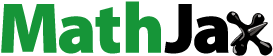
ABSTRACT
The compositions and antioxidant capacity were investigated about the by-products (blanching water concentrate (BWC) and debitterizing water concentrate DWC) produced during the industrial processing of apricot kernels, to evaluate the by-products’ potential utilization. The results indicate that the by-products contain a great number of compounds like amygdalin, protein, phenolics, and flavonoids. Among them, the amygdalin has the highest content both in BWC and DWC, which is 33.48 ± 0.04 and 23.89 ± 0.03 (g/100 g dry base weight), respectively. Furthermore, the by-products exert good antioxidant functions including the higher reducing power and radical-scavenging capacity on the 2,2-Diphenyl-1-Picrylhydrazyl (DPPH) and hydroxyl radicals, which is mainly attributed to the phenolic compounds, not the highest content of amygdalin by the principal component analysis and verification test of DPPH radical-scavenging capacity. In conclusion, the by-products definitely have great potential for utilization and should be recycled from the wastewater in the apricot kernels processing industry, especially for amygdalin.
RESUMEN
El presente estudio se propuso investigar las composiciones químicas y la capacidad antioxidante de los subproductos (BWC, DWC) concentrados a partir del agua para escaldar y desamargar producida durante el procesamiento industrial de huesos de albaricoque. El objetivo de este ejercicio consistió en evaluar el posible desarrollo y utilización de estos subproductos. Los resultados indican que los mismos contienen gran cantidad de compuestos nutricionales, como amigdalina, proteína, azúcar reductor, polisacáridos, fenólicos y flavonoides. Entre ellos, la amigdalina concentra la mayor cantidad de BWC y DWC, esto es, 33.48 ± 0.04 y 23.89 ± 0.03 (g/100 g peso base seca), respectivamente. Asimismo, los subproductos ejercen buenas funciones antioxidantes, incluyendo poder reductor más elevado y mayor capacidad para eliminar los radicales DPPH e hidroxilo; más que al contenido elevado de amigdalina en las muestras valoradas por análisis de componentes principales y la prueba de verificación de la capacidad de eliminar el radical DPPH, esto se atribuye sobre todo a los compuestos fenólicos. En suma, definitivamente existen grandes posibilidades de utilizar los subproductos éstos deben ser reciclados del agua residual generada en la industria procesadora de huesos de albaricoque, en especial tomando en cuenta el alto contenido de amigdalina.
1. Introduction
Apricots (Prunus armeniaca L.) are processed in large quantities, with the global production reaching 4 million tons in 2012, and its large consumption consequently generates significant amounts of apricot fruit pit by-products (Górnaś et al., Citation2015). Due to the significant amounts of oil, protein, carbohydrate, soluble sugars, minerals, vitamins, and fibers, especially the most typical component of amygdalin in the apricot kernels (Femenia, Rossello, Mulet, & Canellas, Citation1995; Rudzińska, Górnaś, Raczyk, & Soliven, Citation2017; Yada, Lapsley, & Huang, Citation2011), researchers are developing some alternatives to utilize these by-products as a source of valuable bio-compounds instead of disposing them. Generally, apricot kernel is typically used as snack foods and ingredients in a variety of processed foods, notably bakery and confectionery products (Esfahlan, Jamei, & Esfahlan, Citation2010). To the above utilization, removing the apricot kernels skin and debitterizing (amygdalin removal) are the two important steps in apricot kernels processing industry (Zhang, Song, Wang, Zhao, & Fan, Citation2016).
Regarding the first step (skin removal), the traditionally effective method is to soak the apricot kernels in the hot water (90–100°C) for about 5 min, then the skins can be removed manually or mechanically (Mandalari et al., Citation2010; Zhang, Song, et al., Citation2016). For the second step, the peeled apricot kernels are immersed in the water of 30–70°C for several hours, even several days (depending on the water temperature) to reduce the content of amygdalin, a potentially toxigenic compound.
However, some water-soluble substances may be transferred to the peeling and debitterizing water during the previous two steps such as protein, amygdalin, and phenolic compounds, thus causing great loss of nutritional compounds and weights for the apricot kernels, simultaneously bringing about the environmental problems with the water directly discharged (Abud, Maiella, Nicodemi, Pettorino, & Yoshida, Citation2013; Garrido, Monagas, Gómez-Cordovés, & Bartolomé, Citation2008). According to our survey conducted in factories, the total losing rate is about 25% of its gross weight after the aforementioned two procedures. To be specific, the discharged wastewater from processing one ton of raw apricot kernels contains about 100 kg of dietary fiber, 70 kg of protein, 5 kg of flavonoids, and 20 kg of amygdalin. With the polyphenols and flavonoids transferred into the water, the color of water gets dark brown and turbidity, so the discharge of this kind of polluted water is severely prohibited by the sectors of environmental protection. Hence, the treatment of the wastewater has become a great issue or bottleneck for the apricot kernels processing factories. So some manufacturers begin to pay attention to the recycling of these active compounds (such as amygdalin and phenolics) from the wastewater. However, the work is only focused on concentrating the wastewater so as to accumulate the compounds and reduce its pollution to the environment. Moreover, the components and function still remain unknown about the concentrated substances (solid sample) at present, which has become a restrictive factor for its developing to products or ingredients in pharmacy, function food, and other fields. For instance, the fact has demonstrated that the amygdalin or the water soaked with apricot kernels (namely aqua armeniacae) has been developed for an oral liquid (cough syrup) with addition of some other medicinal herbs to treat the symptom of asthma, bronchitis, emphysema, and cough (Chang, et al., Citation2006; Smeriglio, Monteleone, & Trombetta, Citation2014; Yada et al., Citation2011).
