Abstract
Fermented plant beverages (FPBs) are non-alcoholic and are produced by lactic acid bacteria (LAB) with different edible plant sources. Owing to the lack of a detailed study on fermented Hericium erinaceus with LAB, the current study was employed to exploit and optimize the fermentation condition for the production of L-glutamic acid (GA)- and γ-aminobutyric acid (GABA)-rich fermented H. erinaceus beverage by Lactobacillus brevis HP2 and Lactobacillus fermentum HP3, respectively. Box–Behnken design (BBD) for response surface methodology was employed with cofactor, pH and temperature as independent variables. The results suggested that L. brevis HP2 and L. fermentum HP3 are good starter cultures for H. erinaceus fermentation with enriched GA and GABA production. In this case, GA production was significantly influenced by K2HPO4, pH and temperature whereas GABA production was significantly influenced by pH and temperature. Further thorough and fine optimization is required to fabricate high-quality fermented drinks with low cost.
Las bebidas de plantas fermentadas (FPB) son sin alcohol y son producidas con bacterias ácido-lácticas (LAB) con diferentes fuentes de plantas comestibles. Debido a la falta de un estudio detallado sobre la fermentación de Hericium erinaceus con LAB, se empleó el presente estudio para explotar y optimizar la condición de fermentación para la producción de ácido L-glutámico (GA) y ácido γ-aminobutírico (GABA) en la bebida de H. erinaceus ricamente fermentado con Lactobacillus brevis HP2 y Lactobacillus fermentum HP3, respectivamente. Se empleó El diseño Box-Behnken para la metodología de superficie de respuesta con cofactor, pH y temperatura como variables independientes. Los resultados mostraron que L. brevis HP2 y L. fermentum HP3 son buenos cultivos de inicio para la fermentación de H. erinaceus con producción de ácido L-glutámico y ácido γ-aminobutírico enriquecidos. En este caso, la producción de ácido L-glutámico estuvo influenciada de manera significativa por K2HPO4, el pH, y la temperatura mientras que la producción de ácido γ-aminobutírico estuvo influenciada de manera significativa por el pH y la temperatura. Se requiere una optimización más exhaustiva y precisa para elaborar bebidas fermentadas de alta calidad con un bajo coste.
Introduction
Fermented plant beverages (FPBs) are prominent in East Asian countries such as Thailand, Japan, Korea and China (Kim, Nam, & Friedman, Citation2013; Lee et al., Citation2010). FPBs are non-alcoholic and are produced by lactic acid bacteria (LAB) from different edible plants such as medicinal plants, vegetables and fruits (Kantachote & Charernjiratrakul, Citation2008a, Citation2008b; Prachyakij, Charernjiratrakul, & Kantachote, Citation2008; Ratanaburee, Kantachote, Charernjiratrakul, Penjamras, & Chaiyasut, Citation2011). Fermented plant juices are well known and are considered to be a novel functional food product since they contain live LAB-based probiotics, plenty of nutrients, antioxidants and antimicrobials (Duangjitcharoen, Kantachote, Ongsakul, Poosaran, & Chaiyasut, Citation2009; Prachyakij et al., Citation2008; Ratanaburee et al., Citation2011).
The mushroom Hericium erinaceus is one of the famous bases for fermented mushroom beverages (FMBs) and known for its medicinal values. Fruiting body and mycelia display various pharmacological activities such as anti-cancer activity, immuno-modulating effect, neuro-protective activities, and antioxidant and antimicrobial activities (Kim et al., Citation2013; Lee et al., Citation2010; Lee, Min, Cho, & Hong, Citation2009; Wong, Sabaratnam, Abdullah, Kuppusamy, & Naidu, Citation2009). H. erinaceus, also known as monkey’s head mushroom, is suitable for use as a natural substrate for glutamic acid (GA) fermentation because it has a high protein content (8.01%) and free amino acid (Choi, Park, Woo, Jeong, & Shin, Citation2003). The fermented juice with high GA can serve as the best substrate for the production of fermented juice with a high content of Gamma-aminobutyric acid (GABA).
GA is widely used in various industries such as food, pharmaceutical, biochemical, medical, cosmetics and analytical industries (Vijayalakshmi & Sarvamangala, Citation2011). GA is also a precursor of GABA, a four-carbon non-protein amino acid, widely distributed in nature, and serves as the major inhibitory neurotransmitter in mammalian nervous system (Lu, Xie, & Gu, Citation2009). GA has been used to treat hepatic coma (McDermott, Adams, & Riddell, Citation1954), mental retardation (Zimmerman, Burgemeister, & Putnam, Citation1946), cancer (act as an adjuvant to anticancer drugs) and antivincristine-induced neurotoxicity prevention (Kulkarni, Kulkarni, & Hamsa, Citation2005). GA has been produced through hydrolysation of L-glutamine by L-glutaminase (Jeya Prakash, Poorani, & Anantharaman, Citation2010). L-glutaminase acts as proteolytic endopeptidase and is the cellular enzyme deaminating the substrate L-glutamine, which hydrolyses the peptide bonds of protein molecules (Nathiya, Sooraj, Angayarkanni, & Palaniswamy, Citation2011).
