ABSTRACT
Essential oil from yarrow (Achillea millefolium L. s. l.) has a broad spectrum of pharmacological activities. However, active chemical components of yarrow oil are sensitive to environmental factors such as, light, oxygen and temperature. Encapsulation of essential oil offers solutions for the limitation. Yarrow oil was encapsulated in hydroxypropyl-β-cyclodextrin (HPβCD) through freeze-drying technique with encapsulation efficiency of 45%. Inclusion complex formation was examined by scanning electron microscope, Fourier transformed infrared spectroscopy and UV–vis analysis and phase solubility study. Yarrow oil gave strong antioxidant activity of 72% DPPH scavenging at 50 μg/mL. HPβCD could protect active compounds of essential oil and retained antioxidant activity after sunlight exposure. Yarrow oil also exhibited antibacterial activity against Staphylococcus aureus and Escherichia coli with the minimum inhibitory concentration (MIC) values of 250 μg/mL and 500 μg/mL, respectively. The antibacterial efficacy was much improved after encapsulation against both S. aureus and E. coli with the MIC value of 62.5 μg/mL.
RESUMEN
El aceite esencial de la milenrama (Achillea millefolium L. s. l.) presenta un amplio espectro de actividades farmacológicas. Sin embargo, los componentes químicos activos del aceite son sensibles a factores ambientales tales como, luz, oxígeno y temperatura. La encapsulación del aceite esencial ofrece soluciones para esta limitación. El aceite se encapsuló en hidroxipropil-β-ciclodextrina (HPβCD) mediante la técnica de liofilización con un rendimiento del 45%. La formación del complejo de inclusión se analizó mediante técnicas de SEM, FT-IR y UV-vis y se realizó un estudio de solubilidad. El aceite presentó una fuerte actividad antioxidante de 72% de DPPH. HPβCD protege los compuestos activos de aceite esencial y retoene la actividad antioxidante después de la exposición a luz solar. El aceite también exhibió actividad antibacteriana contra Staphylococcus aureus y Escherichia coli con valores mínimos de concentración inhibitoria (MIC) de 250 μg/mL y 500 μg/mL, respectivamente. La eficacia antibacteriana mejoró mucho después de la encapsulación contra S. aureus y E. coli con un valor de CIM de 62,5 μg /ml.
1. Introduction
Yarrow (Achillea millefolium L. s. l.) has a broad spectrum of pharmacological activities. It is widely used in folk medicine (Wichtl, Citation1997). In Europe, it has been used as a remedy to treat digestive problems, diabetes, hepatobiliary diseases, amenorrhea, and also consumed for its antitumor and anti-inflammatory properties (Baretta et al., Citation2012; Cavalcanti et al., Citation2006; Jonsdottir, Omarsdottird, Vikingssona, Hardardottirc, Freysdottir, Citation2011). In addition, antimicrobial and antioxidant properties of yarrow have also been reported (Candan et al., Citation2003; Potrich et al., Citation2010; Trumbeckaite et al., Citation2011). Chemical components of yarrow essential oil have been found to be carvacrol, linalool, 1,8-cineole, camphor and thymol, and carvacrol was mostly found as a major component (Alfatemi, Rad, Rad, Mohsenzadeh, & Da Silva, Citation2014). However, some active chemical components of yarrow oil (such as carvacrol and thymol) are sensitive to environmental factors such as, light, oxygen and temperature. Encapsulation of yarrow essential oil could offer possible solutions for the limitation. The use of cyclodextrins for encapsulation of various kinds of essential oil has been found to preserve the sensitive active compounds of essential oils against environmental conditions (Hedges, Shieh, & Sikorski, Citation1995; Qi & Hedges, Citation1997). Cyclodextrins also improve the aqueous solubility of essential oils for increasing their capacity to functionalize the products (Marques, Citation2010).
Cyclodextrins are cyclic oligosaccharides consisting of glucopyranosyl units linked by α-(1,4) bonds (Schmann & Schollmeyer, Citation2002). The widely used natural cyclodextrins are α-, β- and γ-cyclodextrin consisting of 6, 7 and 8 glucopyranose units, respectively. The cyclodextrin molecules have a unique structure with a hydrophobic cavity and a hydrophilic surface which can form inclusion complex with a wide variety of guests. Among those cyclodextrins, β-cyclodextrin is the most widely applicable kind because of its suitable cavity size for common guests and its availability and reasonable price (Waleczek, Marques, Hempel, & Schmidt, Citation2003). However, water solubility of β-cyclodextrin is quite low (1.8 g in 100 mL water at 25°C). Because of this reason, cyclodextrin derivatives have been developed to extend the physiochemical properties and the inclusion capacity of original cyclodextrins. A hydroxyalkylated β-cyclodextrin derivative, hydroxypropyl-β-cyclodextrin (HPβCD), has relatively high aqueous solubility with low toxicity and satisfactory inclusion ability (Garnero, Zoppi, Genovese, & Longhi, Citation2010). This modified β-cyclodextrin has higher water solubility (above 60 g in 100 mL water at 25°C) and a proven safe profile.
Encapsulation of essential oils or their chemical components with β-cyclodextrin or derivatives for improvement of biological properties have been observed (Astray, González-Barreiro, Mejuto, Rial-Otero & Simal-Gándara, Citation2009; Benedek, Geisz, Jager, Thalhammer, & Kopp, Citation2006; Chandler, Hooper, & Harvey, Citation1982; Keser, Celik, Turkoglu, Yilmaz, & Turkoglu, Citation2011, Citation2013). Encapsulation of essential oils from cinnamon and clove could improve their antimicrobial activity has been reported (Hill, Gomes, & Taylor, Citation2013). Similar observation was also reported in the literature (Kamimura, Santos, Hill, & Gomes, Citation2014). The antimicrobial activity of the encapsulated carvacrol with hydroxypropyl-β-cyclodextrin against Escherichia coli and Salmonella enterica serovar Typhimurium could be increased from the free carvacrol. Based on the foregoing, the yarrow essential oil is expected to apply in several fields such as pharmaceutical and food applications. Therefore, the gold of this research was to characterize the microencapsulated yarrow essential oil in hydroxypropyl-β-cyclodextrin and its resulting antioxidant and antibacterial properties, in order to stabilize and improve its physiochemical characteristics and bio-efficacies.
2. Materials
2.1. Microorganisms
The indicator bacteria used for testing antimicrobial activity were Staphylococcus aureus TISTR 517 (ATCC 14458) (representative for gram-positive bacteria) and E. coli TISTR 886 (ATCC 29214) (representative for gram-negative bacteria). These strains were provided from Thailand Institute of Scientific and Technological Research. The indicator bacteria were cultivated in tryptic soy broth and incubated at 37°C with shaking at 200 rpm for 24 h. All bacteria were maintained at −20°C in 25% (v/v) glycerol.
