ABSTRACT
Delay the ripening can improve fruit shelf life. Reduced glutathione (GSH) is an antioxidant that delays the ripening of fruits, though the GSH-mediated mechanism involved in fruit-ripening processes is currently unclear. This study used RNA sequencing to assess the GSH-induced transcriptional and biochemical alterations observed in tomato fruit during the post-harvest process. We found 970 differentially expressed genes (DEGs) after GSH treatment, and 124 were found to be candidate genes related to the ripening of GSH-mediated fruit. In addition, the expression levels of several candidate DEGs observed in ripe tomato fruit after GSH treatments were confirmed using quantitative real-time PCR. Biochemical analyses revealed that the GSH treatment decreased the proline content and the lipid peroxidation and ascorbate peroxidase activity levels. In contrast, it increased the superoxide dismutase, peroxidase, and catalase activity levels, as well as endogenous glutathione and ascorbic acid contents. These results confirm the important role played by GSH during the process of ripening tomato fruit.
1. Introduction
Glutathione (GSH), a thiol compound containing cysteine residues, functions in alleviating antioxidant stress-related toxicity, improving immunity, transporting and storing reduced sulfur, and maintaining the cell redox balance (Gill et al. Citation2013; Uys et al. Citation2014; Ge et al. Citation2019; Yao et al. Citation2021). Whereas GSH has roles in alleviating abiotic stresses, such as high salt (Zhou et al. Citation2019), high temperature (Nahar et al. Citation2015), and toxic metals (Anjum et al. Citation2012), recent studies have found it is also related to the regulation of genes. RNA sequencing (RNA-seq) can reveal GSH-responsive genes in various systems subjected to various physiological and stress environments (Hacham et al. Citation2014).
Tomato (Solanum lycopersicum L.) is an important economic and agricultural crop around the globe. Fruit ripening is an important process that makes the fruit appear attractive, which increases seed liberation and dispersal. Therefore, it is a genetic and closely coordinated process that can change flavor, appearance, smell, and texture (Srivastava et al. Citation2010). Fruit ripening is an oxidative phenomenon that involves alterations in the redox homeostasis of reactive oxygen species (ROS), including superoxide anion (O2.−), hydroxyl radical (OH−), and hydrogen peroxide (H2O2) (Huan et al. Citation2016). Recent biochemical findings have found that tomato fruits have a sulfhydryl-oxidative metabolism due to changes in key reactive ROS parts when the plant ripens from green to red (Morscher et al. Citation2015). Antioxidant enzymes, including, catalase (CAT), superoxide dismutase (SOD), peroxidase (POD), and ascorbate peroxidase (APX), and non-enzymatic antioxidants, such as ascorbic acid (AsA) and GSH, are involved in ROS scavenging (Yao et al. Citation2021). Dehydrogenases, including malic enzyme (ME), 6-phosphogluconate dehydrogenase (6PGDH), glucose-6-phosphate dehydrogenase (G6PD), and isocitrate dehydrogenase (ICDH), produce the NADPH needed for the AsA-GSH cycle, which is important in ROS scavenging (Palma et al. Citation2020). In addition, plant hormones, such as ethylene, play important roles during fruit ripening (Zhu et al. Citation2017). GSH increases ethylene content via transcriptional and posttranscriptional regulating of the key enzymes related to ethylene biosynthesis, including 1-aminocyclopropane-1-carboxylate oxidase (ACO) and 1-aminocyclopropane-1-carboxylate synthase (ACS) (Datta et al. Citation2015). However, molecular information on GSH during the ripening of tomato fruits, particularly when they change color from green to red, is limited.
To study the effects of GSH on the green to red transition during fruit ripening, exogenous GSH has been applied previously. In strawberry, GSH applications decrease the active oxygen content, increase the endogenous antioxidant contents, and improve the antioxidant capacity, maintaining the fruit’s redox balance (Ge et al. Citation2019). Exogenous GSH treatments alleviate chilling injury when peppers are in cold storage by starting the AsA-GSH cycle and increasing antioxidant capacity (Yao et al. Citation2021). Other possible regulators, including abscisic acid (ABA), hydrogen sulfide, and nitric oxide, have been used for fruit ripening (Wang et al. Citation2013; Muñoz-Vargas et al. Citation2018; Luo et al. Citation2020). Research assessing how particular antioxidants affect the ripening process is still scant. In this study, the transcriptome profiles of ripening tomatoes that were identified using high-throughput RNA-seq to analyze the GSH mechanisms involved in ripening. Candidate genes involved in GSH-mediated tomato fruit ripening are related to the following biological processes: cell wall metabolism and modification, ripening-associated transcription factors, plant hormone metabolism and response, and antioxidant systems. These findings lay the groundwork for additional study of postharvest changes in color during tomato fruit ripening due to GSH.
2. Materials and methods
2.1. Plant material
The tomato (Solanum lycopersicum Mill. cv. MicroTom) plants were grown in an incubator under the following conditions: 16-h day (25°C) and 8-h night (17°C) at 70% relative humidity. Fruit from 80 different plants was harvested at the green immature stage. These fruits were all the same shape, size, and color.
Reduced GSH was purchased from Sigma (Chemical Co.St.Louis, MO, USA) and used as a tomato green immature stage treatment. Briefly, 150 fruit were immersed in an aqueous GSH solution (5 mM) in a box for 30 min, and 150 fruit were immersed in distilled water as the control. They were then stored at 25°C for 21 days (). The concentration of GSH was selected according to the results of preliminary experiments (Table S1; Fig.S1). Three replicates for each treatment. Afterward, the samples were frozen in liquid nitrogen and maintained at –80°C until analyzed.
Figure 1. Visual phenotype of tomato fruits at different stages and treatments: mature green (Green), turning-color period without GSH treatment (TP-GSH), turning-color period with GSH treatment (TP+GSH), mature period without GSH treatment (TP-GSH), and mature period with GSH treatment (TP+GSH). Fruits were subjected to a glutathione aqueous solution (5 mM) in a box for 30 min and were then stored at 25°C temperature for 21 d.