GABA is extensively distributed in the environment (Ueno, Citation2000), which has notable physiological functions, for instance, as an anti-stress molecule (Vaiva et al., Citation2004), suppresses the propagation of cancer cells (Park & Oh, Citation2007), acts as an anti-hypertensive (Yoshimura et al., Citation2010) and it prevents diabetes (Soltani et al., Citation2011). GABA production in microbes has been reported through glutamic acid decarboxylase (GAD) (Li & Cao, Citation2010). GABA production is the resultant of an acid-resistance mechanism of bacteria, via decarboxylation of L-glutamate by GAD (Cotter & Hill, Citation2003).
We screened and identified the potential LAB, Lactobacillus brevis HP2 and Lactobacillus fermentum HP3 isolated from Thai fermented foods, which can produce extracellular glutaminase and GAD enzyme, respectively (Woraharn et al., Citation2014). There have been no comprehensive reports regarding the development of fermented H. erinaceus juice (FHJ) with high amounts of GABA using glutaminase and GAD-producing LAB.
Thus, the current study was conceived and carried out to explore the use of identified enzyme producing LAB strains for the production of FHJ with high content of GABA and to evaluate the impact of cofactor, pH and temperature on GA and GABA production. Use of LAB provides added advantage – it may also act as a probiotic. We adapted the response surface methodology (RSM), a collection of statistical techniques for researching multiple process variables with fewer experimental trials. The Box–Behnken design (BBD) is one such popular RSM used to identify the optimum conditions for production with optimum values of multiple variables. Thus, in this study, we applied RSM with BBD (Box & Behnken, Citation1960) to estimate the influence of treatment variables on GA and GABA production using H. erinaceus as the substrate for FMB.
Materials and methods
Materials
H. erinaceus, in a dry form, was purchased from an organic farm at Nakhonratchasima province, northeast of Thailand. Cane sugar and potable water were received from the local market. L. brevis HP2 and L. fermentum HP3 strain were used, which were isolated from Thai fermented foods and identified by 16S rRNA sequencing and deposited in the culture collection centre of faculty of Pharmacy, Chiang Mai University, Thailand (Woraharn et al., Citation2014). These strains were selected for fermentation since they produce significantly higher amounts of glutaminase and GAD (Woraharn et al., Citation2014). The selected LAB was cultured in MRS broth for seed culture preparation.
Methods
Description of the experimental design
Designing of an experiment for enzyme production has been influenced by several factors such as cofactors, temperature, etc. Based on the previous literature survey for GA production, we have selected three factors for experimental design – initial concentration of cofactor, initial pH and incubation temperature. K2HPO4 was used as a cofactor in the concentration range of 0–100 mM. pH (6–8) and temperature (30–40°C) were also selected as independent variables based on the previous literature (Katikala, Bobbarala, Tadimalla, & Guntuku, Citation2009; Vermeulen, Gänzle, & Vogel, Citation2007; Weingand-Ziadé, Gerber-Décombaz, & Affolter, Citation2003). Second-stage fermentation for GABA production also involved the selection of three factors including pylidoxal-5-phosphate (PLP), cofactor, concentration (0–0.05 mM) (Bai et al., Citation2009; Komatsuzaki, Nakamura, Kimura, & Shima, Citation2008), pH (4.5–6.5) (Cho, Chang, & Chang, Citation2007; Komatsuzaki et al., Citation2008) and temperature (30–40°C) (Cho et al., Citation2007; Lu et al., Citation2009; Ratanaburee et al., Citation2011).
The optimal combination of initial cofactor, initial pH and temperature for the production of GA and GABA in H. erinaceus fermentation by selected strains was examined using different concentrations of initial cofactor (X1) (w/v), initial pH (X2) and temperature (X3) (°C). A BBD was used to describe the character of the response surface in the normal experimental region. The levels of the independent variables (X1, X2 and X3) were studied at three levels and coded as −1, 0 and +1 for low, middle and high levels, respectively (). In order to statistically optimize the optimal condition and assess the main effects, interaction effects and quadratic effects of the three factors on the production of GA and GABA, a design with three factors and three levels including five replicates at the centre point was used ( and ). For assessment of the three factors, Equation (1) was used.
Table 1. Codes of independent variables and their actual values used in RSM optimization for L-glutamic acid and GABA production.
Tabla 1. Códigos de variables independientes y sus valores reales utilizados en la optimización RSM para la producción de ácido L-glutámico y ácido γ-aminobutírico.
