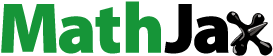
ABSTRACT
Pinctada fucata muscles were hydrolysed by alcalase and filtered using ultrafiltration membranes to obtain peptides with molecular weights (MWs) less than 5 kDa. The percolate was then freeze-dried and named crude antioxidant peptides mixture from Pinctada fucata muscles (AOP). In this study, AOP was purified sequentially using sephadex gel chromatography, next reversed-phase high-performance liquid chromatography. The MW of the antioxidant peptide from P. fucata muscle was 1039.56 Da. The amino acid sequence was Gly–Ala–Gly–Leu–Pro–Gly–Lys–Arg–Glu–Arg based on matrix-assisted laser desorption ionization mass spectrometry (MALDI-TOF). The natural peptide exhibits good scavenging capacity against free radicals; the IC50 of scavenging 2,2-diphenyl-1-picrylhydrazyl and ∙OH of FC2 were closer to vitamin C and butylated hydroxytoluence; however, the IC50 values of of FC2 were a bit poor. Its antioxidant activity was attributed to the hydrophobic amino acid residues enriched in the N-terminal and electrophilic ability mediated by Glu and electron acceptors such as Lys and Arg. The synthesized peptide exhibited a reduced ability to scavenge free radicals compared to the natural peptide. The proposed method is a feasible technique to prepare antioxidant peptides from P. fucata and could be useful to obtain ingredients in nutraceutical and cosmetic applications.
RESUMEN
Mediante el uso de alcalasa y filtrados se hidrolizaron músculos de Pinctada fucata, empleándose membranas de ultrafiltración con el objetivo de obtener péptidos cuyos pesos moleculares fueran inferiores a 5 kDa. Posteriormente, se liofilizó el percolado obtenido y se lo nombró como AOP. En el presente estudio, el AOP fue purificado secuencialmente utilizando cromatografía de gel sephadex y cromatografía líquida de alta resolución de fase inversa (RP-HPLC). El péptido antioxidante procedente de músculos de Pinctada fucata registró un peso molecular de 1039,56 Da. Su secuencia de aminoácidos, determinada mediante la espectrometría de masas MALDI-TOF, fue Gli–Ala–Gli–Leu–Pro–Gli–Lis–Arg–Glu–Arg. El péptido natural obtenido muestra una buena capacidad de eliminación de radicales libres: la IC50, con capacidad de eliminar DPPH y ·OH de FC2, resultó ser más cercana a la vitamina C (Vc) y al butihidroxianisol (BHT); sin embargo, en el caso de ·O2− de FC2 los valores de IC50 fueron más bajos. Su actividad antioxidante puede atribuirse a los residuales de aminoácidos hidrofóbicos enriquecidos en el extremo N-terminal Su capacidad electrofílica fue mediada por Glu y aceptadores de electrones como Lis y Arg. En comparación con el péptido natural, el péptido sintetizado mostró menos capacidad para eliminar radicales libres. El estudio permitió constatar que el método propuesto constituye una técnica viable para preparar péptidos antioxidantes de Pinctada fucata, lo que puede ser útil para obtener ingredientes en sus aplicaciones nutracéutica y cosmética.
Introduction
Natural antioxidant peptides have shown unrivalled strong antioxidant capacity and safety given their excellent physical and chemical properties, including thermal stability and solubility, which differ from synthetic antioxidants (Xie, Huang, Xu, & Jin, Citation2008). Peptide fragments with activity and function could be released from protein chain by specific protease enzymes, whereas the long protein chain does not exhibit these activities. However, the enzymatic hydrolysate was a mixture, and the antioxidant activity of different peptide fragments varied widely based on the amino acid composition, sequence and structure (Zou, He, Li, Tang, & Xia, Citation2016). Recent studies have focused on plant proteins, especially soybean proteins. For example, soybean antioxidant peptides ranging in size from 600 to 1700 Da were obtained by purification, and their sequence structures were explored using mass spectrometry (Chen, Muramoto, & Yamauchi, Citation1995; Liu et al., Citation2014). In addition, numerous antioxidant peptides were purified from milk proteins and aquacultures, and the active sequence was usually different.
At present, there are many researchers studying and explaining the antioxidant properties of peptides. However, the relationship between antioxidant properties and structure has not been fully clarified. However, most researchers agree that antioxidant peptides that contain 2–16 amino acid residues have strong antioxidant effects and are easily absorbed (Sarmadia & Ismaila, Citation2010; Tang et al., Citation2010).