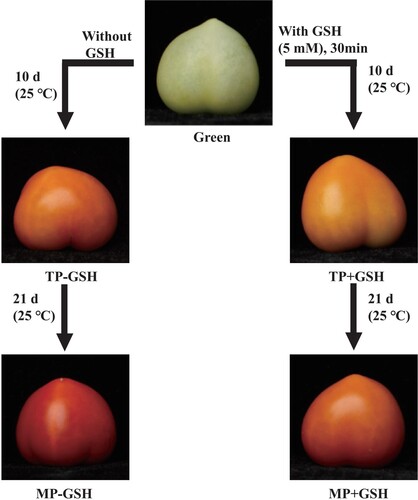
2.2. RNA extraction, library construction, and sequencing
According to the manufacturer’s instructions, a TRIzol reagent kit (Invitrogen, Carlsbad, CA, USA) was used to extract total RNA. An Agilent 2100 Bioanalyzer (Agilent Technologies, Palo Alto, CA, USA) was used to analyze the quality of the RNA, which was then confirmed with RNase-free agarose gel electrophoresis. Following extraction, Oligo (dT) beads were used to enrich eukaryotic mRNA, while a Ribo-ZeroTM Magnetic Kit (Epicenter, Madison, WI, USA) was used to remove rRNA and enrich prokaryotic mRNA. A fragmentation buffer was used to fragment the enriched mRNA, which was reverse transcribed, using random primers, into cDNA. DNA polymerase I, RNase H, and dNTPs were used in a buffer to synthesize second-strand cDNAs, after which cDNA fragments were purified with a QiaQuick PCR extraction kit (Qiagen, Venlo, The Netherlands). They were then end-repaired, poly(A) was added, and they were ligated to Illumina sequencing adapters. The resulting ligation products were chosen using agarose gel electrophoresis based on size, PCR amplified and sequenced by Gene De novo Biotechnology Co. (Guangzhou, China) using an Illumina HiSeq2500 system.
2.3. Sequence pre-processing and assembly
The reads produced by the sequencing system were raw reads with adapters or low-quality bases affecting their assembly and subsequent analyses. Thus, reads were further filtered using fastq software (version 0.18.0) to maintain high-quality clean reads (Chen et al. Citation2018) according to the following: 1) remove reads with adapters; 2) remove reads with >10% unknown nucleotides (N); and 3) remove reads with >50% low quality (Q-value ≤ 20) bases. A reference genome index was constructed, while HISAT2. 2.4 (Kim et al. Citation2015) was used to map paired-end clean reads to the reference genome; ‘-rna-strandness RF’ and other parameters were set to default. StringTie v1.3.1 (Pertea et al. Citation2015; Pertea et al. Citation2016) was used to assemble mapped reads from each sample using a reference-based approach. A fragment per kilobase of transcript per million mapped reads value was produced using StringTie software for each transcriptional location to analyze the variation and abundance of expression. The raw reads generated in this study were deposited in the NCBI database under accession number PRJNA787978.
2.4. Mapping and expression analysis
DESeq2 software was used to perform gene differential expression analyses between two groups, while edgeR was used for two samples (Love et al. Citation2014). In this study, genes and transcripts with absolute fold change ≥ 2 and false discovery rate (FDR) < 0.05 were differentially expressed genes (DEGs)/transcripts. GO terms that were significantly enriched in DEGs were identified using a gene ontology (GO) enrichment analysis, which was then classified by biological function (Ashburner et al. Citation2000). Each DEG was mapped to a GO term in the Gene Ontology database (http://www.geneontology.org/), while gene numbers were generated for each term. A hypergeometric test was used to identify GO terms significantly enriched in DEGs compared to genomic background. FDR correction was applied to the resulting p-values, with FDR ≤ 0.05 as the cutoff. Metabolic pathways or signal transduction pathways with significantly enriched DEGs compared to the whole genome were found using a KEGG pathway enrichment analysis (Kanehisa Citation2000). FDR correction was applied to the resulting p-values, with FDR ≤ 0.05 as the cutoff. Pathways that met all of these criteria were considered significantly enriched with DEGs.
To determine putative target genes for the ripening-associated TFs, promoter sequences for tomato were obtained by extracting a genomic sequence of 1000 bp upstream from the transcription start site (TSS) of each gene. PWMs for each TF were then used to scan promoter sequences using the Find Individual Motif Occurrences (FIMO) tool from the MEME Suite (v 4.11.2) (Olivares-Yañez et al. Citation2021), employing default parameters (p-value < 1×10e-4). The co-expression network analysis of ripening-associated TFs were differentially expressed in ripening tomato fruit using Cytoscape software.
2.5. DEG validation by a quantitative real-time PCR (qRT-PCR) analysis
To validate the transcriptomic results, 16 DEGs were detected in immature green fruit (Green) vs mature-period fruit without the GSH treatment (MP-GSH), Green vs mature-period fruit with the GSH treatment (MP + GSH), and MP-GSH vs MP + GSH were selected for a qRT-PCR analysis that used similar plant materials as in the RNA-seq. Genes were randomly selected based on their functions identified by this study and their high fold changes. Three biological replicates were performed for each sample, while three technical replicates were performed for each gene. A PrimeScript® II First-Strand cDNA Synthesis Kit (TaKaRa, Japan) was used to synthesize cDNA. Primer Premier 5.0 was used to design specific primers, while the length of the resulting amplified PCR products varied from 100 to 245 bp (Table S2). A CFX96 Touch Real-Time PCR Detection System (Bio-Rad, USA) was used to conduct qRT-PCR, with SYBR Premix Ex Taq™ II (TaKaRa). Relative gene expression levels in various samples were assessed using the 2−ΔΔCt method. Relative DEG expression levels were assessed using green fruit as controls. Figures were constructed with GraphPad Prism 8 (GraphPad Software Inc., San Diego, CA).
2.6. Determination of proline, malondialdehyde (MDA), GSH, and AsA contents and enzyme activity assays
MDA concentration was measured to analyze lipid peroxidation, which formed thiobarbituric acid-reactive substances (Buege and Aust Citation1978). Ninhydrin assay (Bates et al. Citation1973) measured proline, spectrophotometrically at 520 nm. The ferricytochrome method (Yim et al. Citation1996) was used to assay total SOD activity (EC 1.15.1.1). POD (EC 1.11.1.7) activity was assayed according to the methods used by Ranieri et al. (Ranieri et al. Citation2000), while CAT activity (EC 1.11.1.6) was assessed by quantifying H2O2 levels (Aebi Citation1984). APX (EC 1.11.1.11) activity was assessed by measuring decreases in A290 owing to ASC oxidation by H2O2 (Mano et al. Citation2001). The GSH and GSH/GSSG contents in the fruit were determined in accordance with the procedure of Yu et al. Citation2002. AsA content was measured following the procedure of Costa et al. Citation2002.
2.7. Statistical analyses
One-way analysis of variance (ANOVA) was performed on the data, expressed as post-hoc multiple comparisons tin SPSS version 21.0 (SPSS Inc., Chicago, IL, USA) to assess the data. Means were analyzed at a P < 0.05 significance level using Duncan’s new multiple range test. The numbers used in the tables and figures are means ± SE (N = 3).
3. Results
3.1. Transcriptome sequencing analysis
After assessing the quality and filtering the data, 61.37 gigabytes (Gb) were obtained from the samples. Each sample had an average of approximately 6.82 Gb of clean data. Of the nine libraries, the GC% of the sequenced data varied from 41.77% to 42.19%, while more than 92.57% of reads had an average quality score exceeding 30 (Table S3). Based on this, the sequencing data was sufficiently accurate and of high enough quality for subsequent analyses. Table S3 displays general sequencing statistics, while the mapping efficiency of none tomato genome samples varied from 96.27%–96.98%. Later analysis of expression profiles only used uniquely mapped reads. Analysis of the correlation coefficients of each pair of biological replicates demonstrated highly consistent gene expression levels in each replicate (Fig. S2a), while replicates demonstrated consistent levels of expression (Fig. S2b), However, different samples were differentially expressed.
