ABSTRACT
Glutelins derived from oats were purified and hydrolyzed sequentially using alcalase proteases for production of antioxidant peptides. The antioxidant activities of oat glutelin peptides were evaluated using reducing power methods including hydroxyl and 1,1-diphenyl-2-pycrylhydrazyl (DPPH) radical-scavenging assays. The results demonstrated that oats glutelin peptide D-1a exhibited the highest antioxidant activity compared to the other hydrolysates. D-1a was identified to be His–Tyr–Asn–Ala–Pro–Ala–Leu (784.38 Da) by electrospray ionization mass spectrometry (ESI-MS/MS).
RESUMEN
Se purificó glutelina derivada de avena e hidrolizada secuencialmente utilizando proteasas de tipo alcalasa para la producción de péptidos antioxidantes. Se evaluó la actividad antioxidante de los péptidos de glutelina de avena utilizando métodos de poder reductor, como por ejemplo ensayos de barrido de radicales hidroxilo y 1,1-difenil-2-picrilhidrazil (DPPH). Los resultados demostraron que los péptidos de glutelina D-1a de la avena exhibieron la mayor actividad antioxidante en comparación con otros hidrolizados. Se identificó D-1a como His-Tyr-Asn-Ala-Pro-Ala-Leu (784,38 Da) mediante espectrometría de masa-ionización por electrospray (ESI-MS/MS).
Introduction
Proteins in oats have been shown to have high nutritional value and functional properties (Klose & Arendt, Citation2012). The major protein fraction of oats is globulin, and the glutelin content is also relatively high (20%~25%) (Hu, Citation2005). Oat glutelin hydrolysates have been shown to possess high antioxidant activity using in vitro analysis (Ma, Zhang, Bao, & Dong, Citation2014). The composition of oat protein molecules is complex and diverse, with variable sizes and structure. Protease treatment of these proteins can cause the formation of enzymatic hydrolysates which have potential bioactive functionalities. In order to characterize the functionalities of these enzymatic hydrolysates, they need to be isolated and purified to obtain specific active peptide fractions. And for determining the relationship between the structure and bioactive functionalities, the active components of the isolated peptide fractions are required to be identified and characterized.
Bioactive peptides generally contain 3–20 amino acid residues derived from a native protein hydrolysis using digestive (in vivo and in vitro) or microbial enzyme. The enzymatic hydrolysis of proteins is one of the common ways to release bioactive peptides; as a result, it improves protein functionalities and nutritional properties (Jun, Park, Jung, & Kim, Citation2004). The biological activity of the peptides is based on the amino acid composition (Gao, Yuan, Zhao, & Gao, Citation1998). During enzymatic hydrolysis, the molecular weight of the protein is reduced significantly. Literature indicates a strong correlation between activity of bioactive peptides and its relative molecular weight (Kim et al., Citation2013; Tsou, Kao, Lu, Kao, & Chiang, Citation2013).
Bioactive peptides can be used in the formulation of functional foods, and for the prevention of oxidative stress responsible for several chronic diseases. Natural antioxidants can be used at higher concentration as they are generally no known side effects (Aruoma, Citation1998; Lee, Koo, & Min, Citation2004). A number of studies have reported in vitro formation of antioxidative peptides from plant-based resources and their potential use as alternative antioxidants. Gao, Cao, and Li (Citation2010) studied antioxidant peptides from cottonseed protein. The hydrolysate derived from cottonseed protein, could be a natural source of antioxidant suitable for use as a food additive, other than used as forage in China. Phengnuam, Goroncy, Rutherfurd, Moughan, and Suntornsuk (Citation2013) studied the antioxidant peptides from Jatropha curcas and Zheng et al. (Citation2012) reported antioxidant properties of peanut peptide. J. curcas seedcake protein hydrolysates were determined to contain a mixture of compounds containing NH-residues, fatty acids, and fatty acid derivatives which exhibited high antioxidant activity. Soy protein and wheat gluten hydrolysates have shown to have strong antioxidant activities (Park, Morimae, Matsumura, Nakamura, & Sato, Citation2008). They found that peptide fractions exhibited higher antioxidant activities than crude enzymatic hydrolysates of gluten and soy protein; the authors also examined the mechanism underlying the antioxidant activities of peptides in food. In addition, the antioxidant activity of phaseolin and bean protein hydrolysates was reported by Carrasco-Castilla et al. (Citation2012). The authors concluded that the smaller molecular weight peptide fractions have higher antioxidant activity than original whole hydrolysate.
