pyran-4-one was prepared according to previous reportCitation22. Compounds 3a–3d, 4a–4e, 6a–6d and 7a–7d were prepared according to previous reportsCitation16,Citation17,Citation23,Citation24. General procedure for the synthesis of compounds 1a–1o and their physical and spectroscopic data are presented in Supplementary Materials.
2.2. Tyrosinase inhibition assay
2.2.1. Monophenolase and diphenolase inhibition assay
Monophenolase and diphenolase activity was measured as described previously by monitoring the dopachrome absorbance at 475 nmCitation17. l-Tyrosine and l-DOPA were used as reaction substrate for monophenolase and diphenolase assays, respectively. In the reaction, l-tyrosine (50 µL, 2 mM) or l-DOPA (50 µL, 0.5 mM), 90 µL of pH 6.8 phosphate buffer and 5 µL volume of different concentrations of final compounds (1a–1o) in DMSO were mixed and incubated at 30 °C. Finally, 20 units of enzyme was quickly added to the reaction mixture and incubated at 30 °C for 10 min. The absorbance at 475 nm was recorded with a microplate reader. During these incubations, the final concentration of DMSO was limited to 3% by volume. The assay was carried out in triplicate and DMSO was used as controlCitation17.
2.2.2. Inhibition kinetics on diphenolase activity of tyrosinase
The same experimental protocol described above was adapted for inhibition kinetics, with different inhibitor concentration. However the absorbance was measured at 1 min reaction intervals for twelve minutesCitation16.
2.2.3. Reversibility of inhibitory effect of compounds on tyrosinase
Measurement method was substantially the same to that described above, changing the added concentration of the enzyme solution, different concentrations of inhibitor on mushroom tyrosinase l-DOPA as reaction substrateCitation16.
2.3. pKa and copper(II) stability constants determination
An automated titration system used in the study consists of a Metrohm 765 Dosimat autoburette, a Mettler Toledo MP230 pH metre with SENTEK pH electrode (P11), and an HP 8453 UV-visible spectrophotometer with a Hellem quartz flow cuvette being circulated through by a Gilson Mini-plus #3 pump—speed capability (20 ml/min). A potassium chloride electrolyte solution (0.1 M) was used to maintain the ionic strength. The temperature of the test solutions was maintained in a thermostatic jacketed titration vessel at 25 °C (± 0.1 °C) using a Fisherbrand Isotemp water bath. The pH electrodes were calibrated using GLEECitation25 with data obtained by titrating a volumetric standard HCl (0.1 M) in KCl (0.1 M) with KOH (0.1 M) under an argon gas atmosphere in the vessel. The solution under investigation was stirred vigorously during the experiment. For pKa determinations, a cuvette path length of 10 mm was used while for metal stability constant determinations, a cuvette path length of 50 mm was used. All instruments were interfaced to a computer and controlled by an in-house programme. An automated titration adopted the following strategy: the pH of a solution was increased by 0.1 pH unit by the addition of KOH solution (0.1 M) from the autoburette. The pH readings were judged to be stable if their values varied by less than 0.01 pH unit after a set incubation period. For pKa determinations, an incubation period of 1.5 min was adopted; for copper(II) stability constant determinations, an incubation period of 3 min was adopted. The cycle was repeated until the defined end point pH value was achieved. Titrations were carried out in the solution with molar ratio of DMSO: H2O being 0.2: 1 due to the solubility issue of samples and/or the corresponding copper(II) complexes. Under this condition, the pH metre readings are shifted, compared to the aqueous solution. All the titration data were analysed with the HypSpec2014 programme (http://www.hyperquad.co.uk/)Citation26,Citation27. The speciation plot and pM values were calculated with the HYSS programmeCitation28. Based on deferiprone titrations, pCu2+ at pH 7.6, denoted as pCu2+7.6, with [ligand]total = 10 µM and [Metal]total = 1 µM, were calculated as close approximations for pCu2+ at pH 7.4 in the aqueous solution. Analytical grade reagent materials were used in the preparation of all solutions.
2.4. Molecular docking study
Molecular docking was performed by using CDOCKER module embedded in Discovery Studio 2.5 software (Accelrys Software, Inc., San Diego, CA, USA)Citation17. The X-ray crystal structure of tyrosinase from Agaricus bisporus (PDB ID: 2Y9X) was retrieved from the Protein Data Bank (http://www.rcsb.org/pdb). All crystallographic water molecules and ions were removed from the protein structure. 3 D structure of compound 1d was generated in Chem3D Ultra 8.0, and conformations were generated by using a modified CHARMm force field. The obtained conformations were then docked into the binding site of tyrosinase. The docked conformation with the lowest energy was used for the analysis of binding mode.