3.2. Transcriptomic analysis after GSH treatment
Assessing differences in gene expression in the MP-GSH and MP + GSH tomato fruit groups is one way of analyzing the effects of GSH treatment. However, our study used a more comprehensive approach by considering the green immature stage a reference (). There were 8,316 DEGs (2,488 up-regulated and 5,828 down-regulated) identified in the Green vs. MP-GSH comparison. In the Green vs. MP + GSH comparison 8,210 DEGs (2,333 up-regulated and 5,877 down-regulated) were identified (a). Additionally, DEGs can be classified based on their various expression patterns (), which allowed us to analyze the data and identify helpful biological information (Khatri et al. Citation2012) (b).
Figure 2. Analysis of gene expression during ripening and modulated by GSH in tomato fruits. (a) Analysis of differentially expressed genes (DEGs) between tomato fruits at immature stage (Green), mature period in untreated fruits (MP-GSH), and mature period in untreated fruits (MP+GSH). (b) Venn diagrams combining DEGs (up- and down regulated) among tomato fruits at different stages of ripening and under GSH treatment.
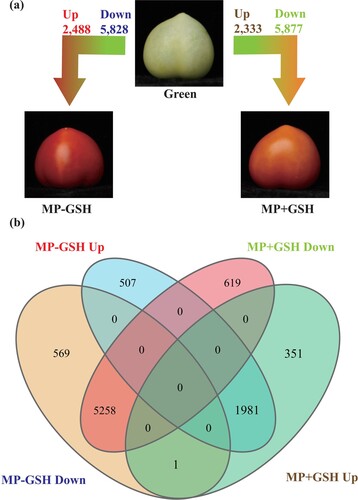
Table 1. Groups of genes modulated by GSH treatment observed in b.
3.3. Functions and processes influenced by the GSH treatment
GSH treatment effects can be difficult to identify based on genetic changes due to natural ripening. This varied with genes and groups. Genes that were up- or down-regulated genes in both comparisons (Green vs. MP-GSH and Green vs. MP + GSH) were considered to have expression not modulated by GSH. This was the case for 7,239 genes (1,981 up-regulated and 5,258 down-regulated) (b). Some genes were up-regulated even without GSH application, leading us to assess biological processes, such as responses to stimuli, including redox state and oxidative stress, cell wall metabolism, and plant hormone metabolism. Additionally, this study identified important biological processes such as fruit ripening, oxidation–reduction, regulation of auxin-mediated signaling, and positive transcriptional regulation (Table S5). Conversely, we identified different categories among the down-regulated genes, which included carbohydrate, polysaccharide and cellular glucan metabolic processes, photosynthesis, and cytoskeletal protein binding (Table S6).
The genes identified from the Green vs. MP-GSH comparison, 507 up-regulated and 569 down-regulated, in which GSH constrained overexpression and repression, respectively. The application of GSH hid the overexpression of genes related to the following processes: protein metabolism-related regulation, negative organ and developmental growth regulation, oxidoreductase activity, and peroxiredoxin activity (Table S7). Furthermore, GSH mitigated the suppression of genes related to protein phosphorylation, lipid storage, response to oxygen-containing compounds, and calcium ion binding (Table S8).
3.4. Genes specifically affected by the GSH treatment
The expression of up- and down-regulated genes only located in MP + GSH (351 and 619, respectively) are modulated by GSH. Enrichment analysis of the 351 genes up-regulated by applying GSH is shown in a. Closer analysis highlighted which genes relate to macromolecule modifications, such as phosphorylation, response to stimuli, such as kinase activity and WRKY proteins, responses to signal transduction, such as NADPH/NADP+ and GSH/GSSG, and regulation of defense responses to bacteria (Table S9).
Figure 3. Functional classification of tomato fruit genes specifically affected by GSH treatment. Genes were classified according to biological processes, molecular functions, and cellular component categories.(a) Genes up-regulated by GSH. (b) Genes down-regulated by GSH.
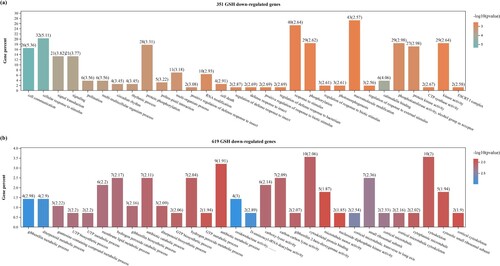
Additionally, this study categorized 619 down-regulated genes (b), including genes encoding proteins associated with biological processes such as metabolic processes, including gibberellin (GA), membrane lipid, and H2O2, as well as H2O2 catabolic processes. This study also identified molecular functions, such as binding and oxidoreductase activity (Table S10). Therefore, after 21 d, GSH application affected post-harvest storage more than gene expression patterns. This suggests that GSH regulates significant genetic alterations during tomatoes ‘ ripening process, which may occur in other crop fruit.
3.5. GSH treatment effects on genes involved in plant hormone metabolism and response, cell wall metabolism, antioxidant systems, and ripening-associated transcription factors (TFs)
GO and KEGG analyses demonstrated that most GSH-responsive DEGs were involved in plant hormone metabolism and response (a, Table S11). GSH treatments affected the expression levels of plant genes related to hormone metabolism and response (Table S11; S12). Approximately 29 genes were related to hormone metabolism and response, of which 11, 1, 1, and 16 genes were related to the ethylene signal, GA, ABA and auxin signal pathways, respectively (Table S12). This study identified a variety of regulators essential to signal transduction and ethylene synthesis, such as ERS1, an ethylene receptor neverripe (1), ETR2, an ethylene receptor (2), ERF3, an ethylene-responsive factor (1), LEA, an ethylene-responsive late embryogenesis-like protein (1), ACO, an ACC oxidase (3), and ACS, an ACC synthase (3) (a). The expression levels of ERS1, ETR2, and ERF3, as well as ACS1/4, were significantly repressed after the GSH treatment, whereas LEA14-A, ACO1/4, and ACS2 were dramatically increased after the GSH treatment (a). One GA-mediated signaling pathway gene was down-regulated and one salicylic acid (SA) synthesis gene was up-regulated after the GSH treatment. In total, 10 auxin response and transport genes were up-regulated after the GSH treatment, while auxin-responsive genes, including auxin response factors, PINs, auxin efflux carrier components, and IAA4, an auxin-responsive protein, were up-regulated following GSH application. The auxin-responsive protein SAUR76, auxin repressed/dormancy-associated protein DRMH1, auxin-responsive protein IAA27, and auxin-binding protein 1 T92 were down-regulated after the GSH treatment.
