ABSTRACT
Fish industry residues are used due to their easy transformation into several products and because they have nutrients with high biological value, being rich in proteins and fatty acids. The gelatin extraction process from the skin of kumakuma (Brachyplatystoma filamentosum) using sodium hydroxide was optimized and the product was characterized. The optimized conditions established in the process were 6 h extraction at 58°C, with yield and gel strength at 19.7% and 244.3 g, respectively, which is considered acceptable for foods. The maximum desirability condition was 0.998. When the technological properties of the gelatin extracted from fish skin was compared with commercial gelatin, a difference (p < 0.05) was observed for all parameters analyzed, but within the appropriate range for gelatin. The gelatin obtained from kumakuma skin may be a new alternative to replace gelatin from mammals, besides contributing to less fish residue released into the environment.
RESUMEN
Los residuos de la industria pesquera se utilizan debido a su fácil transformación en diversos productos, también por sus nutrientes de gran valor biológico, los cuales son ricos en proteínas y ácidos grasos. Se optimizó el proceso de extracción de gelatina de piel de kumakuma (Brachyplatystoma filamentosum) mediante la utilización de hidróxido sódico y se caracterizó el producto. Las condiciones optimizadas establecidas en el proceso fueron 6 h de extracción a 58°C, con un rendimiento y resistencia del gel de 19,7% y 244,3 g, respectivamente, lo cual se consideró aceptable para los alimentos. La máxima condición aceptable fue 0,998. Cuando se compararon las propiedades tecnológicas de la gelatina extraída de la piel del pez con la gelatina comercial, se observaron diferencias (p < 0,05) en todos los parámetros analizados, aunque dentro del rango apropiado en lo que concierne a la gelatina. La gelatina obtenida de la piel de kumakuma podría ser una nueva alternativa para remplazar la gelatina de mamíferos, además de contribuir a reducir los residuos de pescado vertidos en el medioambiente.
KEYWORDS:
PALABRAS CLAVE:
Introduction
The versatility of employing fish gelatin as food depends on its properties such as gel strength, viscosity, and melting point, among others, and it must not be considered an inferior replacement to traditional gelatin. Gelatin extracted from fish skin and bones has been increasingly used to replace gelatin from mammals. Since it can be used to prepare foods, less fish residue can be produced and issues with bovine spongiform encephalopathy can be avoided. This disease, which affects humans, has limited the use of bovine byproducts in the processing of functional foods, cosmetics, and pharmaceutical products (Gudmundsson, Citation2000; Muyonga, Cole, & Duodu, Citation2004; Nagarajan, Benjakul, Prodpran, & Songtipya, Citation2012).
Collagen is part of the supporting structures in vertebrates and invertebrates and it is an important component of the body wall in birds and fish (Herausgegeben, Ward, & Courts, Citation1977). Collagen contains high amounts of hydroxyproline and proline; the more abundant these imino acids, the more rigid and resistant it becomes (Arnesen & Gildberg, Citation2002). Gelatin’s amino acid composition is similar to that of the collagen from which it was obtained and is characterized by the gly–x–y sequence, where x is usually proline and y hydroxyproline (Herausgegeben et al., Citation1977).
Gelatin is a denatured protein derived from collagen by thermo-hydrolysis and has the rheological property of thermoreversible transformation between solid and gel. Gelatin is one of the most important biopolymers, with widespread applications in the food, pharmaceutical, cosmetic, and photographic industries. Recently, its use has been expanding to new applications such as functional foods. The properties of gelatin for a given application are strongly influenced not only by the species from which is it extracted but also by the pretreatment source, by the raw material, and by the process parameters (pH, gel maturation time, temperature, etc.) (Gómez-Guillen, Ihl, Bifani, Silva, & Monteiro, 2007; Kolodziesjska, Skierka, Sadowska, Kolodziejski, & Niecikowska, Citation2008).
Many fish species have been studied as raw material for the extraction of gelatin and its properties. Muyonga et al. (Citation2004) studied the extraction and physicochemical characterization of gelatin from skin and bones of Nile perch (Lates niloticus). Bueno, Alvim, Koberstein, Portela, and Grosso (Citation2011) characterized the gelatin extracted from tilapia (Oreochromis niloticus) skin and compared it with commercial gelatin from pigs. Alfaro (Citation2004) optimized the process (alkaline and acid pretreatment) and determined the functional properties of gelatin from king weakfish (Macrodon ancylodon), while Kolodziesjska et al. (Citation2008) determined the ideal conditions to prepare gelatin from different types of fish residue. However, few studies have investigated procedures to optimize extraction, which is an important tool to understand the processing conditions and obtain a final product with the desired characteristics (Shahiri, Maghsoudlou, Motamedzadegan, & Mahoonak, Citation2010).
The filleting of fish species in Amazonian industries, street markets, and supermarkets generates a considerable amount of skins, which are discarded as residue. Such residue is a viable alternative for gelatin production and one of these species is kumakuma (Brachyplatystoma filamentosum). So far, no gelatin has been extracted from the skin of this species and no information on its properties is available. In face of that, this study aimed to establish the optimal processing conditions to obtain gelatin from kumakuma skin using the response surface methodology (RSM) and the desirability function and to characterize the properties of the gelatin obtained.