Table 2. Box–Behnken design for optimization of three factors for GA production during H. erinaceus juice fermentation. Results are the outcome of three trials (mean ± SD).
Tabla 2. Diseño Box-Behnken para la optimización de tres factores para la producción de ácido L-glutámico durante la fermentación de zumo de H. erinaceus. Los datos son los resultados de tres pruebas (promedio ± SD).
Table 3. Estimated regression coefficients of L-glutamic acid concentration during first-stage fermentation.
Tabla 3. Coeficientes de regresión estimados de la concentración de ácido L-glutámico durante la 1a etapa de fermentación.
Table 4. Analysis of variance for the quadratic model of GA and GABA production in fermented H. erinaceus juice.
Tabla 4. Análisis de la varianza para el modelo cuadrático de la producción de ácido L-glutámico y ácido γ-aminobutírico en zumo de H. erinaceus fermentado.
Table 5. Box–Behnken design for optimization of three factors for GABA production during H. erinaceus juice fermentation. Results are the outcome of three trials (mean ± SD).
Tabla 5. Diseño Box-Behnken para la optimización de tres factores para la producción de ácido γ-aminobutírico durante la fermentación de zumo de H. erinaceus. Los datos son los resultados de tres pruebas (promedio ± SD).
where Y is the predicted response; β0 is the model constant; βi (β1–3) are the linear coefficients; βii (β11–33) are the quadratic coefficients; βij (i.e. β12) are the cross-product coefficients; and X1, X2 and X3 are independent variables.
Statistical analyses of RSM
The behaviour of the response surface was evaluated for the response function (Y) using the regression polynomial equation. After 17 runs of BBD arrangement, we obtained the coefficients of regression equation (β0, β1, β2, β3, β11 and so on) by SPSS 16.0 for Windows® (2007 SPSS, Inc.). The significances of all independent variable in terms of the polynomial were detected statistically by calculating the F-value at a probability level (p) of 0.05. Only the independent variable terms, found statistically significant (p < 0.05), were included in the reduced models. The model is resubmitted for statistical analysis to neglect all terms that are statistically non-significant (p > 0.05). Regression coefficients are used to create the regression equation and generate a response surface map and the acceptance of the model was determined using model p-value (p < 0.05), lack-of-fit p-value test (p > 0.05) and coefficient of determination (R2). The nearness of the coefficient of determination (R2) to 1 or 100% ensures the satisfactory adjustment of the quadratic model to the experimental data. The surface plot based on two independent variables was generated by keeping the third independent variable at a constant level (middle value) with response value (GA or GABA). The behaviour of the response surface was created using a regression equation by Statistica 6.0 (the demo version from http://statistica.software.informer.com/6.0/).
First-stage fermentation for GA production
L. brevis HP2 was propagated in culture medium (50% of MRS medium (Himedia, Cat No. M369) and 50% of 10% (w/v) cane sugar) for 18 h at 37°C. The cells were pelleted out by centrifuge at 6000 rpm for 10 min at 4°C and were washed twice with 0.85% (w/v) NaCl. The cell pellet was resuspended in sterile distilled water to approximately 109 CFU/mL and was used as inoculum (starter culture). Dried H. erinaceus, cane sugar and potable water were mixed at a ratio of 10:5:85 (w:w:v), respectively. The cane sugar and K2HPO4 were dissolved in the potable water and were boiled. Then the mixture was cooled down to 80–90°C and dried mushroom was added to the mixture for pasteurization (75°C for 10 min), then the pH was adjusted according to using 5 M citric acid and 5 M NaOH. Bacterial load of the mixture was also assessed before fermentation to ensure the sterility (data not shown). The mixture of the fermentation after inoculating 10% of the initial culture had a total volume of 16 L in a 20 L plastic bucket. All fermentation containers were incubated at 30 ± 2°C for 30 days to monitor changes in the amount of GA. Fermentation without starter served as the control. Finally, the optimum condition for GA production was used to prepare the H. erinaceus medium for the next step of fermentation.
Second-stage fermentation for GABA production
Starter culture of L. fermentum HP3 was prepared as described in first-stage fermentation. The FHJ with high GA was used as substrate medium for GABA synthesis. First-stage FHJ was added with PLP and pasteurized (75°C for 10 min), then pH was adjusted according to using 5 M citric acid and 5 M NaOH. Bacterial load of the mixture was also assessed before fermentation to ensure the sterility (data not shown). After adding 10% of inoculum to the substrate medium, fermentation containers were incubated at 30 ± 2°C for 30 days. Fermentation without starter served as control.