Figure 4. Differentially expressed genes (DEGs) with the annotated functions in plant hormone metabolism and response (a) and cell wall metabolism and modification (b) at different stages of ripening and under GSH treatment.
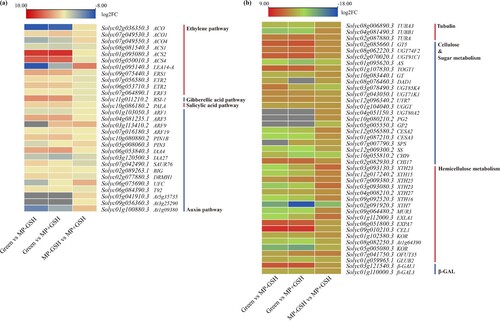
In total, 42 DEGs were involved in cell wall metabolism (b), including tubulin alpha/beta chains (3), glycosyltransferases (GTs) (9), polygalacturonases (2), cellulose synthesis (3), xyloglucan endotransglucosylase/hydrolases (XTHs) (7), expansins (2), O-fucosyltransferase 35 (1), sucrose-phosphate synthase 1 (1), UDP-glucose metabolism-related genes UTR7/UGGT/UGT80A2 (3), beta-galactosidases (2), glucan endo-1,3-beta-glucosidase B (1), endochitinases CHI9/17 (2), endoglucanases CEL1/KOR/At1g64390 (4), and sucrose synthase (1). Several of these genes were repressed after the GSH treatments during the ripening process, while most were significantly elevated. The XTH23 and EXLA1 genes were up-regulated, whereas the AS, UGT85K4, GP2, XTH23, and CHI9 genes were greatly down-regulated after the GSH treatments during fruit ripening.
Many of the DEGs were involved in the antioxidant systems, while most unigenes were up-regulated, including those encoding glutathione-S-transferases (GSTs) (3), glutathione peroxidase (1), POD (3), dehydroascorbate reductase 1 (DHAR1) (1), glucose-6-phosphate 1-epimerase/G6PD2 (2), ICDH-1 (1), an ME (MODA) (1), and 6PGDH_C (1), after the GSH treatment during fruit ripening. GST (8), gamma-glutamyltranspeptidase (1), SODCP.2 (1), thioredoxin peroxidase 1 (1), POD (5), ascorbate peroxidase (APX1) (2), and DHAR1 (1) were down-regulated after the GSH treatment during fruit ripening (a).
Figure 5. Differentially expressed genes (DEGs) with the annotated functions in antioxidant systems (a) and ripening-associated transcription factors (b) at different stages of ripening and under GSH treatment.
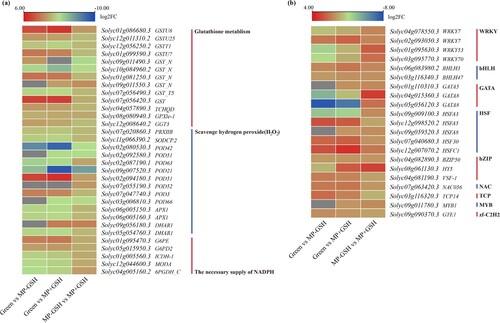
Notably, several TFs were associated with fruit senescence, and most TFs were up-regulated after the GSH treatment during fruit ripening (b). Four WRKYs (two WRKY7, WRKY53, and WRKY70), two BHLHs (BHLH3 and BHLH47), three GATAs (GATA5, GATA8, and GATA8), four HSFs (HSFA3, HSFA8, HSF30, and HSFC1), two bZIPs (bZIP50 and HY5), NAC056, and MYB1, were demonstrated to be positive regulators after the GSH treatment during fruit ripening. Conversely, HSFA5, TCP14, VSF-1, and GTE1 TFs were demonstrated to be negative regulators after the GSH treatment during fruit ripening. To further investigate how transcription factors were involved in regulating tomato postharvest ripening. Co-expression analysis showed that MYB1 was hub gene (Figure S3). Predicting the binding site of MYB1 showed that 187 genes belong to AP2/ERF family (Table S13).
3.6. GSH modulates the content of proline, GSH, and AsA contents, lipid peroxidation level, and antioxidant enzyme activity level during post-harvest ripening
As shown in a, the proline content gradually increased at first and then decreased during the various fruit ripening stages assessed. The highest 2.20-fold increase happened in turning-color-period (TP) fruit, compared to green fruit. Importantly, MP + GSH and MP-GSH fruit each had proline contents like that of green tomato. However, the contents decreased in the GSH-treated fruit compared to the control, though this difference was not statistically significant.
Figure 6. a) Proline content, (b) lipid peroxidation level, (c) SOD activity, (d) POD activity, (e) CAT activity, (f) ascorbate peroxidase (APX) activity, (g) reduced glutathione (GSH) content, (h) GSH/GSSG, (i) AsA contents of tomato fruits at different stages of ripening: immature green (Green), turning-color period with and without GSH treatment (TP+GSH and TP-GSH, respectively), and mature period with and without GSH treatment (MP+GSH and MP-GSH, respectively). Pairwise analysis of variance was used to detect differences in comparison to green fruits. *P<0.05, **P<0.01, ***P<0.001.
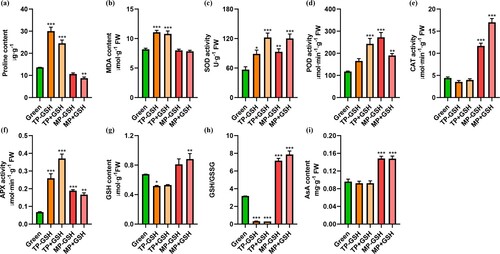
MDA content slowly increased during stages of TP ripening; in yellow fruit, it increased 1.35- fold compared to green fruit (b). Again, MP + GSH and MP-GSH fruit showed similar MDA contents, which were lower in the former than the latter. However, these differences were statistically not significant. MP + GSH and MP-GSH fruit both showed MDA contents similar to that of green fruit. These results confirmed that ripening is related to oxidative stress and that the application of GSH can decrease MDA content.
As shown in c, the SOD activity level gradually increased as ripening proceeded, and MP + GSH fruit had slightly higher values than MP-GSH fruit. The POD activity gradually increased as ripening proceeded in MP-GSH fruit. The POD activity was significantly lower in MP + GSH fruit compared with MP-GSH fruit, but the difference was not statistically significant (d). The CAT activity was significantly increased in MP compared with green fruit (e), and there was a 1.46-fold increase in MP + GSH fruit compared with MP-GSH fruit.
As shown in f, a maximum 5.49-fold increase in APX activity occurred in yellow fruit compared with green fruit. Intermediate APX activity values occurred in both MP + GSH and MP-GSH fruit. The APX activity was 2.47-fold higher in MP + GSH fruit compared with green fruit. The values were slightly lower for MP + GSH fruit compared to the control, though these differences were not statistically significant.
