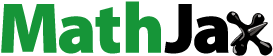
ABSTRACT
We investigated the grinding kinetics of purple-fleshed potato (PFP) and the effects of particle size and damaged starch content of PFP powder (PFP flour [PFPF]) on rheological and microstructural characteristics of noodle dough. Grinding kinetics of PFPF determined maximum grinding yield for each particle size. Before cooking, the particle size decrease of PFPF significantly increased dough hardness and elasticity while none of the differences showed between the doughs after cooking except ball-milled PFPF noodle dough. Maxwell and Peleg models for stress relaxation characteristics were found to be suitable for interpreting viscoelastic behaviour of dough with various sizes of PFPF. Additionally, microscopic structure based on SEM showed connectivity and gelatinization characters of the dough. Results of this study suggest that changes in physical properties of noodle dough prepared with PFPF associated with particle size and starch damage during grinding of PFPF must be considered for noodle processing.
RESUMEN
Investigamos la cinética de trituración de la papa de pulpa púrpura (PFP) y los efectos provocados por el tamaño de la partícula y el contenido de almidón dañado presente en la papa de pulpa púrpura en polvo (PFPF) en las características reológicas y microestructurales de la masa de fideo. Mediante la cinética de trituración de la PFPF se determinó el rendimiento máximo de la trituración para cada tamaño de partícula. Antes de la cocción, la disminución del tamaño de la partícula de la PFPF provocó un aumento significativo en la dureza y elasticidad de la masa; después de la cocción no se presentaron diferencias entre las masas, excepto en la masa de fideo procesada con molino de bolas (BM), añadida con PFPF. Se constató que los modelos de Maxwell y Peleg para determinar las características de relajación de estrés resultan adecuados a efectos de interpretar el comportamiento viscoelástico de la masa con distintos tamaños de PFPF. Asimismo, la estructura microscópica determinada con el MEB mostró la existencia de características de conectividad y gelatinización de la masa. Los hallazgos del presente estudio sugieren que, para el procesamiento de fideos, deben tomarse en cuenta los cambios asociados al tamaño de las partículas y al contenido de almidón dañado en las propiedades físicas de la masa de fideo preparada con PFPF.
Introduction
Potatoes are one of the primary crops consumed by the world’s population and important source of protein, vitamins, minerals, and phenolic compounds (Šimková, Lachman, Hamouz, & Vokál, Citation2013). In recent years, potatoes with coloured fleshed and related products have appeared in Europe and the United States (Rytel et al., Citation2014). Coloured potatoes could be potential source of anthocyanins as natural pigments with antioxidative properties for use in food and non-food industries (Lachman & Hamouz, Citation2005).
Noodles are popularly consumed throughout the world because of their convenience, ready to eat nature, favourable sensory attributes, and long shelf life (Yadav, Yadav, Kumari, & Khatkar, Citation2014). As the demands for healthy food have increased and have therefore impacted the noodle industry, a large number of noodle manufacturers have developed nutritionally enhanced noodles by adding natural ingredients other than wheat. Accordingly, numerous studies have shown the importance of substitutive ingredients that can significantly influence noodle processing and final quality (Chen et al., Citation2011; Li, Huang, Yang, & Wang, Citation2012). Therefore, understanding changes in physical structures and chemical components of dough and noodle due to addition of supplemental ingredients is of high interest for developing novel and high-quality noodles.
Powdering or flouring allows for the convenient use of a natural resource as an ingredient for noodle processing. In the production of food flour, various powdering techniques are used such as grinding, crushing, and milling, which employ mechanical force to break the dried food into fine particles. However, mechanical and friction force during grinding influence the degree of damaged starch and make a variation in particle size of flour. Therefore, developing a grinding kinetics model is efficient to determine and predict an optimum grinding time to obtain suitable particle sizes and levels of damaged starch for specific applications, including noodles or bakery dough.