Material and methods
Fresh kumakuma (B. filamentosum) skins were purchased at the Ver-o-Peso market in the city of Belém, PA, Brazil and transported under refrigeration in isothermic boxes to the Laboratory of Animal-Origin Products (LAPOA) of the Federal University of Pará (UFPA) for later use. Skins were removed at −20°C and kept frozen until use. The skins were cut into small pieces (4 cm × 4 cm) with scissors, placed in polyethylene bags, and kept at −25°C until used (within a week). All chemicals were analytical grade.
Obtaining gelatin
The collagen was obtained following the methodology described by Montero and Gómez-Guillén (Citation2000), with adaptations. After being washed in running water, the fish skins were cut into 4 cm × 4 cm pieces. First, the skins were immersed in a 0.6-mol/L sodium chloride (NaCl) aqueous solution for 15 min followed by immersion in a 0.3-mol/L sodium hydroxide (NaOH) solution for 15 min and, and finally, a 0.02-mol/L acetic acid (C2H4O2) solution for 60 min. In all steps, immersion took place under stirring and the skins were then washed with water, with three repetitions. Water was added to the material obtained and the mix was placed in a water bath to extract the collagen. After heating, the supernatant was collected and filtered with Whatman no. 4 filter paper. The gelatin obtained was placed on trays, frozen at −50°C, and lyophilized for 30 h. The lyophilized product was vacuum packaged, stored at −22°C, and later subjected to the assays in the experimental design.
Amino acid and physicochemical determinations of the skin and gelatin extracted from kumakuma skin
Analyses were performed for moisture (method no. 950.46), total proteins (method no. 928.08), lipids (method no. 960.39), and ashes (method no. 920.153) according to the Association of Official Analytical Chemists (AOAC, Citation2002). Skin pH was determined through AOAC method no. 981.12 and gelatin pH, using the methodology proposed by Schrieber and Gareis (Citation2007). The total amino acid profile was determined using a Waters-PICO Tag™ high-performance liquid chromatograph (Waters model 712 WISP, Watford, Herts, UK) following the methodology proposed by White, Hart, and Kry (Citation1986). Water activity was determined with an Aqualab 3TE electronic hygrometer (Decagon Devices Inc., USA). All analyses were performed in triplicate. Instrumental color was determined with a CR 310 colorimeter (Minolta, Japan) using the CIE (Commission Internationale de l’Eclairage) L*, a*, and b* space, where L* is luminosity, a* is red color intensity, and b* is yellow color intensity. The chroma index (c*) and hue angle (h°) were calculated (Hunterlab Inc, Citation2008).
Characterization of gelatin
The technological properties of the fish gelatin and the flavorless commercial gelatin (Royal®, Brazil) were determined under the same experimental conditions.
The total yield (%) and gelatin yield were calculated from the ratio between the gelatin weight and the skin’s wet weight (Binsi, Shamasundar, Dileep, Badii, & Howell, Citation2009).
Gel strength (Bloom) was determined in a texture analyzer using a cylindrical Teflon probe with 12.5 mm diameter pressed 4 mm into the gelatin at 1 mm/s (Choi & Regenstein, Citation2000).
The morphological analyses were carried out in a LEO-1430 (LEO, USA) scanning electron microscope. The samples were metallized with gold using coating time of 1.5 min. The analysis conditions for the secondary electron images were: electron beam current = 90 µA, constant acceleration voltage = 10 kV, and work distance = 15 mm. The melting point was assessed with a 6.67% gelatin solution, to which a drop of methylene blue dye was added and the solution was placed in a water bath at 15°C. In every 5 min, temperature was raised by 5°C until the displacement of the dye to the center of the solution based on the methodology by Choi and Regenstein (Citation2000). The foaming capacity (FC) was determined in gelatin solutions at different concentrations (1%, 2%, and 3%) and homogenized at 1750 rpm for 1 min at room temperature (24°C). FC was calculated from the ratio between the volumes before and after homogenization (Shahiri et al., Citation2010).
The emulsifying capacity (EC) was determined according to Shahiri et al. (Citation2010), with modifications. A volume of 20 mL 3.3% gelatin solution was mixed with 20 mL soybean oil. The mix was homogenized at 1750 rpm for 30 s and then centrifuged at 2000g for 5 min. EC was calculated as the ratio between the volume of the emulsified portion and the initial volume. Viscosity was determined according to the methodology described by Yang, Wang, Zhou, and Regenstein (Citation2008). The sample was placed in a water bath at 45°C and transferred into the Ostwald–Fensk viscosimeter (no. 100), which was placed in a water bath at 60°C for 10 min for temperature stabilization. The reading was expressed in centipoise (cP).
Statistical analysis
A central rotatable composite design and the RSM were used to define the best conditions for the responses of total process yield and gel strength allied to the appropriate viscosity conditions for the product’s commercial purposes. It was also considered that the process must have low complexity for it to be more viable at industrial scale. The assay was carried out in triplicate.
