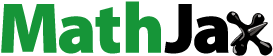
ABSTRACT
The goal of the present study was to determine, through full 23 factorial design, the optimum process conditions to develop a biodegradable film, made from gelatin with buriti oil (BO) as additive, characterizing their physical, mechanical, and antioxidant aspects. The conditions for the optimization were 2.3% of fish gelatin, 30% of BO, and 13.18% of plasticizer. The films produced under this condition presented high antioxidant activity (24.77 µmol de TE/g), mechanical resistance (8.04 MPa), and satisfactory water vapor permeability (WVP; 0.38 × 10−10 g.m. m−2. s−1. Pa−1). The film presented low solubility in water, low humidity, and low water activity, contributing to its integrity, without identifiable flaws. The increase in the concentration of BO provided higher antioxidant properties to the films and the reduction in gelatin and glycerol concentrations provided lower WVP, increasing the traction resistance.
RESUMEN
A través de un diseño factorial completo de 23, el presente estudio se propuso determinar las condiciones óptimas para desarrollar una película biodegradable, hecha de gelatina con aceite de burití añadido, y caracterizar sus aspectos físicos, mecánicos y antioxidantes. Se pudo constatar que las condiciones óptimas para este proceso incluyen el uso de 2.3% de gelatina de pescado, 30% de aceite de burití y 13.18% de plastificante. Las películas producidas bajo estas condiciones presentan elevada actividad antioxidante (24.77 µmol de TE/g), resistencia mecánica (8.04 MPa) y WVP satisfactoria (0.38 × 10−10 g.m. m−2. s−1. Pa−1). Además, la película mostró tener baja solubilidad en el agua, baja humedad y baja actividad en agua, lo cual contribuye a mantener su integridad, sin que se presenten defectos identificables. Al elevarse la concentración de aceite de burití, las películas acusaron propiedades antioxidantes más elevadas. Una reducción de las concentraciones de gelatina y glicerol produjo una permeabilidad más baja al vapor de agua, aumentando la resistencia a la tracción.
Introduction
Over the last years, the consumers and food industries have been searching for the packing systems based on biodegradable materials in order to reduce the environmental impacts caused by petroleum-based plastic materials (Güçbilmez, Yemenicioğlu, & Arslanoğlu, Citation2007; Wu et al., Citation2014).
The fish industry produces a great amount of wasted material, such as fish heads and skin that could be used in food production because of their nutritional value, thus contributing to minimize the environmental impacts (Simões, Mori, Faria, De Castro, & Mendes, Citation2011). For instance, chitosan and the gelatin can be produced from these wastes (Karim & Bhat, Citation2009; Ocak, Citation2012).
The gelatin is a polypeptide obtained through thermal hydrolysis of collagen, which shows the capacity of forming thermoreversible physical gels. The interest in using gelatin for the elaboration of packing has increased due to its excellent film formation, abundancy, non-toxicity, biocompatibility, and biodegradability (Ahmad, Nirmal, & Chuprom, Citation2015; Alfaro, Fonseca, Balbinot, Machado, & Prentice, Citation2013; Gómez-Guillén, Giménez, López-Caballero, & Montero, Citation2011; Tongnuanchan, Benjakul, & Prodpran, Citation2014).
Biodegradable films can be prepared from natural polymers as proteins, lipids, and polysaccharides, or a combination of these compounds (Limpan, Prodpran, Benjakul, & Prasarpran, Citation2010). Moreover, oil can be added to the composition of biodegradable films to improve their properties (Tongnuanchan et al., Citation2014).
In this sense, the oil from buriti palm has drawn worldwide interest due to its chemical and pharmacological features. Indeed, the buriti oil (BO) is rich in carotenoids, fatty acid, and vitamin E (α-tocopherol), with high antioxidant activity and stability against oxidant agents (Batista et al., Citation2012).
The addition of BO is an alternative to enhance properties in biodegradable films such as water vapor barrier, thus increasing the resistance and the life span of final products (Burt, Citation2004; De Silva, Lopes, Da Silva, & Yoshida, Citation2016; Nunez-Flores et al., Citation2012).
The aim of this study was to determine the optimum conditions to develop a gelatin biodegradable film from fish with addition of BO. The films were characterized in relation to their antioxidant activity, mechanical property, WVP, viscosity of the filmogenic solution (FS), thickness, water solubility, color parameters, and microstructure.
Material and methods
Materials
Fish skin from acoupa weakfish (Cynoscion acoupa), used for the gelatin production, was obtained in the fish market Ver-o-Peso (Belém, Brazil). The pure gum Arabic powder (Dinâmica Química Contemporânea Ltda, Brazil) was used as surfactant. The BO was donated by Beraca Company (Beraca, Belém, Brazil) and glycerol (Isofar, Glycerin PA with 99.5% of purity) was used as plasticizer (PL).
