Abstract
Antimicrobial films were formulated with sodium caseinate (C), glycerol and antimicrobial substances (AM) produced by Streptococcus infantarius. The antilisterial activity of the films was evaluated using Listeria monocytogenes as test microorganism and two incubation conditions: T = 35°C, 32 ± 10% RH and T = 4°C, 98 ± 10% RH. A comparison of the antimicrobial action was made with caseinate films carrying equivalent activity units of commercial nisin (Nis). At 35°C and 32% RH, AM increased the lag-phase and inhibited 80% of the growth of L. monocytogenes. At 4°C and 98% RH, C-Nis and C-AM films extended the lag-phase of the bacterial growth up to 11 and 38 days, respectively. AM showed a higher antilisterial effect than nisin at both incubation conditions. The incorporation of AM or Nis did not produce significant differences in the barrier properties of the films, but AM acted as an additional plasticizer into the films.
Se formularon películas con caseinato de sodio (C), glicerol y sustancias antimicrobianas (AM) producidas por Streptococcus infantarius. La actividad antilisterial de las películas fue evaluada usando Listeria monocytogenes como microorganismo indicador y dos condiciones de incubación: T = 35°C, 32 ± 10% HR y T = 4°C, 98 ± 10% HR. Se comparó la actividad antimicrobiana con películas de caseinato que contenían nisina comercial (Nis) en unidades de actividad equivalentes. A 35°C y 32% HR, AM incrementó la fase lag e inhibió el 80% del crecimiento de L. monocytogenes. A 4°C y 98% HR, C-Nis y C-AM extendieron la fase lag del crecimiento bacteriano hasta 11 y 38 días, respectivamente. AM mostró mayor actividad antilisterial que nisina en ambas condiciones de incubación. La incorporación de AM o Nis no generó diferencias significativas en las propiedades de barrera de las películas, pero AM actuó como plastificante adicional en las películas.
1. Introduction
Antimicrobial packaging is a type of active packaging that aims at reducing, inhibiting or retarding microorganism growth that can contaminate packaged foods (Appendini & Hotchkiss, Citation2002). Edible films, based on proteins or polysaccharides, are effective carriers of antimicrobials. Some of the advantages of the use of antimicrobials into a biopolymer film include the slower diffusion of the antimicrobial through the film in such a way that it is available at a desired concentration for an extended period of time. Thus, smaller amounts of antimicrobial compounds would be needed to achieve a targeted shelf life compared with the direct addition of the antimicrobials on the food surface, where they quickly diffuse away from the surface, are rapidly diluted or react with food components (Guiga et al., Citation2009; Min & Krochta, Citation2008). Antimicrobial biopolymer films are emerging as a promising technology owing to the majority of solid or semi-solid foods being sensitive to the high microbial growth present on their surface (Santiago-Silva et al., Citation2009).
Recently, bacteriocins have been incorporated into packaging films based on biopolymers, as an attractive option to develop antimicrobial and biodegradable food packaging (Cao-Hoang, Chaine, Grégoire, & Waché, Citation2010; Cao-Hoang, Grégoire, Chaine, & Waché, Citation2010; Guiga et al., Citation2010; Imran, El-Fahmy, Revol-Junelles, & Desobry, Citation2010; Imran et al., Citation2012; Xu, Li, Kennedy, Xie, & Huang, Citation2007). The implementation of that kind of emerging technology has some advantages, which include its food-grade safety owing to the many bacteriocins being produced by food-grade lactic acid bacteria, the usual heat stability of the bacteriocins and the relatively high efficacies of the different bacteriocins against various primary pathogenic and spoilage microorganisms that can cause problems in minimally processed foodstuffs. Additionally, the films are elaborated from raw materials from crops or by-products from the food industry. Thus, antimicrobial biopolymer films based on bacteriocins can contribute to satisfying the growing consumer demand for fresh-like foods, minimally processed and free of chemical preservatives, and they can be used to contribute to decreasing the high amount of food-packaging plastic waste.
Bacteriocins are ribosomally synthesized antimicrobial peptides produced by one bacterium that are active against other bacteria, either in the same species (narrow spectrum) or across genera (broad spectrum) and as with host defence peptides (Cotter, Hill, & Ross, Citation2005). Nowadays, nisin and pediocin are the most used bacteriocins as additives for food conservation. It has been reported that nisin has a dual mode of action. It can bind to lipid II, the main transporter of peptidoglycan subunits from the cytoplasm to the cell wall, and therefore prevents correct cell wall synthesis, leading to cell death. Furthermore, it can use lipid II as a docking molecule to initiate a process of membrane insertion and pore formation that leads to rapid cell death. Nisin exhibits antimicrobial activity towards a wide range of Gram-positive bacteria, in particular against foodborne pathogens such as Listeria monocytogenes, Staphyloccocus aureus or Bacillus cereus (Cotter et al., Citation2005; Gálvez, Abriouel, López, & Omar, Citation2007). On the other hand, the class IIa pediocin-like or listeria-active bacteriocins are active (also in the nanomolar range) by inducing membrane permeabilization and the subsequent leakage of molecules from target bacteria (Cotter et al., Citation2005).
The purpose of this study was to investigate the antilisterial activity of sodium caseinate films containing a new bacteriocin produced by Streptococcus infantarius, which was isolated from pozol, an indigenous Mexican beverage. Based on our knowledge, little work has been carried out on the evaluation of the antibacterial activity of films during prolonged storage involving different conditions of temperature and relative humidity (RH).