Therefore, the objective of the present investigation was to evaluate the antioxidative potentials and to identify the structure (molecular weight and amino acid sequence) of the bioactive peptides derived from oat glutelin proteins. Therefore, the oat glutelin extracts were enzymatically hydrolyzed by alcalase to obtain antioxidant peptides. The antioxidant activities of different oat glutelin hydrolysate fractions were then evaluated using free radical-scavenging assays. Furthermore, the amino acid sequence and molecular weight distribution were also determined to elucidate their relationship with antioxidant activity.
Materials and methods
Materials and reagents
Oats (Meng nong da yan No.1) were harvested in Inner Mongolia Agricultural University in 2014 and stored at 0°C ~ 4°C. High-performance liquid chromatography (HPLC)-grade acetonitrile and trifluoroacetic acid (TFA) were purchased from Sigma (Beijing, China). Alcalase, (Novozymes China Headquarters, 14 Xinxi Road, Shangdi Zone, Haidian distract, 100,085 Beijing China) and analytical-grade Folin–Ciocalteu, ammonia, glacial acetic acid, ammonium acetate, hydrochloric acid, and sodium hydroxide – were purchased from Tianjin Chemical Reagent Third Factory (Xuzhuangzi, Dongli distract, Tianjin China).
Preparation of enzymatic hydrolysates from oat glutelin
The Osborne method (Osborne, Mendel, Ferry, & Wakeman, Citation1914) was used to prepare oat glutelins.
Oat was ground using grinder (A11BS25; IKA Germany) and sifted through 80-mm mesh, 500 g of ground oat was defatted using Hexane at a ratio of 1:3 (w/v), by magnetic stirring and changing Hexane every 2 h. The total reaction time was 6 h. The defatted ground oat was dispersed fully in 6000 mL of 0.1 mol L−1 NaOH for 2 h at 4°C. The mixture was centrifuged at 10,000 × g for 15 min. The glutelins were precipated by adjusting the pH of the supernatant to 4.8 using 1.0 mol L−1 HCl. Oat glutelins were isolated and freeze-dried after centrifuging at 10,000 × g for 15 min. 5 g of oat glutelins was dispersed in 500 mL of deionized water at room temperature. The pH of the mixture was adjusted to 8.5. Enzyme (alcalase) was added to the above mixture at enzyme-to-substrate (E/S) ratio of 1:20 (v/w), which was incubated in a temperature-controlled water bath kept 55°C for 3 h and then heated at 96°C for 5 min to inactivate the enzyme. During the enzymatic hydrolysis, the pH of the mixture was kept constant by adding 0.5 mol L−1 of NaOH. The changes of the degree of hydrolysis were determined by the pH-stat method (Jens, Citation1986).
Isolation of oat glutelin hydrolysates using ion-exchange chromatography
Oat glutelin hydrolysates were then purified by ion-exchange chromatography. Ammonia acetate buffer (pH 4.5) was taken to dissolve the hydrolysates at concentration of 24 mg mL−1. The same buffer which dissolved the hydrolysates was used to condition the column (2.6 cm × 60 cm) which was packed with 732 cation-exchange resin for 12 h. Then 0.2 mol L−1 ammonia was used to elute the sample at a flow rate of 1.0 mL min−1. The elution peaks were detected at 220 nm. The fraction which indicated the strongest free radical-scavenging activity was concentrated using a rotary evaporator, and lyophilized for 3 days for further purification.