As shown in g, during ripening, the GSH content decreased at first and then increased during ripening, experiencing a 1.31-fold decrease in yellow (TP period) fruit to the control. Independent of the GSH treatment, MP fruit had the maximum GSH contents, with MP + GSH fruit having a slightly higher value. GSH/GSSG proceeded according to a similar pattern to GSH, though values were 10.77-fold lower in yellow (TP period) fruit compared to the control (h). Independent of GSH treatment, the MP fruit, showed maximum AsA contents (i).
3.7. Validation of RNA-seq-based gene expression
The expression trends in the two platforms for the 16 genes assessed using qRT-PCR and RNA-seq () were similar, suggesting that the expression profiles of genes in the RNA-seq assay were similar to the results obtained by the qRT-PCR analysis (). Among the six genes involved in the plant hormone metabolism and response pathway, the increased expression levels of ACO1, ACS2, and IAA4 after the GSH treatment were positively correlated with fruit ripening. GSH treatments severely repressed ACS4, ERS1 and DRMH1 expression. Among the four genes related to cell wall metabolism, TUBA3 expression decreased but the levels of UGT91C1, TOGT1, and β-GAL1 increased after GSH treatments, indicating that these genes play important parts during the fruit ripening process. Among the five genes involved in antioxidant systems, GSTU7, thioredoxin peroxidase IIB (PRXIIB), POD42, and APX1 decreased and glutathione peroxidase le-1 (GRXle-1) increased after the GSH treatment. The expression of the ripening-related TF NAC056 was positively correlated with fruit ripening after the GSH treatment.
4. Discussion
Plant antioxidants are essential for fruit ripening, while GSH is a plant antioxidant with an important role: delaying fruit ripening (Giovinazzo et al. Citation2004; Camejo et al. Citation2010; Palma et al. Citation2020). Therefore, a comprehensive understanding of the fruit post-harvest GSH-regulated ripening mechanism is both of scientific and economic importance. This study used RNA-seq to assess transcriptional and biochemical changes induced by GSH in post-harvest tomato fruit after a GSH treatment. The expression of 970 DEGs was exclusively modulated by GSH (; Tables S9 and S10). 124 GSH-specific DEGs were involved in plant hormone metabolism and response, cell wall metabolism, antioxidant systems, and ripening-associated TFs ( and ; Table S12). qRT-PCR was used to confirm the expression levels of 16 candidate DEGs related to these pathways (). In addition, GSH modulated the proline, glutathione, and ascorbic acid contents, lipid peroxidation level, and antioxidant enzyme activity level during ripening (). These findings indicate that delays in post-harvest ripening due to GSH could be due to the direct or indirect regulation of genes related to cell wall metabolism, plant hormone metabolism and response, antioxidant systems, and ripening-associated TFs.
Plant hormones and antioxidants interact in regulating plant growth, development, and fruit ripening (Bahin et al. Citation2011; Datta et al. Citation2015). In this study, the GSH-regulated DEGs related to ethylene, GA, SA, and auxin pathways (a; Table S12), indicating that these hormone-associated genes could regulate GSH-associated delay during the ripening of tomato fruits. Ethylene can trigger fruit ripening (Pech et al. Citation2012), which regulates fruit ripening by affecting ACS and ACO genes and fruit-specific polygalacturonase (Kou et al. Citation2021). GSH might be related to the ethylene biosynthesis pathway via positive modulation of ACO and ACS gene expression levels (Datta et al., Citation2015). However, our findings demonstrate that applying GSH to tomato fruit reduced the expression levels of the ethylene biosynthetic genes ACS1/4, whereas ACO/ACO1/4 and ACS2 were dramatically up-regulated. These results suggest that these three homologous ACO genes, as well as the ACS gene, play essential but opposing roles in GSH response during tomato ripening, which could lower ethylene content following the application of GSH. We also found that three ethylene signaling genes, ERS1, ETR2, and ERF3, were down-regulated by the GSH application. At the receptor, ethylene action interference significantly represses the expression levels of several genes related to the ethylene signal pathway, including ERF, ETR, and ERS (Tadiello et al. Citation2016). In sweet cherry fruit, GAs is present during the onset of ripening, and exogenous GA3 applications delay ripening (Kuhn et al. Citation2020). Here, the gene encoding the key enzyme (RSI-1) involved in the GA-mediated signaling pathway was down-regulated, indicating that exogenous GSH treatment may decrease the GA level to delay tomato fruit ripening. The GSH treatment of tomatoes also changed levels of GSH-related gene expression, including PALA. PAL is involved in the phenylpropanoid pathway of SA synthesis, and SA can inhibit ethylene production in pear, thereby delaying fruit ripening (Chen et al. Citation2009; Shi et al. Citation2019). Auxin stimulates early receptacle expansion but inhibits later ripening, and involved in fruit development and inhibition of ripening (Iqbal et al. Citation2017; Guo et al. Citation2018). In a previous study, 12 auxin pathway genes were up-regulated by a GSH treatment, which suggested that exogenous GSH treatments may increase the auxin level to delay tomato fruit ripening.
Cell wall degradation is related to fruit quality and is differentially regulated during the development and ripening stages. At least 50 genes related to cell wall structure are expressed during tomato fruit development, which encode cellulose, hemi-cellulose, and pectins (The Tomato Genome Consortium Citation2012; Uluisik and Seymour Citation2020). Major transcriptomic changes were observed in DEGs related to cell wall metabolism when tomato fruit was treated with GSH treatment (b; Table S12). In total, this study identified 42 DEGs related to cellulose synthesis, hemicellulose metabolism, cell wall metabolism, and sucrose metabolism, and 22 genes significantly increased during fruit ripening. The expression levels of GTs, which play important roles during the polysaccharide synthetic process, decrease as fruit maturation progresses (Keegstra and Raikhel Citation2001). GT expression levels in ripening apples are lower and more limited compared with those of genes involved in cell wall disassembly (Zhu et al. Citation2012). Similar to ripening delays in tomatoes following GSH application, there were significant increases in unigenes annotated as GTs in tomatoes treated with GSH compared to the control. Genes involved in hemicellulose showed expression profiles similar to those of genes involved in cellulose and sugar metabolism. Some genes, including five XTHs, EXLA1, O-fucosyltransferase 35, MUR3 and a KOR gene encoding hemicelluloses and β-GAL1, which decreased during fruit ripening, were induced by the GSH treatments. In particular, expression of XTH23 dramatically increased after the GSH treatments, but showed a negative fold-change without the GSH treatment. Xyloglucan plays an important role in fruit softening, and it is expressed during fruit ripening (Keegstra and Raikhel Citation2001). These results suggest that GSH delays the ripening and decay of tomato plants by promoting cell wall assembly and preventing cell wall disassembly.
