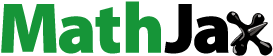
ABSTRACT
Our previous study found that ferric citrate-loaded liposome (FAC-LIP) exhibited stable physical characteristics and have better efficacy to treat anemia of inflammation (AI) than free ferric citrate (FAC). This study was aimed at oral pharmacokinetics of both FAC-LIP and FAC in Sprague Dawley (SD) rats. Results showed that the main pharmacokinetic parameters of FAC-LIP in vivo were as follows: Cmax of 445.89 ± 23.56 μg/mL, Tmax of 4 h, t1/2 of 13.01 ± 2.04 h, MRT of 20.54 ± 2.06 h and AUC0-24 h of 6446.1 ± 516.58 hμg/mL. The relative bioavailability of FAC-LIP formulation was 215.7%, nearly 2-fold in comparison with free FAC formulation. FAC-LIP showed a sustained-release effect, a wide absorption and a complete elimination. These results support the fact that FAC-LIP has the potential of improving oral bioavailability and reducing iron accumulation in liver, which could influence the available iron level in the blood system. FAC-LIP could be used as an effective iron supplement.
RESUMEN
Nuestro estudio previo encontró que el liposoma cargado de citrato férrico (FAC-LIP) exhibió unas características físicas estables y una mayor eficacia para tratar la anemia de la inflamación (AI) en comparación con el citrato férrico solo (FAC). Este estudio investiga la farmacocinética oral de FAC-LIP y FAC en los ratones de laboratorio Sprague Dawley (SD). Los resultados mostraron que los principales parámetros farmacocinéticos de FAC-LIP in vivo fueron los siguientes: Cmax de 445,89±23,56 μg/mL, Tmax de 4 h, t1/2 de 13,01±2,04 h, MRT de 20,54±2,06 h y AUC0-24h de 6446,1±516,58 hμg/mL. La biodisponibilidad relativa de la formulación FAC-LIP fue 215,7%, cerca del doble en comparación con la formulación con citrato férrico solo (FAC). FAC-LIP mostró un efecto sostenido-liberado, una amplia absorción y una eliminación completa. Estos resultados apoyan el hecho que FAC-LIP tiene el potencial de mejorar la biodisponibilidad oral y reducir la acumulación de hierro en el hígado, lo cual podría influenciar el nivel de hierro disponible en el sistema sanguíneo. FAC-LIP podría ser utilizado como un efectivo complemento férrico.
PALABRAS CLAVE:
1. Introduction
Anemia of inflammation (AI), also named anemia of chronic disease (ACD), occurs in hospitalized patients with acute and chronic immune activation and shows a significant clinical problem (Erwin, Peter, Elizabeta, Hans, & Dorine, Citation2005). Especially, AI is seen in the setting of infectious, inflammatory and neoplastic diseases. It partly results from changes in the intracellular metabolism of iron (Jurado, Citation1997). As we all know, iron is vital for microbes, plants and higher animals, and is present as heme and nonheme iron in the diet (Miret, Simpson, & McKie, Citation2003). In humans, iron inorganic moves between the ferrous (Fe2+) and ferric (Fe3+) oxidation states reversibly. It is recycled efficiently from hemoglobin of senescent red blood cells (about 20 mg/day), mainly ending up in new red blood cells. A smaller amount of iron from myoglobin and redox enzymes are also recycled, for the purpose of conserving iron rigorously (Ganz, Citation2005). In order to relieve the symptoms of AI, it is essential to recognize the importance of iron supplements.
Recently, different iron supplements have been researched and developed to mitigate the situation of iron deficiency. These iron supplements included Fe (II) sulfate agent (Ferrari, Nicolini, Manca, Rossi, & Anselmi, Citation2012), ferric citrate (FAC) formulation (Bates, Billups, & Saltman, Citation1967), ferrous-based combination product (Ferall) (Liu, Lin, Chang, Yang, & Chen, Citation2004), heme-iron fortificant (Cao, Thomas, Insogna, & O’Brien, Citation2014), polysaccharide iron complex (Dubiel, Cieslak, Alenkina, Oshtrakh, & Semionkin, Citation2014; Wang et al., Citation2008; Zhang, Liu, Su, Lui, & Li, Citation2009), iron amino acid chelate (Ding et al., Citation2011; Mimura, Breganó, Dichi, Gregório, & Dichi, Citation2008), ferric citrate-loaded liposomes (FAC-LIP) (Yuan et al., Citation2013) and amino acid chelated iron liposomes (Ding et al., Citation2011). Among these iron supplements, iron liposomes have gained considerable attention for its role in the treatment of AI. Liposome is a kind of artificial lipid aggregates that composed of a spherical bilayer arrangement of amphiphiles encasing an aqueous inner core (Hansen, Lomholt, Hansen, Mouritsen, & Arouri, Citation2016). Liposome technology, which is a special kind of microencapsulation technique, promises various applications as a delivery system in many fields such as cosmetic, nutrition, food and drug (Betz, Aeppli, Menshutina, & Leuenberger, Citation2005; Guo et al., Citation2013; Keller, Citation2001; Suzuki et al., Citation2008). Liposomes can improve the drug pharmacokinetics as well as reduce off-target toxicities (Barenholz, Citation2012). Additionally, because of the well characterization, easily preparation, highly utility in their carrier properties, highly biocompatibility and relatively safe materials (Ding, Xia, Hayat, & Zhang, Citation2009; Xia & Xu, Citation2005), liposomes are particularly well suited for use in food and drug industry. In this case, iron liposomes were obtained by our previous study (Yuan et al., Citation2013). It must be noted that FAC formulation, as an inorganic iron agent, is mainly stored in the intestine and macrophages. And its metabolism is regulated by hepcidin and iron export protein ferroportin (FPN1) (Nemeth & Ganz, Citation2009; Nemeth et al., Citation2004). Moreover, compared with non-encapsulated FAC, liposome encapsulation might be a highly effective way to improve drug solubility, to promote drug absorption and to enhance bioavailability.