Scavenging effect against hydroxyl radical
1 mL of ammonium ferrous sulfate (7.5 × 10–3 mol L−1), salicylic acid (7.5 × 10−3mol L−1), hydrogen peroxide (0.3%), and the hydrolysate or peptide extract were added in that order into a colorimetric tube, and the volume was set to 10 mL with deionized water. Extract was taken as reference to measure the absorbance at 510 nm after 30 min. The following formula was used to calculate hydroxyl radical-scavenging capacity (Ma et al., Citation2014):
where: Areference – the absorbance of added Fe2+ and H2O2; Acompare – the absorbance for hydroxyl radical system of added Fe2+, H2O2, and salicylic acid; Asample reference – the absorbance of added Fe2+, H2O2, and different extracts; Asample – the absorbance for hydroxyl radical system of added Fe2+, H2O2, different extracts, and salicylic acid.
Evaluation of DPPH radical-scavenging activity
DPPH radical-scavenging capacity was determined by the method following Brand-Williams et al., (Citation1995), with minor modification. Briefly, 2 mL of deionized water and hydrolysate or peptide extract were added to 2 mL of 0.1 mmol/L DPPH radical solution freshly made in ethanol, respectively, followed by vortexing to dissolve. After the reaction continued 30 min at room temperature in the dark, spectrophotometer was used to measure the absorbance at 517 nm to get A0 and A1. 2 mL of hydrolysate or peptide extract was added to an equal volume of ethanol, and measured at the same condition to get A2. The following calculation was used:
where: A0 – the absorbance of 2 mL deionized water added 2 mL DPPH solution;
A1 – the absorbance of 2 mL hydrolysate or peptide extract added 2 mL DPPH solution; and A2 – the absorbance of 2 mL hydrolysate or peptide extract added 2 mL ethanol.
Purification of the glutelin peptide fraction by high-performance liquid chromatography (HPLC) using a C18 column
The fraction exhibiting the highest antioxidant activity was dissolved in deionized water and further purified using reversed-phase high-performance liquid chromatography (RP-HPLC) on a C18 column (7.8 mm × 300 mm) with a linear gradient of acetonitrile (5–10% in water) at a flow rate of 3.0 mL min−1. The elution peaks were detected at 220 nm. The eluates were concentrated using a rotary evaporator, and lyophilized for 3 days. For further purification, the fraction with the highest hydroxyl radical-scavenging activity from RP-HPLC was dissolved in deionized water and loaded onto a C18 column (4.6 mm × 250 mm) with a linear gradient of acetonitrile (5–10%) at a flow rate of 0.4 mL min−1. Peptide peaks were collected, evaluated for antioxidant activity, and then lyophilized. The fraction which showed the highest hydroxyl radical-scavenging activity was collected to analyze for amino acid sequence.
Identification of peptides using ESI-MS/MS
The desirable fraction after final purification was dissolved in a mixture of 50% acetonitrile/50% H2O which also contains 0.1% TFA, and then loaded onto the mass spectrometer (Waters Synapt G1 HD-MS) with the following settings: capillary voltage 1.5 KV, cone voltage 35 V, MCP detector voltage 2300 V; mass spectrometer was operated in the positive electrosprayionization (ESI+) mode, via the electrospray interface. The high-purity nitrogen was used as a drying and ESI nebulizing gas. Spectra were recorded over the mass/charge (m/z) range 100–1000. The peptide sequencing was performed by processing the MS/MS spectra using BioTools (Version 3.0; Bruker Daltonics Inc., Billerica, MA, U.S.A.) as well as manual calculation.
Statistical analysis
The test was carried out in triplicate, and data were expressed as the mean ± standard deviation (SD). One-way analysis of variance and Duncan’s new multiple range test were used to determine the differences among these means at p < 0.05.