Currently, numerous methods have been applied to the separation and purification of peptides, such as gel filtration chromatography, ion exchange chromatography, reversed-phase high-performance liquid chromatography (RP-HPLC) and high-performance capillary electrophoresis. Gel filtration chromatography (Pan, Cao, Guo, & Zhao, Citation2012) and high-performance liquid chromatography (HPLC) (Parente, Patel, Caldeo, Piraino, & McSweeney, Citation2012) are the most widely used techniques for isolation and identification of bioactive peptides. Mass spectrometry (MS) and protein sequence measuring are commonly used. Compared with electron spray ionization mass spectrometry (ESI-MS) (Shrivas, Kailasa, & Wu, Citation2009), matrix-assisted laser desorption ionization MS (MALDI-TOF–TOF) (Sheng, Covey, Shew, Winger, & Campana, Citation1994; Zhong, Zhang, Wen, & Li, Citation2004) is a more suitable structural analysis method for biological molecules, including proteins, oligosaccharides and peptides. Further studies on purification, structures, functions and mechanisms of peptides will be beneficial to the development and application of bioactive peptide in pharmaceutical, food and cosmetics industries (Li, Jia, & Yao, Citation2009).
Pinctada fucata is an important pearl-culturing mollusc shell. After the pearl is taken, the meat of P. fucata not only has high nutrition value but also contains rich functional active substances. Wu et al. (Citation2012) optimized alcalase to hydrolyse P. fucata muscle and found that the hydrolysis product has high antioxidant activity. The aim of this study was to purify antioxidant peptides mainly using sephadex gel chromatography and RP-HPLC. Moreover, sequence of antioxidant peptides was characterized via MALDI-TOF–TOF, amino acid composition analysis, infrared scans and chemical synthesis. Finally, the method for synthesis of peptides was described to explain the structure–activity relationship of antioxidant peptides from P. fucata muscle.
Materials and methods
Materials
P. fucata muscle was purchased from Hainan Aquaculture Base, South China Sea Fisheries Research Institute, Chinese Academy of Fishery Sciences. Alcalase was purchased from Novozymes Biotechnology Co., Ltd. (Beijing, China). Potassium bromide, cinnamylate (cyano-4-hydroxy-cinnamic acid [CHCA]), Fmoc-amino acids and Kaiser Reagents were of guaranteed reagents and purchased from Sigma-Aldrich. In addition, 2,2-diphenyl-1-picrylhydrazyl (DPPH), salicylic acid and Sephadex G-25 were purchased from Sigma-Aldrich. Acetonitrile and trifluoroacetic acid (TFA) was of LC grade and purchased from Anpel Scientific Co., Ltd. (Shanghai, China). All other reagents used in the experiment were of analytical grade and purchased from Sinopharm Chemical Reagent Co., Ltd. (Guangzhou, China).
Preparation of enzymatic hydrolysates of P. fucata muscle
P. fucata muscle was separated manually and homogenized using homogenizer (DS-1, Shanghai Specimen and Model Factory, Shanghai, China). Then, the homogenate was treated according to the previously described method (Pramanik et al., Citation2002) with a slight modification. The homogenate was mixed with phosphate buffer solution (PBS, 0.2 mol/L) at pH 6.0 at a ratio of 3:2 (w/v), and 5000 U/g alcalase was added. The mixture was subjected to ultrasonic wave treatment at 58 ± 2°C and 300 W for 17 min and then placed into water bath at 58 ± 2°C for 1.5 h. Samples were removed. Next, samples were heated in boiling water bath at 100°C for 10 min to deactivate enzyme. The hydrolysates were centrifuged at 10.000 r/min for 15 min.
The supernatant was filtered using two ultrafiltration (UF) membranes (Millipore ultrafiltration device, Dongrui Technology Co., Ltd., Guangdong, China) with 2-μm pores and a 5-kDa molecular weight (MW) cut-off to obtain peptides with MWs less than 5 kDa (Wu et al., Citation2013). The percolate was then freeze-dried (3K30 high-speed refrigerated centrifuge, Sigma, Germany) and stored at −20°C. The precipitate powder was dissolved in distilled water, diluted to 50 mg/mL and named AOP.
Gel filtration chromatography
AOP was purified using a Sephadex G-25 gel filtration column (1.6 × 40 cm, GE Ltd., USA) on the protein purification apparatus (AKTA purifier100, GE Ltd., USA), which had been previously equilibrated with ultrapure water and eluted with pH 7.0 ultrapure water at a flow rate of 1 mL/min. The fractions (F1, F2, F3, F4 and F5) with different molecular mass distribution were collected and lyophilized. Bacitracin (MW 1422.7 Da), oxidized glutathione (MW 612.6 Da), reduced glutathione (MW 307.32 Da) and l-tyrosine (MW 181.19 Da) were used as standards to measure the MWs of the five fractions under the same condition.