2. Materials and methods
2.1. Production of antimicrobial activity-concentrated supernatant from S. infantarius
S. infantarius strain (LAB-ABMX) isolated from pozol, an indigenous Mexican beverage, was used as the bacteriocin producer strain. The selection of the strain was based on its antimicrobial activity against L. monocytogenes observed in previous studies (Mendoza-Mendoza et al., Citation2013). The LAB-ABMX strain was conserved at −80°C in blends of 20% glycerol and 80% LAB-ABMX-culture broth in BHI (Fluka), 24 h old at 35°C. LAB-ABMX was reactivated into 5 mL of MRS broth (Difco, France) and incubated at 30°C for 24 h. An inoculum (1% v/v) from that culture was transferred into MRS broth and grown at 30°C and 130 rpm for 6 h. Bacterial cells were removed by centrifuging at 6000 rpm for 30 min. The supernatant was collected; its pH was adjusted to 6.5 and then concentrated up to 40% of its initial volume in a Rotavapor® system (Büchi R-215, Labortechnik AG, Switzerland) operating at 60°C. To inactive proteases, the concentrated supernatant was heated at 110°C for 10 min; later, it was cooled and stored at −80°C in sterile Corning® 50 mL centrifuge tubes until use as antimicrobial agent (AM) in the bioactive films.
2.2. Nisin solution preparation
Nisin solution was prepared by dissolving 1 mg/mL of a commercial 2.5% nisin powder (MP Biomedicals) in sterile acetate buffer, pH = 5.5. This solution was stored and used to prepare the film-forming dispersions.
2.3. Antimicrobial activity test
Bacteriocin activity of AM and commercial nisin was assayed by the critical-dilution method (Nuñez, Tomillo, Gaya, & Medina, Citation1996). L. monocytogenes (CFQ-B-104, culture collection FQ-UNAM) was used as the indicator organism. Inhibitory activity was expressed as arbitrary units (AU). The samples were serially two-fold diluted in sterile deionized water. A drop (10 µL) of each dilution was placed on a soft agar plate, which was previously inoculated with 58 µL of a 24 h-old culture of L. monocytogenes in BHI agar at 35°C. Each plate was then incubated at 35°C for 24 h. The activity was expressed as AU, calculated considering the reciprocal of the highest two-fold dilution showing a definite zone of growth inhibition at 24 h of incubation.
2.4. Preparation of bioactive caseinate films
Films were prepared according to the protocol described previously (Mendoza-Mendoza et al., Citation2013). Film-forming solutions (FS) of 4% w/v sodium caseinate (Lactonat EN, LactoProt, Germany) were prepared by dissolving sodium caseinate in distilled water under continuous stirring. After that, glycerol was added as a plasticizer to obtain a final concentration of 0.6% w/v; then, previously defined volumes of AM or nisin solution were added to obtain a final concentration of bacteriocin of 93,000 AU/film (93,000 AU/20 mLFS). Each filmogenic solution was homogenized at 25,000 rpm for 10 min (Polytron-Aggregate Luzerrerstrasse); then they were subjected to a reduced pressure to eliminate air bubbles. After that, 20 mL of FS was taken and poured into levelled polystyrene petri dishes (90 mm diameter), which were then dried at 35 ± 2°C for 17 h in a convection oven (≈ 30% RH). Next, the films were peeled off from the petri dishes and kept in a closed cabinet at constant RH of 50 ± 5% at room temperature (23 ± 3°C). A control film of each treatment was formulated with all components of the film excluding the bacteriocins.
2.5. Antilisterial activity of the films
In this work, a bacteriocin activity (AM or nisin) of 93,000 AU was used to formulate the caseinate films, which was the best condition found previously by our group (Mendoza-Mendoza et al., Citation2013). The antimicrobial activity of control films and films containing bacteriocins was determined using L. monocytogenes (CFQ-B-104, culture collection FQ-UNAM) as the target microorganism. Briefly, a 50 μL sample was taken from a listeria conservation vial to be inoculated in 5 mL of BHI broth and incubated for 24 h at 35°C. Then, 50 µL of this culture broth was transferred into 5 mL of fresh BHI and incubated for 6 h at 35°C. Thereafter, decimal dilutions of this culture broth were carried out to take 600 µL of the proper dilution that were thoroughly spread onto the surface of Oxford agar plates (BD Difco, France), giving 60 CFU per Petri dish. Once the liquid was well absorbed into the agar, the surface was aseptically covered with a film. The plates were then incubated at: 1) T = 35ºC and 32 ± 10% HR or 2) T = 4ºC and 98 ± 10% HR. The growth of listeria colonies was monitored under a light microscope (Nikon Eclipse 80i) for 8 days for the first incubation condition and for 63 days for the last one. The used caseinate films containing nisin (C-Nis) and AM produced by S. infantarius (C-AM) were previously surface sterilized with UV radiation for 24 h (12 h on each side) in a safety cabinet (Purifier™ Delta™ Series Class II, Type A2 LABCONCO). All tests were run in duplicate.
2.6. Film thickness
Film thickness was measured using a digital micrometer (Truper, Mexico). Measurements were taken at 10 different locations of each film sample and the corresponding average values were used in the water vapour permeability, oxygen permeability and mechanical tests.
2.7. Colour evaluation
Colour measurements of the films were performed using a photocolorimeter (Minolta, Japan), which was calibrated with a standard white plate. Film disks of appropriate diameter were tested on white background standard. The colour CIE L* a* b* parameters were measured. L* value signifies the lightness (100 for white and 0 for black), the a* value represents greenness and redness (−80 for green and 100 for red) whereas the b* value signifies change from blueness to yellowness (−80 for blue and 70 for yellow). Total colour differences (ΔE) were calculated as follows (Rotta et al., Citation2009) (Equation 1):
2.8. Water solubility
For this study, water solubility of the films was defined as the percentage of the water-soluble dry matter of the film that is dissolved after immersion in distilled water. Water solubility (S) of the films was determined according to Pinotti, García, Martino, and Zaritzky (Citation2007). The initial dry matter of the film was determined by drying film pieces of 6 cm2 in a desiccator with silica gel (0% RH) for seven days. Furthermore, the films were weighed and immersed in 80 mL of distilled water at 25°C, with continuous stirring for one hour. The samples were filtered using filter paper (No. 1; Whatman International). Non-solubilized film portions were taken out and dried to determine the final weight of the dry matter. Solubility is reported as the difference between the initial and final dry matter with respect to the initial dry matter. Four determinations were performed for each film type.