The preparation, characterization and pharmacological effects of both FAC-LIP and heme liposome (HEME-LIP) had been researched in the previous study (Yuan et al., Citation2013). However, little has been studied in details on the oral pharmacokinetics and tissue distribution of FAC-LIP, which exhibited higher available iron level than HEME-LIP in system circulation. The study was therefore aimed at verifying the pharmacokinetic process of FAC-LIP, which have sustained-release effect, great bioavailability and no obvious toxic effect. Besides, the tissue distribution of FAC-LIP was investigated in normal Sprague Dawley (SD) rats compared with free FAC. All these researches were targeted at providing support for further development of FAC-LIP on the treatment of AI.
2. Materials and methods
2.1. Chemicals and materials
The iron standards were obtained from National Center of Analysis and Testing for Nonferrous Metals and Electronic Materials (NCATN) (Beijing, China). The FAC with a purity of 98% was purchased from Zhengzhou Dragon and Chemical Industry Co., Ltd. (Zhengzhou, Henan, China). All solvents and other chemicals used in this work were of analytical grades and obtained from Sinopharm Chemical Reagent Co., Ltd. (Shanghai, China).
2.2. Preparation of FAC-LIP
FAC-LIP was prepared according to the method described by our previous work (Yuan et al., Citation2013). A proportion of cholesterol and lecithin were added to an ethanol solution. FAC dissolved in PBS solution (pH = 6.5), polyglycerol 10 stearic acid ester and glass beads (3–4 mm diameter) were added to the system after removing the organic solvent by rotary evaporator and filling with N2 for 120–200 min. The mixture was allowed to rotate continually to obtain a lipid film suspension. After dispersed by ultrasonication and cooled in an ice-water bath, the liposomes were filtered through 0.44 μm and 0.22 μm membranes successively. Finally the obtained liposomes were stored in a brown bottle filled with N2 at 4°C. Encapsulation efficiency, microstructure, liposome size, zeta potential, in vitro release experiment and iron supplementation efficacy of FAC-LIP were analyzed by the method that we established before (Yuan et al., Citation2013).
2.3. Inductively coupled plasma optical emission spectrometer (ICP-OES) analysis
In the selection of blank solution, iron standard solution was dissolved in water, 1%, 1.5%, 2%, 2.5%, 3%, 4%, 5%, 6%, 8%, 10%, 20% and 30% HNO3. The results showed that acid concentrations (≤10%) can be used to determine the iron content. In this paper, 2.5% HNO3 was used as a blank solution.
When samples were already taken, all fresh animal samples (including blood, organs and excrements samples) were dissolved with 15 mL mixture of concentrated HNO3 and concentrated HClO4 (v/v, 4:1) and then digested 12 h at room temperature. The samples were heated at 200–250°C until the remaining solution became clear and transparent. Acid was volatilized twice after adding double distilled water. After cooling, the remaining solution was transferred into Eppendorf tubes, and then 2.5% HNO3 was added to increase the final volume of the solution to 10 mL. Fe level in samples was analyzed by the method of Ren et al. (Citation2013) with slight modification. The operating conditions for Vista-MPX Simultaneous Inductively coupled plasma optical emission spectrometer (ICP-OES) (Varian, Inc. U.S.A.) were listed as following: spectral range 175–785 (nm), temperature 35 (°C), resolution ≤0.009 (nm), power range 700–1500 (W) and step width ≤50 (W). Each sample was measured three times.