Purification of peptides by RP-HPLC
The fraction was further separated by RP-HPLC (2545 HPLC, Waters Ltd., USA) on a semi-preparative Zorbax SB C-18 column (9.4 × 250 mm, 5 μm, Agilent, USA). Peptides were collected with an automatic fraction collector from Waters Technologies. The detection was performed at 268 and 280 nm. The optimized conditions were as follows: mobile phase A, Milli-Q water/0.1% (v/v) TFA; mobile phase B, MeOH; flow rate, 2.0 mL/min; temperature, 30°C; and injection volume, 2.0 mL. The elution programme was as follows: 100% A (0–2 min), 100–90% A (2–5 min), 90–50% A (5–11 min), 50% A (11–13 min), 50–30% A (13–14 min) and 30% A (14–30 min). Five purified peptides named FC1, FC2, FC3, FC4 and FC5 were isolated, collected and lyophilized.
The fraction purity was identified by RP-HPLC (1100 Series, Agilent Ltd., USA). In total, 20 μL of fractions was analysed in a Phenomenex column (4.6 × 250 mm, 5 μm) at 268 and 280 nm using a gradient elution of Milli-Q water/0.1% (v/v) TFA and MeOH at 1.0 mL/min.
MALDI-TOF determination
The desirable antioxidant peptide fractions of FC2 after RP-HPLC purification were directed into the mass spectrometer (Ultraflex III TOF/TOF, Bruker Daltonics, Bremen, DE). On stainless steel plates of MALDI, 0.5 μL of the sample of matrix was added and dried. The matrix of α-CHCA (0.5 mg/mL) was dissolved in 50% ACN and 0.1% TFA (Sigma Chemical Co., St. Louis, MO, USA) with final concentration of 0.5 g/L in the solution. The spectra were obtained in reflector mode with an ion source voltage and reflected voltage of 8 and 29.5 kV, respectively, at a frequency of 200 Hz. The mass range for MS was between 800 and 1800 m/z. Multiple replicates of bands from different gels of the same strain were generally analysed (Li, Sadiq, Liu, Chen, & He, Citation2015). The software flexcontrol 3.3.85.0 was used for control and analysis (Lin et al., Citation2007). Observed experimental values for peptide masses were consistent with theoretical calculated values.
Amino acid composition analysis
Briefly, 0.3 g of FC2 was accurately weighed in a hydrolysis tube, and 10 mL of HCl (6 mol/L) was added for acidolysis. The tube was sealed after nitrogen filling three times and stored at 110°C for 22 h. After cooling and evaporating the excess of hydrochloric acid, the sample was repeatedly washed with pH 2.2 sodium citrate buffer solutions and subjected to instrumental analysis on an automatic amino acid analyser (835-50, Hitachi Ltd., Japan). The sample was treated in no. 2619 (52051) ion exchange column (2.6 × 150 mm) at a flow of 0.225 mL/min at 53°C for 72 min.
Synthesize of peptide
The novel antioxidant peptide was synthesized by Fmoc solid phase peptide synthesis using ASP48S (Peptron Inc.) (Domingues et al., Citation2013; Domingues, Riske, & Miranda, Citation2010) and identified using a Gemini-NX C18 column (4.6 × 250 mm, 5 μm). Elution was performed with a linear gradient of acetonitrile containing 0.1% (v/v) TFA and 0.1% (v/v) TFA in water. The molecular mass of the peptide was 1039.56 Da, and the amino acid sequence of the peptide was Gly–Ala–Gly–Leu–Pro–Gly–Lys–Arg–Glu–Arg based on electron spray ionization.
Reducing power
The reducing power was measured according to the previously reported method (Oyaizu, Citation1988; H.-C. Wu, Chen, & Shiau, Citation2003). A volume of 1 mL of the sample was added to 1 mL of 0.2 M phosphate buffer (pH 6.6) and the same volume of 1% potassium ferricyanide. The sample was incubated in a water bath at 50°C for 20 min. Then, 1 mL of 10% TCA was added to the reaction mixture. After centrifugation, 2 mL of the supernatant fluid was taken and mixed with 2 mL of distilled water and 0.4 mL of 0.1% ferric chloride, the mixture solution incubated for 10 min at 50°C in a water bath. The absorbance was measured at 700 nm. High absorbance indicated high reducing power.
DPPH radical scavenging activity
The DPPH assay was performed according to the previously described method (Wang, Li, Chi, Zhang, & Luo, Citation2012) with some appropriate modifications. Briefly, 0.5 mL of the sample was added to 0.5 mL of distilled water and 0.2 mM DPPH in 95% ethanol. The solution was incubated for 30 min at room temperature in the dark. This group was treated as the experimental group. The control sample, including DPPH solution without sample, was also prepared. In addition, 95% ethanol was substituted for DPPH solution in the blank sample. The absorbance was measured at 540 nm. In addition, the samples with different concentration were also measured. The antioxidant activity of the sample was evaluated by the half inhibition concentration (IC50). DPPH radical was calculated with the following equation:
where Ai was sample absorbance rate, A0 and Aj were the absorbance of control group and the blank, respectively.