2.2. Chemicals
Hydroxypropyl-β-cyclodextrin (HPβCD), 1, 1-diphenyl-2-picrylhydrazyl (DPPH), Butylated hydroxytoluene (BHT) and carvacrol were purchased from Sigma-Aldrich (Steinheim, Germany). Yarrow essential oil was purchased from Botanicessence (Bangkok, Thailand). Dimethy sulfoxide (DMSO) was purchased from Fisher Scientific (U.K.).
3. Methods
3.1. Encapsulation of yarrow essential oil
Encapsulation of yarrow essential oil was carried out through inclusion complex formation with hydroxypropyl-β-cyclodextrin (HPβCD) by freeze-drying method (Karathanos, Mourtzinos, Yannakopoulou, & Andrikopoulos, Citation2007). The 0.5 g of yarrow oil was slowly added to an aqueous solution of HPβCD (5 g of HPβCD in 25 mL of water). The mixture was left in a sealed container under stirring (150 rpm/24 h) at room temperature (~25°C) and protected from the light. The inclusion complexes dissolved in the solution were separated from the unencapsulated particles by filtration through 0.45-mm PTFE filters (IC Millex-LH, Millipore, Billerica, MA). The resulting solution containing inclusion complexes was frozen at −20°C and lyophilized at −50°C and 1.09 Pa in a Labconco Freeze Dryer-5 (Kansas City, MO) for approximately 48 h or until all moisture had been sublimated. The lyophilized powder was washed with acetonitrile (to remove particles attaching on the HPβCD surface) and dried in a low temperature incubator at 25°C. The inclusion complex product was stored in sealed container inside a desiccator until use. The encapsulation efficiency was calculated compared to the standard curve of yarrow oil. For standard curve preparation, several concentrations (2–50 mg/mL) of yarrow oil were prepared in ethanol and the absorbance of each concentration was measured at 275 nm. The absorbance at 275 nm was plotted against yarrow oil concentrations to establish a standard curve. To calculate the encapsulation efficiency, the encapsulated compounds were extracted from HPβCD cavity with acetonitrile and shaken for 10 min. The absorbance of solution was measured at 275 nm and compared to a standard curve of yarrow oil. Encapsulation efficiency is percentage (w/w) of the encapsulated compounds from the initial compounds used for inclusion complex formation. The major pure compound of yarrow oil (carvacrol) was also encapsulated and a standard curve of carvacrol was also prepared with the same procedure as yarrow oil.
3.2. Characterization of encapsulated yarrow essential oil
3.2.1. Morphological examination
The particle morphology of the encapsulated yarrow oil was examined using a Quanta 250 scanning electron microscope (SEM) (Quanta 250, Netherlands). The samples (powder of encapsulated yarrow oil and free HPβCD) were fixed on aluminum stubs with double adhesive tape and vacuum coated with a fine layer of gold before viewing under 500 times magnification. Observations were carried out with voltage acceleration of 20 Kv (Guimaraes et al., Citation2015).
3.2.2. FT-IR analysis
The Fourier transformed infrared spectroscopy (FT-IR) spectra of HPβCD, free yarrow oil and encapsulated yarrow oil were collected from 400 to 4000 cm−1 using a Nicolet 550-II FT-IR spectrophotometer (Nicolet, U.S.A.) with 32 scans at a resolution of 4 cm−1. The powder samples (free HPβCD and encapsulated yarrow oil) were diluted with potassium bromide (KBr) powder at a mass ratio of 1:100. Then they were ground and pressed to disks of diameters of 8 mm. A drop of yarrow oil sample was spread on a piece of KBr window uniformly then nipped with another piece of KBr window. FT-IR spectra were analyzed by the spectrophotometer software (OMNIC 5.2) (Gomes, Petito, Costa, Falcao, & Araujo, Citation2014; Wang, Li, Si, & Chen, Citation2011).
3.2.3. UV–vis spectroscopy analysis
The formation of inclusion complex of HPβCD and yarrow essential oil was demonstrated by a UV–vis spectrophotometer (Biochrom, LIBRA-S22, England). Yarrow oil was dissolved in acetonitrile (0.5 mg of yarrow oil/1 mL of acetonitrile). The HPβCD, physical mixture (HPβCD: yarrow oil = 4:1 in a mass ratio) and inclusion complex were diluted in acetonitrile (5 mg/1 mL acetonitrile) and shaken for 10 min. The supernatant was separated by centrifugation (8000 rpm/10 min) and then diluted by 100 times in acetonitrile. The diluted supernatant was scanned using a UV–vis spectrophotometer in the range of 200–400 nm to obtain the UV–vis absorption spectrum (Liu et al., Citation2013; Wang et al., Citation2011).
3.2.4. Phase solubility study
Classification of inclusion complex formation and stability of the inclusion compounds were evaluated by phase solubility study (Higuchi & Connors, Citation1965). The 2 mL of yarrow oil was added to 10 mL aqueous solutions of HPβCD ranging in concentration from 0 to 10 mmol/L and incubated at 25°C and 35°C for 24 h with shaking at 200 rpm. The solution was filtered through 0.45-mm PTFE filters (IC Millex-LH, Millipore, Billerica, MA) prior to measurement to remove any insoluble material. The quantity of yarrow oil remaining in solution was measured spectrophotometrically at 275 nm and compared to a standard curve of carvacrol. The quantity of yarrow oil in the solution was plotted against HPβCD concentration. The inclusion complex was classified according to the pattern of the plot between concentration of HPβCD and solubilized guests with the presence of HPβCD in solution. The stability constant, Ks (L/mol), was calculated from slope and intercept of the plot following Eq. (1):
where Ks (L/mol) is a stability constant, intercept (mmol/L) is the dissolved guest in the aqueous complexation medium when no cyclodextrin is present.
3.3. Evaluation of antioxidant activity
The samples (free yarrow oil and encapsulated yarrow oil) were exposed to sunlight for 12 h. The antioxidant activity of samples was determined by 1, 1-diphenyl-2-picrylhydrazyl (DPPH) radical scavenging assay (Brand-Williams, Cuvelier, & Berset, Citation1995) with some modifications. An ethanolic solution of the radical DPPH• (0.12 mM) was prepared and protected from light. The stock solution of free and encapsulated yarrow oil was prepared in 50% dimethylsulfoxide (DMSO) and kept in the dark. A 500 μL of free and encapsulated yarrow oil in several concentrations (5–50 µg/mL) was mixed with 500 μL of a 0.12 mM DPPH solution. The mixture was kept in darkness for 60 min and the absorbance was measured at wavelength of 515 nm. The DPPH scavenging (%) was calculated according to the equation:
where Atest is the absorbance of the sample with DPPH; Ablank is the absorbance of the sample without DPPH; Acontrol is the absorbance of DPPH without sample.