2.4. Method validation
The calibration curve was established with a series of the different concentrations of iron standard solution (linear ranging from 0 to 102 μg/mL) which were determined by the same spectral conditions as described above. With the spectral intensity as vertical axis (I) and the iron concentration as the horizontal axis (C), regression analysis was used to ascertain the standard curve equation. The sensitivity, precision, stability, recovery and repeatability of the assay were evaluated by analyzing the spectral intensity of the samples representing the low, medium and high concentrations of the standard curves (10, 50 and 100 μg/mL, respectively) in order to ascertain the assay reliability.
2.5. Oral pharmacokinetics and tissue distribution study
2.5.1. Experimental animals
Male SD rats with 250 ± 20 g of body mass were selected from the Laboratory Animal Center of Jiangsu University, China (the license number: SCXK (SU) 2013–0011). All the animals were kept in polypropylene cages and maintained under a 12 h light/dark cycle at a controlled temperature (21 ± 1°C) and humidity (60 ± 5%). They were fed with the standard pellet diet and water ad libitum. The rats were adapted to laboratory conditions for 3 days before commencement of experiment. The author of this research obtained the Jiangsu Province post certificate on experimental animals (the license number: 2131989) in China. All procedures were carried out in accordance with the guidelines involving the use of animals which was issued by the Ethic Committee of Jiangsu University.
2.5.2. Preparation of animal samples
Ninety-six experimental rats were randomly and equally divided into FAC-LIP group and FAC group, respectively. All rats were fasted for 12 h prior to dosing but were allowed free access to drinking water. FAC group (reference group): treated with 27.58 mg/kg body mass by oral administration; FAC-LIP group (test group): treated with a dosage of 316.9 mg/kg body mass by oral administration. About 0.5 mL blood samples were collected before oral administration and at 15, 30, 45 min, 1, 1.25, 1.5, 2, 3, 4, 5, 6, 8, 12, 16, 24 h by the retro-orbital route after oral administration (Yi et al., Citation2013). The blood samples were centrifuged at 4000 g for 10 min at 4°C to separate the serum from the cellular components.
The process of tissue distribution was successively explored after removal of organs in rats (including hearts, livers, spleens, lungs, kidneys, duodenums and brains) before oral administration and at 0.5, 1, 2, 4, 6, 12 and 24 h. The excrements of rats were also collected for further assay. A total of 72 SD rats were killed by cervical dislocation before organ harvesting.
All the samples in the experiment were carefully collected without any contamination and stored at −20°C until analyzed.
2.5.3. Analysis of pharmacokinetic parameters
The pharmacokinetic parameters of FAC-LIP and FAC, namely, the maximum concentration in serum (Cmax, μg/mL), the time to attain the maximum concentration (Tmax, h), elimination half-life (t1/2, h) and the area under the concentration-time curve (AUC0-24 h, hμg/mL), were analyzed using BAPP 2.3 pharmacokinetic software (supplied by the Center of Drug Metabolism of China Pharmaceutical University, China) (Cao et al., Citation2013). The relative bioavailability (F) of FAC-LIP and FAC were determined by calculating the ratio of AUC for the test formulation (AUCT) and that for the reference formulation (AUCR) according to the following equation:
where DR and DT represent the reference and test drug dosage, respectively.
2.5.4. Statistical analysis
All values were presented as means ± SD (standard deviation). Statistical analyses were carried out using SPSS version 16.0 (SPSS Inc, Chicago, U.S.A.). The p-value of less than 0.05 was considered significant.
3. Results and discussion
3.1. ICP-OES assay validation
The analytical performance of ICP-OES analysis depended on the atoms used to access. In the study, the linear regression equation of iron was I = 2.03 C (ng/mL), R2 = 0.9999, indicating a good linear relationship with the spectral intensity. The limits of detection (LOD) and quantification (LOQ) measured for the replicates of the iron standard solutions, was about 5.40 and 17.99 ng/ml, respectively. The interday and intraday precision (RSD%) in vitro ranged from 1.75% to 9.52% at the three different concentrations, which could be compared with other current study (Lech, Citation2014). The RSD% of iron was determined ranging from 1.54% to 5.84%, which support the fact that the samples were stable within 24 h. Similarly, the RSD% of the samples was below 6.00%, which means that the assay for the study was repeatable within 24 h. All the acceptance criteria were met with recoveries within the range of 98.30–117.22% both in vitro and in vivo. It was noteworthy that the ICP-OES analysis even though was simple, but yet the effective method to analyze iron both in vitro and in vivo.
3.2. Oral pharmacokinetics and bioavailability study
In comparison to the empty liposome that we reported (Yuan et al., Citation2013), the liposomal FAC has a slightly higher particle size (150 nm). Moreover, the encapsulation efficiency of FAC-LIP (35%) indicated that the bulk of FAC had been successfully entrapped into liposomes. These properties might contribute to the enhanced absorption of FAC in vivo.