Gelatin extraction from acoupa weakfish (Cynoscion acoupa)
The gelatin extraction from acoupa weakfish skin was performed according to the methodology described by Montero and Gomez-Guillen (Citation2000) with adaptations in the concentration of the solution, time, and temperature of immersion, obtained through preliminary assays. The skins were cut in 4 cm × 4 cm, washed in running tap water and immersed in sodium chloride (Isofar, Brazil) 0.6 M 1:5 (weight/volume) for 15 min, under 85 rpm agitation at 25°C in incubator Shaker (Lucadema, Luca-223, Brazil). Afterwards, the material was submerged in sodium hydroxide solution (Vetec, Brazil) at 0.3 M, 1:5 (weight/volume) for 15 min, under continuous agitation and then washed in running tap water. Afterwards, it was immersed in 0.02 M acetic acid solution (Audaz, Brazil), 1:5 (weight/volume) for 1 h under agitation and washed in tap water. The extraction phase was performed with distilled water in water bath (Tecnal, Te-057, Brazil) for 12 h at 50°C, keeping a ration of 1:5 (weight/volume). After extraction, the filtered material was put on inox trays, frozen in ultra-freezer (Coldlab, Cl, Brazil), and lyophilized at −50°C/36 h (Liotop—L101, Brazil). Finally, the obtained gelatin was packed in vacumm (Fastvac Wrapping Machine, F200, Brazil) and stored at −26°C in ultra-freezer until the confection of the films.
Preparation of the biodegradable film
This procedure was carried out according to Tongnuanchan et al. (Citation2014) with few modifications. Different concentrations of fish gelatin (FG), BO, and PL (glycerol) were mixed with distilled water (w/v) per suspension, following to the full 23 factorial design presented in , reaching a concentration of 30% of surfactant (Arabic gum) in relation to the oil. The final solution was homogenized at 15.000 rpm for 5 min in Turratec homogenizer (Tecnal, TE-102, Brazil) to obtain the FSs. According to the casting method, 120 mL of the FS were put in silicon stand (22 cm diameter × 3 cm high) and submitted to drying in a drier (Quimis, Q315M, Brazil) at 30°C for 20 h. After drying, the films were packed in polyethylene bags at room temperature. Another biodegradable film was produced in the same way without the addition of BO to be used as negative control.
Table 1. Level definition of the studied variables in the films obtainments.
Tabla 1. Definición del nivel de las variables estudiadas en las películas obtenidas.
Full 23 factorial design to establish the variables of film processing
To stablish the best combination of FG, BO, and PL in the obtained film, a full 23 factorial design was used. It comprised linear assays −1 and +1 and assays with axial points –α and +α, defined in −1.68 and 1.68, respectively, plus 3 repetitions in the central point (). The independent variables were the concentrations of FG, BO, and PL; the dependent variables were the antioxidant activity, WVP, and traction resistance (TR).
Determination of biodegradable film properties
The antioxidant activity was determined through UV-Visible Spectrophotometry, utilizing the ABTS radical [2,2ʹ-azino-bis (3-ethylbenzothiazoline-sulfonic-6-sulfonic acid), according to the methodologies described by Re et al. (Citation1999) and Brand-Williams, Cuvelier, and Berset (Citation1995).
The WVP was determined by the use of modified ASTM D882-95 method, described by Arfat, Benjakul, Prodpran, and Osako (Citation2014). The film sample was sealed with silicon patch in a glass container of 4.5 cm × 7.0 cm (diameter × height), containing 10 g of silica gel at 0% Relative humidity (RH) and water vapor pression (WV-Pa) at 30°C equal to zero. The containers were put in desiccators containing distilled water at 30°C at 99% UR and 4244.9 Pa of vapor pressure at 30°C. The containers were weighed each 1 h for a 10-hperiod. The WVP was calculated according to Equation 1
where WVP: water vapor permeability (g.m−1.s−1.Pa−1.); W: weight gain by the desiccator (g); X: film thickness (m); A: superficial area of exposed film (m2) t: incubation period (seconds); ∆P: partial difference of pressure (Pa). Three samples were used in WVP tests.
The TR of the films was determined by employing ASTM D882-91 (ASTM, Citation1996) methodology, described by Limpan et al. (Citation2010), using texturometer (Brookfield, QTS, Midleboro, USA) and it was performed at room temperature. The initial separation of the clamps and the speed of the probe were 50 mm and 1 mm.s−1, respectively. The samples were cut into pieces of 100 mm × 25 mm (length × width) and calculated as shown in Equation 2
where TR: traction resistance (MPa); Fm: maximum force in the moment of film rupture (N); A: area of the film transversal section (m2).
The viscosity of the FS was performed through the determination of flow curves (shear strength × cut-rate) in viscometer (Haake, VT 500, Germany) attached to configuration system of coaxial cylinders cup SV and cylinders SV1. The rheological assays were performed at 28°C, with shearing rate varying between 0 and 300 s−1 in a period of 120 s.
The film thickness was measured by the use of a digital micrometer (Insize, IP54, Iran) with resolution of 0.001 mm. In each film, eight random regions with edge deviation of 60 mm were selected.
In order to evaluate the solubility, the films were trimmed in discs of 2 cm diameter and put in a drier at 105°C for 24 h and weighed. Afterwards, the dry films were immersed in containers with 50 mL of water. This system was shaken in refrigerated incubator Shaker (Lucadema, Luca-223, Brazil) at 150 rpm for 24 h at 25°C. After this period, the samples were filtered and the retained material was dried (105°C for 24 h) to determine the amount of non-dissolved material in water (Gontard, Duchez, Cuq, & Guilbert, Citation1994).