2.9. Water vapour permeability
The water vapour permeability (WVP) of the films was determined gravimetrically using a modification of the ASTM E96-00 (American Society for Testing and Materials [ASTM], Citation2000) procedure. Film disks, previously equilibrated at 53% RH and 25°C for 48 h, were mounted on permeation cells (aluminium cups) containing silica gel (0% RH; ≈ 0 Pa water vapour partial pressure). The air gap inside the cup was 1.68 cm. The covered cells were placed in a cabinet that was previously equilibrated at 75 ± 2% RH and 23 ± 2°C for 24 h prior to the WVP tests. The cups were weighed eight times at 60-min intervals to obtain the linear function WC = f(t), where WC is the cup weight. Then, the WVP was determined according to the procedure reported by Aguirre-Loredo, Rodríguez-Hernández, and Chavarría-Hernández (Citation2014). Four determinations were carried out for each film type.
2.10. Oxygen permeability
The oxygen gas permeability (PO2) through the films was determined in accordance with ASTM D1434-82 (ASTM, Citation1998) using a film-package permeability tester (Labthink™ VAC-V2, China). The tests were performed at 25°C, using research-grade high-purity oxygen gas (34161, INFRA™ Mexico). The films were conditioned at 50 ± 5% RH for 48 h before placing them into the chambers of the permeability tester. All runs were carried out with a constant oxygen pressure in the high-pressure chamber (PO2-H) of 101325 Pa and 25°C. At the beginning of each run, the oxygen pressure in the low-pressure chamber (PO2-L,i) was nearly 0 Pa; then, this pressure increased due to oxygen permeation through the film until reaching a final oxygen pressure in the low-pressure chamber (PO2-L, f). Taking into account Fick’s law (Equation 2), the film oxygen permeability (PO2; g m s−1 Pa−1 m−2) was estimated with Equation (3) (Aguirre-Loredo et al., Citation2014). Four determinations were carried out for each film type:
2.11. Mechanical properties
A universal test Machine (TA plus, Lloyd) was used to determine the mechanical properties of the films, according to ASTM standard method D882 (ASTM, Citation2010). The films were cut as rectangular strips (9 cm × 1 cm). Before testing, all samples were equilibrated for four days at 53 ± 2% RH in a cabinet using magnesium nitrate saturate solution at 25°C. Equilibrated films were mounted in the film-extension grips of the testing machine; the gap between grips was 5 cm. The film specimens were stretched at a rate of 60 mm/min until the sample failed. At least 20 replicates of each formulation film were tested. Specimens that failed at the grip contact point were discarded. The stress–strain curves of the samples were obtained through the force–distance data (Peleg, Citation1987). The true stress (σT) was calculated according to Equation (5), where F(t) is the force that varies with time and A(t) is the momentary cross-sectional area of the film strip
The true strain or Hencky strain (ЄH) was calculated using Equation (6), where L(t) and L0 are the length during the test and the length at zero time, respectively
Young’s modulus (E) was determined through the slope of the linear region of the σT-ЄH curves. The ultimate mechanical properties of the films, stress and strain at break, were determined in the rupture point. The data represent an average of at least seven replicates.
2.12. Statistical analysis
Data are presented as the mean ± standard deviation of each treatment. Data were analysed for statistical significance using analysis of variance (ANOVA) followed by the Tukey test (p < 0.05) (SigmaPlot 11.0, SPSS Inc., USA).
3. Results and discussion
3.1. Antimicrobial activity
shows the inhibition halos of the growth of L. monocytogenes in BHI agar due to the antilisterial activity of AM () and nisin (). The highest dilutions of AM and nisin, where a defined zone of growth inhibition was recorded, were 28 and 26, respectively. According to the method used in this work, the concentrated supernatant, AM, has an antimicrobial activity of 24,220 AU/mL. The volumes of nisin stock and AM were correlated to have 93,000 AU to formulate the bioactive films. shows the growth evolution of L. monocytogenes in Oxford agar plates incubated at 35°C and 32% RH for eight days (i.e. 192 h). The growth of L. monocytogenes in the untreated plate (i.e. Oxford agar plate inoculated with L. monocytogenes with no film) increased substantially in the first 48 h; thereafter, it remained on a near-constant value of 53 CFU/plate. The Oxford plate in depth contact with films containing AM (C-AM) revealed colonies just after 48 h and the CFU-counts were constant (i.e. 10 CFU/plate) up to the final (i.e. 192 h). That treatment presented a notorious inhibitory effect on the growth of listeria compared with the untreated plate. The control film of C-AM treatment (C-AM-control) was formulated with all components of the C-AM film, including the same volume of the concentrated supernatant of the culture medium with no S. infantarius inoculum; thus, basically only the antimicrobial activity produced by S. infantarius was absent in those films. C-AM-control films were less effective in inhibiting the growth of L. monocytogenes; the treated plate with C-AM-control film showed 22 CFU/plate from the first day to the end. The control film hindered the growth of L. monocytogenes, with populations that were near 60% lower than the untreated plate. That partial inhibitory effect can be attributed to the oxygen barrier produced by the biopolymeric film, taking into account that L. monocytogenes is a facultative anaerobe microorganism with the ability to survive low oxygen conditions (Lungu, Ricke, & Johnson, Citation2009). Certainly, the antilisterial effectiveness of C-AM film was due to the antimicrobial peptides produced by S. infantarius, which were slowly released to the plate inoculated with L. monocytogenes. According to these findings, the concentration of bioactive compounds – 93,000 AU/film – was enough to inhibit 80% of the growth of L. monocytogenes even at 35°C, taking into consideration that the optimum temperature range for its growth is 30–37°C (Low & Donachie, Citation1997). On the other hand, C-Nis and C-Nis-control treatments exhibited similar trends, showing no difference on the antilisterial effect due to nisin incorporation into the film. Both treatments showed growth of L. monocytogenes at 24 h with counts higher than C-AM treatment at that time. Furthermore, in C-AM treatment listeria exhibited a higher lag-phase than that recorded in C-Nis treatment.