The serum concentrations of iron at different time points were detected after oral administration of FAC-LIP and free FAC. This preliminary pharmacokinetics study was limited to six rats per experimental group because of ethical reasons, which showed marked variability.
The mean blood concentration-time profiles of Fe after oral delivery of FAC-LIP and free FAC to SD rats at 5.83 mg/kg (Fe content) were shown in . The dosage was determined by the preexperiment. The relevant pharmacokinetic parameters of FAC-LIP and free FAC in serum were calculated and presented in . With respect to free FAC, the value of Cmax was only 534.97 ± 46.90 μg/ml, which was different from that in FAC-LIP (445.89 ± 23.56 μg/ml, p < 0.05, one-way ANOVA analysis). Tmax values of FAC and FAC-LIP were approximately 0.75 and 4 h, respectively. Interestingly, the mean of AUC0-24 h in serum for FAC-LIP was found to be 6446.1 ± 516.58 hμg/ml, which was nearly 2-fold higher than that of free FAC (p < 0.01). It was noteworthy that the relative bioavailability of FAC-LIP was increased to 215.7% compared with FAC. In addition, the elimination half-life (t1/2) values of FAC-LIP and free FAC were 13.01 ± 2.04 and 5.58 ± 0.86 h, respectively. In summary, all pharmacokinetic parameters of FAC-LIP indicated that liposomes could increase the Fe concentration in circulatory system, promote the absorption of Fe and exhibit the property of sustained release in vivo, which were in agreement with other related studies (Chu et al., Citation2011; Xiao, Song, Chen, & Ping, Citation2006).
Table 1. Main pharmacokinetic parameters of ferric citrate (FAC) and its liposome formulation (FAC-LIP), ap<0.01, bp<0.05 vs. free FAC (mean ± SD, n = 6).
Tabla 1. Principales parámetros farmacocinéticos de la formulación citrato férrico (FAC) y su liposoma (FAC-LIP), ap<0,01, bp<0,05 frente a FAC solo (promedio ± SD, n = 6).
Figure 1. Mean plasma concentration-time curves of Fe after oral administration of FAC and FAC-LIP formulations (equivalent to 5.83 mg/kg Fe) in healthy SD rats (mean ± SD, n = 6).
Figura 1. Promedio de las curvas concentración-tiempo de Fe en el plasma después de la administración oral de las formulaciones FAC y FAC-LIP (equivalente a 5,83 mg/kg de Fe) en los ratones SD saludables (promedio±SD, n = 6).
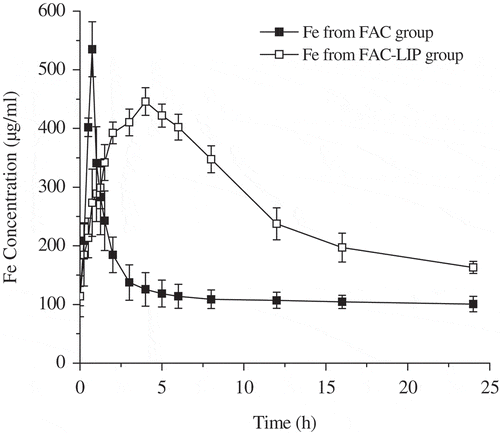
As shown in , the liposomal encapsulation provided a delayed release in circulatory system. However, the concentration of Fe in serum drastically increased from approximately 0.75 h and maintained a high concentration until nearly 12 h after oral administration of FAC-LIP. All these results implied that the prepared liposome, used as a promising drug carrier, could maintain a sustained-release effect and obviously exist in the blood circulation for a significantly longer time period than free FAC. It was therefore not surprising that FAC-LIP could protect drugs from the hostile gastrointestinal environment in liposome delivery system (Han, Shin, & Dong, Citation2012). Furthermore, most Fe could be released from intestinal enterocytes and macrophages into the bloodstream in order to improve the recycling of Fe (Chen et al., Citation2009). Huynh, Passirani, Saulnier, and Benoit (Citation2009) confirmed that liposomal formulation can be delivered via membrane fusion and diffusion and can dramatically enhance the Fe level in circulatory system. Remarkably, the lipid peroxidation level of FAC-LIP is an important indicator of whether it is toxic or not. Obviously, some related studies including our previous research (Vianabaracioli et al., Citation2011; Yuan et al., Citation2013) confirmed that the liposomal encapsulation was not obvious toxic, from the measurement of the malondialdehyde (MDA) level and superoxide dismutase (SOD) activity. However, the mechanism of oral bioavailability enhancement of FAC-LIP will be further elucidated.
3.3. Tissue distribution
The investigation of tissue distribution of FAC-LIP and free FAC groups was conducted in healthy SD rats. From the tissue distribution results and the corresponding mean tissue weight, the concentration of Fe in each tissue per wet tissue weight (μg/g tissue) at different time points was calculated. These values were presented in .