Analysis of L-GA and GABA by HPLC
Centrifugation was performed for 1 mL of culture at 6000 rpm for 10 min and the supernatant was collected for L-GA and GABA analysis. The standard solutions of GA, GABA and sample were determined by pre-column derivatization of phenylthiocarbamyl–GABA (PTC–GABA) from phenyl isothiocyanate (PITC) according to the method detailed by Cho et al. (Citation2007). Briefly, PITC solution was added to samples to form PTC–GABA and PTC–GA. The standard curves for GABA and L-GA were determined by following the same procedure to four concentrations of standards. Standards and samples were analysed for GA and GABA by HPLC (LC-10ADVP, Shimadzu, Japan) with C18 reversed-phase column (5 μm dia), 4.6 × 250 mm. Mobile phase A consisted of 70 mM sodium acetate buffer solution (pH 5.8) and mobile phase B was acetonitrile. The gradient system was optimized for a good separation. UV detector at 254 nm was used.
Statistical analysis
All experiments were performed in triplicate and all data are expressed as means ± SD. Dissimilarities between the mean values of multiple groups were evaluated by one-way analysis of variance (ANOVA). The differences were considered significantly different when p < 0.05.
Results and discussion
GA production
In the current study, RSM was engaged for the optimization of GA production using H. erinaceus as substrate and L. brevis HP2 as the starter culture. RSM helps determine the main and interaction effects of growth and GA production. Codes for independent variables for L-GA production were allotted and tabulated (). The diversity of each response variable was evaluated as a function of linear, quadratic and interaction terms of cofactor, K2HPO4 (X1), pH (X2) and temperature (X3). Phosphate is the reported cofactor of glutaminase (Yuasa, Okamura, & Kataoka, Citation2003). Use of sodium phosphate in beverage may not be appropriate for people with hypertension. Thus, we used potassium phosphate as the cofactor in this study. Seventeen combinations of parameters were selected based on BBD of experiment and all the combinations were tested for GA production. The actual productions of GA and predicted values are tabulated in . Regression coefficients for L-GA concentration in first-stage fermentation were estimated using SPSS and are represented in . Results suggested that the quadratic model was fitted for the GA production because of its best statistic data such as maximum R2 (97.9%) and maximum adjusted R2 (97.2%), and the lowest predicted residual sum of square (3.104) and lack of fit (0.187), implying that the model equation was adequate for predicting the production of GA (). Increased concentration of K2HPO4 resulted in an increased production of GA, whereas pH and temperature showed a linear effect in GA production. Linear interaction reveals that K2HPO4 concentration affects the GA production compared to pH and temperature (0.339 > 0.259 and 0.272) ( and Equation (2)). Besides, co-interaction of variables, K2HPO4 × temperature and K2HPO4 × pH, resulted in synergistic interaction with GA production and the influence of pH × temperature interaction was found to be less ( and Equation (2)). The concentration of cofactor (K2HPO4), pH and temperature of the reaction as 100 mM, 7 and 40°C, respectively, has been identified as optimum conditions for the maximum production of GA in both predicted and actual categories. Based on the regression coefficient values predicted, GA production was calculated by Equation (2). Based on the surface contour plot results, the optimum condition for L-GA enrichment during H. erinaceus fermentation by L. brevis HP2 included the following parameters: 100 mM K2HPO4, pH 8.0 and temperature 40°C (2.20 mg/mL of L-GA from Equation (2)) (). The GA production in control fermentation (fermentation without L. brevis HP2) yielded about 0.10 mg/mL in all 17 trials, which suggested that pH, temperature and cofactor are not influenced. However, in the presence of starter culture, L. brevis HP2, GA production was promoted with the influence of the tested factors.
Figure 1. Response surface for the effect of cofactor (K2HPO4), pH and temperature on L-glutamic acid (GA) concentration (mg/mL) after the first fermentation of H. erinaceus by glutaminase-producing L. brevis HP2: (a) effect of concentration of K2HPO4 (mM) and pH on GA production, (b) effect of concentration of K2HPO4 (mM) and temperature on GA production and (c) effect of pH and temperature on GA production.
Figura 1. Superficie de respuesta para el efecto del cofactor (K2HPO4), el pH y la temperatura en la concentración de ácido L-glutámico (GA) (mg/mL) después de la primera fermentación de H. erinaceus mediante L. brevis HP2 con producción de glutaminasa (a) efecto de la concentración de K2HPO4 (mM) y el pH en la producción de ácido L-glutámico (b) efecto de la concentración de K2HPO4 (mM) y la temperatura en la producción de ácido L-glutámico (c) efecto del pH y la temperatura en la producción de ácido L-glutámico.
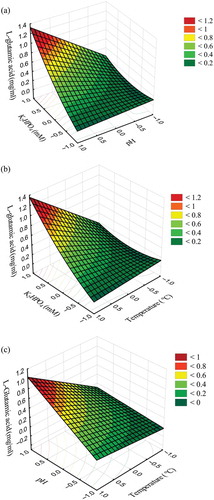
The impact of concentration of the cofactor on GA production in FHJ was correlated with other factors also. For example, an increase in production of GA was observed in trial no. 8, whereas very less amount of GA production was recorded in trial no. 2, with the same concentration of cofactor. GA production was not significantly affected by the pH of the medium, except trial no. 8. This data indicates that pH is one of the rate-limiting factors for GA production with selected LAB strain, but its impact was correlated with cofactor and temperature too (). Thus these data indicated that all parameters are necessary and they should be in precise levels to produce high amounts of GA in H. erinaceus fermentation with L. brevis HP2.