Viscoelastic properties of noodle dough are highly related to characteristics of dough processing abilities such as flattening or sheeting and sensory perception that affect the final noodle quality. For this reason, changes in rheological properties of dough were measured with various methods. Traditional empirical instruments including farinograph, extensograph, and rapid visco-analyser have been used to evaluate dough properties. However, these methods have limitations to generate fundamental data (Faridi & Faubion, Citation2012). The application of stress relaxation might be the most accepted fundamental method with simple operation to evaluate viscoelastic properties of dough and noodles with quantitative measurements of viscosity, elasticity, and relaxation time. Stress relaxation measurements can improve our understanding of dough behaviour that highly influences the processing ability of dough and the quality of the final product.
Many studies have shown that doughs with various ingredients have different characteristics. However, there have been relatively few studies evaluating the effect of ingredient particle size on viscoelastic properties of dough or noodles. In addition, scientific studies for application of coloured potatoes are insufficient while there are new and growing industries in the world. Thus, the objective of this study was to investigate the effects of adding various particle sizes of purple-fleshed potato (PFP) flour (PFPF) into the dough on the properties of noodle dough. To achieve the objective, the grinding kinetics of PFPF and the stress relaxation models of noodle dough were developed and evaluated to control the particle size of PFPF and to characterize the noodle dough properties, respectively.
Materials and methods
Materials and powdering of the PFPFs
PFPs (Solanum tuberosum L.) were provided by the Korea Institute of Science and Technology (Gangneung, Gangwon, Korea). Wheat flour (Daehan Flour Co., Seoul, Korea) and salt (CJ CheilJedang, Seoul, Korea) for making noodle dough were purchased from the local market. The characteristics of wheat flour were as follows: crude protein content 9.27 ± 0.14%, wet gluten 22.46 ± 0.70%, and dry gluten 8.59 ± 0.16%. The methods used to determine wheat flour quality characteristics were American Association of Cereal Chemists (AACC) 46-13 for crude protein by the micro-Kjeldahl method and ISO 21415 for wet gluten by washing manually. The dry gluten was determined by baking in a drying oven at 130°C for 6 h.
The PFP was cut into a cylinder shape (diameter = 20 mm, height = 5 mm) and the cut samples were dried at 60°C for 8 h in a convection dryer (NB-901M, N-BIOTEK, Bucheon, Gyeonggi, Korea) according to the procedures of Moon, Pan, and Yoon (Citation2015). Grinding was carried out on 30 g of dried PFP samples using a 150-W domestic grinder (MFM 002H, Hibell, Hwaseong, Gyeonggi, Korea) in a 300-mL jar for 5, 10, 20, 30, 45, and 60 s. Various sizes of PFPFs were prepared by sifting the final particles with sieves of standard screen mesh sizes (2, 1.4, 1.18, 1, 0.6, 0.425, and 0.25 mm). In this study, the amounts of ground particles having specific sizes at various grinding times were measured, and these values were used to develop a kinetic model for the grinding process of PFPF (Lee, Lee, & Yoon, Citation2013). The kinetic equation representing the quantity of ground particles, of size i, at a given grinding time is obtained by solving the differential equation and the kinetic equation which is
where Pmax is the quantity of mesh with size i, the coefficients a and b are the grinding ability constant and the time (s) needed to grind 50% of the potato flour, respectively.
In addition to grinding methods mentioned above, ball milling was used to make superfine PFPF powders. Ball-milled fine flour was obtained in a 600-g batch of dried PFP. The average size of the ball-milled flour (BM) was measured by a particle analyser (Mastersizer 2000, Malvern Instruments Ltd., Malvern, Worcestershire, UK). The average size of wheat flour and BM were 50.84 ± 0.51 and 54.59 ± 1.16 μm, respectively.