Figure 2. Mean tissue Fe concentrations in free FAC and FAC-LIP at the exact same time (a-0.5 h; b-1 h; c-2 h; d-4 h; e-6 h; f-12 h; g-24 h) after oral administration in SD rats. △p < 0.01, △△p < 0.05 vs. Fe concentration before administration; *p < 0.01, **p < 0.05 vs. FAC group (mean ± SD, n = 6).
Figura 2. Promedio de las concentraciones de Fe en el tejido en FAC y FAC-LIP solo al mismo tiempo exacto (a-0,5 h; b-1 h; c-2 h; d-4 h; e-6 h; f-12 h; g-24 h) después de la administración oral en ratones SD. △p < 0,01, △△p < 0,05 frente a la concentración de Fe antes de la administración; *p < 0,01 **p < 0,05 frente al grupo FAC (promedio±SD, n = 6).
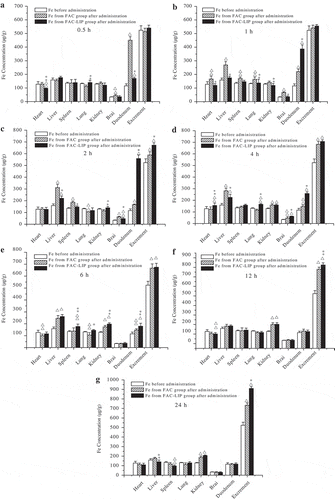
The comparison of Fe concentrations between FAC-LIP and free FAC groups at 0.5 h was shown in (a). Compared with the Fe level before administration, free FAC group yielded a significantly increase (p < 0.01) and even a significantly higher (p < 0.01) than the Fe level of FAC-LIP group in the duodenum, indicating that FAC exhibited a great absorption in a short period of 0.5 h. Additionally, iron supplement is mainly adsorbed in duodenum (pH 6–8). Solubility of iron supplement in duodenum (pH 6–8) is one of the main factors of bioavailability evaluation. In the previous vitro release experiment (Yuan et al., Citation2013), FAC-LIP was well dissolved in simulated intestinal fluid (SIF, pH = 7.5). The result indicated that FAC-LIP was stable in a physiological pH solution; therefore, it was also conducive to the absorption of Fe.
As shown in (b), the Fe concentration of FAC-LIP group was obviously higher (p < 0.01) than that of FAC group in the duodenum. In a short period of 1 h, the Fe of FAC group was progressively distributed in most of the tissues (except kidney). Furthermore, at the time point of 1 h, FAC group exhibited significant accumulation (p < 0.01 vs. Fe level before administration) in the liver.
(c) presented that, at 2 h after administration, the Fe level of FAC group were much more concentrated in the liver than any other related organ such as heart, lung, kidney and brain. Then, significantly higher Fe (p < 0.01) was concentrated in the spleen at the same time point, in comparison with the group before administration. Furthermore, in duodenum distribution, the Fe level of FAC-LIP group at 2 h exhibited the highest among all the time points, indicating that the absorption of Fe reached a maximum. These results were obviously consistent with the absorption of Fe in circulatory system in previous pharmacokinetic study. At the time point of 2 h, both FAC-LIP and free FAC groups appeared to significantly elimination (p < 0.01 vs. Fe level before administration), while the Fe elimination of FAC-LIP group was obviously more (p < 0.01) than that of FAC group.
Generally, after 4 h of oral administration of FAC-LIP, the Fe level was obviously higher than that of FAC, indicating that the distribution of FAC-LIP in rat organs usually lagged behind FAC. Remarkably, Fe levels in these two groups appeared to decrease at the period of 4 h ((d)). In brain distribution, the Fe level of FAC-LIP group was significantly lower (p < 0.01) than that of FAC group in a period of 1, 2 and 4 h. The reason for this phenomenon was probably associated with the blood–brain barrier, which mechanism was reported in details in other study (Tsai & Chien, Citation2011).
Iron was mainly stored in liver and spleen in the form of ferritin and haemosiderin. According to (e),FAC-LIP group appeared to significantly accumulation (p < 0.01 vs. Fe level before administration) in the liver at the time point of 6 h, but no significant difference between this group and FAC group. Furthermore, significantly higher (p < 0.01) Fe was concentrated in the liver from the time point of 1 to even 6 h. Moreover, in liver distribution, the Fe level of FAC-LIP group in a period of 1, 2 and 4 h was significantly lower (p < 0.01) than that of FAC group at the same time. The FAC-LIP group exhibited significant elimination (p < 0.05) at 24 h than FAC group ((g)). Generally, free FAC increased more iron retention and accumulation than FAC-LIP in liver, which is the main storage organ of Fe. All of these factors can reduce the available Fe level in the blood system. After that, a similar conclusion was defined after analyzing the Fe level of both drugs in spleen. Furthermore, at the time point of 6 h, the distribution of Fe levels of FAC group in heart and lung was significantly lower (p < 0.01 vs. Fe level before administration). This result affirmed the consumption and insufficient supply of Fe in FAC group after approximately 6 h.