Zareian, Ebrahimpour, Mohamed, and Saari (Citation2013) have reported the production of GA and GABA by L. plantarum with optimization by BBD of experiment. The authors selected four different parameters to study and optimize the production of GA and GABA. They succeeded with the improvement of 3–10-fold higher productivity upon optimization. Another study revealed the use of BBD for the production of glucuronic acid from grape juice and the authors improved the production up to 178 g/L, which is higher than normal productivity (Yavari, Assadi, Moghadam, & Larijani, Citation2011).
Gamma amino butyric acid production
The second fermentation process was carried out with L. fermentum HP3 as starter culture and the resultant of the first fermentation as substrate. This fermentation process also employed the use of BBD of experiment for the production of GABA. Codes for independent variables for GABA production were allotted (). Each response variable was evaluated as a function of linear, quadratic and interaction terms of cofactor, PLP (X1), pH (X2) and temperature (X3). Seventeen combinations of parameters were generated based on BBD of experiment and all the combinations were tested for GABA production. The actual production of GABA and the predicted values are tabulated in . Regression coefficients for GABA production were estimated using SPSS and are represented in . All independent variables influenced the production of GABA as shown in the quadratic effect (X12, X22 and X32) ( and Equation (3)). Increase in pH and temperature positively regulates the GABA production. The PLP × pH and PLP × temperature had a destructive influence on GABA production, whereas pH × temperature showed a synergistic interaction ( and Equation (3)). The quadratic model is well opted for GABA production in this study, since it produces the greatest statistic data of maximum R2 (97.4%) and maximum adjusted R2 (94.1%), and the lowest predicted residual sum of square (0.948) and lack of fit (0.298), implying that the model equation was adequate for predicting the production of GABA ().
Table 6. Estimated regression coefficients of GABA concentration for second-stage fermentation.
Tabla 6. Coeficientes de regresión estimados de la concentración de ácido γ-aminobutírico para la 2a etapa de fermentación.
Optimum predicted production of GABA was noted with the combination of cofactor (PLP), pH and temperature (°C) at 25 µM, 6.5 and 40, respectively. However, the results of wet lab studies indicated that pH 6.5 and temperature 35°C without cofactor can produce a greater amount of GABA (2.11 ± 0.4 mg/mL) by L. fermentum HP3. This condition was noted as the second best condition for the predicted production (2.07 mg/mL). Based on the predicted regression coefficient values, GABA production was calculated in Equation (3). Based on the surface contour plot results, the optimum condition for GABA production during H. erinaceus fermentation by L. fermentum HP3 is 0 μM of PLP, pH 6.5 and temperature 40°C (2.44 mg/mL of GABA from Equation (3)) (). The GABA production in control fermentation (fermentation without L. fermentum HP3) yielded about 0.26 mg/mL in all 17 trials, which suggested that pH, temperature and cofactor are not influenced. However, in the presence of starter culture L. fermentum HP3, GABA production was promoted with the influence of the tested factors.
Figure 2. Response surface for the effect of cofactor (PLP), pH and temperature on γ-aminobutyric acid (GABA) concentration (mg/mL) after second-stage fermentation of H. erinaceus by L. fermentum HP3: (a) effect of concentration of PLP (µM) and pH on GABA production, (b) effect of concentration of PLP (µM) and temperature on GABA production and (c) effect of pH and temperature on GABA production.
Figura 2. Superficie de respuesta del efecto del cofactor (PLP), el pH y la temperatura en la concentración de ácido γ-aminobutírico (GABA) (mg/mL) después del segundo estado de fermentación de H. erinaceus con L. fermentum HP3 (a) efecto de la concentración de PLP (µM) y el pH en la producción de ácido γ-aminobutírico (b) efecto de la concentración de PLP (µM) y la temperatura en la producción de ácido γ-aminobutírico (c) efecto del pH y la temperatura en la producción de ácido γ-aminobutírico.