Hydroxyl radical scavenging activity
Hydroxyl radical scavenging activity was measured according to the reported method (Ajibola, Fashakin, Fagbemi, & Aluko, Citation2011) with slight modifications. In the assay, hydroxyl radicals are briefly generated by the Fenton reaction (Richmond, Halliwell, Chauhan, & Darbre, Citation1981). Ai: 0.5 mL of the sample was mixed with 1, 10-phenanthroline solution (0.5 mL, 0.75 mM) and pH 7.4 PBS (1 mL, 0.15 M). FeSO4 solution (0.5 mL, 0.75 mM) was added into the mixture. The hydroxyl radicals were generated by 0.01% H2O2 (v/v, 0.5 mL). The solution was incubated for 60 min at 37°C in a water bath, next, measured under wavelength of 536 nm. Deionized water instead of the sample and repeat the operation H2O2 solution at 536 nm is measured for absorbance A0, deionized water instead of the sample solution to repeat the above operation, the absorbance measured at 536 nm Aj. The hydroxyl radical scavenging activity was calculated by the following formula:
Superoxide anion radical scavenging activity
Superoxide anion radical scavenging activity was measured by means of improved pyrogallol autoxidation (Marklund & Marklund, Citation1974) with some modifications. Briefly, the reaction was initiated by 0.1 mL of the sample, 2.8 mL of Tris–HCl buffer (pH 8.2, 0.1 M). The mixture was incubated in a 25°C water bath for 10 min, 0.1 mL of pyrogallic acid (3 mM) was added and absorbance was then measured at 325 nm every 30 s per 5 min. The blank contained an equal volume of deionized water instead of sample. The absorbance regression was drawn based on time, and its slope was marked as V, which indicated pyrogallol autoxidation rate. The capacity of scavenging the superoxide anion radical was calculated using the following equation:
where Vcontrol and Vsample were the absorbances of the control group without sample and the sample group, respectively.
Statistical analysis
Statistical analysis was performed using SPSS Statistic 17.0 (SPSS, Inc., Chicago, IL). Analysis of variance was applied, and every sample was analysed in triplicate to identify statistically significant differences using Duncan’s multiple range test (P < 0.05).
Results and discussion
Gel filtration chromatography of AOP and the antioxidant activity
As shown in , AOP was separated into five fractions (F1–F5). Four standards were used to indicate the MWs. The MW of AOP was primarily under 1 kDa. The MWs of F1–F5 were 1345.19, 989.13, 499.10, 309.91 and 102.14 Da, respectively.
The results of the reducing power, DPPH, hydroxyl and superoxide anion-free radicals scavenging ability expressed as IC50 were presented in . The DPPH IC50 of F2 at 4.64 mg/mL was slightly higher than F5 at 4.21 mg/mL. However, the ∙OH and IC50 values of F2 with the maximum reducing power were the minimum values, indicating that F2 was the strongest antioxidant component.
Table 1. Antioxidant capacity of F series of separations.
Tabla 1. Capacidad antioxidante de la serie de separaciones F.
As shown in , the purity analysis of F2 was performed via HPLC. The diagram clearly showed that F2 was still a mixture and required further purification. According to the conditions of gel separation, the F2 was enriched as much as possible before being evaporated, freeze-dried and resolved to 50 mg/mL for the next RP-HPLC purification.
Isolation of F2 peptides by RP-HPLC and their antioxidant activity
Using a 0.2-μm UF membrane, F2 was further separated by semi-preparative RP-HPLC on a Zorbax, SB C-18 column, and five purified peptides named FC1, FC2, FC3, FC4 and FC5 were separated as shown in . A variety of peptides were eluted on RP-HPLC given their different polarities, and this technique was popularly applied in the isolation and purification of small peptide segments (Dan, Ganesan, Flood, Tsai, & Reif, Citation2000), especially those less than 5000 Da (Sanz-Nebot, Benavente, Toro, & Barbosa, Citation2001).
Figure 3. The RP-HPLC purified profile by Semi-PrepHT C18 column of F2 separation.
Figura 3. El perfil purificado RP-HPLC mediante la columna Semi-PrepHT C18 de separación F2.
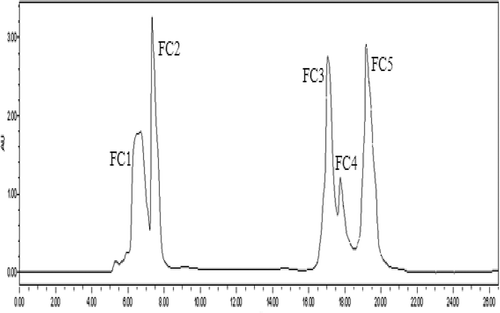
The antioxidant activities are presented in . Reducing power, DPPH, hydroxyl and superoxide anion-free radical scavenging ability presented as IC50 values of the FC series were compared with ascorbic acid (vitamin C [Vc]), which served as a positive control. The DPPH and ∙OH IC50 of FC2 were the lowest and similar to those of Vc. The IC50 value of FC4 (0.658 mg/mL) was the minimum value. Taking all indicators into consideration, the antioxidant ability of FC2 was the highest.