Figure 1. Inhibition halos of the growth of L. monocytogenes in BHI agar during the quantification of bacteriocin activity by the critical dilution method. (A) Antilisterial activity of the antimicrobial peptide (AM) produced by S. infantarius, 21 to 212 were the dilutions examined. (B) Antilisterial activity of nisin, 21 to 28 were the dilutions examined.
Figura 1. Halos de inhibición del crecimiento de L. monocytogenes en agar BHI durante la cuantificación de la actividad de bacteriocinas por el método de dilución crítica. (A) Actividad antilisterial del péptido antimicrobiano (AM) producido por S. infantarius, 20 a 212 fueron las diluciones examinadas. (B) Actividad antilisterial de nisina, 20 a 28 fueron las diluciones examinadas.
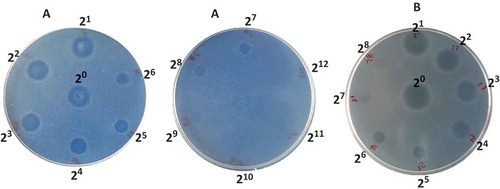
Figure 2. Growth evolution of L. monocytogenes in Oxford agar plates without films (white circles) and with: C-AM film (grey squares); C-AM-control film (white squares); C-Nis film; (grey triangles); C-Nis-control film (white triangles). Plates incubated at 35°C and 32% RH.
Figura 2. Evolución del crecimiento de L. monocytogenes en placas de agar Oxford sin película (círculos blancos) y con: películas C-AM (cuadros grises); película C-AM-control (cuadros blancos); película C-Nis (triángulos grises) y película C-Nis-control (triángulos blancos). Placas incubadas a 35°C y 32% HR.
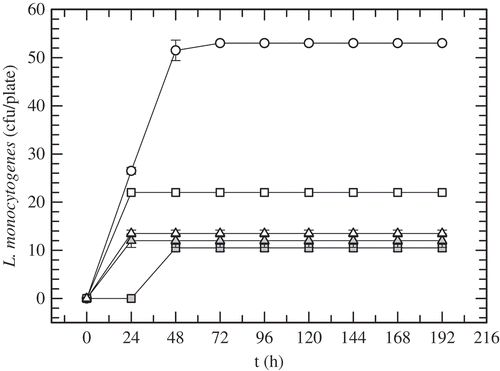
shows the growth evolution of L. monocytogenes in Oxford agar plates with the antimicrobial films evaluated in this work, but incubated at 4°C and 98% RH (i.e. refrigeration conditions). Colonies of L. monocytogenes were observed just after the seventh day (i.e. 168 h) in the plate with no film, and the growth (46–48 CFU/plate) was maintained till the end of the experiment (i.e. 63 days). Both C-Nis and C-AM films exhibited long lag-phases of the bacterial growth up to 11 and 38 days, respectively, and the counts were maintained without changes until the end. AM showed a major antilisterial effect, with the L. monocytogenes counts being 6 CFU/plate, whereas the C-AM-control film had 30 CFU/plate. Nisin produced only a marginal antilisterial effect in the films, resulting in an increase of just one day in the bacterial growth lag-phase compared to its control film. Besides, significantly more colonies of L. monocytogenes were grown in plates treated with C-Nis films than those with C-AM films. According to these findings, the antimicrobial substances produced by S. infantarius, a strain isolated from a Mexican traditional fermented beverage, have higher antimicrobial activity against L. monocytogenes in caseinate films than that promoted by commercial nisin at the tested conditions. Currently, our group is conducting some experiments to separate and identify the biochemical nature of the bacteriocin produced by S. infantarius, which is a heat-stable peptide (121°C/15 min) with a molecular weight near 9 kDa and a notable antilisterial activity. Thus, it likely would be categorized within the class-II bacteriocins (Balciunas et al., Citation2013). Moreover, nisin is a class I bacteriocin, widely used as a biopreservative against L. monocytogenes and other Gram-positive pathogens; it has a lower molecular weight (≈ 4 kDa) than class II bacteriocins (<10 kDa). Thus, although diffusion studies were not carried out in this work, it is likely that nisin diffuses faster from the film to the agar medium assay; subsequently, its concentration on the film-medium interface probably is not enough to show significant inhibition of L. monocytogenes for extended time. Besides, the nisin concentration was considerably lower than that reported in other studies (Cao-Hoang, Grégoire, et al., Citation2010; Guiga et al., Citation2010; Kristo, Koutsoumanis, & Biliaderis, Citation2008; Li, Kennedy, Peng, Yie, & Xie, Citation2006) where growth inhibition of L. monocytogenes or L. innocua was observed. On the contrary, AM could have been released more slowly from the caseinate films, thus maintaining an effective concentration on the interface film-agar surface where L. monocytogenes were grown. Similar findings were found by Kristo et al. (Citation2008) in sodium caseinate films incorporating nisin or potassium sorbate or sodium lactate, incubated at 10°C for 10 days. They observed that nisin films (0.5 mg nisin/film) were the most effective in reducing L. monocytogenes growth, as nisin perhaps would be released more slowly from the sodium caseinate matrix than the two salts examined, Na-lactate and K-sorbate, thus maintaining an effective concentration on the contact surface. Other authors have reported similar deductions about the influence of the antimicrobial activity releasing on other functional films (Otoni et al., Citation2014; Sanjurjo, Flores, Gerschenson, & Jagus, Citation2006). Most of the findings have reported greater retention and enhancement of the antimicrobial activity of nisin against Listeria spp. when it is added to the films or coatings compared to its direct application on the surface of foods (Guiga et al., Citation2010; Sanjurjo et al., Citation2006). However, some studies concerning the desorption phenomenon of the antimicrobial agent from biopolymer film showed that a quick desorption of the those agents induces a rapid loss of its activity (Guiga et al., Citation2010). Nonetheless, other factors might also affect the effectiveness of the antimicrobial film, such as the intermolecular forces between polymer matrix and antimicrobial agent, and the connectivity of the macromolecular network.