The color of the obtained film was determined using a portable colorimeter (MINOLTA, CR 310, USA), which generated parameters such as L* (luminosity), a* (red intensity), b* (yellow intensity), C* (chroma value), and h° (tonality angle).
The microstructure of the superior surface and the transversal section of the film were determined by analysis in Sweep Electron Microscope (Leo-Zeiss, 1430, Germany).
Statistical analysis
The results were statistically analyzed in the software STATISTICA version 7.0 (STATSOFT Inc., Citation2004) based on the antioxidant, WVP, and TR analyses to provide the hierarchical effects of each variable, variance analysis, and response surface to the complete 23 factorial planning as well as the desirability function to estimate the optimum conditions to the obtained film. Tukey’s test was performed to verify putative significant differences with 95% confidence (p < 0.05) between the optimized film and the control test (free of BO).
Results and discussion
Full 23 factorial design
The codified matrix and its respective levels among the experimental values of antioxidant activity, WVP, and TR are presented in .
Table 2. Experimental design and the responses to antioxidant activity (ABTS), WVP, and TR obtained under different conditions of the full factorial design 23.
Tabla 2. Diseño experimental y respuestas a la actividad antioxidante (ABTS), WVP y TR obtenidas bajo distintas condiciones del diseño factorial completo de 23.
A total of 17 factorial assays were carried out (), where eight of them combined within ± 1 level, three in the central point with variables at 0.0 level, and six axial assays within ± α. The results from the different combinations of FG, BO, and PL are shown in , revealing a wide variation in the responses, mainly related to the antioxidant activity. The antioxidant activity values ranged from 23.28 to 4.50 µmol of TE/g, while the values for WVP and TR varied from 2.49 to 0.52 × 10−10 g.m−1.s−1.Pa−1 and 5.78 to 0.64 MPa, respectively. When low concentrations of FG, BO, and PL were used (e.g. assay 1), the film presented the best results, characterized mainly by low WVP and high resistance, features that are interesting for industrial processes. Furthermore, we showed that high-quality film in relation to both physical and mechanical properties can be obtained using low amounts of each tested component.
Estimative of the variable, significance, and variance analysis of responses in the full 23 factorial design
For the antioxidant activity (), the linear oil and the quadratic PL presented significant positive effect, indicating that the increase in the concentration of these components in the film formation results in higher antioxidant activity. The increased antioxidant activity associated with the concentration of BO is due to the presence of carotenoids, phenolic compounds, and vitamin E under α-tocopherol form in the oil of this palm tree (Batista et al., Citation2012; Koolen, Da Silva, Gozzo, De Souza, & De Souza, Citation2013).
Table 3. Estimated effect, error, level of statistical significance for pure error, regression, lack of adjustment, and R2 for antioxidant activity, WVP, and TR.
Tabla 3. Efecto estimado, error, nivel de significación estadística para el error puro, falta de ajuste y R2 para la actividad antioxidante, WVP y TR.
The concentration of linear PL had the greatest effect over the TR, inasmuch as the higher the concentration of PL the lower the TR of film products, which become less resistant and more elastic. Indeed, the role of PL is to provide flexibility to the film (Rodríguez-Núñez, Madera-Santana, Sánchez-Machado, López-Cervantes, & Soto Valdez, Citation2014).
After eliminating the parameters with nonsignificant effects, the significance of regression and lack of adjustment were verified within 95% of confidence level.
The variance analysis (ANOVA) showed that the adjusted models for all the tested responses (antioxidant activity, WVP, and TR) presented significant regression (Fcal>Ftab), nonsignificant, and predictive lack of adjustment. According to Box and Wetz (Citation1973), a statistically significant regression to be used in predictive models should present F value, at least, four to five times higher than the tabled F value, as observed in this study.
In addition, the R2 value for the antioxidant activity, WVP, and TR showed that the model properly described the behavior of the process, explaining about 90–93% of the variation in the experimental data.
In , the response surfaces to antioxidant activity, WVP, and TR are shown, considering the concentrations of gelatin, BO, and PL. In (a), it is verified that increased oil concentration from the central point leads to higher levels of antioxidant activity, corroborating the presence of antioxidant compounds in the oil. As pointed out by Pastor et al. (Citation2011) and Bonilla, Talón, Atarés, Vargas, and Chiralt (Citation2013), films composed of antioxidant material might expand the life span of fruits by conserving them for longer periods and reducing the undesirable effects on their nutritional properties.
Figure 1. Graphics of surface response for antioxidant activity, water vapor permeability (WVP), and traction resistance (TR), related to the concentrations of fish gelatin (FG), buriti oil (BO), and plasticizer (PL) for the film obtainment.
Figura 1. Gráficas de la respuesta en superficie para la actividad antioxidante, la permeabilidad de vapor de agua (WVP) y la resistencia a la tracción, relacionadas con las concentraciones de gelatina de pescado, aceite de burití y plastificador utilizadas para obtener las películas.