Preparation of noodle dough
Flours of different particle sizes 425–600 (P1), 600–1000 (P2), and 1000–1150 (P3) μm and BM were prepared to investigate the effects of flour particle size on dough. Dough containing only wheat flour (W) was used as a control sample. The dough samples consisted of 30 g of each size of PFPFs, 70 g of wheat flour, 50 g of deionized distilled water, and 2 g of NaOH. At first, the flours were mixed in a bowl using a dough mixer (5K5SS, KitchenAid, St. Joseph, MI, USA) for 30 s for homogenization. Subsequently, water containing salt was added slowly for 30 s to obtain a uniform water distribution during the dough mixing. The mixture was blended in the bowl for 5 min until the dough was consistent, and the final dough product was stored in a polyethylene bag for 10 min at 25°C to stabilize the dough structure.
Starch damage assay
The degree of damage to starch was determined enzymatically according to the AACC method 76-31 with starch damage assay kit (Megazyme International Ireland, Ltd., Bray, Ireland). The 100-mg of samples and the control starch included in the assay kit were put into glass test tubes and incubated with 1 mL of purified fungal α-amylase at 40°C for 10 min to hydrolyse damaged starch into maltosaccharides and α-limit dextrins. The 8-mL of dilute sulphuric acid solution was added (0.2% v/v) to each tube to terminate the reaction and centrifuged at 1000 × g for 5 min. After that, 0.1 mL of purified amyloglucosidase (2 U) was added to 0.1 mL supernatant of the samples in glass tubes and incubated at 40°C for 10 min to provide a complete degradation of dextrins to glucose. A glucose oxidase peroxidase (GODPOD) reagent mixture (4 mL) was added to each tube including glucose standards, and the tubes were incubated at 40°C for 20 min. The damaged starch percentage of the samples was evaluated by the absorbance at 510 nm by UV–vis spectrophotometer. This analysis was performed in triplicate.
Microstructure of noodle dough
The microstructures of the fractured surface of raw and cooked noodle dough mixed with PFPF of various particle sizes were examined with a variable pressure field emission scanning electron microscope (VP-FESEM, Supra 55VP, Carl Zeiss, Oberkochen, Germany). Before examination by SEM, samples were freeze-dried at −40°C for 48 h. Dried samples were coated with gold and used at an accelerating potential of 2 kV.
Textural analysis
Texture profile analysis
The textural properties of dough with various particle sizes of PFPF were measured using a texture analyser (CT3, Brookfield, Middleboro, MA, USA). A 30-g sample of PFPF dough was formed into a cylindrical shape (38 mm diameter and 18 mm height) using a mould. The dough sample was cooked in boiling water (1000 mL of distilled deionized water) for 10 min and cooled at 25°C for 5 min. Texture profile analysis (TPA) was conducted on the raw and cooked dough using a cylindrical acrylic probe (38 mm diameter) with 0.5 mm/s of crosshead speed at 30% deformation based on the linear properties obtained from preliminary tests. Values of hardness, cohesiveness, springiness, gumminess, and chewiness were evaluated. The measurements were performed 10 times per treatment and the average value was used for further analysis. All experiments were conducted in triplicate.
Stress-relaxation test
The raw and cooked doughs (30 g) were prepared for stress-relaxation tests. The geometry of the samples for the stress-relaxation tests was the same as those used in the TPA tests (38 mm diameter and 18 mm height). Stress-relaxation test was conducted under uniaxial compression with an acrylic cylindrical probe with diameter of 38 mm at cross head speed of 0.3 mm/s and deformation of 3.6 mm from the top of the original sample. Deformation was determined in linear viscoelastic range using various deformation values (data not shown). The holding time was 600 s. Changes of force (or stress) during the holding time were recorded as a function of time.