2.5. Statistical analysis
All the experiments were performed in triplicate. The data were statistically analysed using MS-Excel and GraphPad Prism 6 software.
3. Results and discussion
3.1. Chemistry
The synthetic route and complete reaction conditions for the preparation of compounds 1a–1o are illustrated in Scheme 1. The benzyl protection of kojic acid with BnCl in presence of sodium hydroxide and methanol:water (1:1) as reaction solvent at 70 °C gave the intermediate compound 2. Further, refluxing of intermediate 2 with different aliphatic amines in ethanol gave the intermediates (3a–3d) in good yield. The corresponding aldehydes (4a–4e) were afforded by the selective oxidation of compounds 3a–3d with active MnO2, in moderate yield. The phosphonium salts (7a–7d) were synthesised by reaction of α-bromo acetophenones (6a–6d) with triphenylphosphine in dichloromethane in excellent yield. Finally, the target hybrid compounds (1a–1o) were obtained using the Wittig reaction by the condensation of phosphonium salts 7 with aldehydes (4a–4e) in presence of tert-BuOK in dry THF and subsequent deprotection of benzyl group with BBr3 in dichloromethane at 0 °C. All the newly synthesised compounds were characterised using 1H NMR, 13 C NMR and HRMS.
Scheme 1. Synthetic route of compounds 1. Reagents and conditions: (i) BnCl, NaOH, MeOH/H2O, 70 °C, 6 h, 80% yield; (ii) appropriate amines, EtOH, reflux, overnight, (iii) MnO2, 1,4-dioxane, (iv) Br2, CHCl3, rt, 10 min; (v) PPh3, CH2Cl2, 30 min, (vi) tert-BuOK, dry THF, (vii) BBr3, DCM, 0 °C to rt, 2 h, 55–72% yield.
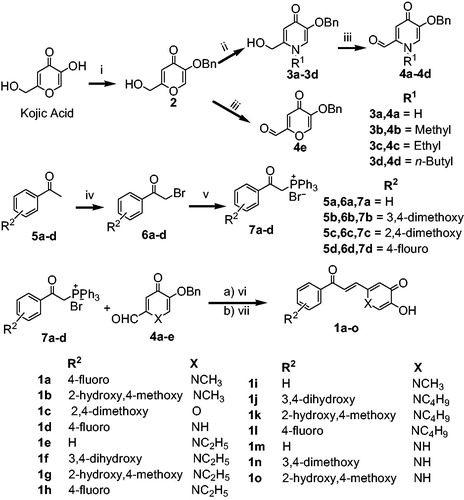
3.2. Inhibitory effect of compounds 1a–1o on monophenolase activity
All the final compounds (1a–1o) were screened for monophenolase inhibitory activity at 50 μM (). Four compounds (1a, 1d, 1f, and 1n) were found to possess a stronger inhibitory activity on monophenolase activity of mushroom tyrosinase than kojic acid under the conditions defined in . The inhibition rate of 1a, 1d, 1f, and 1n was 85.3%, 89.5%, 78.8% and 87.6% at 50 μM, respectively, which was higher than that of kojic acid (75.4%). These four compounds were tested at different concentrations to realise IC50 values (); 1a 3.07 ± 0.85 μM, 1d 2.25 ± 0.80 μM, 1f 8.11 ± 2.67 μM, and 1n 2.75 ± 1.19 μM. The inhibitory effects of 1a, 1d, 1f, and 1n were 5.7-, 7.8-, 2.2-, and 6.4-fold higher than that of kojic acid (IC50 = 17.55 ± 1.91 μM). Compounds 1a, 1d, and 1n possess obviously superior monophenylase inhibitory activity to that of 2′,4′,4-trihydroxychalcone (IC50 = 8.1 μM, inhibition rate is 67% at 50 μM)Citation20, while the activity of 1f is close to that of 2′,4′,4-trihydroxychalcone. The substituents on position-1 (X) and on the benzene ring (R2) (Scheme 1), and hydrophobicity of these molecules reflected by their calculated partition coefficients (clogP) influence the monophenylase inhibitory activity (). The four most active inhibitors possessed clogP values equal to or less than 1.6 and they also have strong electron withdrawing functions on the benzene ring, i.e. F, OH or OMe. The inductive effect will dominate for F and OMe substituents. When the pyridinone ring N is replaced by an oxygen (1c), low inhibitory activity resulted. When the clogP value was greater than 1.6, there was an appreciable reduction in inhibitory potency (1c, 1 g, 1 h, 1j, 1k, 1 l). The 2-hydroxy-4-methoxybenzene aromatic rings (1 b, 1o) and unsubstituted benzene (1e, 1 m) were also found to lack potent inhibitory properties. Thus the presence of 4-fluoro- or 3,4-dimethoxy benzene in the structure leads to optimal activity together with either a NH or NMe in the pyridinone ring.