The antioxidant activity of free yarrow oil and encapsulated yarrow oil was compared to the chemical antioxidant, butylated hydroxytoluene (BHT).
3.4. Evaluation of antibacterial activity
Antibacterial activity of free and encapsulated yarrow oil was evaluated against S. aureus and E. coli by broth microdilution assay (Hill et al., Citation2013). For preparation of the tested samples, the encapsulated yarrow oil was diluted directly in the culture broth, while the free yarrow oil was prepared as aqueous microemulsion containing 10% (w/w) ethanol and 1% (w/w) Tween 20 (Basheer, Noordin, & Ghareeb, Citation2013). For broth microdilution assay, 20 µL of microbial suspension (cultured at 37°C/24 h and diluted to yield 105 CFU/mL) was added to 180 µL of Muller–Hinton broth (MHB) containing various concentrations of free yarrow oil and encapsulated yarrow oil ranged from 2 to 2000 μg/mL in each well of microtiter plate (amount of encapsulated yarrow oil was based on encapsulation efficiency).
Negative control wells were prepared with sterile culture medium (MHB) containing tested samples (free yarrow oil and encapsulated yarrow oil). Positive control wells were prepared with microbial suspension inoculating in culture medium (MHB) and culture medium containing Tween 20 and ethanol in the same concentration as yarrow oil microemultion. The microtiter plate was incubated at 37°C for 24 h and the turbidity was determined by a microplate reader (PowerwaveX Biotek, U.S.A.) at 600 nm. The minimum inhibitory concentrations (MICs) of free and encapsulated yarrow oil were recorded as the lowest concentration where no visible growth (≤0.05 changed in OD600) was observed in the wells after 24 h incubation.
The viability of the test microorganisms in the culture broth, which showed no turbidity at (OD600) was determined by transferring and spreading the culture broth on Muller–Hinton agar and incubated at 37°C for 24 h. The lowest concentrations of free yarrow oil and encapsulated yarrow oil required to completely destroy the test microorganisms (no growth on the agar plate) after incubation were reported as minimum bactericidal concentrations (MBCs). All determinations were done in triplicate on two separate runs.
3.5. Statistical analysis
The data were calculated with mean values and standard deviations (mean ± SD) from triplicate trials. Statistical significance of the results was evaluated by one-way analysis of variance (ANOVA) with Duncan’s multiple range tests (p < 0.05) using the Statistical Package for Social Sciences (SPSS) version software 10.
4. Results and discussion
4.1. Encapsulation efficiency of yarrow essential oil
shows encapsulation efficiency values of yarrow oil and carvacrol (yarrow oil major component) which were 45.05% and 86.59%, respectively. This difference in encapsulation efficiency between carvacrol and yarrow oil would result from the presence of other yarrow oil components (such as 1,8 cineole, thymol, camphor and linalool), which have also high affinities for CD (Astray, Mejuto, Morales, Rial-Otero, & Simal-Gándara, Citation2010; Haiyee et al., Citation2009; Jiang, Tan, Tan, & Li, Citation2016; Kfoury, Auezova, Fourmentin, & Greige-Gerges, Citation2014; Kfoury et al., Citation2016; Numanoǧlu et al., Citation2007; Tao, Hill, Peng, & Gomes, Citation2014). These components then competed with the major compound for inclusion complex formations with the HPβCD. Kamimura et al. (Citation2014) reported that the encapsulation efficiency values of pure carvacrol in HPβCD prepared by kneading and freeze-drying methods were around 78% and 84%, respectively. Chemical components from yarrow plant was also encapsulated in the other carriers such as alginate–chitosan system and the system exhibited 82.4% efficiency of polyphenol encapsulation (Belscak-Cvitanovic et al., Citation2011; Dordevic et al., Citation2015). This technique provided high encapsulation efficiency of phenolic compounds extracted from aqueous phase of yarrow plant. Thus, there have not been reported the encapsulation of essential oil from this plant.
Table 1. Encapsulation efficiency value determined of yarrow oil and carvacrol encapsulated in HPβCD.
Tabla 1. Determinación del valor de la eficiencia de encapsulación del acetie de milenrama y carvacrol en in HPβCD.
4.2. Characterization of encapsulated yarrow oil
4.2.1. Morphological examination
The particle morphology of the encapsulated yarrow essential oil was then observed under a SEM to characterize the evidence of inclusion formation compared with the free HPβCD. shows SEM images of free HPβCD () and encapsulated products () at 500 times magnifications. The particle shape and morphology of the encapsulated yarrow oil were similar to those of the free HPβCD (), whereas the agglomeration of the free HPβCD occurred (). It indicates the hydrogen bonding of the free HPβCD molecules interact with each other in solution producing the cluster of HPβCD (Batzdorf & Muller-Goymann, Citation1993; Le Bas & Rysanek, Citation1987). This case not occurs in inclusion complex because inclusion complex formation also induces the conformation change of cyclodextrin and obstructs the agglomeration among them. Similar observations have been previously reported that the distribution of inclusion complex of carvacrol and β-cyclodextrin, and the gathering of free β-cyclodextrin were also found (Guimaraes et al., Citation2015).
Figure 1. SEM micrographs of free HPβCD (a) and encapsulated yarrow oil (b) at 500 times magnification. The samples used were in powder form derived from inclusion complex formation via freeze-drying technique.
Figura 1. Microfotografías SEM de HPβCD libre (a) y aceite de milenrama encapsulado (b) con 500 aumentos. Las muestras empleadas en forma de polvo derivadas de la formación de complejos de inclusión mediante la técnica de liofilización.