Eleven assays () were performed, four of which factorial (combination between the levels ±1), three in the central point (two variables at level 0), and four axial (one variable at level ±α and the other variable at level 0). For each response, variable significance or interactions were verified using a polynomial equation:
where Y is the dependent variable (gel yield and strength), β0 is the constant, βi and βii are the regression coefficient, and Xi and Xj are the level of the independent variables.
The deviations and relative deviations between the experimental values and those predicted by the models for the responses at the optimal condition were calculated by Equations 2 and 3, respectively.
where Y is the experimental response and is the response predicted by the model.
The desirability function was applied to determine the design’s optimal point and the statistical analysis of the data was carried out through analysis of variance and Tukey’s test to determine the significant differences of the means of the analyses performed on the gelatins, with 95% confidence (p < 0.05). The software STATISTICA 7 for Windows was used.
Results and discussion
Optimizing the gelatin obtention process
presents the experimental results obtained for gelatin yield (%) and gel strength (g). The estimates of the factor coefficients for the model of each response assessed are presented in . As for total yield, the effects were significant (p < 0.05) for lineal extraction temperature (B), where the higher temperature led to higher gelatin yield. Using high temperatures causes greater collagen hydrolysis, thus leading to higher yields (Holzer, Citation1996). The quadratic extraction temperature (BB) has a significant (p < 0.05) negative effect on gel strength, i.e. a reduction in this factor may lead to lower gel strength.
Table 1. 22 experimental design matrix with the results obtained for yield (%) and gel strength (g).
Tabla 1. Matriz de diseño experimental 22 con los resultados obtenidos del rendimiento (%) y la resistencia del gel (g).
Table 2. Estimate of the variables of second-order polynomials (Equation 1) associated with the significance for each response studied (pure error).
Tabla 2. Estimación de las variables de polinomios de segundo orden (Equation. 1) asociados a la significancia de cada respuesta estudiada (error puro).
shows that the value of F calculated for the lack-of-fit was lower than F tabulated both for gel yield and strength. The R2 value was 0.83 for both parameters studied, which suggests that the model appropriately defined the process behavior by explaining 83% of the variation in the experimental data. In addition, the lack-of-fit for the equations of gel yield and strength was not significant (p ≥ 0.05), which suggests that the equations can be used for predictive purposes in the experimental domain studied.
Table 3. Analysis of variance (ANOVA) for gel yield and strength as functions of the independent variance, F test, and R2.
Tabla 3. Análisis de varianza (ANOVA) del rendimiento del gel y la resistencia como funciones de varianza independiente, prueba F y R2.
Based on the experimental data, employing higher temperatures led to higher yields ()). However, lower gel strength was observed in the gelatin, which is attributed to the formation of a larger amount of compounds with low-molecular weight (Holzer, Citation1996). Extraction temperatures of up to 60°C are appropriate.
Figure 1. Yield (A) and gel strength (B) response surface as a function of time and temperature.
Figura 1. Superficie de respuesta del rendimiento (A) y la resistencia del gel (B) como función de tiempo y temperatura.
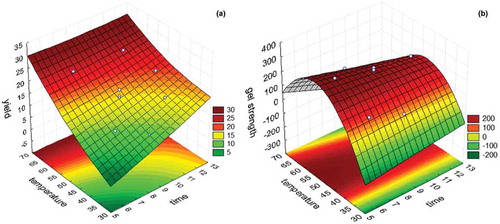
) shows that the higher temperature and longer extraction time led to greater gel strength; however, gel strength decreased as temperature gradually increased. The highest gel strength values were obtained between 50 and 60°C and between 6 and 9 h. Biluca, Marquetti, and Alfaro (Citation2001) observed that the variation in extraction time and temperature greatly impacted gelatin yield. Arnesen and Gildberg (Citation2002); Muyonga et al. (Citation2004); and Cho, Gu, and Kim (Citation2005) reported that gelatins obtained through extractions at high temperatures could lead to the extraction of low-molecular-weight proteins, thus increasing yield while decreasing gel strength.
At 6 h of extraction at 58°C, the maximum desirability conditions were found (0.998), with gel yield and strength of 19.7% and 244.3 g, respectively, both of which desirable. Jamilah and Harvinder (Citation2002) found yields of 6% and 19% and gel strength between 250 and 260 g for gelatin from two species of tilapia.
Assays were carried out to obtain gelatin at the optimal temperature (58°C) and extraction time (6 h) conditions so that the experimental values of gel yield and strength were compared to those predicted by the regression models. The difference between the experimental and predicted values resulted in a low relative deviation (2% for yield and 7% for gel strength), which shows that the method can be used to predict the yield and gel strength of gelatin obtained under these conditions.
Chemical characterization and amino acid content of the skin and gelatin
presents the physicochemical properties of kumakuma skin and of the gelatin extracted from it. Skin moisture is in accordance with the value found in corvina (62.3%) and shortfin scad (Decapterus macrosoma) (60.4%) (Cheow, Norizah, Kyaw, & Howell, Citation2007). Likewise, gelatin moisture was similar to that observed by Cho and Kim (Citation2004) in shark cartilage (7.98%) and lower than what Eastoe and Leach (Citation1997) reported for commercial gelatins (9–14%).