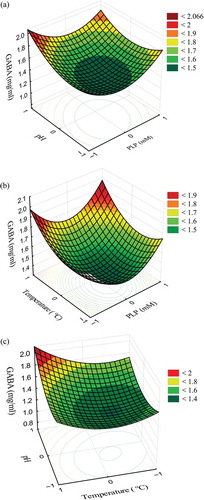
The cofactor in GABA production is not necessarily important for the optimum production of GABA. The third maximum production condition also revealed the unimportance of PLP (). Regarding pH, increased GABA production was observed in the trials in the pH range of 5.5–6.5. The optimum production (2.11 ± 0.4 mg/mL) of GABA was observed at pH 6.5 in the case of both prediction and real. Reducing the pH up to 5.5 did not have a huge impact on GABA production (2.04 ± 0.4 mg/mL) ( and ). Improved GABA production was obtained in trials in the temperature range of 35–40°C. These results indicated that all the parameters are necessary for the optimum production of GABA by L. fermentum HP3 using fermented H. erinaceus with L. brevis HP2 as substrate.
Lu et al. (Citation2009) studied the culture temperature, initial pH of the medium, monosodium glutamate (MSG) addition and MSG addition time affecting GABA production by Lactococcus lactis subsp. lactis (L. lactis) and they found that temperature 31.8°C, pH 7.1 and MSG 15 g/L were optimum. Influence of MSG on Lactobacillus plantarum DW12-mediated GABA production from red seaweed was reported (Ratanaburee et al., Citation2011). L. plantarum isolated from kimchi – a fermented Korean dish made of vegetables – produced 170.42 μg/mL of GABA in the presence of 3% MSG (Park, Lee, & Lim, Citation2014).
Another study by Cho et al. (Citation2007) also explains the optimum GABA production by another kimchi isolate of LAB – Lactobacillus buchneri – and they reported that 5% MSG, 1% NaCl and 1% glucose, with pH of 5.0 and temperature at 30°C, can yield about 94% GABA conversion rate after 36 h. These results were fairly far from the results of the current investigation. Although starter cultures are isolated from food sources and they are LAB, the ability of enzyme production and the optimum fermentation condition are varied between LAB strains and also depend on the substrate for the fermentation process.
GA production was increased up to threefold under the condition of pH 4.5, temperature 37°C, 12% (w/v) glucose and 0.7% (w/v) ammonium nitrate; however, GABA production enhancement was reported up to 10-fold under the condition of pH 4.5, temperature 37°C, 6% (w/v) glucose and 0.7% (w/v) ammonium nitrate by Lactobacillus plantarum MNZ (Zareian et al., Citation2013). MSG (1%), sucrose (6%) and an initial pH 6 are found as optimal conditions for the production of fermented red seaweed beverage with GABA (Ratanaburee et al., Citation2011). Guo, Hagiwara, Masuda, and Watabe (Citation2011) reported that Pichia anomala MR-1 strain produced high GABA (350 mg/100 mL of media) with the supplementation of 5% glucose and 1% MSG, but improper temperature profile and reaction mixture reduce the concentration’s desired product (120 mg/100 mL of media).
Conclusions
In conclusion, production of GA and GABA by LAB strains depends on the starter culture and substrate for the fermentation. The factors manipulating GA and GABA were studied by RSM. The results suggested that cofactor (K2HPO4) is the significant cause, which greatly influences the GA production rather than pH and temperature. About 100 mM of K2HPO4, with pH 7 and temperature at 40°C were found as the best conditions for the maximum production of GA. Increase in pH (4.5–6.5) and temperature (30–40°C) positively regulates the GABA production, whereas cofactor concentration (PLP) did not influence the GABA production during H. erinaceus fermentation by L. fermentum HP3. The results suggested that pH 6.5, temperature at 35°C and without factor (PLP) were found as the standard conditions for the maximum production of GABA.
The current study demonstrated and revealed the best conditions for the production of GA and GABA during H. erinaceus fermentation using L. brevis HP2 and L. fermentum HP3 as safe starter cultures. Further detailed and subtle optimization will provide the information for the production of plant-based, probiotic, high-quality fermented drinks with low cost.
Disclosure statement
No potential conflict of interest was reported by the authors.
Acknowledgements
The authors wish to acknowledge the use of instrumentation facility at Faculty of Pharmacy, Chiang Mai University.
Additional information
Funding
References
- Bai, Q., Chai, M., Gu, Z., Cao, X., Li, Y., & Liu, K. (2009). Effects of components in culture medium on glutamate decarboxylase activity and γ-aminobutyric acid accumulation in foxtail millet (Setaria italica L.) during germination. Food Chemistry, 116, 152–157. doi:10.1016/j.foodchem.2009.02.022
- Box, G. P., & Behnken, D. W. (1960). Some new three level designs for the study of quantitative variables. Technometrics, 2, 455–475. doi:10.1080/00401706.1960.10489912
- Cho, Y., Chang, J. Y., & Chang, H. C. (2007). Production of γ-aminobutyric acid (GABA) by Lactobacillus buchneri isolated from Kimchi and its neuroprotective effect on neuronal cells. Journal of Microbiology and Biotechnology, 17, 104–109.
- Choi, M. A., Park, N. Y., Woo, S. M., Jeong, Y. J., & Shin, S. R. (2003). Characteristics of Hericium erinaceus and it extracts. Korean Journal of Food Preservation, 10, 560–564.