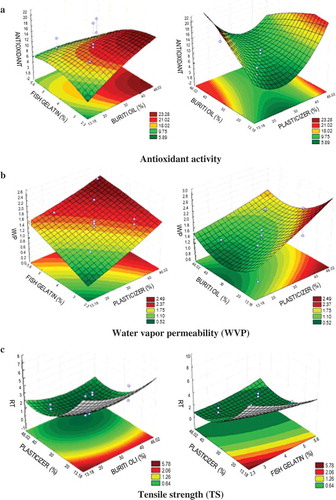
As for the WVP, (b) shows that the WVP increases along with high concentrations of FG and PL. This behavior can be explained by the hidrophilic properties of the gelatin fish due to its high content of polar aminoacids, thus absorbing the environmental water (Krochta, Citation2002). The same behavior was observed in relation to glycerol, a hidrophilic compound that is located between the peptides when incorporated to the protein net, thereby reducing the proximity between the protein chains. Consequently, this facilitates the molecular mobility and intensifies the water affinity, which increases the WVP (Monterrey-Quintero and Sobral, Citation2000). Therefore, the area with lower WVP values is related to the lower concentrations of FG and PL, whereas the concentrations of BO ranged from 30% to 40%.
(c) shows that lower values of gelatin and glycerol provide an increase in the studied response, since they produce more resistant film. This fact is related to the hydrophilic nature of both FG and glycerol inasmuch as high values of these compounds provide higher water absorption and elasticity to the film and, therefore, less resistance. In relation to the BO, we observed high TR levels without changes in the mechanical properties of the film in all studied ranges (from −1 to +1).
Tongnuanchan et al. (Citation2014) elaborated FG-based films added with essential oils and observed that the oils decreased the TR. This fact was not observed in the present study since, independently on the oil concentration, high TR values were obtained. According to Bakkali, Averbeck, Averbeck, and Idaomar (Citation2008) and Hoque, Benjakul, and Prodpran (Citation2011), this differential behavior is related to the oil composition which interacts distinctively with the proteins, thus modifying the mechanical properties of film products.
Optimization of the biodegradable film by desirability
The estimative of the optimum conditions for the biodegradable film was determined according to the desirability function. The desirability profiles are presented in .
Figure 2. Desirability profiles for concentrations of FG, BO, and PL in the obtainment of the biodegradable film.
Figura 2. Perfiles de deseabilidad para concentraciones de gelatina de pescado, aceite de burití y plastificantes utilizadas para obtener películas biodegradables.
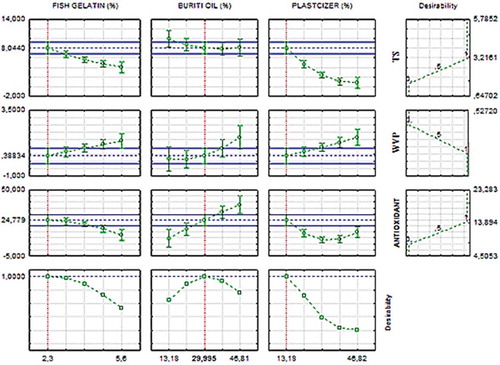
In , the first nine superior graphics present the individual desirability profile and the predicted values for the TR, WVP, and antioxidant responses, where the blue dashed line represents the optimum values for each response. The last three graphics present the optimum concentrations of FG, BO, and PL, indicated by the red dashed lines.
According to the desirability function, films with maximum values of global desirability (100%) should present concentrations of gelatin fish, BO, and PL equal to −1.68, 30%, and −1.68, respectively, according to the full 23 factorial design. Therefore, the concentrations defined as optimum to obtain biodegradable films were 2.3% of FG, 30% of BO, and 13.18% of PL. The film elaborated as abovementioned will present mean values of 8.04 MPa for TR, 0.38 × 10−10 g.m−1.s−1.Pa−1 for WVP, and 24.77 µmol of TE/g for antioxidant activity. Under these conditions, the film will putatively present low WVP, high TR, and high antioxidant activity.
The results obtained in the desirability function are compatible with the experimental data based on the surface response, suggesting the utilization of low concentrations of FG and PL and low values of BO, close to the central point.
Rheological properties of the FS
The outflow curves, referent to the FS of the FG with BO, are shown in .
Figure 3. (a) Outflow curve referent to the filmogenic solution (emulsions), (b) viscosity curve versus deformation rate.
Figura 3. (a) Curva de salida referida a la solución filmogénica (emulsiones), (b) Curva de viscosidad versus tasa de deformación.
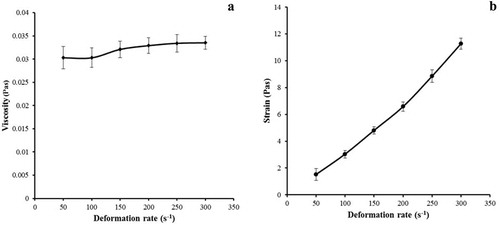
The viscosity found in the FS in the present study was considered low ((a)), which is recommended to the biodegradable film production, once it facilitates the dissolution, handling, and transportation, besides interfering in the thickness, molding, and drying. Peressini, Bravin, Lapasin, Rizzotti, and Sensidoni (Citation2003) reported that low viscosity values provide uniformity to the film, influencing in the appearance and facilitating the flow of the film over the contact surface. Cuq, Aymard, Cuq, and Guilbert (Citation1995) suggest the use of FSs with viscosity under 0.7 Pas.