The most frequently used models to define stress-relaxation behaviour of food are Maxwell model (Equation (2)), which comprises a spring (elastic) and a dashpot (viscous) in a series, and Peleg model (Equation (4)) that can analyse non-linear viscoelastic properties of food under large deformation (Peleg & Normand, Citation1983):
where n is the number of Maxwell bodies, Ei is the modulus of the spring of the Maxwell body, λi is the relaxation time of the Maxwell body, and Ee is the modulus of the lone spring. The stress is reduced exponentially during relaxation and the relaxation time (λ), which is defined as the ratio of the viscosity to the elasticity, was calculated as stated by the following equation:
The force relaxation data were normalized and then linearized according to the Peleg model:
where σ0 is the initial stress, σ is the decaying stress at time t, and k1 and k2 are constants. The results from these two models were compared to find a suitable model, which describes the viscoelastic behaviour of PFPF dough.
Statistical analysis
Differences between the experimental data were studied by one-way analysis of variance. The significant difference (P < 0.05) was evaluated using Duncan’s multiple range test with the software IBM SPSS Statistics 21 (IBM Corporation, New York, NY, USA). A curve fitting tool in Matlab® (R2015a, MathWorks, Natick, MA, USA) was used to find constants of grinding kinetic model and stress-relaxation models.
Results and discussion
Grinding kinetics of PFP
The changes in total mass of flour for each particle size during grinding showed a typical sigmoidal shape (). As grinding time was increased, grinding yield was dramatically increased until 30 s. It then reached an equilibrium state. The kinetic model (Equation (1)) was applied to characterize the grinding kinetics of PFP and evaluate the model parameters (). As the particle size decreased, more time was required to reach at a saturation stage. Higher values of a and b, and an increased grinding ability and time needed to grind 50% of the PFPF, were observed with smaller particle sizes. The coefficient Pmax, which represents the maximum quantity of ground PFPF passing through a sieve, was only 11.32 g out of 30.00 g with 0.250 mm diameter mesh size.
Table 1. Grinding kinetic parameters of dried purple-fleshed potato.
Tabla 1. Parámetros de la cinética de trituración de la papa de pulpa púrpura deshidratada.
Figure 1. Grinding kinetics of purple-fleshed potato flour (PFPF) by particle size during grinding.
Figura 1. Cinética de trituración de la harina de papa de pulpa púrpura (PFPF) según el tamaño de la partícula durante la trituración.
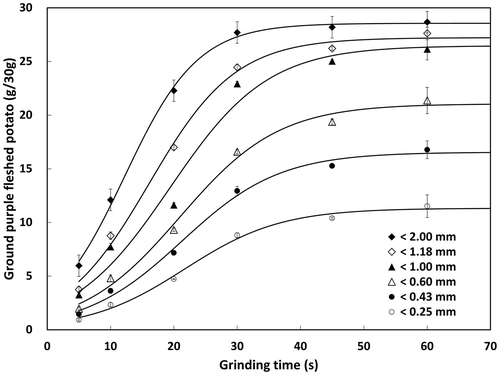
A few studies have shown that the grinding time is not always inversely proportional to particle size. In the case of soybean flours, the small size of soybean particles less than 0.15 mm with high moisture content (≥8%) showed drastically decreasing yield and grinding parameters due to agglomeration (Lee et al., Citation2013). In this study, the moisture content of dried PFP was low enough, at 3.66 ± 0.16%, to produce high yields of small particles. The grinding kinetics model was practically used for powdering process because the model could enable the prediction of grinding time required to obtain flour particles of suitable size and enable the estimation yield of a certain size of particle.
Effect of grinding on starch damage of PFPF
The amount of damaged starch was assessed for PFPF (P1, P2, and P3) with three different particle sizes and superfine particle sized PFPF (BM) (). The largest potato particle (P3) had the lowest degree of damaged starch content (1.83 ± 0.07%). There was a significant increase (P < 0.05) in the degree of damaged starch as the particle size was decreased. The highest degree of damaged starch was found in BM (32.76 ± 0.18%), which was smaller than the typical granule size of potato starch (15–75 μm) (Jane, Kasemsuwan, Leas, Zobel, & Robyt, Citation1994). In addition, friction, collision, shock, shearing, and other mechanical actions are involved in breaking the starch structure during ball milling (Liu, Ma, Yu, Shi, & Xue, Citation2011). This result implies that the high grinding energy for size reduction of PFPF might have induced a physical damage to starch granules. Thus, the degree of damaged starch was highly associated with particle size of PFPF, both correlated with noodle quality.