Figure 3. Growth evolution of L. monocytogenes in Oxford agar plates without films (white circles) and with: C-AM film (grey squares); C-AM-control film (white squares); C-Nis film; (grey triangles); C-Nis-control film (white triangles). Plates incubated at 4°C and 98% RH.
Figura 3. Evolución del crecimiento de L. monocytogenes en placas de agar Oxford sin película (círculos blancos) y con: películas C-AM (cuadros grises); película C-AM-control (cuadros blancos); película C-Nis (triángulos grises) y película C-Nis-control (triángulos blancos). Placas incubadas a 4°C y 98% HR.
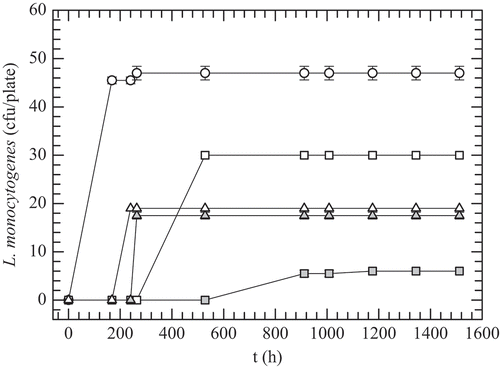
3.2. Colour evaluation
Homogeneous, thin, flexible and transparent films were obtained from sodium caseinate. The films were easily removed from the cast plate. The inclusion of the AM into the films yields films thicker than C-Nis films (90–107 micrometres for C-Nis films and 170–200 micrometres for C-AM films); additionally, C-AM films showed an amber colouration. shows the L*, a*, b* and ΔΕ values of caseinate films. C-Nis films were transparent with L*, a* and b* values close to white background; consequently, ΔE values were small. In contrast, the AM incorporation in the caseinate films reduced their lightness (L*) and their chromaticity parameters increased, which confirm the red (a*: 10.52 and 10.83) to yellow (b*: 29.55 and 29.29) appearance of the films, attributed to the concentrated supernatant from the culture medium of S. infantarius since there is no significant difference between C-AM and C-AM-control. Moreover, the colour differences (ΔE) of the C-AM films, compared to the white background, increased up to values near 50.
Table 1. Physical properties of caseinate films with antimicrobial agents from S. infantarius isolated from pozol (AM) and nisin (Nis).
Tabla 1. Propiedades físicas de películas de caseinato con agentes antimicrobianos de S. infantarius aislado del Pozol (AM) y nisina (Nis).
3.3. Water solubility
Water solubility is a valuable property of food biopolymer films; it determines their biodegradability. Nonetheless, high water solubility of the films could be a disadvantage for those films used on high RH environments. The water solubility of the films is shown in . The C-AM films were more soluble than the C-Nis ones. The significant increase in the water solubility of the C-AM films can be attributed to the plasticization effect exerted by the antimicrobial peptides from S. infantarius, including remnants of the culture media, which presumably increased the interstitial spaces in the caseinate matrix, improving the swelling and thus the dissolution of the film during its water exposition. Otherwise, the lower solubility of C-Nis films might be due to the major formation of crystalline zones in these films. This hypothesis is consistent with the behaviour of the mechanical and permeability properties of the films, which will be discussed later.
3.4. Water vapour permeability
The WVP values of the different films are given in . As can be seen there, the WVP values of the C-AM films were one order of magnitude higher than those of C-Nis films. These results suggest that AM could have produced a less-dense protein network than nisin. Both antimicrobial agents act as plasticizers, increasing the free volume of the system, the solvent mobility and, consequently, the water diffusion in the matrix of the film. Moreover, probably nisin is a smaller molecule than the antimicrobial peptides from AM. Moreover, this latter is not a highly purified peptide; later some components from the culture medium, including water, could also be acting as plasticizers in C-AM films. Previous reports on edible films have reported that polyols and other small molecules increase the WVP values of the films (Kristo et al., Citation2008; Sobral, Menegalli, Hubinger, & Roques, Citation2001). On the other hand, no significant differences (p < 0.05) were observed between the antimicrobial active film and its control. This behaviour could be attributed to the low concentrations of the bioactive peptides used in the films, relative to protein content. These results are in agreement with those of Ko, Janes, Hettiarachchy, and Johnson (Citation2001) who observed that nisin (4.0–160 IU/film disk) had no significant effect on the WVP values of whey protein isolates, soy protein isolates, egg albumen and wheat gluten films.
The WVP values of the bioactive caseinate films were similar to those reported by Jouki, Yazdi, Mortazavi, and Koocheki (Citation2014) (WVP = 0.76 to 1.7 × 10−10 g m s−1 m−2 Pa−1) for quince seed mucilage films with oregano essential oil. Results in the same order of magnitude have been also reported for protein-nisin films (Ko et al., Citation2001) (WVP = 4.02 to 6.7 × 10−10 g m s−1 m−2 Pa−1), carboxymethycelullose films containing oleic acid (WVP = 1.86 to 2.31 × 10−10 g m s−1 m−2 Pa−1) (Ghanbarzadeh & Almasi, Citation2011) and for caseinate-nisin films (WVP = 9.8 × 10−10 g m s−1 m−2 Pa−1) (Kristo et al., Citation2008).