Table 4. Physicochemical properties of the skin and gelatin extracted from kumakuma skin.*
Tabla 4. Propiedades fisicoquímicas de la piel y gelatina extraída de la piel de kumakuma.*
The skin’s fat content was high (14.25 ± 0.22%), a characteristic of the fish species studied, which was classified as a fatty fish by Souza, Baccarin, Viegas, Macedo, and Kronka (Citation2004). Shahiri, Maghsoudlou, Motamedzadegan, Sadeghi, and Rostamzad (Citation2012) observed similar lipid content (13.12%) in rainbow trout (Onchorhynchus mykiss) skin. The gelatin’s high lipid content (29.7 ± 2.48%) suggests the need for the extraction of the lipid fraction from the fish skin prior to collagen extraction. The protein content in kumakuma skin (31.08 ± 0.99%) was similar to the value found by Bueno et al. (Citation2011) (28.5 ± 1.8%). It is important to point out that the protein content in the skin represents the amount of collagen in the gelatin and, consequently, the extraction yield (Biluca et al., Citation2001). The gelatin obtained had lower protein content (72.76 ± 2.02%) than that observed by Alfaro, Fonseca, Balbinot, Machado, and Prentice (Citation2013) (81.16 ± 2.15%) for gelatin from tilapia skin. The ash content in the skin and gelatin was lower than that reported by Bueno et al. (Citation2011) for tilapia skin (1.9%) and by Haug, Draget, and Smidsrod (Citation2004) for gelatin from cold-water fish (0.82%).
Higher pH was observed in the gelatin than in the skin, which is attributed to the chemical treatment with NaOH employed in the collagen extraction step (Gudmundsson & Hafsteinsson, Citation1997). According to Shahiri et al. (Citation2012), collagen solubility is minimum at pH 7 and 9, which makes dissolving the gelatin in water more difficult.
The total imino acid contents (proline + hydroxyproline) in the skin and in the lyophilized gelatin were 13.67% and 13.53%, respectively (). A higher imino acid content was found by Alfaro et al. (Citation2013) (19.5%) in gelatin from the skin of Nile tilapia (Johnius dussumieri). The imino acid content is the main difference between the gelatin from fish and from mammals since these imino acids stabilize the structures during gel formation and confer better viscoelastic properties to gelatin from fish skin (Foegeding, Lanier, & Hultin, Citation1996). Lysine also stabilized the gelatin structure by forming cross-links between the chains while the values found in the skin (3.08%) and gelatin (3.43%) from kumakuma skin were higher than those found by Cho and Kim (Citation2004) in gelatin from shark (2.27%). Gelatin from cold-water fish has lower imino acid content than from warm-water fish, which is closer to gelatin from mammals (Haug et al., Citation2004). Glycine was the main amino acid both in the kumakuma skin and in the gelatin extracted from it.
Table 5. Total amino acid profile in kumakuma skin and gelatin (g/100 g protein).
Tabla 5. Perfil de total de aminoácidos en la piel de kumakuma y la gelatina (g/100 g de proteína).
Characterization of gelatin
shows the results of the technological characterization of gelatin from kumakuma skin and of commercial gelatin, which was used as a comparison. The gelatin extraction yield from kumakuma skin was similar to that reported by Songchotikunpan, Tattiyakul, and Supaphol (Citation2008) (18.1%) and Bueno et al. (Citation2011) (18.3%) for gelatin from tilapia skin. Such yield might vary according to the extraction method employed and to the fish species and age (Jongiareonrak, Benjakul, Visessanguan, & Tanaka, Citation2006; Muyonga et al., Citation2004). Higher temperatures also lead to higher yields and complete extraction (Holzer, Citation1996).
Table 6. Characterization of kumakuma gelatin and a commercial gelatin.
Tabla 6. Caracterización de la gelatina de kumakuma y una gelatina comercial.
The gel strengths of gelatin from kumakuma skin and from commercial gelatin were statistically different (p < 0.05) but are within the proper range for foods. Commercial gelatins usually have gel strength between 90 and 300 g, while values between 250 and 160 g are desirable (Jones, Citation1977; Karim & Bhat, Citation2009). Gel strength is gelatin’s main physical property and determines its commercial value.
Gel consistency is the property determined by the product’s amino acid composition and, in the fish gelatin studied, the content of imino acids proline and hydroxyproline can be related to the product’s good viscoelastic properties. The gelatin extracted from the head of common carp had gel strength of 240 g (Silva, Bandeira, Petry, & Pinto, Citation2011). Grossman and Bergman (Citation1992) and Muyonga et al. (Citation2004) observed gel strength values between 263 and 229 g for gelatin from tilapia skin and Nile perch, respectively.
The viscosity of the gelatin from kumakuma skin was higher than that of commercial gelatin (p < 0.05), however, within the range of 2–7 cP observed in commercial gelatins (Jamilah & Harvinder, Citation2002; Johnston-Banks, Citation1990). Silva, Bandeira, and Pinto (Citation2014) found viscosity of 4.32 cP for gelatin from cobia (Rachycentron canadum), while Grossman and Bergman (Citation1992) reported 5.1 cP for tilapia skin.