Figure 2. Inhibitory effect of 1a, 1d, 1f, and 1n on the monophenolase activity of mushroom tyrosinase. The assays were performed at 30 °C and pH 6.8.
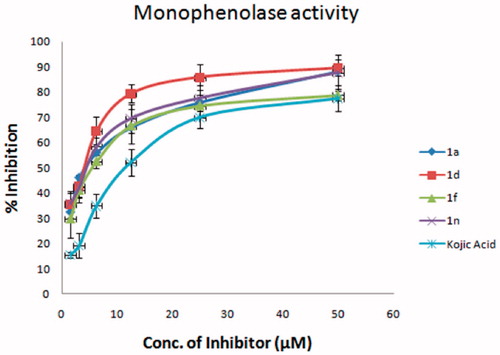
Table 1. Inhibition of compounds (1a–1o) (50 µM) on monophenolase activity of mushroom tyrosinase under the conditions of 30 °C and pH 6.8.
3.3. Inhibitory kinetics and reversibility on diphenolase activity of tyrosinase
The inhibitory kinetic courses of mushroom tyrosinase in the presence of different concentrations of compounds 1a and 1d was investigated by monitoring their inhibition on diphenolase inhibitory activity using l-Dopa as a substrate. The formation of o-quinone generated by the oxidation of l-Dopa increased with time, and the absorbance values reduced with increasing concentration of compounds 1a and 1d (). In addition, the reaction process catalysed by the diphenolase activity of tyrosinase had no lag time. This result is in agreement with those of previous reportsCitation18,Citation19.
Figure 3. Inhibitory effect of different concentrations of 1a (a) and 1d (b) on the diphenolase activity of tyrosinase. The assays were performed at 30 °C and pH 6.8.
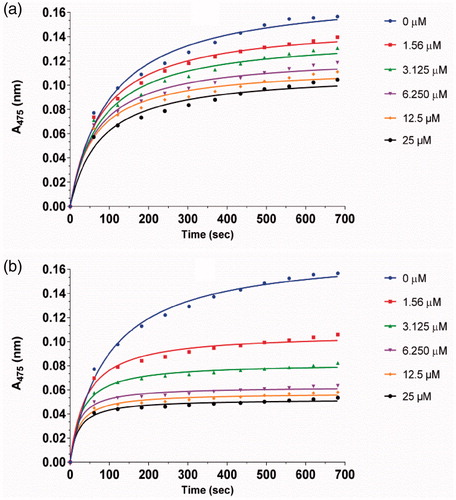
The inhibition of compounds 1a, 1d, and 1n on the diphenolase activity of tyrosinase increased with increasing concentration of inhibitor. The IC50 values of 1a, 1d, and 1n were determined to be 17.1 ± 0.07 µM, 11.7 ± 0.03 µM, and 19.3 ± 0.28 respectively.
The inhibitory reversibility of 1a and 1d on mushroom tyrosinase was investigated using l-DOPA as a substrate. For both compounds, investigation on the relationship between enzyme activity and its concentration in the presence of compounds 1a and 1d indicated that the plots of the remaining enzyme activity versus the concentration of enzyme at different inhibitor concentrations gave a family of straight lines, which all passed through the origin (). Increase of inhibitor concentration resulted in descent of the slope of the line, indicating that the presence of inhibitor resulted in the inhibition of enzyme activity. Thus, the inhibition of both compounds 1a and 1d on diphenolase activity of tyrosinase is reversible.