3.5. Oxygen permeability
also shows the oxygen permeability (PO2) of the bioactive caseinate films. The AM did not promote significant differences between the antimicrobial active film and its control, although its incorporation showed a slight tendency to increase the PO2 value of the film. Generally, biopolymer films have showed a good oxygen barrier capacity. The oxygen permeability through caseinate films was notably lower when compared with WVP, with differences involving three orders of magnitude. It is noteworthy that the films containing nisin had higher oxygen permeability values; they were up to four times those of films containing AM. These results are in concordance with those reported by Srinivasa, Ramesh, and Tharanathan (Citation2007) in plasticized and non-plasticized chitosan films. According to these authors, plasticization results in higher mobility of polymer chains yielding a gas transmission resistance decrease of the films. However, several factors might be involved in the observed differences in oxygen permeability of biopolymer films. These include the molecular weight and physical state of the plasticizer, modifications in the film structure due to chemical interactions of plasticizer, the ratio between crystalline and amorphous zones, the hydrophilic–hydrophobic ratio, the polymeric chain mobility and the absorption of oxygen molecules due to the oxygen solubility in the film (Srinivasa et al., Citation2007; Valenzuela, Abugoch, & Tapia, Citation2013). Thus, oxygen, which is a non-polar molecule, can diffuse more efficiently through less-polar films, such as C-Nis films, compared with others, such as C-AM films, where the water and low molecular solutes (like antimicrobial peptides and culture media remnants) can restrict the flux of oxygen. PO2 values of the caseinate bioactive films evaluated in this work were similar to those reported for caseinate films with antioxidants (PO2 = 5.6 to 8.3 × 10−13 g m s−1 m−2 Pa−1) (Fabra, Hambleton, Talens, Debeaufort, & Chiralt, Citation2011), chitosan-steric acid films (PO2 = 3.49 × 10−13 g m s−1 m−2 Pa−1) (Srinivasa et al., Citation2007) and chitosan-oleic acid films (PO2 = 1.4 to 4.6 × 10−13 g m s−1 m−2 Pa−1) (Aguirre-Loredo et al., Citation2014).
3.6. Mechanical properties
The mechanical properties are usually determined through uniaxial tension tests. A convenient parameter to quantify the stiffness of the film is Young’s modulus (Peleg, Citation1987). Tensile and elongation at break represent film resistance to elongation and the film’s capacity for stretching, respectively. The mechanical properties of caseinate films are listed in . C-Nis films displayed higher Young’s modulus values compared to C-AM ones. This effect suggests that AM acts as an additional plasticizer, decreasing the stiffness of the film. shows representative true stress–Hencky strain curves of the films. C-AM films showed a marked plastic deformation and C-Nis films were less extensible. According to Young’s modulus, the stiffness of the films diminished, even slightly, in the order in which the concentration of low molecular solutes (i.e. bioactive peptides and culture media remnants) increased within the film. The elongation at break of C-AM films was up to four times higher than that of C-Nis films. Also as expected, the stress at break was lower in C-AM films. Moreover, C-AM films showed significantly lesser values of Young’s modulus and stress at break compared to C-AM control films, whereas the mechanical properties of C-Nis films were not significantly different from those of their corresponding control films. The mechanical behaviour of the caseinate films agrees with their barrier and solubility properties discussed before. It is complicated to compare the tensile parameter obtained in this work with others reported for caseinate films because of the differences in experimental conditions (i.e. caseinate concentration, RH, temperature, film specimen size, crosshead rate) and data analysis. Fabra, Talens, and Chiralt (Citation2008) reported values of elastic modulus (EM), tensile strength (TS) and elongation (E) at break of 850 MPa, 14.4 MPa and 4%, respectively, for 8% caseinate-glycerol films conditioned at 58% RH and 5°C, and stretched at 50 mm/min. Other ambient conditions (33% RH, 25°C) yielded higher tensile parameters (EM = 1290 MPa, TS = 27.1 MPa, E = 3.1%) for 8% caseinate-glycerol films stretched at the same rate (Fabra et al., Citation2011). Besides, Jiménez, Fabra, Talens, and Chiralt (Citation2013) reported lower tensile parameters (EM = 400 MPa, TS = 6 MPa, E = 18%) for 2% caseinate-glycerol films conditioned at 53% RH and 25°C, and stretched at 50 mm/min.
Figure 4. Typical true stress (σT) vs. Hencky strain (ЄH) curves obtained in tensile test carried out on some representative caseinate films at 25°C. Film containing antimicrobial agent from S. infantarius (C-AM); control film of C-AM (C-AM-control); film with nisin (C-Nis) and control film of C-Nis (C-Nis-control).
Figura 4. Curvas típicas de esfuerzo verdadero (σT) vs. deformación de Hencky (εH) obtenidas en pruebas de tensión realizadas a 25°C en algunas películas representativas de caseinato. Películas con agente antimicrobiano de S. infantarius (C-AM); película control de C-AM (C-AM-control); película con nisina (C-Nis) y película control de C-Nis (C-Nis-control).
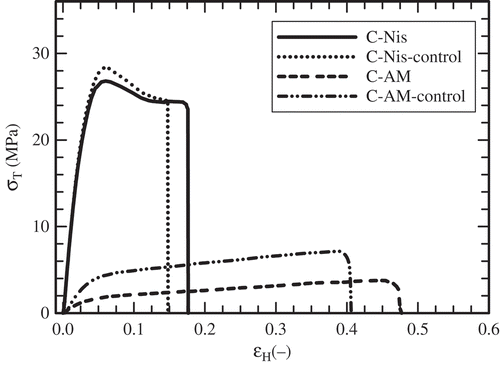
4. Conclusions
The antimicrobial substances produced by S. infantarius, a strain isolated from a Mexican traditional fermented beverage, had more antimicrobial activity against L. monocytogenes in caseinate films than that of commercial nisin under the examined conditions. The antilisterial effect was more effective at 4°C and 98% RH where C-AM films extended the lag-phase of the bacterial growth up to 38 days; after that period, 90% of the listeria present was inhibited till the end of the experiment (63 days). The combination of set temperature and caseinate films containing bacteriocins produced by S. infantarius may imply a higher effectiveness of antimicrobial films in long-term refrigeration storage. Additionally, the incorporation of antimicrobial compounds (AM or nisin) did not produce significant differences in the WVP and PO2 of the films compared with their control ones. AM acted as an additional plasticizer into the films; thus, C-AM films were less stiff and more plastic during the tensile tests.