An increase in the proline content of fruit, including tomato, has been associated with various physiological processes, including senescence and ripening, indicating that it can be a proxy of fruit quality (Claussen et al. Citation2006). These results confirm that proline accumulates during the ripening stage and suggests that GSH is responsible for the negative regulation of proline biosynthesis. Our previous study suggested GSH decreases the proline content under salt-stress conditions to alleviate oxidative stress (Zhou et al. Citation2017). An increase in the MDA content indicates that lipid peroxidation increased and that plant cells were subjected to oxidative stress (Chou et al. Citation2012). Our findings confirmed that ripening is related to oxidative stress and that applying GSH can decrease the MDA content. Lipid peroxidation increases have been observed during the ripening process, which is related to decreased antioxidant enzyme activities (López-Huertas and Palma Citation2020). All these data agree with GSH-treated tomato fruit having significant increases in SOD, POD, and CAT activity levels, as well as POD51/52/63 expression, which supports the scavenging of excess H2O2 (Jimenez et al. Citation2002; López-Huertas and Palma Citation2020). Findings in various plant species subjected to a various environmental stressors demonstrate that exogenous GSH treatments stimulate the different antioxidant enzyme activities (Qiu et al. Citation2013; Nemat Alla and Hassan Citation2014; Zhou et al. Citation2019). APX is an important component of the antioxidant system and takes electrons from ascorbate to convert H2O2 into H2O and O2 (Verma et al. Citation2021). APX and DHAR are important parts of the AsA-GSH cycle, which is important for recycling both components in cells (Verma et al. Citation2021). G6PD, 6PGDH, ICDH, and ME produce the NADPH needed for the AsA-GSH cycle (López-Huertas and Palma Citation2020). In our study, APX activity and its encoding genes decreased, and the expression levels of DHAR1, glucose-6-phosphate 1-epimerase, G6PD2, ICDH-1, MODA, and 6PGDH_C genes were increased by the GSH treatment. AsA is a low-molecular weight antioxidant and the first electron donor in the AsA-GSH cycle, which is related to direct ROS scavenging (Palma et al. Citation2020). Reduced glutathione is the main cellular antioxidant and part of the AsA-GSH cycle, and it is oxidized to GSSG by ROS to maintain the redox balance of cells (López-Vidal et al. Citation2016). Our study found that the AsA and GSH contents and the GSH/GSSG level increased significantly in the GSH-treated tomato compared with the untreated tomato. Seven GSTs were down-regulated in GSH-treated tomato fruit, which maintain high GSH levels (Zhang et al. Citation2020). These results indicated that GSH delays fruit ripening by regulating the ROS level and redox signals.
Many TFs regulate fruit-ripening processes (Onik et al. Citation2018). After the GSH treatment, 21 ripening-associated TFs were differentially expressed in ripening tomato fruit. The expression levels of WRKY7/53/70, GATA8, HSFA3, bZIP50, and HY5 genes increased after the GSH treatments and decreased in the untreated fruit. WRKY53 regulates the expression of senescence-associated genes and acts at an upstream position in the WRKY-signaling cascade (Zentgraf et al. Citation2010). SA delays pear fruit senescence by up-regulating the WYKY70 expression level (Changwal et al. Citation2021). The expression levels of HSFA5, TCP14, VSF-1, and GTE-1 genes decreased after the GSH treatments and increased in the untreated fruit. The tomato bZIP gene, as corroborated in this work, is highly expressed during ripening (Lovisetto et al. Citation2013). The FvTCP9 gene is transiently expressed in cultivated strawberries (Fragaria × vesca) and demonstrated that FvTCP9 overexpression promotes the ripening process in fruit (Xie et al. Citation2020). However, how TCP14 affects fruit ripening remains unclear. This study suggests that GSH delays tomato fruit ripening by up-regulating WRKY7/53/70, GATA8, HSFA3, bZIP50, and HY5 and down-regulating HSFA5, TCP14, VSF-1, and GTE-1. In apple, MdMYB1 is transcriptionally regulated MdERF3, a key regulator of ethylene biosynthesis, and involved in anthocyanin biosynthesis during fruit ripening (Gao et al. Citation2020). In our study, most AP2/ERF genes were predicted to have MYB1 binging sites. This indicates that GSH treatment may regulate tomato fruit ripening through MYB1 binding to ethylene-related transcription factors.
5. Conclusions
RNA-seq was used to identify variations in the transcription and biochemical levels of tomato fruits treated with GSH and a control, which allowed us to assess how GSH affects ripening in tomato fruits after being harvested. There were 970 DEGs that were significantly responsive to GSH, including 619 down-regulated genes and 351 up-regulated genes. Bioinformatics analyses demonstrated that GSH could delay the ripening of tomato fruits by regulating genes related to antioxidant systems, ripening-associated TFs, plant hormone metabolism and response, and cell wall metabolism. This study lays the groundwork for subsequent identification of genes related to the ripening of tomato fruits after they have been harvested, though the additional study of these candidate genes is needed to identify the GSH-mediated molecular mechanisms governing this complex process.
Disclosure statement
No potential conflict of interest was reported by the author(s).
Additional information
Funding
References
- Aebi H. 1984. [13] catalase in vitro. Methods Enzymol. 105:121–126.
- Anjum NA, Ahmad I, Mohmood I, Pacheco M, Duarte AC, Pereira E, Umar S, Ahmad A, Khan NA, Iqbal M, Prasad MNV. 2012. Modulation of glutathione and its related enzymes in plants’ responses to toxic metals and metalloids—A review. Environ Exp Bot. 75:307–334.
- Ashburner M, Ball CA, Blake JA, Botstein D, Butler H, Cherry JM, Davis AP, Dolinski K, Dwight SS, Eppig JT. 2000. Gene Ontology: tool for the unification of biology. Nat Genet. 25(1):25–29.
- Bahin E, Bailly C, Sotta B, Kranner I, Corbineau F, Leymarie J. 2011. Crosstalk between reactive oxygen species and hormonal signalling pathways regulates grain dormancy in barley: ROS and hormonal signalling in barley dormancy. plant. Cell Environ. 34(6):980–993.
- Bates LS, Waldren RP, Teare ID. 1973. Rapid determination of free proline for water-stress studies. Plant Soil. 39(1):205–207.
- Buege JA, Aust SD. 1978. [30] microsomal lipid peroxidation. Methods Enzymol. 52:302–310.
- Camejo D, Martí MC, Román P, Ortiz A, Jiménez A. 2010. Antioxidant system and protein pattern in peach fruits at two maturation stages. J Agric Food Chem. 58:11140–11147.
- Changwal C, Shukla T, Hussain Z, Singh N, Kar A, Singh VP, Abdin MZ, Arora A. 2021. Regulation of postharvest tomato fruit ripening by endogenous salicylic acid. Front Plant Sci. 12:663943.
- Chen S, Zhou Y, Chen Y, Gu J. 2018. . fastp: an ultra-fast all-in-one FASTQ preprocessor. Bioinformatics. 34(17):884–890.