3.4. pKa values and copper(II) affinity constant of 1n
It is well known that the main inhibitory mechanism of kojic acid on tyrosinase involves chelation of copper in the active site in tyrosinase. Thus, for the purpose of exploring the inhibitory mechanism of compounds 1 on tyrosinase, the pKa values of 1n and its affinity for copper(II) were determined. The proton equilibria of 1n are presented in Scheme 2. Using the spectrophotometric titration method, the three pKa values of 1n obtained from nonlinear least-squares regression analysis were found to be 3.3, 8.8, and 12.0, which correspond to the 4-oxo functional group, the 5-hydroxyl group, and 1-NH, respectively. As a bidentate, 1n can form two species of copper complexes, CuLH and CuL2H2 (assuming 1-NH group does not deprotonate when forming the complexes with copper). The distribution of these two complexes varies with the pH (). The log stability constants of these two complexes (CuLH and CuL2H2), log β1 and log β2, were determined to be 20.9 and 40.4, respectively. The pCu2+ value is a more suitable parameter to reflect the copper affinity of ligands, which is measured under the conditions of [Cu]total=10−6M, [L]total=10−5M, pH 7.4. The pCuCitation2+ value of 1n was calculated from the pKa values and the log stability constants of its copper complexes using HYSS programme, being 9.9, which is greater than that of kojic acid (pCu2+=7.3)Citation29. Thus, copper chelation is undoubtedly an important inhibitory mechanism on tyrosinase.
3.5. Molecular docking study
In order to understand the interaction mode of inhibitor binding to tyrosinase, the molecular docking of compound 1d to Agaricus bisporus tyrosinase was performed. As shown in , compound 1d binds to the active site of tyrosinase at the bottom of hydrophobic cavity of the receptor. The 4-oxy is located between Cu1 and Cu2 with a distance of 2.47 and 2.13 Å, respectively. The distance between Cu1 and the oxygen in 5-hydroxy group is 2.53 Å. Thus, the 4-oxy and 5-hydroxy group on the pyridinone ring can coordinate with Cu1, forming a 5-membered chelating ring; the 4-oxy can also coordinate with Cu2. Compound 1d interacts with the side chain of Val283 and Val 248 via hydrophobic contacts, and interacts with the copper ligands His 61 and His263 via pi–pi stack, indicating the formation of a stable conformation.
4. Conclusions
Tyrosinase is an essential enzyme in melanogenesis metabolic process in microorganisms, plants, and animals, and thus is an attractive target for the discovery of novel anti-tyrosinase agents. In the present study, we have synthesised and screened a series of new chalcone analogues containing hydroxypyridinone moiety. Using enzyme inhibition assays, we have identified compound 1a, 1d, and 1n as potential lead molecules. The inhibitory effect of compounds 1a and 1d on diphenolase activity of mushroom tyrosinase activity is reversible. These findings support the previous proposal that kojic acid modification is a promising strategy for developing new potential tyrosinase inhibitorsCitation30,Citation31
Supplemental Material
Download PDF (230.1 KB)Disclosure statement
The authors declare no conflict of interest, financial or otherwise.
Additional information
Funding
References
- Zaidi KU, Ali AS, Ali SA, Naaz I. Microbial tyrosinases: promising enzymes for pharmaceutical, food bioprocessing, and environmental industry. Biochem Res Int 2014; 2014:854687.
- Kim YJ, Uyama H. Tyrosinase inhibitors from natural and synthetic sources: structure, inhibition mechanism and perspective for the future. Cell Mol Life Sci 2005;62:1707–23.
- Decker H, Schweikardt T, Tuczek F. The first crystal structure of tyrosinase: all questions answered? Angew Chem Int Ed Engl 2006;45:4546–50.
- Olivares C, Solano F. New insights into the active site structure and catalytic mechanism of tyrosinase and its related proteins. Pigment Cell Melanoma Res 2009; 22:750–60.
- Lai SC, Chen CC, Hou RF. Electron microscopic observations on wound-healing in larvae of the mosquito Armigeres subalbatus (Diptera: Culicidae). J Med Entomol 2001; 38:836–43.
- Crespo MI, Chaban MF, Lanza PA, et al. Inhibitory effects of compounds isolated from Lepechinia meyenii on tyrosinase. Food Chem Toxicol 2019;125:383–91.
- Mosneaguta R, Alvarez V, Barringer SA. The effect of antibrowning agents on inhibition of potato browning, volatile organic compound profile, and microbial inhibition. J Food Sci 2012;77:C1234–C1240.
- Lee SY, Baek N, Nam TG. Natural, semisynthetic and synthetic tyrosinase inhibitors. J Enzyme Inhib Med Chem 2016;31:1–13.
- Zheng ZP, Zhang YN, Zhang S, Chen J. One-pot green synthesis of 1,3,5-triarylpentane-1,5-dione and triarylmethane derivatives as a new class of tyrosinase inhibitors. Bioorg Med Chem Lett 2016;26:795–8.