Disclosure statement
No potential conflict of interest was reported by the authors.
Additional information
Funding
References
- Aguirre-Loredo, R. Y., Rodríguez-Hernández, A. I., & Chavarría-Hernández, N. (2014). Physical properties of emulsified films based on chitosan and oleic acid. CyTA - Journal of Food, 12(4), 305–312. doi:10.1080/19476337.2013.853207
- Appendini, P., & Hotchkiss, J. H. (2002). Review of antimicrobial food packaging. Innovative Food Science & Emerging Technologies, 3(2), 113–126. doi:10.1016/S1466-8564(02)00012-7
- ASTM. (1998). Standard test method for determining gas permeability characteristics of plastic film and sheeting. D1434-82. Annual Book of ASTM Standards. Philadelphia, PA: Author.
- ASTM. (2000). Standard methods for water vapour transmission of material. E96-00. Annual Book of ASTM Standards. Philadelphia, PA: Author.
- ASTM. (2010). Standard test method for tensile properties of thin plastic sheeting, D882-10. Annual Book of ASTM Standards. Philadelphia, PA: Author.
- Balciunas, E. M., Castillo Martinez, F. A., Todorov, S. D., Franco, B. D. G. D. M., Converti, A., & Oliveira, R. P. D. S. (2013). Novel biotechnological applications of bacteriocins: A review. Food Control, 32(1), 134–142. doi:10.1016/j.foodcont.2012.11.025
- Cao-Hoang, L., Chaine, A., Grégoire, L., & Waché, Y. (2010). Potential of nisin-incorporated sodium caseinate films to control Listeria in artificially contaminated cheese. Food Microbiology, 27(7), 940–944. doi:10.1016/j.fm.2010.05.025
- Cao-Hoang, L., Grégoire, L., Chaine, A., & Waché, Y. (2010). Importance and efficiency of in-depth antimicrobial activity for the control of listeria development with nisin-incorporated sodium caseinate films. Food Control, 21(9), 1227–1233. doi:10.1016/j.foodcont.2010.02.004
- Cotter, P. D., Hill, C., & Ross, R. P. (2005). Bacteriocins: Developing innate immunity for food. Nature Reviews Microbiology, 3(10), 777–788. doi:10.1038/nrmicro1273
- Fabra, M. J., Hambleton, A., Talens, P., Debeaufort, F., & Chiralt, A. (2011). Effect of ferulic acid and α-tocopherol antioxidants on properties of sodium caseinate edible films. Food Hydrocolloids, 25(6), 1441–1447. doi:10.1016/j.foodhyd.2011.01.012
- Fabra, M. J., Talens, P., & Chiralt, A. (2008). Effect of alginate and λ-carrageenan on tensile properties and water vapour permeability of sodium caseinate–lipid based films. Carbohydrate Polymers, 74(3), 419–426. doi:10.1016/j.carbpol.2008.03.010
- Gálvez, A., Abriouel, H., López, R. L., & Omar, N. B. (2007). Bacteriocin-based strategies for food biopreservation. International Journal of Food Microbiology, 120(1–2), 51–70. doi:10.1016/j.ijfoodmicro.2007.06.001
- Ghanbarzadeh, B., & Almasi, H. (2011). Physical properties of edible emulsified films based on carboxymethyl cellulose and oleic acid. International Journal of Biological Macromolecules, 48(1), 44–49. doi:10.1016/j.ijbiomac.2010.09.014
- Guiga, W., Galland, S., Peyrol, E., Degraeve, P., Carnet-Pantiez, A., & Sebti, I. (2009). Antimicrobial plastic film: Physico-chemical characterization and nisin desorption modeling. Innovative Food Science & Emerging Technologies, 10(2), 203–207. doi:10.1016/j.ifset.2008.09.006
- Guiga, W., Swesi, Y., Galland, S., Peyrol, E., Degraeve, P., & Sebti, I. (2010). Innovative multilayer antimicrobial films made with Nisaplin® or nisin and cellulosic ethers: Physico-chemical characterization, bioactivity and nisin desorption kinetics. Innovative Food Science & Emerging Technologies, 11(2), 352–360. doi:10.1016/j.ifset.2010.01.008
- Imran, M., El-Fahmy, S., Revol-Junelles, A.-M., & Desobry, S. (2010). Cellulose derivative based active coatings: Effects of nisin and plasticizer on physico-chemical and antimicrobial properties of hydroxypropyl methylcellulose films. Carbohydrate Polymers, 81(2), 219–225. doi:10.1016/j.carbpol.2010.02.021
- Imran, M., Revol-Junelles, A.-M., René, N., Jamshidian, M., Akhtar, M. J., Arab-Tehrany, E., … Desobry, S. (2012). Microstructure and physico-chemical evaluation of nano-emulsion-based antimicrobial peptides embedded in bioactive packaging films. Food Hydrocolloids, 29(2), 407–419. doi:10.1016/j.foodhyd.2012.04.010
- Jiménez, A., Fabra, M. J., Talens, P., & Chiralt, A. (2013). Physical properties and antioxidant capacity of starch–sodium caseinate films containing lipids. Journal of Food Engineering, 116(3), 695–702. doi:10.1016/j.jfoodeng.2013.01.010
- Jouki, M., Yazdi, F. T., Mortazavi, S. A., & Koocheki, A. (2014). Quince seed mucilage films incorporated with oregano essential oil: Physical, thermal, barrier, antioxidant and antibacterial properties. Food Hydrocolloids, 36, 9–19. doi:10.1016/j.foodhyd.2013.08.030