- Cotter, P. D., & Hill, C. (2003). Surviving the acid test: Responses of gram-positive bacteria to low pH. Microbiology and Molecular Biology Reviews, 67, 429–453. doi:10.1128/MMBR.67.3.429-453.2003
- Duangjitcharoen, Y., Kantachote, D., Ongsakul, M., Poosaran, N., & Chaiyasut, C. (2009). Potential use of probiotic Lactobacillus plantarum SS2 isolated from a fermented plant beverage: Safety assessment and persistence in the murine gastrointestinal tract. World Journal of Microbiology and Biotechnology, 25, 315–321. doi:10.1007/s11274-008-9894-0
- Guo, X.-F., Hagiwara, T., Masuda, K., & Watabe, S. (2011). Optimal reaction conditions for the production of γ-aminobutyric acid by the marine yeast isolate Pichia anomala MR-1 strain. Bioscience, Biotechnology, and Biochemistry, 75, 1867–1871. doi:10.1271/bbb.100425
- Jeya Prakash, P., Poorani, E., & Anantharaman, P. (2010). Effect of media composition on L-glutaminase production from lagoon Vibrio sp. SFL-2. International Journal of Biotechnology & Biochemistry, 6, 769–782.
- Kantachote, D., & Charernjiratrakul, W. (2008a). Selection of lactic acid bacteria from fermented plant beverages to use as inoculants for improving the quality of the finished product. Pakistan Journal of Biological Sciences, 11, 2545–2552. doi:10.3923/pjbs.2008.2545.2552
- Kantachote, D., & Charernjiratrakul, W. (2008b). Effects of initial air removal methods on microorganisms and characteristics of fermented plant beverages. Pakistan Journal of Biological Sciences, 11, 173–180. doi:10.3923/pjbs.2008.173.180
- Katikala, P. K., Bobbarala, V., Tadimalla, P., & Guntuku, G. S. (2009). Screening of L-glutaminase producing marine bacterial cultures for extracellular production of L-glutaminase. International Journal of ChemTech Research, 1, 1232–1235.
- Kim, S. P., Nam, S. H., & Friedman, M. (2013). Hericium erinaceus (Lion’s Mane) mushroom extracts inhibits metastasis of cancer cells to the lung in CT-26 colon cancer-tansplanted mice. Journal of Agricultural and Food Chemistry, 61, 4898−4904. doi:10.1021/jf400916c
- Komatsuzaki, N., Nakamura, T., Kimura, T., & Shima, J. (2008). Characterization of glutamate decarboxylase from a high γ-aminobutyric acid (GABA)-producer, Lactobacillus paracasei. Bioscience Biotechnology and Biochemistry, 72, 278–285. doi:10.1271/bbb.70163
- Kulkarni, C., Kulkarni, K. S., & Hamsa, B. R. (2005). L-Glutamic acid and glutamine: Exciting molecules of clinical interest. Indian Journal of Pharmacology, 37, 148–154. doi:10.4103/0253-7613.16210
- Lee, J. S., Min, K. M., Cho, J. Y., & Hong, E. K. (2009). Study of macrophage activation and structural characteristics of purified polysaccharides from the fruiting body of Hericium erinaceus. Journal of Microbiology and Biotechnology, 19, 951–959. doi:10.4014/jmb.0901.0013
- Lee, S. J., Kim, E. K., Hwang, J. W., Kim, C. G., Choi, D. K., Lim, B. O., … Park, P. J. (2010). Neuroprotective effect of Hericium erinaceum against oxidative stress on PC12 cells. Journal of the Korean Society for Applied Biological Chemistry, 53, 283–289. doi:10.3839/jksabc.2010.044
- Li, H., & Cao, Y. (2010). Lactic acid bacterial cell factories for gamma-aminobutyric acid. Amino Acids, 39, 1107–1116. doi:10.1007/s00726-010-0582-7
- Lu, X. X., Xie, C. H., & Gu, Z. X. (2009). Optimisation of fermentative parameters for GABA enrichment by Lactococcus lactis. Czech Journal of Food Sciences, 27, 433–442.
- McDermott, W. V., Adams, R. D., & Riddell, A. G. (1954). Ammonia metabolism in man. Annals of Surgery, 140, 539–554. doi:10.1097/00000658-195410000-00010
- Nathiya, K., Sooraj, S. N., Angayarkanni, J., & Palaniswamy, M. (2011). Paddy straw: An inexpensive substrate for the production of L-glutaminase using native strain Aspergillus fumigates. Research Journal of Pharmaceutical, Biological and Chemical Sciences, 2, 297–302.