As presented in (c and f), in excrement, the Fe level of FAC-LIP group was higher than that of free FAC group in a period of 2 and 12 h. Additionally, at the time point of 24 h ((g)), approximately 1122.7 μg of Fe in the excrement per excrement weight (μg/g excrement) was cumulative for FAC-LIP, almost 1.24-fold in comparison with Fe concentration per excrement weight for FAC. All the observations above implied that FAC-LIP could almost achieve complete elimination, which was in accordance with other studies (Zhao et al., Citation2011). However, in combination with the absorption, distribution, metabolism process, its mechanism of elimination should be further investigated. In addition, the kidney is a vital organ of the body, whose basic function is to generate the urine in order to remove certain metabolites and wastes, while retaining moisture and other useful substances by reabsorption. Thus, it was not surprising that both FAC-LIP and free FAC groups partly exhibited elimination from approximately 4 h (p < 0.01) in kidney distribution ((d,e,f)). The metabolism of kidney reabsorption needs to be further investigated.
Taken together, all of the observations above indicated that the liposomal FAC could improve both the bioavailability and accumulated in the liver and also keep the sustained release in comparison with the free FAC. It is important to note the decreased iron concentration in the liver of FAC-LIP group during the first 4 h and the rapid elimination in the procedure after oral administration. Drug distribution in tissues, which got a relationship with the bioavailability, was mainly influenced by its concentration in target organs. In contrast, the higher serum concentration of iron in FAC-LIP group could obviously enhance the oral bioavailability because of the close relationship between them. Therefore, encapsulated in liposome for improved the bioavailability, the FAC has potential to serve as a novel nutraceutical agent.
4. Conclusions
In summary, the prepared FAC-LIP was successfully utilized in the pharmacokinetic test and the tissue distribution studies. The pharmacokinetic parameters of FAC-LIP have been calculated by BAPP 2.3 pharmacokinetic software. Namely, the value of Cmax was 445.89 ± 23.56 μg/mL, Tmax value was 4 h, t1/2 value was 13.01 ± 2.04 h, MRT value was 20.54 ± 2.06 h and the mean of AUC0-24 h was 6446.1 ± 516.58 hμg/mL. The relative bioavailability of this formulation was 215.7%, in comparison with free FAC. Thus, FAC-LIP could maintain a sustained-release effect and remain a significantly higher drug concentration than free FAC. The tissue distribution study showed that FAC-LIP could be absorbed widely and eliminated rapidly from the body. Furthermore, FAC-LIP could effectively reduce the liver burden caused by iron accumulation. All our findings revealed that liposomes with inorganic iron were superior to free iron agent in the treatment of AI.
Acknowledgments
This work was supported financially by the National Natural Science Foundation of China (31340008,31201305) and Open Project of State Key Laboratory of Food Science and Technology, Nanchang University (SKLF-KF-201410). This work also was funded by youth backbone teacher training project of Jiangsu University (2012) and the priority academic program development of Jiangsu higher education institutions (PAPD). The preparation of the iron liposomes was assisted by Prof. Yanzhong Chang (the Key Lab of Physiology Biochemistry & Molecular Biology of Hebei Province, Hebei Normal University).
Disclosure statement
No potential conflict of interest was reported by the authors.
Additional information
Funding
References
- Barenholz, Y.C. (2012). Doxil®-the first FDA-approved nano-drug: Lessons learned. Journal of Controlled Release, 160, 117–134. doi:10.1016/j.jconrel.2012.03.020
- Bates, G.W., Billups, C., & Saltman, P. (1967). The kinetics and mechanism of iron (III) exchange between chelates and transferrin I. The complexes of citrate and nitrilotriacetic acid. Journal of Biology Chemistry, 242, 2810–2815.