Results and discussions
Antioxidant activity of oat glutelin hydrolysates
As is shown in , the scavenging activity against hydroxyl and DPPH radical showed a quadratic upward trend when the concentrate of hydrolysate was increased. The IC50 of scavenging activity against hydroxyl radical was 3.93 mg mL−1. Since the chemical activity of the hydroxyl radical is the strongest among the oxygen radicals (You et al., Citation2002), the scavenging effect against hydroxyl of the alcalase 2.4 L hydrolysate was a good indication of its potential antioxidant activity. Alcalase 2.4 L hydrolysate also exhibited a strong capacity for DPPH radical, with an IC50 value of 2.32 mg mL−1. Gao et al. (Citation2010) reported that higher antioxidant cottonseed protein peptide fraction exhibited 52.67% and 72.19% for hydroxyl and DPPH free radical-scavenging activity at concentration of 10 mg mL−1, which was close to that of ascorbate. Phengnuam et al. (Citation2013) studied the J. curcas seedcake protein hydrolysate and the IC50 of scavenging effect against DPPH radical was 3.31 mg mL−1. And they came to conclusion that cottonseed protein peptide and protein hydrolysate of J. curcas seedcake had strong antioxidant activity. Therefore, the antioxidative oat glutelin hydrolysate was further isolated in order to identify the antioxidant peptides.
High-performance liquid chromatography (HPLC) using a C18 column
Oat glutelin hydrolysate was divided into four fractions after purified by ion-exchange chromatography. Fraction D exhibited the highest free radical-scavenging activity. Therefore, fraction D was selected for further purification. The lyophilized active fraction D was further separated by RP-HPLC on a C18 column (7.8 mm × 300 mm) using a linear gradient of acetonitrile (5–10%), and the fraction was divided into two subfractions (D-1 and D-2) (). The lyophilized active fraction D-1 had the higher hydroxyl radical-scavenging activity with an IC50 value of 0.82 mg mL−1 (). Fraction D-1 was further subjected to a secondary separation by RP-HPLC on a C18 column (4.6 mm × 250 mm) using a linear gradient of acetonitrile (5–10%), and fractionated into one main subfraction (). The fraction was pooled and lyophilized. Fraction D-1a exhibited strong hydroxyl radical-scavenging activity (IC50, 0.68 mg mL−1) (). To obtain a purified peptide, D-1a was further purified by a third separation on HPLC using a C18 column (4.0 mm × 250 mm). Finally, the purified antioxidant peptide was isolated from the oat glutelin hydrolysate ().
Figure 2. RP-HPLC pattern on a C18 column (7.8 mm × 300 mm) of the active fraction D.
Figura 2. RP- Patrón HPLC en la columna C18 (7,8 mm × 300 mm) de la fracción activa D.
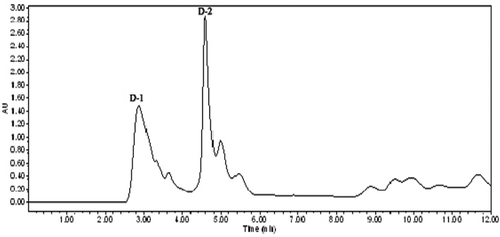
Figure 3. Hydroxyl radical-scavenging activity of the fractions after RP-HPLC pattern on a C18 column (7.8 mm × 300 mm) purification.
Figura 3. Actividad de barrido de radicales hidroxilo en las fracciones después del patrón RP- HPLC en la columna C18 (7,8 mm × 300 mm) purificación.
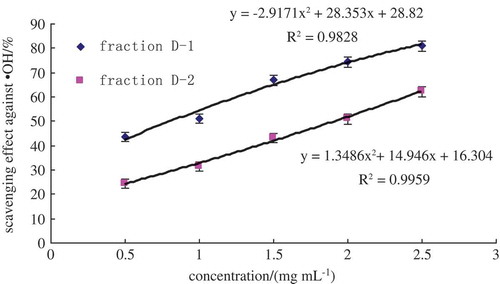
Figure 4. RP-HPLC pattern from a C18 column (4.6 mm × 250 mm) of the active fraction D-1.
Figura 4. Patrón RP-HPLC para la columna C18 (4,6 mm × 250 mm) de la fracción activa D-1.