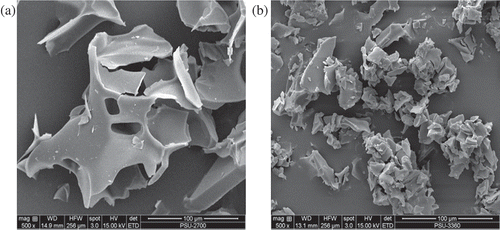
4.2.2. FT-IR analysis
FT-IR technique was used to confirm the achievement of encapsulation process by investigating the variation of shape, intensity and position of peaks (Szente, Citation1996; Zeng, Fang, & Ji, Citation2012). The FT-IR spectra of free HPβCD, free yarrow oil and encapsulated yarrow oil show in . The FT-IR spectrum of yarrow oil () shows prominent absorption bands at 2956 cm−1 for =CH2 symmetrical stretching vibration, 2926 cm−1 for C–H stretching vibration of methylene group, 2869 cm−1 for –CH stretching, 1652 cm−1 for H–O–H bending, 1626 cm−1 for C=C stretching vibration of the allyl group, 1446 cm−1 for C–H scissoring vibration, 1380 cm−1 for symmetrical deformation vibration of –CH3, 1366 cm−1 for symmetrical deformation vibration of –CH3, 1240 and 1103 cm−1 for P–O and P=O, 1022 cm−1 for C–O–C stretching vibration, 916 cm−1 for C–S–C stretching vibration, 875 and 865 cm−1 for C–H bending of aromatic ring. The spectrum of HPβCD () shows prominent absorption bands at 3406 cm−1 for O–H stretching vibration, 2970 cm−1 for =CH2 symmetrical stretching vibration, 2930 cm−1 for C–H stretching vibration, 1646 cm−1 for H–O–H bending vibration, 1157 cm−1 for C–O–C asymmetrical stretching vibration, 1083 and 1033 cm−1 for symmetric C–O–C stretching vibration (Wang, Luo, & Xiao, Citation2014). The FT-IR spectrum of inclusion complex was identical to HPβCD and no feature similar to yarrow oil. The results indicated that all the absorption bands of yarrow oil were covered by HPβCD in the spectrum of inclusion complex () indicating the entering to the cavity of HPβCD and the formation of inclusion complex.
Figure 2. FT-IR spectra of yarrow oil (a); hydroxypropyl-β-cyclodextrin (HPβCD) (b); encapsulated yarrow oil (c).
Figura 2. Espectro FT-IR para el aceite de milenrama libre (a); HPβCD (b); aceite encapsulado (c).
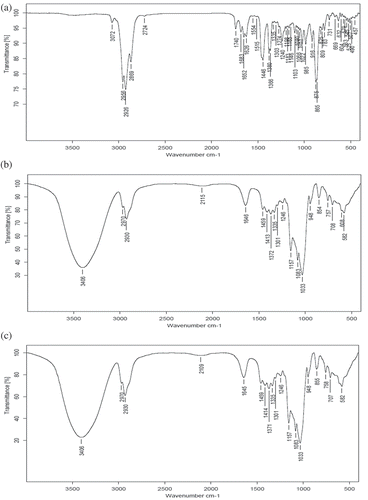
Similar observations have been reported. The inclusion complex formation of soybean lecithin and β-cyclodextrin was previously determined by Wang et al. (Citation2014) using FT-IR. All the absorption bands of soybean lecithin were not detected in the absorption spectrum of the inclusion complex. Those were related to the inclusion complex formation of β-cyclodextrin and soybean lecithin which whole of the soybean lecithin could be contained in the cyclodextrin cavity. The absorption bands of β-caryophyllene encapsulated in β-cyclodextrin were covered by β-cyclodextrin spectrum showing that inclusion complex of β-cyclodextrin and β-caryophyllene was formed. Gomes et al. (Citation2014) reported different results that the prominent absorption band at the 1738 cm−1 region of red bell pepper pigments encapsulated in β-cyclodextrin was observed from FT-IR spectrum indicating that some region of the guest was not contained in the cavity of β-cyclodextrin.
4.2.3. UV–vis spectroscopy analysis
The results in showed the UV absorption spectra of free HPβCD, yarrow oil, carvacrol, encapsulated yarrow oil, encapsulated carvacrol and extracted encapsulated compounds. The UV absorption spectrum of yarrow oil is shown in Line 2. The absorption peaks at 270–275 nm indicated the presence of carvacrol, 1,8-cineole, thymol and camphor. A minor peak at 243 nm attributed to linalool. The spectra of the physical mixture of HPβCD with yarrow oil (Line 4) and with pure compound (carvacrol) (Line 5) conformed to UV spectra of yarrow oil (Line 2) and pure carvacrol (Line 3), respectively. When the active compounds in yarrow oil or carvacrol were entrapped with HPβCD, the absorption peaks of each compound also disappeared in the spectrum of the inclusion complexes (Line 6 and 7). After extraction from the inclusion complex, the absorption peaks of entrapped compounds in yarrow oil appeared at 270–275 nm implying carvacrol and also be 1,8-cineole, thymol, camphor and linalool (Line 8). In this study, the chemical components of yarrow oil were successfully entrapped in the HPβCD. However, the encapsulation efficiency of yarrow oil was much lower than those of its pure compound. This was likely because the competition of major active compound among other components in essential oil has occurred during inclusion complex formation.
Figure 3. UV absorption spectra of free HPβCD (1), free yarrow oil (2), free carvacrol (3), physical mixture of yarrow oil and HPβCD (4), physical mixture of carvacrol and HPβCD (5), encapsulated yarrow oil (6), encapsulated carvacrol (7), compounds extracted from encapsulated yarrow oil (8).
Figura 3. Espectro de absorción UV para HPβCD libre (1), aceite ensencial libre (2), carvacrol libre (3), mecla fisica de aceite sencial y HPβCD (4), mezcla física de carvacrol y HPβCD (5), aceite encapsulado (6), carvacrol encapsulado (7), compuestos extraidos del aceite esencial encapsulado (8).
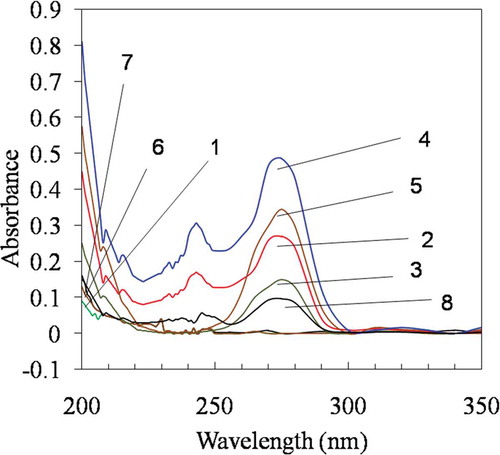
4.2.4. Phase solubility study
To evaluate the stability and to classify the inclusion complexes when they are in the solution, the phase solubility study is performed. The phase solubility profiles of the inclusion complexes can be established from the plots of host concentrations (HPβCD) against guest concentrations (encapsulated compounds) dissolved in the solution (). Type of inclusion complex can be considered from the plot curve.
Figure 4. Phase solubility diagram of encapsulated yarrow oil and carvacrol at specified temperatures.