- Chen Z, Zheng Z, Huang J, Lai Z, Fan B. 2009. Biosynthesis of salicylic acid in plants. Plant Signal Behav. 4(6):493–496.
- Chou TS, Chao YY, Kao CH. 2012. Involvement of hydrogen peroxide in heat shock- and cadmium-induced expression of ascorbate peroxidase and glutathione reductase in leaves of rice seedlings. J Plant Physiol. 169(5):478–486.
- Claussen W, Brückner B, Krumbein A, Lenz F. 2006. Long-term response of tomato plants to changing nutrient concentration in the root environment—the role of proline as an indicator of sensory fruit quality. Plant Sci. 171(3):323–331.
- Costa H, Gallego SM, Tomaro ML. 2002. Effect of UV-B radiation on antioxidant defense system in sunflower cotyledons. Plant Sci. 162(6):939–945.
- Datta R, Kumar D, Sultana A, Hazra S, Bhattacharyya D, Chattopadhyay S. 2015. Glutathione regulates ACC synthase transcription via WRKY33 and ACC oxidase by modulating mRNA stability to induce ethylene synthesis during stress. Plant Physiol. 169(4):01543.
- Gao J, Zhang Y, Li Z, Liu M. 2020. Role of ethylene response factors (ERFs) in fruit ripening. Food Qual Saf. 4(1):15–20.
- Ge C, Luo Y, Mo F, Xiao YH, Li NY, Tang HR. 2019. Effects of glutathione on the ripening quality of strawberry fruits. AIP Conf Proc. 2079:020013.
- Gill SS, Anjum NA, Hasanuzzaman M, Gill R, Trivedi DK, Ahmad I, Pereira E, Tuteja N. 2013. Glutathione and glutathione reductase: A boon in disguise for plant abiotic stress defense operations. Plant Physiol Biochem. 70:204–212.
- Giovinazzo G, D’Amico L, Paradiso A, Bollini R, Sparvoli F, DeGara L. 2004. Antioxidant metabolite profiles in tomato fruit constitutively expressing the grapevine stilbene synthase gene: antioxidant levels in tomato synthesizing resveratrol. Plant Biotechnol J. 3(1):57–69.
- Guo J, Wang S, Yu X, Dong R, Li Y, Mei X, Shen Y. 2018. Polyamines regulate strawberry fruit ripening by abscisic acid, auxin, and ethylene. Plant Physiololy. 177:339–351.
- Hacham Y, Koussevitzky S, Kirma M, Amir R. 2014. Glutathione application affects the transcript profile of genes in Arabidopsis seedling. J Plant Physiol. 171(15):1444–1451.
- Huan C, Jiang L, An X, Kang R, Yu M, Ma R, Yu Z. 2016. Potential role of glutathione peroxidase gene family in peach fruit ripening under combined postharvest treatment with heat and 1-MCP. Postharvest Biol Technol. 111:175–184.
- Iqbal N, Khan NA, Ferrante A, Trivellini A, Francini A, Khan MIR. 2017. Ethylene role in plant growth, development and senescence: interaction with other phytohormones. Front Plant Sci. 8:475.
- Jimenez A, Creissen G, Kular B, Firmin J, Robinson S, Verhoeyen M, Mullineaux P. 2002. Changes in oxidative processes and components of the antioxidant system during tomato fruit ripening. Planta. 214(5):751–758.
- Kanehisa M. 2000. KEGG: kyoto encyclopedia of genes and genomes. Nucleic Acids Res. 28(1):27–30.
- Keegstra K, Raikhel N. 2001. Plant glycosyltransferases. Curr Opin Plant Biol. 4(3):219–224.
- Khatri P, Sirota M, Butte AJ. 2012. Ten years of pathway analysis: current approaches and outstanding challenges. PLoS Comput Biol. 8:e1002375.
- Kim D, Langmead B, Salzberg SL. 2015. HISAT: a fast spliced aligner with low memory requirements. Nat Methods. 12(4):357–360.
- Kou X, Feng Y, Yuan S, Zhao X, Wu C, Wang C, Xue Z. 2021. Different regulatory mechanisms of plant hormones in the ripening of climacteric and non-climacteric fruits: a review. Plant Mol Biol. 107(6):477–497.
- Kuhn N, Ponce C, Arellano M, Time A, Sagredo B, Donoso JM, Meisel LA. 2020. Gibberellic acid modifies the transcript abundance of ABA pathway orthologs and modulates sweet cherry (prunus avium) fruit ripening in early- and mid-season varieties. Plants. 9:1796.
- Love MI, Huber W, Anders S. 2014. Moderated estimation of fold change and dispersion for RNA-seq data with DESeq2. Genome Biol. 15(12):550.
- Lovisetto A, Guzzo F, Tadiello A, Confortin E, Pavanello A, Botton A, Casadoro G. 2013. Characterization of a bZIP gene highly expressed during ripening of the peach fruit. Plant Physiol Biochem. 70:462–470.
- López-Huertas E, Palma JM. 2020. Changes in glutathione, ascorbate, and antioxidant enzymes during olive fruit ripening. J Agric Food Chem. 68:12221–12228.
- López-Vidal O, Camejo D, Rivera-Cabrera F, Konigsberg M, Villa-Hernández JM, Mendoza-Espinoza JA, Pérez-Flores LJ, Sevilla F, Jiménez A, de León-Sánchez F. D. 2016. Mitochondrial ascorbate–glutathione cycle and proteomic analysis of carbonylated proteins during tomato (Solanum lycopersicum) fruit ripening. Food Chem. 194:1064–1072.
- Luo Y, Ge C, Ling Y, Mo F, Yang M, Jiang L, Chen Q, Lin Y, Sun B, Zhang Y. 2020. ABA and sucrose co-regulate strawberry fruit ripening and show inhibition of glycolysis. Mol Genet Genomics. 295(2):421–438.
- Mano J, Ohno C, Domae Y, Asada K. 2001. Chloroplastic ascorbate peroxidase is the primary target of methylviologen-induced photooxidative stress in spinach leaves: its relevance to monodehydroascorbate radical detected with in vivo ESR. Biochimica et Biophysica Acta (BBA) - Bioenergetics. 1504(2–3):275–287.
- Morscher F, Kranner I, Arc E, Bailly C, Roach T. 2015. Glutathione redox state, tocochromanols, fatty acids, antioxidant enzymes and protein carbonylation in sunflower seed embryos associated with after-ripening and ageing. Ann Bot. 116(4):669–678.
- Muñoz-Vargas MA, González-Gordo S, Cañas A, López-Jaramillo J, Palma JM, Corpas FJ. 2018. Endogenous hydrogen sulfide (H2S) is up-regulated during sweet pepper (Capsicum annuum L.) fruit ripening. In vitro analysis shows that NADP-dependent isocitrate dehydrogenase (ICDH) activity is inhibited by H2S and NO. Nitric Oxide. 81:36–45.