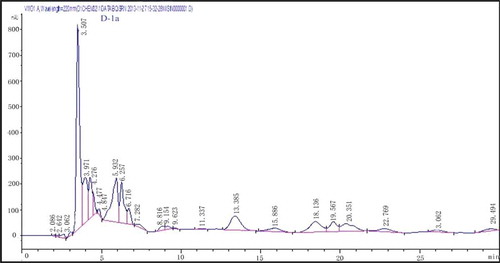
Figure 5. Hydroxyl radical-scavenging activity of the fraction after RP-HPLC pattern on a C18 column (4.6 mm × 250 mm) purification.
Figura 5. Actividad de barrido de radicales hidroxilo en la fracción después del patrón RP-HPLC en la columna C18 (4,6 mm × 250 mm) purificación.
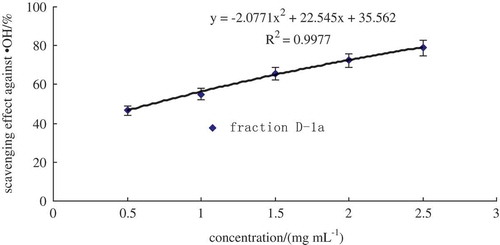
Figure 6. RP-HPLC with C18 column (4.0 mm × 250 mm) of the active fraction D-1a.
Figura 6. RP-HPLC con la columna C18 (4,0 mm × 250 mm) de la fracción activa D-1a.
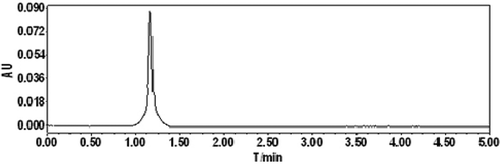
Hydroxyl radicals are highly reactive, and they can easily react with biomolecules, such as proteins, amino acids, lipids, and DNA (Je, Qian, Byun, & Kim, Citation2007). Thus, the removal of excess amount of hydroxyl radicals is probably one of the most effective defenses of a living body against various diseases. Therefore, the determination of hydroxyl radical-scavenging activity of the bioactive peptide provides valuable information to evaluate the potential antioxidant activity of the oat glutelin hydrolysates.
Comparison of free radical-scavenging activity of oat glutelin hydrolysates before and after purification
According to , IC50 of the scavenging effect against hydroxyl and DPPH radicals are 3.93 mg mL−1 and 2.32 mg mL−1. After purification by ion-exchange chromatography, fraction D which exhibited the highest free radical-scavenging activity, had an IC50 of 1.53 mg mL−1, and 1.28 mg mL−1 for hydroxyl and DPPH radical, respectively. The IC50 of scavenging effect against hydroxyl radical of fraction D-1 was 0.85 mg mL−1 and D-1a was 0.68 mg mL−1. The purification process caused an increase in the antioxidant activity of the oat glutelin hydrolysate fractions.
Table 1. IC50 of oat glutelin hydrolysates and purified fractions in free radical-scavenging assays.
Tabla1. IC50 de hidrolizados de glutelina de avena y fracciones purificadas en los ensayos de barrido de radicales libres.
Amino acid composition and sequence identification of the fraction D-1a
To identify the bioactive peptides, fraction D-1a was subjected to mass spectrometer evaluation which was operated in the positive electrosprayionization (ESI+) mode, via the electrospray interface. The MS spectrum of fraction D-1a is shown in , the fragmentation spectrum contained a major double-charged ion with MW of 393.19 Da. The molecular weight was 784.38 Da, which was consistent with the estimated molecular weight of the amino acid residues of the peptides. The MS/MS spectrum of a double-charged ion with m/z at 393.19 Da is illustrated in . The amino acid sequence was calculated using BioTools (Version 3.0; Bruker Daltonics), where each mass signal and corresponding fragmentation spectra could be matched to a single peptide fragment by manual calculation from the MS/MS spectrum (). The amino acid sequence was determined as His–Tyr–Asn–Ala–Pro–Ala–Leu.