Figura 4. Diagrama de fase para el aceite de milenrama y carvacrol encapsulados a diferentes temperaturas.
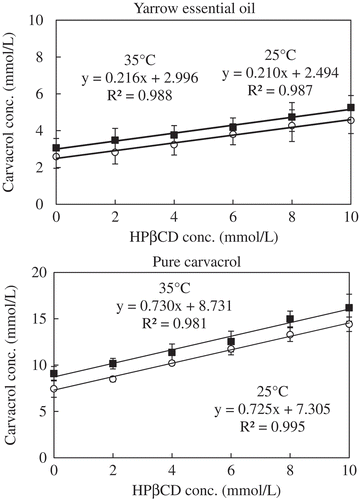
A linear relationship between the amount of dissolved yarrow oil and the concentration of HPβCD in this study with slope less than one was classified as a typical AL-type (type A reveals an inclusion complex formation where the amount of entrapped essential oil increases as the HPβCD concentration increases, subscript L indicates a 1:1 molecular ratio formation of soluble complexes) (Higuchi & Connors, Citation1965). A 1:1 inclusion complex formation can be observed when the major entrapped compounds are monoterpenoids/sesquiterpenoids or phenylpropane derivatives with an average molecular weight of 120–160 g/mol (Szente & Szejtli, Citation2004). The molar ratio of host-to-guest molecules is usually 1:1 for inclusion complexes formed in solution, except for complexes with long-chain or bifunctional guest molecules (e.g. guest molecules having two aromatic rings on opposite sides of a small central molecule segment).
As the y-intercepts are concentrations of essential oils or pure compounds solubilized in the solution without cyclodextrin, the y-intercepts for both yarrow oil and carvacrol increased with temperature () indicated that the water solubility of inclusion complexes increased with increasing temperature (Yuan, Jin, & Li, Citation2008). The aqueous solubility of yarrow oil components or carvacrol increased with increasing HPβCD concentration indicating that after inclusion complex formation the solubility of yarrow oil has been improved.
In solution, a fundamental parameter such as stability constant (Ks) of inclusion complex formation can be used to evaluate the stability of the inclusion complexes. The Ks can be obtained from the linear portion of the phase solubility diagrams (the plot of host concentration against guest concentration dissolved in the solution). Difference in stability constant (Ks) between yarrow oil and carvacrol () is due to difference in equilibrium between free and complex states (Higuchi & Connors, Citation1965). The decrease in Ks values with increasing temperatures was expected for exothermic processes (Hill et al., Citation2013).
Table 2. Phase solubility parameters and stability constants (Ks) of encapsulated yarrow oil and carvacrol at specified temperatures.
Tabla 2. Parámetros de solubilidad y constantes de estabilidad (Ks) para el aceite de milenrama y carvacrol encapsultado a diferentes temperaturas.
Similar observations have been reported. Kamimura et al. (Citation2014) reported that water solubility of the pure carvacrol increased and the Ks value decreased with increasing temperatures. Hill et al. (Citation2013) also found that the water solubility of pure compounds (trans-cinnamaldehyde and eugenol) and essential oils (from cinnamon bark and clove bud extract) increased with increasing temperatures, while the Ks values decreased with increasing temperature.
4.3. Evaluation of antioxidant activity of yarrow oil encapsulated
shows antioxidant activity in term of DPPH radical scavenging capacity (%) of free yarrow oil and encapsulated yarrow oil at concentration ranged from 5 to 50 μg/mL compared to a synthetic chemical antioxidant, butylated hydroxytoluene (BHT). It should be noted that the free HPβCD did not show antioxidant activity. The DPPH radical scavenging capacity of free yarrow oil or encapsulated yarrow oil increased with increasing sample concentrations.
Figure 5. DPPH radical scavenging (%) of free and encapsulated yarrow oil before and after exposure to sunlight for 12 h at various concentrations (5–50 μg/mL). Reaction temperature: room temperature (28 ± 2°C). Reaction time: 60 min. Concentration of encapsulated yarrow oil based on the encapsulation efficiency. Values given are in triplicate (n = 3).
Figura 5. Depuración de radicales DPPH (%) de aceite de milenrama libre y encapsulado antes y después de la exposición a la luz solar durante 12 h a diversas concentraciones (5–50 μg/mL). Temperatura de reacción: temperatura ambiente (28 + 2°C). Tiempo de reacción: 60 min. Concentración de aceite encapsulado basado en la eficiencia de encapsulación. Los valores dados están en triplicado (n = 3).
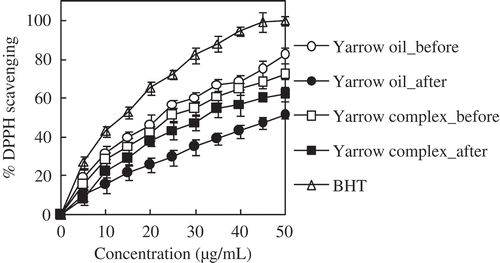
Yarrow oil containing carvacrol as a major component shows strong antioxidant activity (72% DPPH radical scavenging at 50 μg/mL) (). The most effective antioxidants usually contain aromatic or phenolic rings which interrupt the free radical chain reaction by donating H• to the free radicals (Nawar, Citation1996). The encapsulated yarrow oil gave slightly lower antioxidant activity than the activity of the free yarrow oil (). It was a result of the HPβCD was blocking the functional groups of active compounds during reacting with DPPH radicals (Kamimura et al., Citation2014). However, the encapsulation has been reported to increase the stability of the essential oils (Hedges et al., Citation1995; Qi & Hedges, Citation1997).
As the light impacts on the functional groups of active compounds in essential oils, the effect of sunlight on DPPH radical scavenging capacity of yarrow oil was determined ( and ). The DPPH radical scavenging of yarrow oil with concentration range from 5 to 35 μg/mL decreased around 41–51% after exposure to sunlight for 12 h. The yarrow oil with higher concentration range (40–50 μg/mL) exhibited lower loss of DPPH radical scavenging (36–37%). Obviously, the encapsulation of yarrow oil in HPβCD could protect the active components against the effect of sunlight. The complexation with HPβCD improved the stability of yarrow oil by 27–30%.
Figure 6. DPPH radical scavenging (%) of free yarrow oil and encapsulated yarrow oil before and after exposure to sunlight for 12 h at 50 μg/mL. Reaction temperature: room temperature (28 ± 2°C). Reaction time: 60 min. Concentration of encapsulated yarrow oil based on the encapsulation efficiency. Values given are in triplicate (n = 3).