As observed in (b), the FS presented a non-Newtonian behavior, since the viscosity increases along with the shear rate. The behavior of non-Newtonian fluids can be explained by changes in the structures of molecule chains with increased speed gradient (Nascimento, Calado, & Carvalho, Citation2012). In a liquid medium, the viscosity is a function of intermolecular forces that limit the molecular movement (Nurul, Mohd. Azemi, & Manan, Citation1999).
Characterization of the optimized biodegradable and control films
The thickness value in the present study was 0.095 mm, being higher than the results reported by Tongnuanchan et al. (Citation2014) in fish-gelatin fish but closer to those produced with essential oils. According to this author, such increased thickness after oil addition is related to the penetration of oil into the film net, restraining the interaction of gelatin chains, thus hindering the formation of compact structures, leading to higher thickness vales. Similarly, the control film (oil-free) presented lower thickness, with significant differences in relation to the optimized film (p ≤ 0.05).
The solubility of the optimized film was close to the control test (oil-free film), without significant differences (p ≥ 0.05). However, the solubility was lower than that reported in fish-gelatin film (94.9%) by Ahmad et al. (Citation2015) and cattle-gelatin film (100%) by Pereda, Ponce, Marcovich, Ruseckaite, and Martucci (Citation2011).
The high solubility of gelatin films is associated with the presence of hydrophilic aminoacids that rehydrate in contact with water, thus increasing their solubility levels (Hoque et al., Citation2011; Yao, Ding, Shao, Peng, & Huang, Citation2017). In the present study, the comparative lower values are related to the addition of BO as well as to the low concentration of gelatin, which altogether contribute to the hydrophobicity of the optimized film, thus decreasing its dissolution. Moreover, low humidity and water activity values increase the structural integrity of components, providing fewer hydrophilic areas.
Humidity and water activity are key features in food industries once the amount of water determines the speed and amount of chemical, enzymatic, and microbial reactions, as well as the applicability of film products. In this study, the biodegradable film with FG and BO presented 18.92% of humidity and water activity equal to 0.45, while these values were, respectively, equal to 5.31% and 0.39 for the control film (). Gutierrez et al. (Citation2015) reported values from 32% to 52% for humidity and 0.473 to 0.524 for water activity in film made with starch.
Table 4. Physical–chemical characterization and solubility of the optimized biodegradable film.
Tabla 4. Caracterización fisicoquímica y solubilidad de la película biodegradable optimizada.
The low values of humidity and water activity in the present study indicate effective homogeneity of samples and proper interaction between the PL and the gelatin, resulting in less available areas for water retention. Nonetheless, the addition of BO had no effects on humidity or water activity, thus showing satisfactory properties for the control film. This result differs from those reported in starch films once the reduced interaction between glycerol and amylopectin generates free hydroxyls that favor water absorption, increasing the hydrophilic nature and the humidity of films (Flores, Famá, Rojas, Goyanes, & Gerschenson, Citation2007; Hu, Chen, & Gao, Citation2009; Garcia et al., Citation2009). Moreover, films with high humidity hinder the components interaction, thus decreasing the degradation temperature, affecting their thermal stability and commercial life span (González et al., Citation2017; Gutierrez et al., Citation2015).
The L* values indicate high luminosity, demonstrating the control film is significantly clearer than that with addition of BO. This result is related to the presence of high levels of carotenoids, particularly b-carotene in the BO. Based on C* values, the film made with FG and BO is bright yellow, characterized by values much higher than those observed in the control film, proving both films differ in coloration. The angle of 92.51 for the FG film with BO confirms the yellow coloration while the control film is characterized by a more transparent coloration (Little, Citation1975). On the other hand, the appearance of both types of film was similar, being free of wrinkles or other visible defects.
The BO accounted for the yellow coloration of fish-gelatin film, as revealed by the b* parameter (76.08) in and confirmed in . When the present results were compared to those reported by Silva et al. (Citation2016) in chitosan film with addition of BO (b* parameter of 40.99), the film obtained in this study presented a more conspicuous yellow color. Furthermore, the b* values in the control film revealed it is significantly clearer (p ≤ 0.05).
Figure 4. (a) Controle film and (b) biodegradable film, optmized by the desirability, obtained with 2,3% of FG, 30% of BO, and 13,18 of PL.
Figura 4. (a) película de control y (b) película biodegradable, optimizada por la deseabilidad, obtenida mediante el uso de 2.3% de gelatina de pescado, 30% de aceite de burití y 13.18% de plastificante.
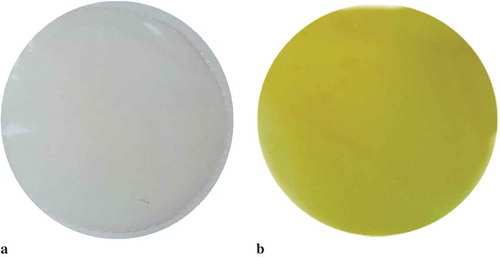
The results of the electron microscopy for the surface and cross section of the film optimized by the desirability are presented in (a, b), respectively.