The melting point of the gelatin obtained in the present study was lower (p < 0.05) than the value obtained for the commercial gelatin used as reference. This behavior can be explained by the lower contents of hydroxyproline and proline amino acids in fish gelatin compared to gelatin from mammals (15–20%) (Grossman & Bergman, Citation1992). In this context, it has been shown that the thermal stability of gelatin is proportional to the number and stability of proline-rich regions in collagen and gelatin molecules (Gomez-Guillen et al., Citation2002).
Cho et al. (Citation2005) observed a melting point of 24.3°C for gelatin from tuna (Thunnus albacares), while Bueno et al. (Citation2011) reported values between 24 and 26°C for the melting point of gelatin from Nile tilapia.
The emulsifying power of the gelatin from kumakuma skin was different (p < 0.05) from that of the commercial gelatin. The EC depends on the level of exposition to hydrophobic residues inside the gelatin and the presence of low-molecular-weight peptides in fish gelatins may have improved their EC by facilitating the diffusion at the surface of oil droplets (Surh, Decker, & McClements, Citation2006). The hydrophobic amino acid tyrosine accounted for 0.63% of the gelatin in kumakuma skin. Cho and Kim (Citation2004) found 0.10–0.24% of tyrosine in the gelatin from pig skin. The greater amount of tyrosine in fish gelatin was likely responsible for its higher EC (Shahiri et al., Citation2012). Rawdkuen, Thitipramote, and Benjaku (Citation2013) observed an EC for fish gelatin (38.37 ± 0.72%) above that observed for commercial gelatin from bovines (36.40 ± 1.01).
At the different protein concentrations, the foaming capacities of the solutions of gelatin from kumakuma skin were statistically different (p < 0.05) from that of the commercial gelatin. In turn, in all gelatin solutions, the FC increased with higher protein concentrations. This is in line with the study of Kaewruang, Benjakul, and Prodpran (Citation2013), who reported that increasing concentrations of gelatin extracted at different temperatures from the skin of leatherjacket lead to the increase in foam expansion levels. The hydrophobic surfaces of the peptide chain are responsible for the gelatin’s emulsifying and foaming properties (Cole, Cad, & Benameur, Citation2008; Galazka, Dickinson, & Ledward, Citation1999). Rawdkuen et al. (Citation2013) observed that gelatin from fish skin had greater FC compared to commercial gelatin.
A significant difference (p < 0.05) was observed in all color parameters analyzed between the gelatin from kumakuma skin and the commercial gelatin. Fish gelatin’s color was clear and shiny, whereas the commercial gelatin was bright yellow. The color of commercial gelatins usually ranges from pale yellow to dark amber (Cole & Roberts, Citation1997). The color difference between the gelatins may be due to the presence of the pigment melanin, which is dark brown, produced by the melanocytes present in fish skin (Junqueira & Carneiro, Citation2008) or due to the manufacturing process. The thermal extraction of gelatin causes Maillard reactions (Wang, Qian, & Yao, Citation2011); thus, the color intensity depends on the extraction temperature.
The electromicrographies of gelatin from kumakuma skin () clearly show the formation of interconnected pores, capillary canals, and cross-links in the pores’ periphery. In gelatin processing, during lyophilization, ice crystals are formed during freezing, followed by sublimation during vacuum drying, which causes pore formation (Frydrych, Wan, Stengler, O’Kelly., & Chen, Citation2011). The differences in gelatin microstructure were probably associated with the length of extracted gelatins, as well as the disruption of the interchain hydrogen bonds stabilizing the triple helix structure (Jridi et al., Citation2013).
Figure 2. Electron micrography of kumakuma gelatin at 50 (A) and 200× (B) magnification.
Figura 2. Micrografía electrónica de la gelatina de kumakuma a una magnificación de 50 (A) y 200x (B).
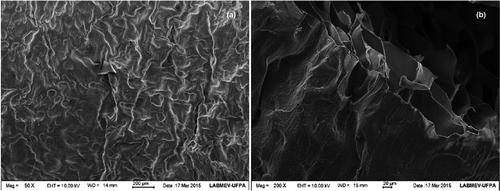
Large empty and uneven spaces were observed in the gelatin’s structure. Usually, the arrangement and association of protein molecules in the gel matrix directly contribute to the gelatin’s gel strength (Benjakul, Oungbho, Visessanguan, Thiansilakul, & Roytrakul, Citation2009). The gel’s microstructure is related to the gelatin’s physical properties (Yang et al., Citation2008). Gels with thicker chains are harder to be unstructured by an applied force, which results in greater gel strength. Similar results were observed by Soottawat, Kwunchit, Wonnop, Yaowapa, and Sittiruk (Citation2009); Bhat, Tripathi, and Kumar (Citation2011); Liu, Li, Zhou, Fan, and Fan (Citation2012); and Henderson et al. (Citation2012) in gelatin from fish skin.