- Betz, G., Aeppli, A., Menshutina, N., & Leuenberger, H. (2005). In vivo comparison of various liposome formulations for cosmetic application. International Journal of Pharmaceutics, 296, 44–54. doi:10.1016/j.ijpharm.2005.02.032
- Cao, C., Thomas, C., Insogna, K., & O’Brien, K. (2014). Duodenal expression of heme and non-heme iron transporters and tissue partitioning of dietary heme and non-heme iron in a rat mode of iron overload. Journal of Neurochemistry, 102, 1831–1841. doi:10.1096/fj.1530-6860
- Cao, X., Deng, W., Fu, M., Zhu, Y., Liu, H., Wang, L., … Yu, J. (2013). Seventy-two-hour release formulation of the poorly soluble drug silybin based on porous silica nanoparticles: In vitro release kinetics and in vitro/in vivo correlations in beagle dogs. European Journal of Pharmaceutical Sciences, 48, 64–71. doi:10.1016/j.ejps.2012.10.012
- Chen, Y., Lu, Y., Chen, J., Lai, J., Sun, J., Hu, F., & Wu, W. (2009). Enhanced bioavailability of the poorly water-soluble drug fenofibrate by using liposomes containing a bile salt. International Journal of Pharmaceutics, 376, 153–160. doi:10.1016/j.ijpharm.2009.04.022
- Chu, C., Tong, S., Xu, Y., Wang, L., Fu, M., Ge, Y., … Xu, X. (2011). Proliposomes for oral delivery of dehydrosilymarin: Preparation and evaluation in vitro and in vivo. Acta Pharmacologica Sinica, 32, 973–980. doi:10.1038/aps.2011.25
- Ding, B., Xia, S., Hayat, K., & Zhang, X. (2009). Preparation and pH stability of ferrous glycinate liposomes. Journal of Agriculture and Food Chemistry, 57, 2938–2944. doi:10.1021/jf8031205
- Ding, B., Zhang, X., Hayat, K., Xia, S., Jia, C., Xie, M., & Liu, C. (2011). Preparation, characterization and the stability of ferrous glycinate nanoliposomes. Journal of Food Engineering, 102, 202–208. doi:10.1016/j.jfoodeng.2010.08.022
- Dubiel, S., Cieslak, J., Alenkina, I., Oshtrakh, M., & Semionkin, V. (2014). Evaluation of the Debye temperature for iron cores in human liver ferritin and its pharmaceutical analogue Ferrum Lek using Mossbauer spectroscopy. Journal of Inorganic Biochemistry, 140, 89–93. doi:10.1016/j.jinorgbio.2014.07.005
- Erwin, K., Peter, P., Elizabeta, N., Hans, V.D.H., & Dorine, S. (2005). Time-course analysis of hepcidin, serum iron, and plasma cytokine levels in humans injected with LPS. Blood, 106, 1864–1866. doi:10.1182/blood-2005-03-1159
- Ferrari, P., Nicolini, A., Manca, M.L., Rossi, G., Anselmi, L., Conte, M., … Bonino, F. (2012). Treatment of mild non-chemotherapy-induced iron deficiency anemia in cancer patients: Comparison between oral ferrous bisglycinate chelate and ferrous sulfate. Biomedicine & Pharmacotherapy, 66, 414–418. doi:10.1016/j.biopha.2012.06.003
- Ganz, T. (2005). Hepcidin–A regulator of intestinal iron absorption and iron recycling by macrophages. Best Practice & Research Clinical Haematology, 18, 171–182. doi:10.1016/j.beha.2004.08.020
- Guo, L., Chen, J., Qiu, Y., Zhang, S., Xu, B., & Gao, Y. (2013). Enhanced transcutaneous immunization via dissolving microneedle array loaded with liposome encapsulated antigen and adjuvant. International Journal of Pharmaceutics, 447, 22–30. doi:10.1016/j.ijpharm.2013.02.006
- Han, H., Shin, H., & Dong, H. (2012). Improved oral bioavailability of alendronate via the mucoadhesive liposomal delivery system. European Journal of Pharmaceutical Sciences, 46, 500–507. doi:10.1016/j.ejps.2012.04.002
- Hansen, A.H., Lomholt, M.A., Hansen, P.L., Mouritsen, O.G., & Arouri, A. (2016). Optimization and modeling of the remote loading of luciferin into liposomes. International Journal of Pharmaceutics, 508, 128–134. doi:10.1016/j.ijpharm.2016.04.055
- Huynh, N., Passirani, C., Saulnier, P., & Benoit, J. (2009). Lipid nanocapsules: A new platform for nanomedicine. International Journal of Pharmaceutics, 379, 201–209. doi:10.1016/j.ijpharm.2009.04.026
- Jurado, R.L. (1997). Iron, infections, and anemia of inflammation. Clinical Infectious Diseases, 25, 888–895. doi:10.1086/515549
- Keller, B.C. (2001). Liposomes in nutrition. Trends in Food Science & Technology, 12, 25–31. doi:10.1016/S9024-2244(01)00044-9
- Lech, T. (2014). ICP OES and CV AAS in determination of mercury in an unusual fatal case of long-term exposure to elemental mercury in a teenager. Forensic Science International, 237, e1–e5. doi:10.1016/j.forsciint.2014.02.015