The researchers suggested that the lower molecular weight peptides are the more efficient antioxidant and each molecular species of those bioactive peptides usually contain less than 20 amino acid residues (Pihlanto-Leppälä, Citation2000). In addition, low-molecular-weight peptides have better opportunity to cross the intestinal barrier and provide potential biological effects. Researchers have reported that molecular weight distribution dominates the antioxidant activity of enzymatic hydrolysates (Moure, Domínguez, & Parajó, Citation2006). A peptide fraction which has a molecular weight between 200 and 3000 Da may exhibit higher antioxidant activity as suggested by Li, Jiang, Zhang, Mu, and Liu (Citation2008). However, most food sources have antioxidative peptides ranged 500–1500 Da in size (Kou et al., Citation2013).
Other than the peptide size, certain amino acid residues and peptide sequences can also contribute to the overall antioxidant activity of a material (Suetsuna, Ukeda, & Ochi, Citation2000). The present study suggests that the most reactive amino acids had the following characteristics: aromatic (Trp, Tyr, and Phe), nucleophilic sulfur-containing (Cys and Met), and imidazole-containing (His). Some researchers have also suggested that the antioxidant activity of peptides often has direct relationship with the presence of one or several hydrophobic amino acid residues, such as Cys, His, Pro, Tyr, Trp, and Met, in their sequences (Elias, Kellerby, & Decker, Citation2008). The antioxidant activity of the free amino acids is not so high as that of the peptides, which is attributed to the amino acid sequences providing unique physical and chemical properties to the peptides, in particular, the resultant radicals are stable and tend to inhibit oxidative reactions effectively (Elias et al., Citation2008). Furthermore, the presence of His and Pro, enhance the free radical-scavenging activity of peptide. For example, Pro–His–His (PHH) showed the highest antioxidant activity among the peptides tested previously, and there are good synergistic effects between PHH-containing peptide and lipid-soluble antioxidants, such as tocopherols and butylated hydroxyanisole (BHA) (Chen, Muramoto, Yamauchi, & Nokihara, Citation1996). The presence of aromatic amino acids in the peptide could also enhance its free radical-scavenging capacity since the hydroxyl group of aromatic amino acids could act as a hydrogen donor to free radicals. Although the previous studies were unable to define the relationship between the structure and activity of His-containing peptides, lipid peroxyl radical trapping, hydrogen donating ability, and/or the metal ion-chelating ability of the imidazole group may be attributing to the activity (Ma, Xiong, Zhai, Zhu, & Dziubla, Citation2010).
The hydrophobic amino acids in the purified peptide, can increase the solubility of the lipid peptides, and enhance lipid peroxides inhibitory activity subsequently, thereby, advance interaction with radical species. It has been reported that Ala, the nonpolar aliphatic amino acid, and hydrophobic PUFA react highly. As a result, the radical-mediated peroxidizing chain reaction could be inhibited. In general, composition, sequence, and molecular weight of amino acids play an important role in the antioxidant activity of peptides.
The antioxidant activity of oat glutelin peptides may be related to their different structure. There are an abundance of hydrophobic amino acid residues, with aromatic side-chains, which can be regarded as proton donors and direct free radical scavengers. The molecular structures of final purified peptides were composed of seven amino acids. It is further verified that 2–10 peptides had stronger antioxidant activity than the original protein and the polypeptides (Ma et al., Citation2010). Oat glutelin peptides show a promising natural antioxidant component for food and medicine.
Conclusion
The establishment of the bioactive potential of peptides and identification of possible mechanisms by which they can exert antioxidant activities in biological systems is the most challenging task in the antioxidant peptide research area at present.
In the present study, oat glutelin protein was effectively hydrolyzed by alcalase 2.4 L to obtain hydrolysates with strong antioxidant activities. Fraction D, which was initially purified by ion-exchange chromatography, was further purified by RP-HPLC. The molecular weight and sequence of the final purified antioxidant peptide D-1a were identified by ESI-MS/MS analysis as 784.38 Da and His–Tyr–Asn–Ala–Pro–Ala–Leu, respectively. The amino acid sequence of the peptide may be responsible for its antioxidant activities. Furthermore, this study demonstrated that the oat glutelin peptide, D-1a, had significantly higher antioxidant activity than other fractions. Hydrophobic amino acid residues, Pro and His, aromatic amino acid Tyr, and nonpolar amino acid Ala played an important role in its antioxidant activity.