Conclusion
According to the model proposed, the optimal extraction conditions were established at 6 h and 58°C, the maximum desirability conditions were found (0.998), with gel yield and strength of 19.7% and 244.3 g, respectively, both of which were desirable. The gelatin from kumakuma skin had chemical and functional properties similar to those of commercial gelatin, clear and shiny color, and lower amino acid content than what is reported in the literature for warm-water fish. The electromicrographies of gelatin from kumakuma skin show large empty and uneven spaces in the gelatin’s structure. Based on the functional properties, gelatin from kumakuma skin can be used as one more alternative in the food industries and areas alike.
Acknowledgments
The authors are thankful to Pará State University for the financial support and to the Graduate Program in Food Science and Technology of the Federal University of Pará for the technical support.
Disclosure statement
No potential conflict of interest was reported by the authors.
Additional information
Funding
References
- Alfaro, A.T. (2004). Optimization of process and determination of functional properties of fish bones gelatin (Master’s thesis). Federal University of Rio Grande, Brazil.
- Alfaro, A.T., Fonseca, G.G., Balbinot, E., Machado, A., & Prentice, C. (2013). Physical and chemical properties of wami tilapia skin gelatin. Food Science and Technology, 33(3), 592–595. doi:10.1590/S0101-20612013005000069
- AOAC. (2002). AOAC, Official methods of analysis of AOAC international (17th ed.). Gaithersburg, MD: AOAC International.
- Arnesen, J.A., & Gildberg, A. (2002). Preparation and characterisation of gelatine from the skin of harp seal (Phoca groendlandica). Bioresource Technology, 82(2), 191–194. Pubmed 12003322. doi:10.1016/S0960-8524(01)00164-X
- Benjakul, S., Oungbho, K., Visessanguan, W., Thiansilakul, Y., & Roytrakul, S. (2009). Characteristics of gelatin from the skins of bigeye snapper, Priacanthus tayenus and Priacanthus macracanthus. Food Chemistry, 116(2), 445–451. doi:10.1016/j.foodchem.2009.02.063
- Bhat, S., Tripathi, A., & Kumar, A. (2011). Supermacroprous chitosan–agarose–gelatin cryogels: In vitro characterization and in vivo assessment for cartilage tissue engineering. Journal of the Royal Society Interface, 8(57), 540–554. doi:10.1098/rsif.2010.0455
- Biluca, F.C., Marquetti, C., & Alfaro, A.T. (2001). Production catfish skin and bone gelatin (Clarias gariepinus). Revista Brasileira De Tecnologia Agroindustrial, 05, 418–426. doi:10.3895/S1981-36862011000100005S1
- Binsi, P.K., Shamasundar, B.A., Dileep, A.O., Badii, F., & Howell, N.K. (2009). Rheological and functional properties of gelatin from the skin of Bigeye snapper (Priacanthus hamrur) fish: Influence of gelatin on the gel-forming ability of fish mince. Food Hydrocolloids, 23(1), 132–145. doi:10.1016/j.foodhyd.2007.12.004
- Bueno, C.M., Alvim, I.D., Koberstein, T.C.R.D., Portela, M.C., & Grosso, C. (2011). Production of tilapia skin gelatin and its use in the production of micro-particles containing salmon oil. Brazilian Journal of Food Technology, 14(1), 65–73. doi:10.4260/BJFT2011140100009
- Cheow, C.S., Norizah, M.S., Kyaw, Z.Y., & Howell, N.K. (2007). Preparation and characterisation of gelatins from the skins of sin croaker (Johnius dussumieri) and shortfin scad (Decapterus macrosoma). Food Chemistry, 101(1), 386–391. doi:10.1016/j.foodchem.2006.01.046
- Cho, S.M., Gu, Y.S., & Kim, S.B. (2005). Extracting optimization and physical properties of yellowfin tuna (Thunnus albacares) skin gelatin compared to mammalian gelatins. Food Hydrocolloids, 19(2), 221–229. doi:10.1016/j.foodhyd.2004.05.005
- Cho, S.M., & Kim, S.B. (2004). Processing optimization and functional properties of gelatin from shark (Isurus oxyrinchus) cartilage. Food Hydrocolloids, 18(4), 573–579. doi:10.1016/j.foodhyd.2003.10.001
- Choi, S.S., & Regenstein, J.M. (2000). Physicochemical and sensory characteristics of fish gelatin. Journal of Food Science, 65(2), 194–199. doi:10.1111/j.1365-2621.2000.tb15978.x
- Cole, C.G.B., & Roberts, J.J. (1997). Gelatine colour measurement. Meat Science, 45(1), 23–31. Pubmed: 22061134. doi:10.1016/S0309-1740(96)00096-4
- Cole, E.T., Cad, D., & Benameur, H. (2008). Challenges and opportunities in the encapsulation of liquid and semi-solid formulations into capsules for oral administration. Advanced Drug Delivery Reviews, 60(6), 747–756. doi:10.1016/j.addr.2007.09.009
- Eastoe, J.E., & Leach, A.A. (1997). Chemical constitution of gelatin. The science and technology of gelatin. London: Academic Press.