Table 2. Results of the texture profile analysis (TPA) of the dough with various sizes of PFPF.
Tabla 2. Resultados del análisis del perfil de textura (TPA) de la masa añadida con distintos tamaños de PFPF.
Effects of particle sizes of PFPF on textural properties of dough
The textural properties obtained from the TPA tests of the raw and cooked noodle dough are shown in . The dough with PFPF had significantly higher hardness, gumminess, and chewiness than control dough (W) before and after cooking. It might be due to the competiveness of water uptake between wheat gluten and potato components such as starch, protein, and fibre. Competitive absorption of water among different components in dough might have reduced the amount of water for softening and lubricating internal structures of dough, eventually increasing the resistance of doughs with PFPF to external forces with higher values of textural properties. This is in agreement with Shiau, Wu, and Liu (Citation2012), in that the addition of wheat fibre increased the required cutting force of raw and cooked noodles. It is an interesting observation that the hardness, gumminess, and chewiness of raw dough were reduced as the particle size of PFPF increased. These results may be due to less water absorption, which is associated with smaller surface area and a lower degree of starch damage in PFPF. The dough made from BM showed the highest values of hardness, gumminess, and chewiness due to the physically damaged starch spontaneously swells in cold water, forming a gel.
A decreased recovery was observed in raw doughs containing the largest particle size (P3). Cohesiveness and springiness in cooked dough showed no significant difference among PFPFs. Because of gelatinization of starch in dough may replace the role of gluten in cooked dough in terms of consistency and strength. Chen et al. (Citation2011) reported similar results that the hardness of cooked white Chinese noodles with wheat bran flour increased with decreasing wheat bran particle size from 1.72 to 0.21 mm.
Changes in TPA parameters after cooking showed a particle size dependence. Increasing ratios of each parameter before and after cooking are shown in . The smallest change of hardness, gumminess, and chewiness among treatments showed in BM dough, while these properties increased to a greater degree with increasing particle size in the PFPF doughs. Because highly damaged starches have the gel formation and swelling abilities at lower temperatures improved due to a higher degree of hydration.
Particle size dependence of viscoelastic properties of dough with PFPF
The stress-relaxation data of raw and cooked dough with various particle sizes of PFPF were fitted using a generalized three-element Maxwell model (Equation (2)) (). All stress-relaxation curves of the noodle dough followed a typical stress-relaxation decay pattern of other viscoelastic food materials. Bhattacharya (Citation2010) also have fitted the stress-relaxation behaviour of moth bean flour dough with the Maxwell model. Maxwell elements reflect the basic cellular structure of food on the viscoelastic behaviour.
Table 3. Coefficients of the Maxwell model and non-linear model of the raw and cooked doughs with various sizes of PFPF.
Tabla 3. Coeficientes del modelo de Maxwell y del modelo no lineal de masas crudas y cocidas añadidas con distintos tamaños de PFPF.
From data in , an addition of PFPF into the dough before cooking significantly increased in elastic components (Ei) and viscous components (ηi) but decreased relaxation times (λi). The damaged starch and water soluble components of PFPF leached out from potato powder and contributed to a cohesive and rigid dough. The decrease in particle size of PFPF in the noodle dough before cooking caused an increase in the magnitude of Maxwell elements. The significant increase of Maxwell elements in BM dough indicated that increasing amounts of damaged starch affected noodle structure more than particle size increase. A previous study (Hatcher, Edwards, & Dexter, Citation2008) has also found that the effect of damaged starch in wheat flour on mechanical properties of noodles is greater than that of particle size.