Further investigations of individual oat glutelin compounds, in vivo antioxidant activity of these compounds, and different antioxidant mechanisms are warranted, and these investigations are ongoing in our lab.
Disclosure statement
No potential conflict of interest was reported by the authors.
Additional information
Funding
References
- Aruoma, O.I. (1998). Free radicals oxidative stress and antioxidants in human health and disease. Journal of the American Oil chemists’ Society, 75, 199–212. doi:10.1007/s11746-998-0032-9
- Brand-Williams, W., Cuvelier, M.E., & Berset, C. (1995). Use of a free radical method to evaluate antioxidant activity. lwt - Food Science and Technology, 28, 25–30. doi:10.1016/S0023-6438(95)80008-5
- Carrasco-Castilla, J., Hernández-Álvarez, A.J., Jiménez-Martínez, C., Jacinto-Hernández, C., Alaiz, M., Girón-Calle, J., … Dávila-Ortiz, G. (2012). Antioxidant and metal chelating activities of peptide fractions from phaseolin and bean protein hydrolysates. Food Chemistry, 135, 1789–1795. doi:10.1016/j.foodchem.2012.06.016
- Chen, H.M., Muramoto, K., Yamauchi, F., & Nokihara, K. (1996). Antioxidant activity of designed peptides based on the antioxidative peptide isolated from digests of a soy bean protein. Journal of Agricultural and Food Chemistry, 44, 2619–2623. doi:10.1021/jf950833m
- Elias, R.J., Kellerby, S.S., & Decker, E.A. (2008). Antioxidant activity of proteins and peptides. Critical reviews in Food Science and Nutrition, 48, 430–441. doi:10.1080/10408390701425615
- Gao, D.D., Cao, Y.S., & Li, H.X. (2010). Antioxidant activity of peptide fractions derived from cottonseed protein. Journal of the Science of Food and Agriculture, 90, 1855–1860. doi:10.1002/jsfa.4024
- Gao, R.M., Yuan, Z.B., Zhao, Z.Q., & Gao, X.R. (1998). Mechanism of pyrogallol autoxidation and determination of superoxide dismutase enzyme activity. Bioelectrochemistry and Bioenergetics, 45, 41–45. doi:10.1016/S0302-4598(98)00072-5
- Hu, X.Z. (2005). Advances in oats food processing and functional properties. Journal of Triticeae Crops, 25, 122–124 (in Chinese). ISSN: .
- Je, J.Y., Qian, Z.J., Byun, H.G., & Kim, S.K. (2007). Purification and characterization of an antioxidant peptide obtained from tuna backbone protein by enzymatic hydrolysis. Process Biochemistry, 42, 840–846. doi:10.1016/j.procbio.2007.02.006
- Jens, A.N. (1986). Methods in food protein hydrolysis in Enzymatic hydrolysis of food proteins (pp. 110–130). New York, NY: Elsevier Applied Science Publishers. ISBN: 0-85334-38-1
- Jun, S.Y., Park, P.J., Jung, W.K., & Kim, S.K. (2004). Purification and characterization of an antioxidative peptide from enzymatic hydrolysate of yellowfin sole (Limanda aspera) frame protein. European Food Research Technology, 219, 20–26. doi:10.1007/s00217-004-0882-9
- Kim, E.K., Oh, H.J., Kim, Y.S., Hwang, J.W., Ahn, C.B., Lee, J.S., … Park, P.-J. (2013). Purification of a novel peptide derived from Mytilus coruscus and in vitro/in vivo evaluation of its bioactive properties. Fish & Shellfish Immunology, 34, 1078–1084. doi:10.1016/j.fsi.2013.01.013
- Klose, C., & Arendt, E.K. (2012). Proteins in oats; their synthesis and changes during germination: A review. Food Science and Nutrition, 52, 629–639. doi:10.1080/10408398.2010