- Foegeding, E.A., Lanier, T.C., & Hultin, H.O. (1996). Collagen. In O.R. Fennema (Ed.), Food Chemistry (3 rd ed., pp. 902–906). New York: Marcel Dekker, Inc.
- Frydrych, M., Wan, C., Stengler, R., O’Kelly., K.U., & Chen, B. (2011). Structure and mechanical properties of gelatin/sepiolite nanocomposite foams. Journal of Materials Chemistry, 1(21), 9103–9111. doi:10.1039/C1JM10788G
- Galazka, V.B., Dickinson, E., & Ledward, D.A. (1999). Emulsifying behaviour of 11S globulin Vicia faba in mixtures with sulphated polysaccharides: Comparison of thermal and high-pressure treatments. Food Hydrocolloids, 13(5), 425–435. doi:10.1016/S0268-005X(99)00028-4
- Gómez-Guillen, M.C., Ihl, M., Bifani, V., Silva, A., & Monteiro, P. (2007). Edible filmes made from tuna-fish gelatina with antioxidant extracts of two different murta ecotypes leaves (Ugni molinae Tuczs). Food Hydrocolloids, 21(7), 1133–1143. doi:10.1016/j.foodhyd.2006.08.006
- Gomez-Guillen, M.C., Turnay, J., Fernandez-Diaz, M.D., Ulmo, N., Lizarbe, M.A., & Montero, P. (2002). Structural and physical properties of gelatin extracted from different marine species: A comparative study. Food Hydrocolloids, 16, 25–34. doi:10.1016/S0268-005X(01)00035-2
- Grossman, S., & Bergman, M. (1992).U.S. Patent No 5.093.474. Washington, DC: U.S. Patent and Trademark Office.
- Gudmundsson, M. (2000). Rheological properties of fish gelatins. Journal of Food Science, 67(6), 2172–2176. doi:10.1111/j.1365-2621.2002.tb09522.x
- Gudmundsson, M., & Hafsteinsson, H. (1997). Gelatin from cod skins as affected by chemical treatments. Journal of Food Science, 62(1), 37–39. doi:10.1111/j.1365-2621.1997.tb04363.x
- Haug, I.J., Draget, K.I., & Smidsrod, O. (2004). Physical and rheological properties of fish gelatin compared to mammalian gelatin. Food Hydrocolloids, 18(2), 203–213. doi:10.1016/S0268-005X(03)00065-1
- Henderson, L.A., Stathis, A., James, C., Brown, R., McDonald, S., & Macefield, V.G. (2012). Real-time imaging of cortical areas involved in the generation of increases in skin sympathet- ic nerve activity when viewing emotionally charged images. NeuroImage, 62(1), 30–40. doi:10.1016/j.neuroimage.2012.04.049
- Herausgegeben, V., Ward, A.G., & Courts, U.A. (1977). The Science and Technology of Gelatin. London: Academic Press.
- Holzer, D. (1996).U.S. Patent No 5.484.888. Washington, DC: U.S. Patent and Trademark Office.
- Hunterlab Inc. (2008). Applications note: CIE L * a * b * Color scale. 8, 1–4.
- Jamilah, B., & Harvinder, K.G. (2002). Properties of gelatins from skins of fish: Black tilapia (Oreochromis mossambicus) and red tilapia (Oreochromis nilotica). Food Chemistry, 77(1), 81–84. doi:10.1016/S0308-8146(01)00328-4
- Johnston-Banks, F.A. (1990). Gelatin. New York: Elsevier Applied Sciences.
- Jones, N.R. (1977). Uses of gelatin in edible products.: The science and technology of gelatin. London: Academic Press.
- Jongiareonrak, A., Benjakul, S., Visessanguan, W., & Tanaka, M. (2006). Skin gelatin from bigeye snapper and borwnstripe red snapper: Chemical compositions and effect of microbial transglutaminase on gel properties. Food Hydrocolloids, 20(6), 1216–1222. doi:10.1016/j.foodhyd.2006.01.006
- Jridi, M., Souissi, N., Mbarek, A., Chadeyron, G., Kammoun, M., & Nasri, M. (2013). Comparative study of physico-mechanical and antioxidant properties of edible gelatin films from the skin of cuttlefish. International Journal of Biological Macromolecules, 61, 17–25. doi:10.1016/j.ijbiomac.2013.06.040
- Junqueira, L.C., & Carneiro, J. (2008). Basic histology. Rio de Janeiro: Guanabara Koogan.
- Kaewruang, P., Benjakul, S., & Prodpran, T. (2013). Molecular and functional properties of gelatin from the skin of unicorn leatherjacket as affected by extracting temperatures. Food Chemistry, 138, 1431–1437. doi:10.1016/j.foodchem.2012.09.114.