Table 2. Antioxidant capacity of FC series of components.
Tabla 2. Capacidad antioxidante de la serie de componentes FC.
FC2 purity analysis was performed (). The area of the peak at 17 min reached 65.4%, indicating that FC2 was considered to be of high purity.
Characterization and analysis of the structure–activity relationship
MALDI-TOF characterization
MALDI-TOF was used to characterize the amino acid sequence of FC2. The mass spectrum and the secondary mass spectrum are presented in and , respectively. In , the mass-to-charge ratio (m/z) of the fragment was 1040.56 Da, indicating that the MW of FC2 was 1039.56 Da.
Figure 6. The mass spectrum of the fraction FC2 (m/z: 1040.56).
Figura 6. El espectro de masa de la fracción FC2 (m/z: 1040.56).
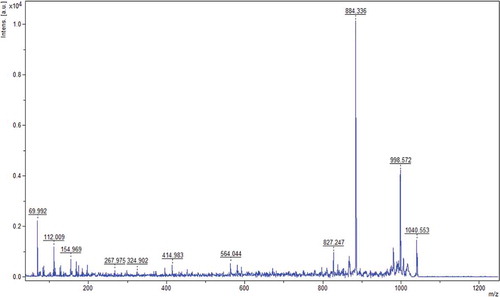
The molecular ion peak with the strongest signal was chosen for the secondary time of flight MS analysis. Using flexanalysis to analyse the spectrum, sequence information was inferred from the fragments, which was matched and compared with Blast sequence databases for proteins and polypeptides. The following sequences were obtained: GAGLPGKRER and GAGLPGKVWR. The corresponding amino acid sequences were Gly–Ala–Gly–Leu–Pro–Gly–Lys–Arg–Glu–Arg and Gly–Ala–Gly–Leu–Pro–Gly–Lys–Val–Trp–Arg, respectively.
Given the affinity of a basic amino acid for protons, these amino acids play a special role in peptide bond cleavage. The MW of the P. fucata antioxidant peptide was in the range of 750–1700 Da, which was consistent with the results of Wu et al., (Citation2003). In addition, the antioxidant peptides that were isolated contained the acidic amino acid Glu and the alkaline amino acids Lys and Arg, which are in line with Dr. Je, Qian and Kim (Citation2007) research results. Some researchers (Rival, Fornaroli, Boeriu, & Wichers, Citation2001; Suetsuna, Ukeda, & Ochi, Citation2000) found that the antioxidant activity was dependent not only on the content of hydrophobic amino acids but also on the position, especially in the C-terminus. It was inferred that FC2 with strong antioxidant activity was attributed to its electrophilic ability through Glu and the electron acceptors Lys and Arg. Moreover, a large amount of hydrophobic amino acids preferred to combine with hydrophobic groups, preventing the chain reaction by competing with substrate and performing its antioxidant activity (Chen, Muramoto, Yamauchi, & Nokihara, Citation1996). Combined with amino acid composition analysis, comprehensive analysis was necessary to determine the species and amounts of amino acids in a peptide.
Amino acid composition analysis
Some amino acids and their derivatives exhibited antioxidant capability, such as cysteine, tryptophan, tyrosine, leucine and so on. Some researchers found that amino acids containing sulphur, including cysteine and methionine (Qian, Jung, & Kim, Citation2008), could be directly related to free radicals or enzyme action, as an effective antioxidant in vivo (Hernández-Ledesma, Dávalos, Bartolomé, & Amigo, Citation2005). Togashi et al. (Citation2002) also extracted antioxidant peptides from collagen, which is enriched with hydroxyproline and proline. Parts of the amino acid composition of the oligopeptide are presented in .
Table 3. Results of some Pinctada fucata muscle oligopeptides amino acids composition analysis.
Tabla 3. Resultados de algunos análisis composicionales de aminoácidos oligopéptidos de músculos de Pinctada Fucata.
As shown in , Trp was not included in FC2. The result also showed that FC2 contained high levels of Glu, Leu, Lys and Arg, which was consistent with the result of the amino acid sequence. In addition, there were many active amino acids in the FC2, such as γ-aminobutyric acid, ornithine and taurine. γ-Aminobutyric acid is an important inhibitory neurotransmitter in the central nervous system. It is considered as a natural and non-protein amino acid that is beneficial for sleep, skin beauty and exhibits anti-epilepsy properties. Ornithine participates in urea cycle metabolism, transforming ammonia to urea for detoxification. Taurine plays a key role in infant growth and intellectual development. It also has biological functions of protecting eyesight and heart.