- Liu, T., Lin, S., Chang, C., Yang, W., & Chen, T. (2004). Comparison of a combination ferrous fumarate product and a polysaccharide iron complex as oral treatments of iron deficiency anemia: A Taiwanese study. International Journal of Hematology, 80, 416–420. doi:10.1532/IJH97.A10409
- Mimura, É.C.M., Breganó, J.W., Dichi, J.B., Gregório, E.P., & Dichi, I. (2008). Comparison of ferrous sulfate and ferrous glycinate chelate for the treatment of iron deficiency anemia in gastrectomized patients. Nutrition, 24, 663–668. doi:10.1016/j.nut.2008.03.017
- Miret, S., Simpson, R.J., & McKie, A.T. (2003). Physiology and molecular biology of dietary iron absorption. Annual Review of Nutrition, 23, 283–301. doi:10.1146/annurev.nutr.23.011702.073139
- Nemeth, E., & Ganz, T. (2009). The role of hepcidin in iron metabolism. Acta Haematologica, 122, 78–86. doi:10.1159/000243791
- Nemeth, E., Rivera, S., Gabayan, V., Keller, C., Taudorf, S., Pedersen, B.K., Ganz, T. (2004). IL-6 mediates hypoferremia of inflammation by inducing the synthesis of the iron regulatory hormone hepcidin. Journal of Clinical Investigation, 113, 1271–1276. doi:10.1172/JCI200420945
- Ren, Y., Zhao, T., Mao, G., Zhang, M., Li, F., Zou, Y., … Wu, X. (2013). Antitumor activity of hyaluronic acid–selenium nanoparticles in Heps tumor mice models. International Journal of Biological Macromolecules, 57, 57–62. doi:10.1016/j.ijbiomac.2013.03.014
- Suzuki, R., Takizawa, T., Kuwata, Y., Mutoh, M., Ishiguro, N., Utoguchi, N., … Maruyama, K. (2008). Effective anti-tumor activity of oxaliplatin encapsulated in transferrin-PEG-liposome. International Journal of Pharmaceutics, 346, 143–150. doi:10.1016/j.ijpharm.2007.06.010
- Tsai, Y.M., & Chien, C.F. (2011). Curcumin and its nano-formulation: The kinetics of tissue distribution and blood-brain barrier penetration. International Journal of Pharmaceutics, 416, 331–338. doi:10.1016/j.ijpharm.2011.06.030
- Vianabaracioli, L.M., Tukamoto Junior, N.C., Ricci, J.O., Mattos, L.C., Ângulo, I.L., & Boninidomingos, C.R. (2011). Comparison of oxidative stress and the frequency of polymorphisms in the hfe gene between hemoglobin s trait blood donors and sickle cell disease patients. Genetics & Molecular Research GMR, 10, 3446–3454. doi:10.4238/2011.December.8.4
- Wang, K.P., Chen, Z.X., Zhang, Y., Wang, P.P., Wang, J.H., & Dai, L.Q. (2008). Molecular weight and proposed structure of the angelica sinensis polysaccharide-iron complex. Chinese Journal of Chemistry, 26, 1068–1074. doi:10.1002/cjoc.200890189
- Xia, S., & Xu, S. (2005). Ferrous sulfate liposomes: Preparation, stability and application in fluid milk. Food Research International, 38, 289–296. doi:10.1016/j.foodres.2004.04.010
- Xiao, Y., Song, Y., Chen, Z., & Ping, Q. (2006). The preparation of silybin-phospholipid complex and the study on its pharmacokinetics in rats. International Journal of Pharmaceutics, 307, 77–82. doi:10.1016/j.ijpharm.2005.10.001
- Yi, C., Fu, M., Cao, X., Tong, S., Zheng, Q., Firempong, C.K., … Yu, J. (2013). Enhanced oral bioavailability and tissue distribution of a new potential anticancer agent, Flammulina velutipes sterols, through liposomal encapsulation. Journal of Agricultural and Food Chemistry, 61, 5961–5971. doi:10.1021/jf3055278
- Yuan, L., Geng, L., Ge, L., Yu, P., Duan, X., Chen, J., & Chang, Y. (2013). Effect of iron liposomes on anemia of inflammation. International Journal of Pharmaceutics, 454, 82–89. doi:10.1016/j.ijpharm.2013.06.078
- Zhang, Y.Y., Liu, J.H., Su, F., Lui, Y.T., & Li, J.F. (2009). Single-dose bioequivalence assessment of two formulations of polysaccharide iron complex capsules in healthy adult male Chinese volunteers: A sequence-randomized, double-blind, two-way crossover study. Current Therapeutic Research, 70, 104–115. doi:10.1016/j.curtheres.2009.04.006
- Zhao, Y.Y., Cheng, X.L., Liu, R., Ho, C.C., Wei, F., Yan, S.H., … Sun, W.J. (2011). Pharmacokinetics of ergosterol in rats using rapid resolution liquid chromatography–atmospheric pressure chemical ionization multi-stage tandem mass spectrometry and rapid resolution liquid chromatography/tandem mass spectrometry. Journal of Chromatography B, 879, 1945–1953. doi:10.1016/j.jchromb.2011.05.025