Figure 5. Microphotographs (a) of the surface of the buriti film and (b) surface of the biodegradable film of FG without addition of control oil, (c) cross section of the buriti film, (d) cross section of the FG film without addition of oil control.
Figura 5. Microfotografías de la (a) superficie de la película de burití y la (b) superficie de la película biodegradable de la gelatina de pescado sin adicionar el aceite de control; (c) sección transversal de la película de burití, y (d) sección transversal de la película de gelatina de pescado sin adicionar el aceite de control.
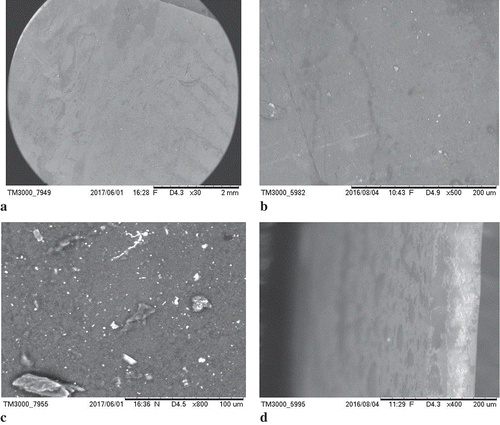
The surface image of films ((a, b)) shows a relatively homogeneous structure characterized by the cohesive and continuous aggregation of gelatin, without differences between the optimized and the oil-free films. The pelicles were smooth, falt, with a few pores and cracks. The white spots represent incomplete solubilization of the Arabic gum. The lack of full solubilization in fish-gelatin fish was also reported by Tongnuanchan et al. (Citation2014).
The transversal film cuts ((c, d)) show the internal film products structure, revealing several wrinkles in optimized film with BO when compared to the oil-free film. This result indicates that the addition of BO interferes in the film structure putatively associated with the presence of gelatin and oil drops. The low amount of FG used in the formulation of the optimized film and the presence of oil droplets should constrain the water transferability, thus favoring the lower water permeability of the film. On the other hand, structural fissures and irregularities might jeopardize the structural film products integrity, disturbing their functional properties (Souza, Sobral, & Menegalli, Citation2004). Nonetheless, the biodegradable film obtained in the present study presented excellent physical and mechanical properties in spite of the presence of a few fissures.
Conclusions
The FG derived from wastes after filleting of acoupa weakfish (Cynoscion acoupa) and the BO are alternatives for the development of biodegradable films, whose properties can be modified by controlling the concentrations of the used compounds. The gelatin, the oil, and the PL significantly interfere in the physical, mechanical, and antioxidant properties of the film products. These compounds allow the production of resistant films with low WVP, low solubility in water, and appealing appearance. The FG film added with BO has industrial potential in the development of biodegradable packing with natural antioxidant activity, which helps to extend the life span and preservation of lipid-rich food, such as margarine, olive oil, and fish, by avoiding the oxidation of these products.
Acknowledgments
We gratefully acknowledge the help and assistance provided by the Dean of Research and Graduate Studies of the Federal University of the State of Pará, Pro-Rector of Research and Graduate Studies (PROPESP) of Federal University of Pará, and the Coordination for the Improvement of Higher Education Personnel (CAPES, Brazil).
Disclosure statement
No potential conflict of interest was reported by the authors.
Additional information
Funding
References
- Ahmad, M., Nirmal, N. P., & Chuprom, J. (2015). Blend film based on fish gelatine/curdlan for packaging applications: Spectral, microstructural and thermal characteristics. RSC Advances, 5(120), 99044–99057.
- Alfaro, A. D. T., Fonseca, G. G., Balbinot, E., Machado, A., & Prentice, C. (2013). Physical and chemical properties of wami tilapia skin gelatin. Food Science and Technology, Campinas, 33(3), 592–595. https://doi.org/10.1590/S0101-20612013005000069
- American Society for Testing and Materials (ASTM). (1996). Standard test methods for tensile properties of thin plastic sheeting. Philadelphia Annual Book of ASTM Standards. D882-91.
- Arfat, Y. A., Benjakul, S., Prodpran, T., & Osako, K. (2014). Development and characterization of blend films based on fish protein isolate and fish skin gelatin. Food Hydrocolloids, 39, 58–67. https://doi.org/10.1016/j.foodhyd.2013.12.028
- Bakkali, F., Averbeck, S., Averbeck, D., & Idaomar, M. (2008). Biological effects of essential oils – A review. Food and Chemical Toxicology, 46(2), 446–475.
- Batista, J. S., Olinda, R. G., Medeiros, V. B., Rodrigues, C. M. F., Oliveira, A. F., Paiva, E. S., & Da Medeiros, A. C. (2012). Atividade antibacteriana e cicatrizante do óleo de buriti Mauritia flexuosa L. Ciência Rural, 42(1), 136–141.
- Bonilla, J., Talón, E., Atarés, L., Vargas, M., & Chiralt, A. (2013). Effect of the incorporation of antioxidants on physicochemical and antioxidant properties of wheat starch-chitosan films. Journal of Food Engineering, Essex, 118(3), 271–278.
- Box, G. E. P., & Wetz, J. Criteria for judging adequacy of estimation by an approximate response function. University of Wisconsin Technical Report, 9, 1973.