Identification of the synthetic peptide (Gly–Ala–Gly–Leu–Pro–Gly–Lys–Arg–Glu–Arg)
The peptide synthesized in a solid phase was identified by RP-HPLC and ESI-MS, and the chromatogram is presented in . The main peak of the total area occupied 98.35% at 10.85 min. The Fmoc-protected amino acid was a synthetic peptide of high purity under these conditions of identification and purification. In addition, the Kaise test was exacted under 180°C. The MW of synthetic peptide was 1039.33 Da, and the synthetic requirements were met.
Comparison of antioxidant activity between FC2 and the synthetic peptide
Synthetic peptides easily formed β-folding structures when the N-terminus was enriched in hydrophobic amino acid residues. The synthetic peptide in this article contained Gly and Pro, which unfolded the structure to eliminate the effect of the hydrophobic amino acids. presents the antioxidant activity of the natural antioxidant peptide (Gly–Ala–Gly–Leu–Pro–Gly–Lys–Arg–Glu–Arg) and synthetic peptide compared with reduced glutathione (GSH), Vc and butylated hydroxytoluence (BHT). The results showed that GSH is the strongest antioxidant capacity, followed by the Vc, BHT, FC2 and synthetic peptide in . Compared with FC2, synthetic peptide IC50 of scavenging DPPH and ·OH were 12 times and 3 times, respectively. However, the IC50 values of clearance and reducing power were similar, which potentially resulted from its possible secondary structure of natural sequences (Kashirin, Sibilev, Beloborodov, & Deigin, Citation2000). Although the chemical synthesis of linear peptide when dissolved N-terminal hydrophobic amino acid residues tends to β-folding structure, it contains Gly and Pro which can weaken fold and open the peptide structure. This may affect its oxidation resistance different from natural peptide. Compared with Vc and BHT, the IC50 of scavenging DPPH and ∙OH of FC2 were closer to Vc and BHT, but the IC50 values of
of FC2 were a bit poor, accounting for 23.81% of Vc and 28.57% of BHT, respectively.
Table 4. Antioxidant capacity of different components.
Tabla 4. Capacidad antioxidante de los distintos componentes.
Conclusion
In the present study, a new antioxidant peptide was isolated, purified and identified from P. fucata muscle. Its MW was 1039.56 Da, and the amino acid sequence was Gly–Ala–Gly–Leu–Pro–Gly–Lys–Arg–Glu–Arg. The antioxidant peptide from P. fucata had good scavenging capacity against free radicals, such as the IC50 of scavenging DPPH and ∙OH of nature antioxidant were closer to Vc and BHT, although the IC50 values of of FC2 were a bit poor. In addition, its antioxidant activity was attributed to its hydrophobic amino acid residues enriched in the N-terminus and its electrophilic ability mediated by Glu and electron acceptors, such as Lys and Arg. It also presumed that the synthesized peptide was inferior to the natural corresponding peptide regarding scavenging free radicals, but it was a feasible method to prepare antioxidant peptides. This research might provide the basis for further cloning expression research. The results indicated that the antioxidant peptides of P. fucata might be useful ingredients in nutraceutical and cosmetic applications.
Acknowledgements
This work was supported by the National Natural Science Foundation of China [Grant Numbers 31571869 and 31301454], The Special Scientific Research Funds for Central Non-profit Institutes, South China Sea Fisheries Research Institute, Chinese Academy of Fishery Sciencs [Grant Number 2012TS03], and Special Project of Marine fishery technology and industrial development in Guangdong province [Grant Number Z2015008].
Disclosure statement
No potential conflict of interest was reported by the authors.