Figura 6. Depuración de radicales DPPH (%) de aceite de milenrama libre y encapsulado, antes y después de la exposición a la luz solar durante 12 h a 50μg/mL. Temperatura de reacción: temperatura ambiente (28 + 2°C). Tiempo de reacción: 60 min. Concentración de aceite encapsulado basado en la eficiencia de encapsulación. Los valores dados están en triplicado (n = 3).
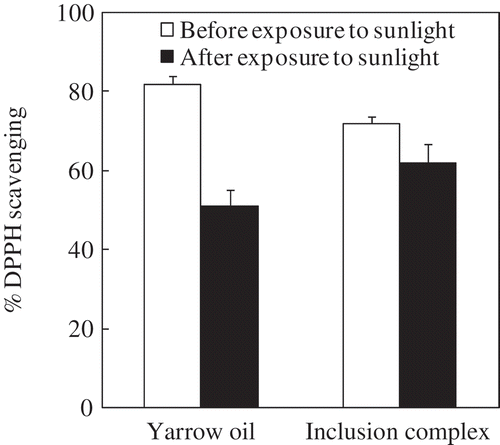
4.4. Antimicrobial activity of encapsulated yarrow oil
shows MIC and MBC values of free and encapsulated yarrow oil. Yarrow oil exhibited antibacterial activity against S. aureus and E. coli with the MIC values of 250 μg/mL and 500 μg/mL, respectively. The antimicrobial activity of yarrow essential oil might be because its oxygenated phenolic compounds, such as carvacrol and thymol, have been reported to give strong antimicrobial activity. These compounds were found to increase membrane permeability and membrane disruption of microbial cells (Pseudomonas aeruginosa and S. aureus) (Lambert, Skandamis, Coote, & Nychas, Citation2001). Antimicrobial potential of the oxygenated phenolics were also reported by Couladis, Tzakou, Kujundzić, Soković and Mimica-Dukić (Citation2004), Soković, Grubišić and Ristić (Citation2005), Soković, Tzakou, Pitarokili and Couladis (Citation2002), Soković and Van Griensven (Citation2006). In addition, S. aureus, a representative for gram-positive bacteria, was more sensitive to tested samples than E. coli. This was because the external surface of outer membrane of E. coli that composes of lipopolysaccharides and proteins is more tolerate to the tested samples, and the O-side chains of the lipopolysaccharides of E. coli has a hydrophilic surface protecting the hydrophobic molecules to enter the bilayer (Zengin & Baysal, Citation2014).
Table 3. Minimum inhibitory concentration (MIC) and minimum bactericidal concentration (MBC) against Staphylococcus aureus and Escherichia coli for both free yarrow oil and encapsulated yarrow oil.
Tabla 3. Concentración inhibitoria mínima (MIC) y concentración bactericida minima (MBC) frente Staphylococcus aureus y Escherichia coli del aceite de milenrama tanto libre como encapsulado.
The antibacterial efficacy of yarrow oil was much improved after encapsulated in HPβCD by four and eight times against S. aureus and E. coli, respectively. It has been reported that the HPβCD could increase aqueous solubility of the compounds and improve antimicrobial activity of essential oils at lower concentration of active compounds (Hill et al., Citation2013). The primitive sites of antimicrobial action of essential oils are at the cell membrane and inside the bacterial cytoplasm and HPβCD may enhance the accession of essential oils to these regions by improving water solubility of essential oils (Wang et al., Citation2011).
5. Conclusion
Encapsulation of yarrow essential oil in HPβCD was successfully performed as shown by SEM images, FT-IR spectra, UV–vis absorption spectra and phase solubility analysis with difference morphological and physiochemical characteristics of the encapsulated yarrow oil from the free one. Yarrow oil gave strong antioxidant activity of 72% DPPH scavenging at 50 μg/mL. Although encapsulation in HPβCD decreased the DPPH radical scavenging capacity of yarrow essential oil, it could protect the active components of the yarrow oil against the effect of sunlight by 27–30%. The encapsulation also improved the antibacterial activity of yarrow oil against S. aureus and E. coli by four to eight times over the free yarrow oil revealing that the encapsulation in HPβCD can also decrease the minimum concentration of antimicrobial compounds needed for inhibition of microbial growth.
Acknowledgments
This work was financially supported by the Graduate School of Prince of Songkla University and Thai Government under Grant No.AGR560387S.
Disclosure statement
No potential conflict of interest was reported by the authors.
Additional information
Funding
References
- Alfatemi, S.M.H., Rad, J.S., Rad, M.S., Mohsenzadeh, S., & Da Silva, J.A.T. (2014). Chemical composition, antioxidant activity and in vitro antibacterial activity of Achilleab wilhelmsii C. Koch essential oil on methicillin-susceptible and methicillin-resistant Staphylococcus aureus spp. 3 Biotech, 5, 39–44. doi:10.1007/s13205-014-0197-x
- Astray, G., Gonazlez-Barreiro, C., Mejuto, J.C., Rial-Otero, R., & Simal-Gándara, J. (2009). A review on the use of cyclodextrins in foods. Food Hydrocolloids, 23, 1931–1940. doi:10.1016/j.foodhyd.2009.01.001
- Astray, G., Mejuto, J.C., Morales, J., Rial-Otero, R., & Simal-Gándara, J. (2010). Factors controlling flavors binding constants to cyclodextrins and their applications in foods. Food Research International, 43, 1212–1218. doi:10.1016/j.foodres.2010.02.017.
- Baretta, I.P., Felizardo, R.A., Bimbato, V.F., Santos, M.G.J., Kassuya, C.A.L., & Junior, A.G. (2012). Anxiolytic-like effects of acute and chronic treatment with Achillea millefolium L extract. Journal of Ethnopharmacology, 140, 46–54. doi:10.1016/j.jep.2011.11.047
- Basheer, H.S., Noordin, M.I., & Ghareeb, M.M. (2013). Characterization of microemulsions prepared using isopropyl palmitate with various surfactants and cosurfactants. Tropical Journal of Pharmaceutical Research, 2, 305–310. doi:10.4314/tjpr.v12i3.5
- Batzdorf, T., & Muller-Goymann, C.C. (1993). Release of ketoprofen from aqueous systems in the presence of hydrophilic β-cyclodextrin derivatives. Pharmazeutische Industrie, 55, 857–860.