- Brand-Williams, W., Cuvelier, M. E., & Berset, C. (1995). Use of a free radical method to evaluate antioxidant activity. LWT - Food Science and Technology, 28(1), 25–30. https://doi.org/10.1016/S0023-6438(95)80008-5
- Burt, S. (2004). Essential oils: Their antibacterial properties and potential applications in foods—A review. International Journal Of Food Microbiology, 94(3), 223–253. doi: 10.1016/j.ijfoodmicro.2004.03.022
- Cuq, B., Aymard, C., Cuq, J. L., & Guilbert, S. (1995). Edible packaging films based on fish myofibrillar proteins: Formulation and functional properties. Journal of Food Science, 60(6), 1369–1374. https://doi.org/10.1111/j.1365-2621.1995.tb04593.x
- De Silva, F. M., Lopes, P. S., Da Silva, C. F., & Yoshida, C. M. P. (2016). Active packaging material based on buriti oil - Mauritia flexuosa L.f. (Arecaceae) incorporated into chitosan films. Journal of Applied Polymer Science, 133(12), 1–9. https://doi.org/10.1002/app.43210
- Flores, S., Famá, L., Rojas, A. M., Goyanes, S., & Gerschenson, L. (2007). Physical properties of tapioca-starch edible films: Influence of filmmaking and potassium sorbate. Food Research International, 40(2), 257–265. https://doi.org/10.1016/j.foodres.2006.02.004
- García, N. L., Famá, L., Dufresne, A., Aranguren, M., & Goyanes, S. (2009). A comparison between the physico-chemical properties of tuber and cereal starches. Food Research International, 42, 976–982.
- Gómez-Guillén, M. C., Giménez, B., López-Caballero, M. E., & Montero, M. P. (2011). Functional and bioactive properties of collagen and gelatin from alternative sources: A review. Food Hydrocolloids, 25(8), 1813–1827. https://doi.org/10.1016/j.foodhyd.2011.02.007
- Gontard, N., Duchez, C., Cuq, J., & Guilbert, S. (1994). Edible composite films of wheat gluten and lipids - water-vapor permeability and other physical-properties. International Journal of Food Science and Technology, 29(1), 39–50. Retrieved from http://apps.webofknowledge.com/full_record.do?product=UA&search_mode=GeneralSearch&qid=54&SID=Z1gk1JRTrw3X1tkSpeg&page=1&doc=1
- González, K., Martin, L., González, A., Retegi, A., Eceiza, A., & Gabilondo, N. (2017). D-isosorbide and 1,3-propanediol as plasticizers for starch-based films: Characterization and aging study. Journal of Applied Polymer Science, 134(20), 1–10. https://doi.org/10.1002/app.44793
- Güçbilmez, Ç. M., Yemenicioğlu, A., & Arslanoğlu, A. (2007). Antimicrobial and antioxidant activity of edible zein films incorporated with lysozyme, albumin proteins and disodium EDTA. Food Research International, 40(1), 80–91. https://doi.org/10.1016/j.foodres.2006.08.007
- Gutiérrez, T. J., Morales, N. J., Pérez, E., Tapia, M. S., & Famá, L. (2015). Physico-chemical properties of edible films derived from native and phosphated cush-cush yam and cassava starches. Food Packaging and Shelf Life, 3, 1–8. https://doi.org/10.1016/j.fpsl.2014.09.002
- Hoque, M. S., Benjakul, S., & Prodpran, T. (2011). Effects of partial hydrolysis and plasticizer content on the properties of film from cuttlefish (Sepia pharaonis) skin gelatin. Food Hydrocolloids, 25(1), 82–90. https://doi.org/10.1016/j.foodhyd.2010.05.008
- Hu, G., Chen, J., & Gao, J. (2009). Preparation and characteristics of oxidized potato starch films. Carbohydrate Polymers, 76(2), 291–298. https://doi.org/10.1016/j.carbpol.2008.10.032
- Karim, A. A., & Bhat, R. (2009). Fish gelatin: Properties, challenges, and prospects as an alternative to mammalian gelatins. Food Hydrocolloids, 23(3), 563–576. https://doi.org/10.1016/j.foodhyd.2008.07.002
- Koolen, H. H. F., Da Silva, F. M. A., Gozzo, F. C., De Souza, A. Q. L., & De Souza, A. D. L. (2013). Antioxidant, antimicrobial activities and characterization of phenolic compounds from buriti (Mauritia flexuosa L. f.) by UPLC-ESI-MS/MS. Food Research International, 51(2), 467–473. https://doi.org/10.1016/j.foodres.2013.01.039
- Krochta, J. (2002). Proteins as raw materials for films and coatings: Definitions, current status, and opportunities. Protein-Based Films and Coatings, 1–41. https://doi.org/10.1201/9781420031980.ch1
- Limpan, N., Prodpran, T., Benjakul, S., & Prasarpran, S. (2010). Properties of biodegradable blend films based on fish myofibrillar protein and polyvinyl alcohol as influenced by blend composition and pH level. Journal of Food Engineering, 100(1), 85–92. https://doi.org/10.1016/j.jfoodeng.2010.03.031
- Little, A. C. (1975). A research note off on a tangent. Journal Of Food Science, 40(2), 410-411. doi: 10.1111/j.1365-2621.1975.tb02213.x
- Montero, P., & Gomez-Guillen, M. C. (2000). Extracting conditions for megrim (Lepidorhombus boscii) Skin collagen affect functional properties of the resulting gelatin. Journal of Food Science, 65(3), 434–438. https://doi.org/10.1111/j.1365-2621.2000.tb16022.x
- Monterrey-Quintero, E. S., & Sobral, P. J. A. (2000). Preparo e caracterização de proteínas miofibrilares de tilápia-do-nilo para elaboração de biofilmes. Pesquisa Agropecuária Brasileira, 35(1), 179–189.