Additional information
Funding
References
- Ajibola, C.F., Fashakin, J.B., Fagbemi, T.N., & Aluko, R.E. (2011). Effect of peptide size on antioxidant properties of African yam bean seed (Sphenostylis stenocarpa) protein hydrolysate fractions. International Journal of Molecular Sciences, 12(12), 6685–6702. doi:10.3390/ijms12106685
- Chen, H.-M., Muramoto, K., & Yamauchi, F. (1995). Structural analysis of antioxidative peptides from Soybean. beta.Conglycinin. Journal of Agricultural and Food Chemistry, 43(3), 574–578. doi:10.1021/jf00051a004
- Chen, H.-M., Muramoto, K., Yamauchi, F., & Nokihara, K. (1996). Antioxidant activity of designed peptides based on the antioxidative peptide isolated from digests of a soybean protein. Journal of Agricultural and Food Chemistry, 44(9), 2619–2623. doi:10.1021/jf950833m
- Dan, N., Ganesan, R., Flood, K.G., Tsai, D., & Reif, V.D. (2000). Determination of enantiomers in a synthetic argininal peptide using capillary zone electrophoresis and high-performance liquid chromatography. Journal of Chromatography A, 891(1), 115–127. doi:10.1016/S0021-9673(00)00621-X
- Domingues, T.M., Mattei, B., Seelig, J., Perez, K.R., Miranda, A., & Riske, K.A. (2013). Interaction of the antimicrobial peptide gomesin with model membranes: A calorimetric study. Langmuir, 29(27), 8609–8618. doi:10.1021/la401596s
- Domingues, T.M., Riske, K.A., & Miranda, A. (2010). Revealing the lytic mechanism of the antimicrobial peptide gomesin by observing giant unilamellar vesicles. Langmuir, 26(13), 11077–11084. doi:10.1021/la100662a
- Hernández-Ledesma, B., Dávalos, A., Bartolomé, B., & Amigo, L. (2005). Preparation of antioxidant enzymatic hydrolysates from α-lactalbumin and β-lactoglobulin. Identification of active peptides by HPLC-MS/MS. Journal of Agricultural and Food Chemistry, 53(3), 588–593. doi:10.1021/jf048626m
- Je, J.-Y., Qian, Z.-J., & Kim, S.-K. (2007). Antioxidant peptide isolated from muscle protein of bullfrog, Rana catesbeiana Shaw. Journal of Medicinal Food, 10(3), 401–407. doi:10.1089/jmf.2006.169
- Kashirin, D.M., Sibilev, A.V., Beloborodov, V.L., & Deigin, V.I. (2000). The use of IR spectroscopy for the identification of synthetic peptide preparations – Thymogen, thymodepressin, and neogen. Pharmaceutical Chemistry Journal, 34(11), 619–622. doi:10.1023/A:1010308708779
- Li, F., Jia, D., & Yao, K. (2009). Amino acid composition and functional properties of collagen polypeptide from Yak (Bos grunniens) bone. LWT-Food Science and Technology, 42(5), 945–949. doi:10.1016/j.lwt.2008.12.005
- Li, Y., Sadiq, F.A., Liu, T.J., Chen, J.C., & He, G.Q. (2015). Purification and identification of novel peptides with inhibitory effect against angiotensin I-converting enzyme and optimization of process conditions in milk fermented with the yeast Kluyveromyces marxianus. Journal of Functional Foods, 16, 278–288. doi:10.1016/j.jff.2015.04.043
- Lin, S., Lin, Z., Yao, G., Deng, C., Yang, P., & Zhang, X. (2007). Development of microwave‐assisted protein digestion based on trypsin‐immobilized magnetic microspheres for highly efficient proteolysis followed by matrix‐assisted laser desorption/ionization time‐of‐flight mass spectrometry analysis. Rapid Communications in Mass Spectrometry, 21(23), 3910–3918. doi:10.1002/rcm.v21:23
- Liu, P., Zhao, M.M., Cao, Y.L., Lu, Y.J., Liang, M., Huang, Z.B., & Ren, J.Y. (2014). Purification and identification of anti-oxidant soybean peptides by consecutive chromatography and electrospray ionization-mass spectrometry. Rejuvenation Research, 17(2), 209–211. doi:10.1089/rej.2013.1520
- Marklund, S., & Marklund, G. (1974). Involvement of the superoxide anion radical in the autoxidation of pyrogallol and a convenient assay for superoxide dismutase. European Journal of Biochemistry, 47(3), 469–474. doi:10.1111/ejb.1974.47.issue-3
- Oyaizu, M. (1988). Antioxidative activities of browning products of glucosamine fractionated by organic solvent and thin-layer chromatography. Nippon Shokuhin Kogyo Gakkaishi, 35(11), 771–775. doi:10.3136/nskkk1962.35.11_771
- Pan, D., Cao, J.H., Guo, H., & Zhao, B. (2012). Studies on purification and the molecular mechanism of a novel ACE inhibitory peptide from whey protein hydrolysate. Food Chemistry, 130(1), 121–126. doi:10.1016/j.foodchem.2011.07.011
- Parente, E., Patel, H., Caldeo, V., Piraino, P., & McSweeney, P.L.H. (2012). RP-HPLC peptide profiling of cheese extracts: A study of sources of variation, repeatability and reproducibility. Food Chemistry, 131(4), 1552–1560. doi:10.1016/j.foodchem.2011.10.003