- Nahar K, Hasanuzzaman M, Alam MM, Fujita M. 2015. Exogenous glutathione confers high temperature stress tolerance in mung bean (vigna radiata L.) by modulating antioxidant defense and methylglyoxal detoxification system. Environ Exp Bot. 112:44–54.
- Nemat Alla MM, Hassan NM. 2014. Alleviation of isoproturon toxicity to wheat by exogenous application of glutathione. Pestic Biochem Physiol. 112:56–62.
- Olivares-Yañez C, Sánchez E, Pérez-Lara G, Seguel A, Camejo PY, Larrondo LF, Vidal EA, Canessa P. 2021. A comprehensive transcription factor and DNA-binding motif resource for the construction of gene regulatory networks in botrytis cinerea and trichoderma atroviride. Comput Struct Biotechnol J. 19:6212–6228.
- Onik J, Hu X, Lin Q, Wang Z. 2018. Comparative transcriptomic profiling to understand pre- and post-ripening hormonal regulations and anthocyanin biosynthesis in early ripening apple fruit. Molecules. 23:1908.
- Palma JM, Terán F, Contreras-Ruiz A, Rodríguez-Ruiz M, Corpas FJ. 2020. Antioxidant profile of pepper (Capsicum annuum L.) fruits containing diverse levels of capsaicinoids. Antioxidants. 9:878.
- Pech JC, Purgatto E, Bouzayen M, Latché A. 2012. Ethylene and fruit ripening. Annual Plant Reviews. 44:275–304.
- Pertea M, Kim D, Pertea GM, Leek JT, Salzberg SL. 2016. Transcript-level expression analysis of RNA-seq experiments with HISAT, StringTie and ballgown. Nat Protoc. 11(9):1650–1667.
- Pertea M, Pertea GM, Antonescu CM, Chang TC, Mendell JT, Salzberg SL. 2015. Stringtie enables improved reconstruction of a transcriptome from RNA-seq reads. Nat Biotechnology. 33(3):290–295.
- Qiu B, Zeng F, Cai S, Wu X, Haider SI, Wu F, Zhang G. 2013. Alleviation of chromium toxicity in rice seedlings by applying exogenous glutathione. J Plant Physiol. 170(8):772–779.
- Ranieri A, Petacco F, Castagna A, Soldatini GF. 2000. Redox state and peroxidase system in sunflower plants exposed to ozone. Plant Sci. 159(1):159–167.
- Shi HY, Zhang YX, Chen L. 2019. Expression and regulation of PpEIN3b during fruit ripening and senescence via integrating SA, glucose, and ACC signaling in pear (pyrus pyrifolia nakai. whangkeumbae). Genes (Basel). 10(6):476.
- Srivastava A, Gupta AK, Datsenka T, Mattoo AK, Handa AK. 2010. Maturity and ripening-stage specific modulation of tomato (Solanum lycopersicum) fruit transcriptome. Gm Crops. 1(4):237–249.
- Tadiello A, Longhi S, Moretto M, Ferrarini A, Tononi P, Farneti B, Busatto N, Vrhovsek U, Molin AD, Avanzato C, Biasioli F. 2016. Interference with ethylene perception at receptor level sheds light on auxin and transcriptional circuits associated with the climacteric ripening of apple fruit (malus x domestica borkh. Plant J. 88(6):963–975.
- The Tomato Genome Consortium. 2012. The tomato genome sequence provides insights into fleshy fruit evolution. Nature. 485:635–641.
- Uluisik S, Seymour GB. 2020. Pectate lyases: their role in plants and importance in fruit ripening. Food Chem. 309:125559.
- Uys JD, Mulholland PJ, Townsend DM. 2014. Glutathione and redox signaling in substance abuse. Biomed Pharmacother. 68(6):799–807.
- Verma D, Upadhyay SK, Singh K. 2021. Characterization of APX and APX-R gene family in brassica juncea and B. rapa for tolerance against abiotic stresses. Plant Cell Rep. 41:571–592.
- Wang Y, Luo Z, Du R, Liu Y, Ying T, Mao L. 2013. Effect of nitric oxide on antioxidative response and proline metabolism in banana during cold storage. J Agric Food Chem. 61:8880–8887.
- Xie YG, Ma YY, Bi PP, Wei W, Liu J, Hu Y, Gou YJ, Zhu D, Wen YQ, Feng JY. 2020. Transcription factor FvTCP9 promotes strawberry fruit ripening by regulating the biosynthesis of abscisic acid and anthocyanins. Plant Physiol Biochem. 146:374–383.
- Yao M, Ge W, Zhou Q, Zhou X, Luo M, Zhao Y, Wei B, Ji S. 2021. Exogenous glutathione alleviates chilling injury in postharvest bell pepper by modulating the ascorbate-glutathione (AsA-GSH) cycle. Food Chem. 352:129458.
- Yim MB, Kang JH, Yim HS, Kwak HS, Chock PB, Stadtman ER. 1996. A gain-of-function of an amyotrophic lateral sclerosis-associated Cu, Zn-superoxide dismutase mutant: An enhancement of free radical formation due to a decrease in Km for hydrogen peroxide. Proc Natl Acad Sci USA. 93(12):5709–5714.
- Yu CW, Murphy TM, Sung WW, Lin CH. 2002. H2o2 treatment induces glutathione accumulation and chilling tolerance in mung bean. Funct Plant Biol. 29(9):1081.
- Zentgraf U, Laun T, Miao Y. 2010. The complex regulation of WRKY53 during leaf senescence of Arabidopsis thaliana. Eur J Cell Biol. 89:133–137.
- Zhang L, Kang J, Xie Q, Gong J, Shen H, Chen Y, Chen G, Hu Z. 2020. The basic helix-loop-helix transcription factor bHLH95 affects fruit ripening and multiple metabolisms in tomato. J Exp Bot. 71:6311–6327.
- Zhou Y, Diao M, Chen X, Cui J, Pang S, Li Y, Hou C, Liu H. 2019. Application of exogenous glutathione confers salinity stress tolerance in tomato seedlings by modulating ions homeostasis and polyamine metabolism. Sci Hortic. 250:45–58.
- Zhou Y, Wen Z, Zhang J, Chen X, Cui J, Xu W, Liu H. 2017. Exogenous glutathione alleviates salt-induced oxidative stress in tomato seedlings by regulating glutathione metabolism, redox status, and the antioxidant system. Sci Hortic. 220:90–101.
- Zhu Y, Zheng P, Varanasi V, Shin S, Main D, Curry E, Mattheis JP. 2012. Multiple plant hormones and cell wall metabolism regulate apple fruit maturation patterns and texture attributes. Tree Genet Genomes. 8(6):1389–1406.
- Zhu Z, Chen Y, Shi G, Zhang X. 2017. Selenium delays tomato fruit ripening by inhibiting ethylene biosynthesis and enhancing the antioxidant defense system. Food Chem. 219:179–184.