As shown in , the BM dough showed a higher resistance to the deformation of the dough because of the high surface area and damaged starch of BM reduced free water acting as a lubricant or a plasticizer in dough. At the end of relaxation, the relaxation decay curves converged on equilibrium values, known as residual stress. The residual stress Ee showed the highest value in raw BM dough (P < 0.05), indicating more solid-like sample with difficulty at flattening. Long relaxation time generally exhibits elastic characteristics (Bhattacharya, Citation2010). However, the portions of PFPF substituted into wheat flour may reduce the amount of gluten and shortened the relaxation time (Belton, Citation1999).
After cooking, there were no significant differences among the stress-relaxation parameters of PFPF dough. The gelatinization of less damaged starch in large particle sized flours contributes to the viscoelastic properties of cooked dough (noodles). This is in agreement with our TPA results described in .
The number of terms in the Maxwell model causes difficulty when describing their physical meaning because all the constants may vary independently (Peleg, Citation1979). To overcome difficulties of the Maxwell model, Peleg model has been widely used in food materials because of natural instability, degradation, or non-linear behaviour of the material. Thus, both Maxwell model and the Peleg model were used in this study to fit the viscoelastic behaviour of dough with PFPF in a more accurate manner. Constants k1 and k2 in indicate the initial rate of relaxation and the extent of relaxation, respectively. k1 was the highest in both raw and cooked W dough and raw BM dough. In most studies, k1 value indicates more solid-like material with a higher resistance to compression. However, elastic-like properties of wheat dough from the TPA test and values of Maxwell elements showed the lowest values. Thus, the k1 value of Peleg model should be interpreted carefully due to the inherent nature of this parameter (Singh, Rockall, Martin, Chung, & Lookhart, Citation2006). This result might be due to different amount of gluten complexes and consistency of the dough.
The k2 value indicates the extent of relaxation and degree of solidity, with values equal to one for a pure liquid and to infinity for a perfect elastic body, the k2 value may represent the influence of available or excess water on the viscoelastic properties of dough in this study (Peleg & Normand, Citation1983). Higher k2 values were observed with higher competition for water in dough. The BM dough with a high degree of damaged starch showed the largest k2 values for both raw and cooked doughs.
Effect of particle size on the microstructure of PFPF noodle dough
The cross-section microstructures of control dough and dough with various sizes of PFPF were investigated (). Before cooking, the wheat dough showed a continuous structure of elliptical or circular shape of starches surrounded by a gluten network (GM in ). In the mixing process of wheat dough, gluten initially exists in a lump shape; then, it becomes a linear structure upon hydration and eventually forms proteins cross-links (Auger, Morel, Lefebvre, Dewilde, & Redl, Citation2008). PFPF particles including potato cellular structure and potato starch (PCS and PS in ) were clearly observed in the continuous phase composed of wheat gluten cross-linked networks and potato cellular structure as shown in (e,g,i). Regarding the dough containing BM flour, the microstructure demonstrates that the cellular structure of potato had nearly disappeared and the potato starch was apart and broken into small damaged pieces. In addition, less developed gluten matrix can be observed by decreasing available water in BM dough ().
Figure 3. Microstructure of the wheat and PFPF doughs: (a) raw control dough; (b) cooked control dough; (c) raw BM dough; (d) cooked BM dough; (e) raw P1 dough; (f) cooked P1 dough; (g) raw P2 dough; (h) cooked P2 dough; (i) raw P3 dough; (j) cooked P3 dough. WS: wheat starch; GM: gluten matrix; PS: potato starch; DPS: damaged potato starch; PCS: potato cellular structure (magnification, 800× for a–d; 300× for e–j).
Figura 3. Microestructura de las masas de trigo y PFPF. (a) masa de control cruda; (b) masa de control cocida; (c) masa cruda procesada mediante BM; (d) masa cocida procesada mediante BM; (e) masa cruda P1; (f) masa cocida P1; (g) masa cruda P2; (h) masa cocida P2; (i) masa cruda P3; (j) masa cocida P3. WS, almidón de trigo; GM, matriz de gluten; PS, almidón de papa; DPS, almidón de papa dañado; PCS, estructura celular de la papa. (Ampliación, 800× para a, b, c y d; 300× para e, f, g, h, i y j.)