- Pramanik, B.N., Mirza, U.A., Hain, Y., Liu, Y.H., Bartner, P.L., Weber, P.C., & Bose, A.K. (2002). Microwave‐enhanced enzyme reaction for protein mapping by mass spectrometry: A new approach to protein digestion in minutes. Protein Science, 11(11), 2676–2687. doi:10.1110/ps.0213702
- Qian, Z.-J., Jung, W.-K., & Kim, S.-K. (2008). Free radical scavenging activity of a novel antioxidative peptide purified from hydrolysate of bullfrog skin, Rana catesbeiana Shaw. Bioresource Technology, 99(6), 1690–1698. doi:10.1016/j.biortech.2007.04.005
- Richmond, R., Halliwell, B., Chauhan, J., & Darbre, A. (1981). Superoxide-dependent formation of hydroxyl radicals: Detection of hydroxyl radicals by the hydroxylation of aromatic compounds. Analytical Biochemistry, 118(2), 328–335. doi:10.1016/0003-2697(81)90590-X
- Rival, S.G., Fornaroli, S., Boeriu, C.G., & Wichers, H.J. (2001). Caseins and casein hydrolysates. 1. Lipoxygenase inhibitory properties. Journal of Agricultural and Food Chemistry, 49(1), 287–294. doi:10.1021/jf000392t
- Sanz-Nebot, V., Benavente, F., Toro, I., & Barbosa, J. (2001). Optimization of HPLC conditions for the separation of complex crude mixtures produced in the synthesis of therapeutic peptide hormones. Chromatographia, 53(S1), S167–S173. doi:10.1007/BF02490324
- Sarmadia, B.H., & Ismaila, A. (2010). Antioxidative peptides from food proteins: A review. Peptides, 31, 1949–1956. doi:10.1016/j.peptides.2010.06.020
- Sheng, L.-S., Covey, J.E., Shew, S.L., Winger, B.E., & Campana, J.E. (1994). Matrix‐assisted laser desorption ionization Fourier‐transform mass spectrometry. Rapid Communications in Mass Spectrometry, 8(6), 498–500. doi:10.1002/(ISSN)1097-0231
- Shrivas, K., Kailasa, S.K., & Wu, H.-F. (2009). Quantum dots–electrospray ionization mass spectrometry: 3‐mercaptopropanic acid capped CdS quantum dots as accelerating and enrichment probes for microwave tryptic digestion of proteins. Rapid Communications in Mass Spectrometry, 23(22), 3603–3607. doi:10.1002/(ISSN)1097-0231
- Suetsuna, K., Ukeda, H., & Ochi, H. (2000). Isolation and characterization of free radical scavenging activities peptides derived from casein. The Journal of Nutritional Biochemistry, 11(3), 128–131. doi:10.1016/S0955-2863(99)00083-2
- Tang, X., He, Z., Dai, Y., Xiong, Y.L., Xie, M., & Chen, J. (2010). Peptide fractionation and free radical scavenging activity of zein hydrolysate. Journal of Agricultural and Food Chemistry, 58, 587–593. doi:10.1021/jf9028656
- Togashi, S.-I., Takahashi, N., Iwama, M., Watanabe, S., Tamagawa, K., & Fukui, T. (2002). Antioxidative collagen-derived peptides in human-placenta extract. Placenta, 23(6), 497–502. doi:10.1053/plac.2002.0833
- Wang, B., Li, Z.-R., Chi, C.-F., Zhang, Q.-H., & Luo, H.-Y. (2012). Preparation and evaluation of antioxidant peptides from ethanol-soluble proteins hydrolysate of Sphyrna lewini muscle. Peptides, 36(2), 240–250. doi:10.1016/j.peptides.2012.05.013
- Wu, H.-C., Chen, H.-M., & Shiau, C.-Y. (2003). Free amino acids and peptides as related to antioxidant properties in protein hydrolysates of mackerel (Scomber austriasicus). Food Research International, 36(9–10), 949–957. doi:10.1016/S0963-9969(03)00104-2
- Wu, Y.Y., Li, L.H., Duan, Z.H., Yang, X.Q., Shang, J., & Chen, S.J. (2012). Application of response surface methodology to optimise preparation high antioxidant activity product from Pinctada fucata muscle. Advanced Materials Research, 396-398, 1341–1348. doi:10.4028/www.scientific.net/AMR.396-398.1341
- Wu, Y.Y., Tian, Q., Li, L.H., Khan, M.N., Yang, X.Q., Zhang, Z.M., … Chen, S.J. (2013). Inhibitory effect of antioxidant peptides derived from Pinctada fucata protein on ultraviolet-induced photoaging in mice. Journal of Functional Foods, 5(2), 527–538. doi:10.1016/j.jff.2013.01.016
- Xie, Z., Huang, J., Xu, X., & Jin, Z. (2008). Antioxidant activity of peptides isolated from alfalfa leaf protein hydrolysate. Food Chemistry, 111(2), 370–376. doi:10.1016/j.foodchem.2008.03.078
- Zhong, H., Zhang, Y., Wen, Z., & Li, L. (2004). Protein sequencing by mass analysis of polypeptide ladders after controlled protein hydrolysis. Nature Biotechnology, 22(10), 1291–1296. doi:10.1038/nbt1011
- Zou, T.B., He, T.P., Li, H.B., Tang, H.W., & Xia, E.Q. (2016). The structure-activity relationship of the antioxidant peptides from natural proteins. Molecules, 21(1), 72.