- Kou, X.H., Jie., G., Xue, Z.H., Zhang, Z.J., Wang, H., & Wang, X. (2013). Purification and identification of antioxidant peptides from chickpea (Cicer arietinum L.) album in hydrolysates. Food Science and Technology, 50, 591–598. doi:10.1016/j.foodchem.2015.02.046
- Lee, J., Koo, N., & Min, D.B. (2004). Reactive oxygen species, aging, and antioxidative nutraceuticals. Comprehensive Reviews in Food Science and Food Safety, 3, 21–33. doi:10.1111/j.1541-4337.2004.tb00058.x
- Li, Y.H., Jiang, B., Zhang, T., Mu, W.M., & Liu, J. (2008). Antioxidant and free radical scavenging activities of chickpea protein hydrolysate (CPH). Food Chemistry, 106, 444–450. doi:10.1016/j.foodchem.2007.04.067
- Ma, S., Zhang, M.L., Bao, X.L., & Dong, T. (2014). The purification of free radical scavenging peptides from naked oats glutelin produced by enzymatic hydrolysis. Journal of Food and Nutrition Research, 2, 675–680. doi:10.12691/jfnr-2-10-4
- Ma, Y.Y., Xiong, Y.L., Zhai, J.J., Zhu, H.N., & Dziubla, T. (2010). Fractionation and evaluation of radical scavenging peptides from in vitro digests of buckwheat protein. Food Chemistry, 118, 582–588. doi:10.1016/j.foodchem.2009.05.024
- Moure, A., Domínguez, H., & Parajó, J.C. (2006). Antioxidant properties of ultrafiltration-recovered soy protein fractions from industrial effluents and their hydrolysates. Process Biochemistry, 41, 447–456. doi:10.1016/j.procbio.2005.07.014
- Osborne, L.B., Mendel, L.B., Ferry, E.L., & Wakeman, A.J. (1914). Nutritional properties of proteins of maize kernel. Journal of Biological Chemistry, 18, 1–16.
- Park, E.Y., Morimae, M., Matsumura, Y., Nakamura, Y., & Sato, K. (2008). Antioxidant activity of some protein hydrolysates and their fractions with different isoelectric points. Journal of Agricultural and Food Chemistry, 56, 9246–9251. doi:10.1021/jf801836u
- Phengnuam, T., Goroncy, A.K., Rutherfurd, S.M., Moughan, P.J., & Suntornsuk, W. (2013). DPPH radical scavenging activity of a mixture of fatty acids and peptide-containing compounds in a protein hydrolysate of Jatropha curcas seed cake. Journal of Agricultural and Food Chemistry, 61, 11808–11816. doi:10.1021/jf4035964
- Pihlanto-Leppälä, A. (2000). Bioactive peptides derived from bovine whey proteins: Opioid and CE-inhibitory peptides. Trends in Food Science & Technology, 11, 347–356. doi:10.1016/S0924-2244(01)00003-6
- Suetsuna, K., Ukeda, H., & Ochi, H. (2000). Isolation and characterization of free radical scavenging activities peptides derived from casein. The Journal of nutritional Biochemistry, 11, 128–131. doi:10.1016/S0955-2863(99)00083-2
- Tsou, M.J., Kao, F.J., Lu, H.C., Kao, H.C., & Chiang, W.D. (2013). Purification and identification of lipolysis-stimulating peptides derived from enzymatic hydrolysis of soy protein. Food Chemistry, 138, 1454–1460. doi:10.1016/j.foodchem.2012.10.149
- You, H.J., Oh, D.H., Choi, C.Y., Lee, D.G., Hahm, K.S., Moon, A.R., & Jeong, H.G. (2002). Protective effect of metallothionein-III on DNA damage in response to reactive oxygen species. Biochimica Et Biophysica Acta (BBA) - General Subjects, 1573, 33–38. doi:10.1016/S0304-4165(02)00325-2
- Zheng, L., Su, G.W., Ren, J.Y., Gu, L.J., You, L.J., & Zhao, M.M. (2012). Isolation and characterization of an oxygen radical absorbance activity peptide from defatted peanut meal hydrolysate and its antioxidant properties. Journal of Agricultural and Food Chemistry, 60, 5431–5437. doi:10.1021/jf3017173