- Park, K.-B., & Oh, S.-H. (2007). Production of yogurt with enhanced levels of gamma-aminobutyric acid and valuable nutrients using lactic acid bacteria and germinated soybean extract. Bioresource Technology, 98, 1675–1679. doi:10.1016/j.biortech.2006.06.006
- Park, S.-Y., Lee, J.-W., & Lim, S.-D. (2014). The probiotic characteristics and GABA production of Lactobacillus plantarum K154 isolated from Kimchi. Food Science and Biotechnology, 23, 1951–1957. doi:10.1007/s10068-014-0266-2
- Prachyakij, P., Charernjiratrakul, W., & Kantachote, D. (2008). Improvement in the quality of a fermented seaweed beverage using an antiyeast starter of Lactobacillus plantarum DW3 and partial sterilization. World Journal of Microbiology and Biotechnology, 24, 1713–1720. doi:10.1007/s11274-008-9662-1
- Ratanaburee, A., Kantachote, D., Charernjiratrakul, W., Penjamras, P., & Chaiyasut, C. (2011). Enhancement of γ-aminobutyric acid in a fermented red seaweed beverage by starter culture Lactobacillus plantarum DW12. Electronic Journal of Biotechnology, 14, 1–14. doi:10.2225/vol14-issue3-fulltext-2
- Soltani, N., Qiu, H., Aleksic, M., Glinka, Y., Zhao, F., Liu, R., … Wang, Q. (2011). GABA exerts protective and regenerative effects on islet beta cells and reverses diabetes. Proceedings of the National Academy of Sciences, 108, 11692–11697. doi:10.1073/pnas.1102715108
- Ueno, H. (2000). Enzymatic and structural aspects on glutamate decarboxylase. Journal of Molecular Catalysis B: Enzymatic, 10, 67–79. doi:10.1016/S1381-1177(00)00114-4
- Vaiva, G., Thomas, P., Ducrocq, F., Fontaine, M., Boss, V., Devos, P., … Goudemand, M. (2004). Low posttrauma GABA plasma levels as a predictive factor in the development of acute posttraumatic stress disorder. Biological Psychiatry, 55, 250–254. doi:10.1016/j.biopsych.2003.08.009
- Vermeulen, N., Gänzle, M. G., & Vogel, R. F. (2007). Glutamine deamidation by cereal-associated lactic acid bacteria. Journal of Applied Microbiology, 103, 1197–1205. doi:10.1111/j.1365-2672.2007.03333.x
- Vijayalakshmi, P., & Sarvamangala, D. (2011). Fermentative production of L-glutamic acid. International Journal of Advanced Biotechnology and Research, 2, 376–381.
- Weingand-Ziadé, A., Gerber-Décombaz, C., & Affolter, M. (2003). Functional characterization of a salt- and thermotolerant glutaminase from Lactobacillus rhamnosus. Enzyme and Microbial Technology, 32, 862–867. doi:10.1016/S0141-0229(03)00059-0
- Wong, K. H., Sabaratnam, V., Abdullah, N., Kuppusamy, U. R., & Naidu, M. (2009). Effects of cultivation techniques and processing on antimicrobial and antioxidant activities of Hericium erinaceus (Bull.: Fr.) Pers. extracts. Food Technology and Biotechnology, 47, 47–55.
- Woraharn, S., Lailerd, N., Sivamaruthi, B. S., Wangcharoen, W., Sirisattha, S., & Chaiyasut, C. (2014). Screening and kinetics of glutaminase and glutamate decarboxylase producing lactic acid bacteria from fermented Thai foods. Food Science and Technology (Campinas), 34, 793–799. doi:10.1590/1678-457X.6519
- Yavari, N., Assadi, M. M., Moghadam, M. B., & Larijani, K. (2011). Optimizing glucuronic acid production using tea fungus on grape juice by response surface methodology. Australian Journal of Basic and Applied Sciences, 5, 1788–1794.
- Yoshimura, M., Toyoshi, T., Sano, A., Izumi, T., Fujii, T., Konishi, C., … Obata, A. (2010). Antihypertensive effect of a γ-aminobutyric acid rich tomato cultivar ‘DG03-9’ in spontaneously hypertensive rats. Journal of Agricultural and Food Chemistry, 58, 615–619. doi:10.1021/jf903008t
- Yuasa, A., Okamura, H., & Kataoka, J. (2003). U.S. Patent No. 2003/0124646 A1. Washington, DC: U.S. Patent and Trademark Office.
- Zareian, M., Ebrahimpour, A., Mohamed, A., & Saari, N. (2013). Modeling of glutamic acid production by Lactobacillus plantarum MNZ. Electronic Journal of Biotechnology, 4. doi:10.2225/vol16-issue4-fulltext-10
- Zimmerman, F. T., Burgemeister, B. B., & Putnam, T. J. (1946). Effect of glutamic acid on mental functioning in children and in adolescents. Archives of Neurology and Psychiatry, 6, 489–506. doi:10.1001/archneurpsyc