- Nascimento, T. A., Calado, V., & Carvalho, C. W. P. (2012). Development and characterization of flexible film based on starch and passion fruit mesocarp flour with nanoparticles. Food Research International, 49(1), 588–595. https://doi.org/10.1016/j.foodres.2012.07.051
- Núñez-Flores, R., Giménez, B., Fernández-Martín, F., López-Caballero, M. E., Montero, M. P., & Gómez-Guillén, M. C. (2012). Role of lignosulphonate in properties of fish gelatin films. Food Hydrocolloids, 27(1), 60–71. https://doi.org/10.1016/j.foodhyd.2011.08.015
- Nurul, I. M., Mohd. Azemi, B. M. N., & Manan, D. M. A. (1999). Rheological behaviour of sago (Metroxylon sagu) starch paste. Food Chemistry, 64, 501–505. https://doi.org/10.1016/S0308-8146(98)00145-9
- Ocak, B. (2012). Complex coacervation of collagen hydrolysate extracted from leather solid wastes and chitosan for controlled release of lavender oil. Journal of Environmental Management, 100, 22–28. https://doi.org/10.1016/j.jenvman.2012.01.026
- Pastor, C., Sánchez-González, L., Marcilla, A., Chiralt, A., Cháfer, M., & González-Martínez, C. (2011). Quality and safety of table grapes coated with hydroxypropylmethylcellulose edible coatings containing propolis extract. Postharvest Biology and Technology, Amsterdam, 60(1), 64–70.
- Pereda, M., Ponce, A. G., Marcovich, N. E., Ruseckaite, R. A., & Martucci, J. F. (2011). Chitosan-gelatin composites and bi-layer films with potential antimicrobial activity. Food Hydrocolloids, 25(5), 1372–1381. https://doi.org/10.1016/j.foodhyd.2011.01.001
- Peressini, D., Bravin, B., Lapasin, R., Rizzotti, C., & Sensidoni, A. (2003). Starch-methylcellulose based edible films: Rheological properties of film-forming dispersions. Journal of Food Engineering, 59(1), 25–32. https://doi.org/10.1016/S0260-8774(02)00426-0
- Re, R., Pellegrini, N., Proteggente, A., Pannala, A., Yang, M., & Rice-Evans, C. (1999). Antioxidant activity applying an improved abts radical cation decolorization assay. Free Radical Biology and Medicine, 26(9), 1231–1237. https://doi.org/10.1016/S0891-5849(98)00315-3
- Rodríguez-Núñez, J. R., Madera-Santana, T. J., Sánchez-Machado, D. I., López-Cervantes, J., & Soto Valdez, H. (2014). Chitosan/Hydrophilic plasticizer-based films: Preparation, physicochemical and antimicrobial properties. Journal of Polymers and the Environment, 22(1), 41–51. https://doi.org/10.1007/s10924-013-0621-z
- Simões, A. S., Mori, R. Y., Faria, R., De Castro, H. F., & Mendes, A. A. (2011). Desempenho da matriz híbrida SiO2-quitosana na imobilização da lipase microbiana de. Candida Rugosa. Quimica Nova, 34(1), 33–38.
- Souza, S. M. A., Sobral, P. J. A., & Menegalli, F. C. (2004). Extração de proteínas miofibrilares de carne bovina para elaboração de filmes comestíveis. Ciência E Tecnologia De Alimentos, 24(4), 619–626.
- STATSOFT Inc. (2004). Statistica for Windows (Computer program manual), version 7.0.
- Tongnuanchan, P., Benjakul, S., & Prodpran, T. (2014). Comparative studies on properties and antioxidative activity of fish skin gelatin films incorporated with essential oils from various sources. International Aquatic Research, 6(62), 1–12. https://doi.org/10.1007/s40071-014-0062-x
- Wu, J., Ge, S., Liu, H., Wang, S., Chen, S., Wang, J., & Zhang, Q. (2014). Properties and antimicrobial activity of silver carp (Hypophthalmichthys molitrix) skin gelatin-chitosan films incorporated with oregano essential oil for fish preservation. Food Packaging and Shelf Life, 2(1), 7–16. https://doi.org/10.1016/j.fpsl.2014.04.004
- Yao, Y., Ding, D., Shao, H., Peng, Q., & Huang, Y. (2017). Antibacterial activity and physical properties of fish gelatin-chitosan edible films supplemented with D-Limonene. International Journal of Polymer Science, 2017, 1–9. https://doi.org/10.1155/2017/1837171