- Seo SY, Sharma VK, Sharma N. Mushroom tyrosinase: recent prospects. J Agric Food Chem 2003;51:2837–53.
- Chang TS. An updated review of tyrosinase inhibitors. Int J Mol Sci 2009;10:2440–75.
- Burdock GA, Soni MG, Carabin IG. Evaluation of health aspects of kojic acid in food. Regul Toxicol Pharmacol 2001;33:80–101.
- Zolghadri S, Bahrami A, Hassan Khan MT, et al. A comprehensive review on tyrosinase inhibitors. J Enzyme Inhib Med Chem 2019;34:279–309.
- Chaves S, Piemontese L, Hiremathad A, Santos MA. Hydroxypyridinone derivatives: a fascinating class of chelators with therapeutic applications - an update. Curr Med Chem 2018;25:97–112.
- Nurchi VM, de Guadalupe Jaraquemada-Pelaez M, Crisponi G, et al. A new tripodal kojic acid derivative for iron sequestration: synthesis, protonation, complex formation studies with Fe3+, Al3+, Cu2+ and Zn2+, and in vivo bioassays. J Inorg Biochem 2019;193:152–65.
- Li DF, Hu PP, Liu MS, et al. Design and synthesis of hydroxypyridinone-L-phenylalanine conjugates as potential tyrosinase inhibitors. J Agric Food Chem 2013;61:6597–603.
- Shao LL, Wang XL, Chen K, et al. Novel hydroxypyridinone derivatives containing an oxime ether moiety: synthesis, inhibition on mushroom tyrosinase and application in anti-browning of fresh-cut apples. Food Chem 2018;242:174–81.
- Zhao DY, Zhang MX, Dong XW, Hu YZ, et al. Design and synthesis of novel hydroxypyridinone derivatives as potential tyrosinase inhibitors. Bioorg Med Chem Lett 2016;26:3103–8.
- Chen K, Zhao DY, Chen YL, et al. A novel inhibitor against mushroom tyrosinase with a double action mode and its application in controlling the browning of potato. Food Bioprocess Technol 2017;10:2146–55.
- Nerya O, Musa R, Khatib S, et al. Chalcones as potent tyrosinase inhibitors: the effect of hydroxyl positions and numbers. Phytochemistry 2004;65:1389–95.
- Jun N, Hong G, Jun K. Synthesis and evaluation of 2',4',6'-trihydroxychalcones as a new class of tyrosinase inhibitors. Bioorg Med Chem 2007;15:2396–402.
- Yi W, Dubois C, Yahiaoui S, et al. Refinement of arylthiosemicarbazone pharmacophore in inhibition of mushroom tyrosinase. Eur J Med Chem 2011;46:4330–5.
- Singh LR, Avula SR, Raj S, et al. Coumarin-benzimidazole hybrids as a potent antimicrobial agent: synthesis and biological elevation. J Antibiot 2017;70:954–61.
- Sashidhara KV, Palnati GR, Avula SR, et al. Synthesis and evaluation of anti-thrombotic activity of benzocoumarin amide derivatives. Bioorg Med Chem Lett 2012;22:3115–21.
- Gans P, O'Sullivan B. GLEE, a new computer program for glass electrode calibration. Talanta 2000;51:33–7.
- Gans P, Sabatini A, Vacca A. Determination of equilibrium constants from spectrophometric data obtained from solutions of known pH: the program pHab. Ann Chim 1999;89:45–9.
- Gans P, Sabatini A, Vacca A. Investigation of equilibria in solution. Determination of equilibrium constants with the HYPERQUAD suite of programs. Talanta 1996;43:1739–53.
- Alderighi L, Gans P, Ienco A, et al. Hyperquad simulation and speciation (HySS): a utility program for the investigation of equilibria involving soluble and partially soluble species. Coordin Chem Rev 1999;184:311–8.
- Lachowicz JI, Nurchi VM, Crisponi G, et al. Metal coordination and tyrosinase inhibition studies with Kojic-βAla-Kojic. J Inorg Biochem 2015;151:36–43.
- Xie WL, Zhang HL, He JJ, et al. Synthesis and biological evaluation of novel hydroxybenzaldehyde-based kojic acid analogues as inhibitors of mushroom tyrosinase. Bioorg Med Chem Lett 2017;27:530–2.
- Asadzadeh A, Sirous H, Pourfarzam M, et al. In vitro and in silico studies of the inhibitory effects of some novel kojic acid derivatives on tyrosinase enzyme. Iran J Basic Med Sci 2016;19:132–44.