- Belscak-Cvitanovic, A., Stojanovic, R., Manojlovic, V., Komes, D., Cindric, I.J., Nedovic, V., & Bugarski, B. (2011). Encapsulation of polyphenolic antioxidants from medicinal plant extracts in alginate–chitosan system enhanced with ascorbic acid by electrostatic extrusion. Food Research International, 44, 1094–1101. 10.1016/j.foodres.2011.03.030
- Benedek, B., Geisz, N., Jager, W., Thalhammer, T., & Kopp, B. (2006). Choleretic effects of yarrow (Achillea millefolium s.L.) in the isolated perfused rat liver. Phytomedicine, 13, 702–706. doi:10.1016/j.phymed.2005.10.005
- Brand-Williams, W., Cuvelier, M.E., & Berset, C. (1995). Use of a free radical method to evaluate antioxidant activity. LWT - Food Science and Technology, 28, 25–30. doi:10.1016/S0023-6438(95)80008-5
- Candan, F., Unlu, M., Tepe, B., Daferera, D., Polissiou, M., Sokmenc, A., & Akpulat, H.A. (2003). Antioxidant and antimicrobial activity of the essential oil and methanol extracts of Achillea millefolium subsp. millefolium Afan. (Asteraceae). Journal of Ethnopharmacology, 87, 215–220. doi:10.1016/S0378-8741(03)00149-1
- Cavalcanti, A.M., Baggio, C.H., Freitas, C.S., Rieck, L., Sousa, R.S., Santos, J.E.S., … Marques, M.C.A. (2006). Safety and antiulcer efficacy studies of Achillea millefolium L. after chronic treatment in Wistar rats. Journal of Ethnopharmacology, 107, 277–284. doi:10.1016/j.jep.2006.03.011
- Chandler, R.F., Hooper, S.N., & Harvey, N.J. (1982). Ethnobotany and phytochemistry of yarrow Achillea millefolium Compositae, Economic Botany, 36, 203–215. doi:10.1007/BF02858720
- Couladis, M., Tzakou, O., Kujundzić, S., Soković, M., & Mimica-Dukić, N. (2004). Chemical analysis and antifungal activity of Thymus striatus Phytotherapy Research, 18, 40–42. doi:10.1002/ptr.1353
- Dordevic, V., Balanc, B., Belscak-Cvitanovic, A., Levic, S., Trifkovic, K., Kalusevic, A., … Nedovic, V. (2015). Trends in encapsulation technologies for delivery of food bioactive compounds. Food Engineering Reviews, 7, 452–490. Doi:10.1007/s12393-014-9106-7
- Garnero, C., Zoppi, A., Genovese, D., & Longhi, M. (2010). Studies on trimethoprim: Hydroxypropyl-β-cyclodextrin: Aggregate and complex formation. Carbohydrate Research, 345, 2550–2556. doi: 10.1016/j.carres.2010.08.018
- Gomes, L.M.M., Petito, N., Costa, V.G., Falcao, D.Q., & Araujo, K.G.L. (2014). Inclusion complexes of red bell pepper pigments with β-cyclodextrin: Preparation, characterisation and application as natural colorant in yogurt. Food Chemistry. 148, 428–436. doi:10.1016/j.foodchem.2012.09.065
- Guimaraes, A.G., Oliveira, M.A., Alves, R.S., Menezes, P.P., Serafini, M.R., Araujo, A.A.S., … Junior, L.J.Q. (2015). Encapsulation of carvacrol, a monoterpene present in the essential oil of oregano, with β-cyclodextrin, improves the pharmacological response on cancer pain experimental. Chemico-Biological Interactions. 227, 69–76. doi: 10.1016/j.cbi.2014.12.020
- Haiyee, Z.A., Saim, N., Said, M., Illias, R.M., Mustapha, W.A.W., & Hassan, O. (2009). Characterization of cyclodextrin complexes with turmeric oleoresin. Food Chemistry, 114, 459–465. doi:10.1016/j.foodchem.2008.09.072
- Hedges, A.R., Shieh, W.J., & Sikorski, C.T. (1995). Use of cyclodextrins for encapsulation in the use and treatment of food products. In S.J. Risch & G.A. Reineccius (Eds.). Encapsulation and controlled release of food ingredients, Vol. 590 (pp. 60–73). Washington, DC: American Chemical Society.
- Higuchi, T., & Connors, K.A. (1965). Phase solubility techniques. Advances in Analytical Chemistry and Instrumentation, 4, 117–122.
- Hill, L.E., Gomes, C., & Taylor, T.M. (2013). Characterization of beta-cyclodextrin inclusion complexes containing essential oils (trans-cinnamaldehyde, eugenol, cinnamon bark, and clove bud extracts) for antimicrobial delivery applications. LWT – Food Science and Techology, 51, 86–93. doi: 10.1016/j.lwt.2012.11.011
- Jiang, Z.T., Tan, J., Tan, J., & Li, R. (2016). Chemical components and molecular microcapsules of Folium Artemisia argyi essential oil with β-cyclodextrin derivatives. Journal of Essential Oil-Bearing Plants, 19, 1155–1169. doi:10.1080/0972060X.2016.1185973
- Jonsdottir, G., Omarsdottird, S., Vikingssona, A., Hardardottirc, I., & Freysdottir, J. (2011). Aqueous extracts from Menyanthes trifoliate and Achillea millefolium affect maturation of human dendritic cells and their activation of allogeneic CD4+ T cells in vitro. Journal of Ethnopharmacology, 136, 88–93. 10.1016/j.jep.2011.04.006
- Kamimura, J.A., Santos, E.H., Hill, L.E., & Gomes, C.L. (2014). Antimicrobial and antioxidant activities of carvacrol microencapsulated in hydroxypropyl-beta-cyclodextrin. LWT – Food Science and Technology, 57, 701–709. 10.1016/j.lwt.2014.02.014
- Karathanos, V.T., Mourtzinos, I., Yannakopoulou, K., & Andrikopoulos, N.K. (2007). Study of the solubility, antioxidant activity and structure of inclusion complex of vanillin with β-cyclodextrin. Food Chemistry, 101, 652–658. 10.1016/j.foodchem.2006.01.053
- Keser, S., Celik, S., Turkoglu, S., Yilmaz, O., & Turkoglu, I. (2011). Determination of antioxidant properties of ethanol and water extracts of Achillea millefolium L. (Yarrow). Asian Journal of Chemistry, 23, 3172–3176.
- Keser, S., Celik, S., Turkoglu, S., Yilmaz, O., & Turkoglu, I. (2013). Antioxidant activity, total phenolic and flavonoid content of water and ethanol extracts from Achillea millefolium L. Turkish Journal of Pharmaceutical Sciences, 10, 385–392.