- Karim, A.A., & Bhat, R. (2009). Fish gelatin: Properties, challenges, and prospects as an alternative to mammalian gelatins. Food Hydrocolloids, 23(3), 563–576. doi:10.1016/j.foodhyd.2008.07.002
- Kolodziesjska, I., Skierka, E., Sadowska, M., Kolodziejski, W., & Niecikowska, C. (2008). Effect of extracting time and temperature on yield of gelatin from different fish offal. Food Chemistry, 107(2), 700–706. doi:10.1016/j.foodchem.2007.08.071
- Liu, H., Li, X., Zhou, G., Fan, H., & Fan, Y. (2012). Electrospun sulfated silk fibroin nanofibrous scaffolds for vascular tissue engineering. Biomaterials, 32(15), 3784–3793. doi:10.1016/j.biomaterials.2011.02.002
- Montero, P., & Gómez-Guillén, M.C. (2000). Extracting conditions for megrim (Lepidorhombus boscii) skin collagen affect functional properties of the resulting gelatin. Jounal of Food Science, 65(3), 434–438. doi:10.1111/j.1365-2621.2000.tb16022.x
- Muyonga, J.H., Cole, C.G.B., & Duodu, K.G. (2004). Extraction and physico-chemical characterisation of Nile perch (Lates niloticus) skin and bone gelatin. Food Hydrocolloids, 18(4), 581–592. doi:10.1016/j.foodhyd.2003.08.009
- Nagarajan, M., Benjakul, S., Prodpran, T., & Songtipya, H.K. (2012). Characteristics and functional properties of gelatin from splendid squid (Loligo formosana) skin as affected by extraction temperature. Food Hydrocolloids, 29(2), 389–397. doi:10.1016/j.foodhyd.2012.04.001
- Rawdkuen, S., Thitipramote, N., & Benjaku, S. (2013). Preparation and functional characterisation of fish skin gelatin and comparison with commercial gelatin. International Journal of Food Science & Technology, 48(5), 1093–1102. doi:10.1111/ijfs.12067
- Schrieber, R., & Gareis, H. (2007). Gelatine handbook: Theory and industry practice. Weinheim: Wiley-VCH Verlag GmbH and Co. KgaA.
- Shahiri, T.H., Maghsoudlou, Y., Motamedzadegan, A., & Mahoonak, A.R.S. (2010). Optimization of physico-chemical properties of gelatin extracted from fish skin of rainbow trout (Onchorhynchus mykiss). Bioresource Technology, 101(15), 6207–6214. doi:10.1016/j.biortech.2010.02.071
- Shahiri, T.H., Maghsoudlou, Y., Motamedzadegan, A., Sadeghi, M.A.R., & Rostamzad, H. (2012). Study on some properties of acid-soluble collagens isolated from fish skin and bones of rainbow trout (Onchorhynchus mykiss). International Food Research Journal, 19(1), 251–257.
- Silva, R., Bandeira, S.F., Petry, F.C., & Pinto, L.A.A. (2011). Gelatin extraction from skins of common carp heads. Ciência Rural, 41(5), 904–909. doi:10.1590/S0103-84782011005000059
- Silva, R., Bandeira, S.F., & Pinto, L.A.A. (2014). Characteristics and chemical composition of skins gelatin from cobia (Rachycentron canadum). Food Science and Technology, 57(2), 580–585. doi:10.1016/j.lwt.2014.02.026
- Songchotikunpan, P., Tattiyakul, J., & Supaphol, P. (2008). Extraction and electrospinning of gelatin from fish skin. International Journal of Biological Macromolecules, 42(3), 247–255. doi:10.1016/j.ijbiomac.2007.11.005
- Soottawat, B., Kwunchit, O., Wonnop, V., Yaowapa, T., & Sittiruk, R. (2009). Characteristics of gelatin from the skins of bigeye snapper, Priacanthus tayenus and Priacanthus macracanthus. Food Chemistry, 116(2), 445–451. doi:10.1016/j.foodchem.2009.02.063
- Souza, M.L.R., Baccarin, A.E., Viegas, I., Macedo, E.M., & Kronka, S.N. (2004). Smoking process of whole eviscerated and fillet of nile tilapia (oreochromis niloticus): Organoleptic characteristics, proximate composition and losses during processing. Revista Brasileira De Zootecnia, 33(1), 27–36. doi:10.1590/S1516-35982004000100005
- Surh, J., Decker, E.A., & McClements, D.J. (2006). Properties and stability of oil-in water emulsions stabilized by fish gelatin. Food Hydrocolloids. 20, 596–606. doi:10.1016/j.foodhyd.2005.06.002.
- Wang, H.Y., Qian, H., & Yao, W.R. (2011). Melanoidins produced by the Maillard reaction: Structure and biological activity. Food Chemistry, 128(3), 573–584. doi:10.1016/j.foodchem.2011.03.075
- White, J.A., Hart, R.J., & Kry, J.C. (1986). An evaluation of the waters pico-tag system for the amino acid analysis of food materials. Journal Automatic Chemical, 8(4), 170–177. doi:10.1155/S1463924686000330
- Yang, H., Wang, Y., Zhou, P., & Regenstein, J.M. (2008). Effects of alkaline and acid pretreatment on the physical properties and nanostructures of the gelatin from channel catfish skins. Food Hydrocolloids, 22(8), 1541–1550. doi:10.1016/j.foodhyd.2007.10.007