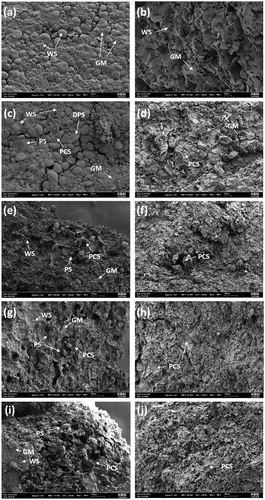
The microstructure of cooked wheat dough clearly showed that swollen wheat starch granules were associated with gelatinization (), whereas less swollen wheat starch granules observed with the cooked dough containing BM (). The dough samples with PFPF after cooking appeared to have a cavitary matrix and the potato starch granules were agglomerated and the dough formed a cytoplasmic starch matrix without the granules when gelatinized (f, h, and j in ). In the cooked potato, swollen, hydrated, and gelatinized starch granules are fused together and occupy the entire volume of the cell (Bordoloi, Kaur, & Singh, Citation2012). Gelatinization occurring in undamaged potato starch of large particle size PFPF can build continuous and compact structure in cooked noodle dough, consequently increasing the rigidity in texture characteristics.
Conclusion
The grinding process of PFP and the size of PFPF were controlled before preparing the dough by conducting grinding kinetics of PFP. The effects of particle size and damaged starch content of PFPF on rheological and microstructural characteristics of noodle dough were investigated. The mathematical model for grinding kinetics showed a practical application for controlling and predicting the various particle sizes of PFPF which highly influences the dough properties. The addition of PFPF, small particle size, and high damaged starch content resulted the higher competition in absorption of water in dough with increasing difficulty for sheeting or flattening in noodle processing. Maxwell model and Peleg model were suitable for describing and classifying characteristics of noodle dough after the addition of PFPF. It is desirable that the noodle dough with relatively large particle sized (larger than starch ingredient size) PFPFs were well suited for noodle production due to the suitable flexibility for flattening and improved shape retention with lower relaxation times. It is suggested that the characteristics of dough depend on particle components and modification of starch by milling conditions must be considered when adding substitutive ingredients for noodle processing.
Acknowledgments
This research was financially supported by the Ministry of Trade, Industry and Energy (MOTIE) and Korea Institute for Advancement of Technology (KIAT) through the Promoting Regional specialized Industry: [Grant Number R0004761-1].
Disclosure statement
No potential conflict of interest was reported by the authors.
Additional information
Funding
References
- Auger, F., Morel, M., Lefebvre, J., Dewilde, M., & Redl, A. (2008). A parametric and microstructural study of the formation of gluten network in mixed flour–water batter. Journal of Cereal Science, 48(2), 349–358. doi:10.1016/j.jcs.2007.10.006
- Belton, P. S. (1999). Mini review: On the elasticity of wheat gluten. Journal of Cereal Science, 29(2), 103–107. doi:10.1006/jcrs.1998.0227
- Bhattacharya, S. (2010). Stress relaxation behaviour of moth bean flour dough: Product characteristics and suitability of model. Journal of Food Engineering, 97(4), 539–546. doi:10.1016/j.jfoodeng.2009.11.014
- Bordoloi, A., Kaur, L., & Singh, J. (2012). Parenchyma cell microstructure and textural characteristics of raw and cooked potatoes. Food Chemistry, 133(4), 1092–1100. doi:10.1016/j.foodchem.2011.11.044
- Chen, J. S., Fei, M. J., Shi, C. L., Tian, J. C., Sun, C. L., Zhang, H., … Dong, H. X. (2011). Effect of particle size and addition level of wheat bran on quality of dry white Chinese noodles. Journal of Cereal Science, 53(2), 217–224. doi:10.1016/j.jcs.2010.12.005
- Faridi, H., & Faubion, J. M. (2012). Dough rheology and baked product texture. Berlin: Springer.