- Kfoury, M., Auezova, L., Fourmentin, S., & Greige-Gerges, H. (2014). Investigation of monoterpenes complexation with hydroxypropyl-β-cyclodextrin. Journal of Inclusion Phenomena and Macrocyclic Chemistry, 80, 51–60. doi: 10.1007/s10847-014-0385-7
- Kfoury, M., Landy, D., Ruellan, S., Auezova, L., Greige-Gerges, H., & Fourmentin, S. (2016). Determination of formation constants and structural characterization of cyclodextrin inclusion complexes with two phenolic isomers: Carvacrol and thymol. Beilstein Journal of Organic Chemistry, 12, 29–42. doi: 10.3762/bjoc.12.5
- Lambert, R.J.W., Skandamis, P.N., Coote, P.J., & Nychas, G.J.E. (2001). A study of the minimum inhibitory concentration and mode of action of oregano essential oil, thymol and carvacrol. Journal of Applied Microbiology, 91, 453–462. doi:10.1046/j.1365-2672.2001.01428.x
- Le Bas, D., & Rysanek, N. (1987). Structural aspect of cyclodextrins. In D. Duchene (ed.), Cyclodextrins and their industrial uses. Paris: Editions de Sante. 105–211: 351–393.
- Liu, H., Yang, G., Tang, Y., Cao, D., Qi, T., Qi, Y., & Fan, G. (2013). Physicochemical characterization and pharmacokinetics evaluation of β-caryophyllene/β-cyclodextrin inclusion complex. International Journal of Pharmaceutics, 450, 304–310. doi:10.1016/j.ijpharm.2013.04.013
- Marques, H.M.C. 2010. A review on cyclodextrin encapsulation of essential oils and volatiles. Flavour and Fragance Journal, 25: 313–326. doi:10.1002/ffj.2019
- Nawar, W.F. (1996) Lipids. In: O. Fennema (Eds.), Food Chemistry, New York: Marcel Dekker, Inc.. pp 225–320.
- Numanoǧlu, U., Şen, T., Tarimci, N., Kartal, M., Koo, O.M.Y., & Önyüksel, H. (2007). Use of cyclodextrins as a cosmetic delivery system for fragrance materials: Linalool and benzyl acetate. AAPS Pharmscitech, 8, 34–42. 10.1208/pt0804085
- Potrich, F.B., Allemand, A., Silva, L.M., Santos, A.C., Baggio, C.H., Freitas, C.S., … Marques, M.C.A. (2010). Antiulcerogenic activity of hydroalcoholic extract of Achillea millefolium L.: Involvement of the antioxidant system. Journal of Ethnopharmacology, 130, 85–92. doi: 10.1016/j.jep.2010.04.014
- Qi, Z.H., & Hedges, A.R. (1997) Use of cylodextrins for flavors. In: C.T. Ho, C.T. Tan, & C.H. Tong (Eds.), Flavor technology: Physical chemistry, modification, and process, Vol. 610 (pp. 231–243). Washington, DC: American Chemical Society.
- Schmann, H.J., & Schollmeyer, E. (2002). Applications of cyclodextrinsin cosmetic products: A review. Journal of Cosmetic Science, 53, 185–191.
- Soković, M., Grubišić, D., & Ristić, M. 2005. Chemical composition and antifungal activities of essential oils from leaves, calyx and corolla of Salvia brachyodon Vandas. Journal of Essential Oil Research, 17, 227–229.doi: 10.1080/10412905.2005.9698884
- Soković, M., Tzakou, O., Pitarokili, D., & Couladis, M. (2002). Antifungal activities of selected aromatic plants growing wild in Greece. Nahrung, 46, 317–320. 10.1002/1521-3803(20020901)46:5<317::AID-FOOD317>3.0.CO;2-B
- Soković, M., & Van Griensven, L.J.L.D. (2006). Antimicrobial activity of essential oils and their components against the three major pathogens of the cultivated button mushroom, Agaricus bisporus, Agaricus Bisporus. European Journal of Plant Pathology, 116, 211–224. doi:10.1007/s10658-006-9053-0
- Szente, L. (1996). Analytical methods for cyclodextrins, cyclodextrin derivatives andcyclodextrin complexes. Supramolecular Chemistry, 3, 78–253.
- Szente, L., & Szejtli, J. (2004). Cyclodextrins as food ingredients. Trends in Food Science & Technology, 15, 137–142. 10.1016/j.tifs.2003.09.019
- Tao, F., Hill, L.E., Peng, Y., & Gomes, C.L. (2014). Synthesis and characterization of β-cyclodextrin inclusion complexes of thymol and thyme oil for antimicrobial delivery applications. LWT - Food Science and Technology, 59, 247–255. doi:10.1016/j.lwt.2014.05.037
- Trumbeckaite, S., Benetis, R., Bumblauskiene, L., Burdulis, D., Janulis, V., Toleikis, A., … Jakštas, V. (2011). Achillea millefolium L. s.l. herb extract: Antioxidant activity and effect on the rat heart mitochondrial functions. Food Chemistry, 127, 1540–1548. 10.1016/j.foodchem.2011.02.014
- Waleczek, K.J., Marques, H.M.C., Hempel, B., & Schmidt, P.C. (2003). Phase solubility studies of pure (−)-α-bisabolol and camomile essential oil with β-cyclodextrin. European Journal of Pharmaceutics and Biopharmaceutics, 55, 247–251. 10.1016/S0939-6411(02)00166-2
- Wang, T., Li, B., Si, H., & Chen, L. (2011). Release characteristics and antibacterial activity of solid state eugenol/β-cyclodextrin inclusion complex. Journal of Inclusion Phenomena and Macrocyclic Chemistry, 71, 207–213. 10.1007/s10847-011-9928-3
- Wang, X., Luo, Z., & Xiao, Z. (2014). Preparation, characterization, and thermal stability of β-cyclodextrin/soybean lecithin inclusion complex. Carbohydrate Polymers, 101, 1027–1032. 10.1016/j.carbpol.2013.10.042
- Wichtl, M. (1997). Teedrogen und phytopharmaka. Stuttgart: Wissenschaftl. Verlagsges. mbH pp. 395–399.
- Yuan, C., Jin, Z., & Li, X. (2008). Evaluation of complex forming ability of hydroxypropyl-β-cyclodextrins. Food Chemistry, 106, 50–55. 10.1016/j.foodchem.2007.05.045
- Zeng, Z., Fang, Y., & Ji, H. (2012). Side chain influencing the interaction between β-cyclodextrin and vanillin. Flavour and Fragrance Journal, 27, 378–385. 10.1002/ffj.v27.5
- Zengin, H., & Baysal, A.H. (2014). Antibacterial and antioxidant activity of essential oil terpenes against pathogenic and spoilage-forming bacteria and cell structure-activity relationships evaluated by SEM microscopy. Molecules, 19, 17773–17798. 10.3390/molecules191117773