- Ko, S., Janes, M. E., Hettiarachchy, N. S., & Johnson, M. G. (2001). Physical and chemical properties of edible films containing nisin and their action against Listeria monocytogenes. Journal of Food Science, 66(7), 1006–1011. doi:10.1111/j.1365-2621.2001.tb08226.x
- Kristo, E., Koutsoumanis, K. P., & Biliaderis, C. G. (2008). Thermal, mechanical and water vapor barrier properties of sodium caseinate films containing antimicrobials and their inhibitory action on Listeria monocytogenes. Food Hydrocolloids, 22(3), 373–386. doi:10.1016/j.foodhyd.2006.12.003
- Li, B., Kennedy, J. F., Peng, J. L., Yie, X., & Xie, B. J. (2006). Preparation and performance evaluation of glucomannan–chitosan–nisin ternary antimicrobial blend film. Carbohydrate Polymers, 65(4), 488–494. doi:10.1016/j.carbpol.2006.02.006
- Low, J. C., & Donachie, W. (1997). A review of Listeria monocytogenes and listeriosis. The Veterinary Journal, 153(1), 9–29. doi:10.1016/S1090-0233(97)80005-6
- Lungu, B., Ricke, S. C., & Johnson, M. G. (2009). Growth, survival, proliferation and pathogenesis of Listeria monocytogenes under low oxygen or anaerobic conditions: A review. Anaerobe, 15(1–2), 7–17. doi:10.1016/j.anaerobe.2008.08.001
- Mendoza-Mendoza, B., Rodríguez-Hernández, A. I., Vargas-Torres, A., Díaz-Ruiz, G., Ramos-Aboites, H. E., Castro-Rosas, J., & Chavarría-Hernández, N. (2013). Characterization of the effects on the growth kinetics of Listeria monocytogenes in solid culture in contact with caseinate base edible films added with antilisterial activity from Streptococcus sp. ABMX isolated from Pozol, an indigenous Mexican beverage. International Food Research Journal, 20(5), 2917–2925.
- Min, S., & Krochta, J. M. (2008). Edible coatings containing bioactive antimicrobial agents. In J. H. Han (Ed.), Packaging for nonthermal processing of food (pp. 29–52). Hoboken, NJ: Wiley-Backwell.
- Nuñez, M., Tomillo, J., Gaya, P., & Medina, M. (1996). Bacteriocin quantification by the critical dilution method: A comparison of arbitrary units with diameter and area of the zone of growth inhibition. Milchwissenschaft, 51(1), 7–10.
- Otoni, C. G., De Moura, M. R. D., Aouada, F. A., Camilloto, G. P., Cruz, R. S., Lorevice, M. V., … Mattoso, L. H. C. (2014). Antimicrobial and physical-mechanical properties of pectin/papaya puree/cinnamaldehyde nanoemulsion edible composite films. Food Hydrocolloids, 41, 188–194. doi:10.1016/j.foodhyd.2014.04.013
- Peleg, M. (1987). The basics of solid foods rheology. In H. R. Moskowitz (Ed.), Food texture. Instrumental and sensory measurement (pp. 3–34). New York, NY: Marcel Dekker.
- Pinotti, A., García, M. A., Martino, M. N., & Zaritzky, N. E. (2007). Study on microstructure and physical properties of composite films based on chitosan and methylcellulose. Food Hydrocolloids, 21(1), 66–72. doi:10.1016/j.foodhyd.2006.02.001
- Rotta, J., Ávila-Ozório, R., Kehrwald, A. M., De Oliveira Barra, G. M., De Melo Castanho Amboni, R. D., & Manrique Barreto, P. L. M. (2009). Parameters of color, transparency, water solubility, wettability and surface free energy of chitosan/hydroxypropylmethylcellulose (HPMC) films plasticized with sorbitol. Materials Science and Engineering: C, 29(2), 619–623. doi:10.1016/j.msec.2008.10.032
- Sanjurjo, K., Flores, S., Gerschenson, L., & Jagus, R. (2006). Study of the performance of nisin supported in edible films. Food Research International, 39(6), 749–754. doi:10.1016/j.foodres.2006.01.016
- Santiago-Silva, P., Soares, N. F. F., Nóbrega, J. E., Júnior, M. A. W., Barbosa, K. B. F., Volp, A. C. P., … Würlitzer, N. J. (2009). Antimicrobial efficiency of film incorporated with pediocin (ALTA® 2351) on preservation of sliced ham. Food Control, 20(1), 85–89. doi:10.1016/j.foodcont.2008.02.006
- Sobral, P. J. A., Menegalli, F. C., Hubinger, M. D., & Roques, M. A. (2001). Mechanical, water vapor barrier and thermal properties of gelatin based edible films. Food Hydrocolloids, 15(4–6), 423–432. doi:10.1016/S0268-005X(01)00061-3
- Srinivasa, P. C., Ramesh, M. N., & Tharanathan, R. N. (2007). Effect of plasticizers and fatty acids on mechanical and permeability characteristics of chitosan films. Food Hydrocolloids, 21(7), 1113–1122. doi:10.1016/j.foodhyd.2006.08.005
- Valenzuela, C., Abugoch, L., & Tapia, C. (2013). Quinoa protein-chitosan-sunflower oil edible film: Mechanical, barrier and structural properties. LWT - Food Science and Technology, 50(2), 531–537. doi:10.1016/j.lwt.2012.08.010
- Xu, X., Li, B., Kennedy, J. F., Xie, B. J., & Huang, M. (2007). Characterization of konjac glucomannan–gellan gum blend films and their suitability for release of nisin incorporated therein. Carbohydrate Polymers, 70(2), 192–197. doi:10.1016/j.carbpol.2007.03.017