- Hatcher, D. W., Edwards, N. M., & Dexter, J. E. (2008). Effects of particle size and starch damage of flour and alkaline reagent on yellow alkaline noodle characteristics. Cereal Chemistry, 85(3), 425–432. doi:10.1094/CCHEM-85-3-0425
- Jane, J., Kasemsuwan, T., Leas, S., Zobel, H., & Robyt, J. F. (1994). Anthology of starch granule morphology by scanning electron microscopy. Starch-Stärke, 46(4), 121–129. doi:10.1002/(ISSN)1521-379X
- Lachman, J., & Hamouz, K. (2005). Red and purple coloured potatoes as a significant antioxidant source in human nutrition-a review. Plant, Soil and Environment, 51(11), 477–482.
- Lee, Y. J., Lee, M. G., & Yoon, W. B. (2013). Effect of seed moisture content on the grinding kinetics, yield and quality of soybean oil. Journal of Food Engineering, 119(4), 758–764. doi:10.1016/j.jfoodeng.2013.06.034
- Li, P., Huang, C., Yang, M., & Wang, C. (2012). Textural and sensory properties of salted noodles containing purple yam flour. Food Research International, 47(2), 223–228. doi:10.1016/j.foodres.2011.06.035
- Liu, T. Y., Ma, Y., Yu, S. F., Shi, J., & Xue, S. (2011). The effect of ball milling treatment on structure and porosity of maize starch granule. Innovative Food Science & Emerging Technologies, 12(4), 586–593. doi:10.1016/j.ifset.2011.06.009
- Moon, J. H., Pan, C., & Yoon, W. B. (2015). Drying characteristics and thermal degradation kinetics of hardness, anthocyanin content and colour in purple- and red-fleshed potato (Solanum tuberosum L.) during hot air drying. International Journal of Food Science & Technology, 50(5), 1255–1267. doi:10.1111/ijfs.2015.50.issue-5
- Peleg, M. (1979). Characterization of the stress relaxation curves of solid foods. Journal of Food Science, 44(1), 277–281. doi:10.1111/jfds.1979.44.issue-1
- Peleg, M., & Normand, M. D. (1983). Comparison of two methods for stress relaxation data presentation of solid foods. Rheologica Acta, 22(1), 108–113. doi:10.1007/BF01679835
- Rytel, E., Tajner-Czopek, A., Kita, A., Aniołowska, M., Kucharska, A. Z., Sokół-Łętowska, A., & Hamouz, K. (2014). Content of polyphenols in coloured and yellow fleshed potatoes during dices processing. Food Chemistry, 161, 224–229. doi:10.1016/j.foodchem.2014.04.002
- Shiau, S., Wu, T., & Liu, Y. (2012). Effect of the amount and particle size of wheat fiber on textural and rheological properties of raw, dried and cooked noodles. Journal of Food Quality, 35(3), 207–216. doi:10.1111/jfq.2012.35.issue-3
- Šimková, D., Lachman, J., Hamouz, K., & Vokál, B. (2013). Effect of cultivar, location and year on total starch, amylose, phosphorus content and starch grain size of high starch potato cultivars for food and industrial processing. Food Chemistry, 141(4), 3872–3880. doi:10.1016/j.foodchem.2013.06.080
- Singh, H., Rockall, A., Martin, C. R., Chung, O. K., & Lookhart, G. L. (2006). The analysis of stress relaxation data of some viscoelastic foods using a texture analyzer. Journal of Texture Studies, 37(4), 383–392. doi:10.1111/jts.2006.37.issue-4
- Yadav, B. S., Yadav, R. B., Kumari, M., & Khatkar, B. S. (2014). Studies on suitability of wheat flour blends with sweet potato, colocasia and water chestnut flours for noodle making. LWT – Food Science and Technology, 57(1), 352–358. doi:10.1016/j.lwt.2013.12.042