The studies were firstly conducted on the compositions of the by-products recycled from the peeling/blanching and debitterizing wastewater during the processing of apricot kernels, then the phenolic profiles and antioxidant capacity were investigated by the high performance liquid chromatography (HPLC), three antioxidant evaluation methods, and principle component analysis (PCA), respectively. The main purpose was to verify the by-products’ potential for utilization, improve its added-value and reduce the pollution to the environment in the apricot kernels processing industry.
2. Materials and methods
2.1. Chemicals
Protocatechuic acid, protocatechuic aldehyde, chlorogenic acid, epigallocatechin (EGC), catechin, and procyanidin B1 were purchased from the Chengdu Must Bio-Technology Co. Ltd. (Sichuan Province, China). Amygdalin was purchased from the Chengdu Perferred Bio-Technology Co. Ltd. (Sichuan Province, China). Other reagents like Folin-Ciocalteu phenol reagent, DPPH (1, 1-diphenyl-2-picrylhydrazyl), gallic acid, rutin, coomassie brilliant blue G-250, BSA (bovine serum albumin), glucose, and 3,5-dinitrosalicyclic acid (DNS) were of analytical grade and bought from the National Institute for Food and Drug Control, China.
2.2. Samples and preparation
Samples of BWC and DWC were dried to solid. These samples are the by-products from the skin-peeling and debitterizing procedures of apricot kernels processing. The samples (dark brown solid) were kindly provided by the Shaanxi Tianshou Apricot Food Co. Ltd. (Shaanxi Province, China).
The samples of BWC and DWC were dissolved in water to prepare the solutions for determination, respectively.
2.3. Chemical composition analysis of BWC and DWC
2.3.1. Determination of moisture content
Five grams of sample were taken and oven-dried at 100°C till the constant weight to calculate the moisture content. In order to easily be compared, the following contents were all expressed as the percentage of the dry base weight.
2.3.2. Determination of protein content
Protein content was determined according to Coomassie brilliant blue G-250 method (Liu, Gonzalez, Wang, Gunasekaran, & Runge, Citation2015) with a TU-1810 type UV-Visible Spectrophotometer (Beijing Persee General Instruments Co. Ltd., Beijing, China). All determinations were performed in triplicate.
2.3.3. Determination of carbohydrates content
The DNS acid reagent was employed to determine the reducing sugar content (Eleazu & Eleazu, Citation2012) and the phenol-sulfuric acid method was applied to detect the total polysaccharides content (Rover, Johnston, Lamsal, & Brown, Citation2013).
2.3.4. Determination of total phenolics content
The total phenolics content of BWC and DWC were determined according to the Folin-Ciocalteu method (Górnaś, Dwiecki, Siger, & Polewski, Citation2016; Zhang, Shen, Fan, & García Martín, Citation2016). Gallic acid was used as the reference standard and the results were expressed as mg of gallic acid equivalents per g dry base weight.
2.3.5. Determination of total flavonoids content
The total flavonoids content was determined by the described method (Curcic et al., Citation2012) with some modifications. Rutin was used as the reference standard and the total flavonoids content was expressed as mg of rutin equivalents per g dry base weight.
2.3.6. Analysis of amygdalin content
The amygdalin in the samples was separated by the HPLC system (Dalian elite analytical instruments Co. Ltd., Liaoning Province, China) with a column of TC-C18 (250 mm × 4.6 mm, 5 μm). The isocratic elution consisting of solvent A (methanol), solvent B (water) was applied at a flow rate of 1.0 mL/min (28% A and 72% B). Samples were filtered through a 0.45 μm membrane and the injection volume was 20 μL. The detection wavelength was set at 215 nm. Data acquisition was performed using the EC2006 software, and quantification was carried out according to the calibration curves constructed with standards.
2.4. HPLC analysis of phenolic compounds
The determination of phenolic compounds in BWC and DWC was performed by the HPLC, and the elution consisting of solvent A (methanol /acetic acid, 99/1, v/v) and solvent B (water/acetic acid, 99/1, v/v) was applied at a flow rate of 1.0 mL/min as the following gradients for solvent A: 0 min 5%, 0–15 min 5–35%, 15–25 min 35%, and 25–35 min 35–5%. Samples were filtered through a 0.45 μm membrane and the injection volume was 20 μL. The detection wavelength was set at 280 nm. Data acquisition was performed using the EC2006 software. Identification of the compounds was carried out by comparing their spectra and retention time with the added internal standards, and quantification was calculated by the external standard calibration curves.
2.5. Antioxidant capacity evaluation of BWC and DWC
2.5.1. Reducing power
The reducing power of the BWC and DWC was determined according to the procedures in the literature (Martorana et al., Citation2013). The higher the absorbance, the stronger the reducing power.
2.5.2. DPPH·−scavenging activity
The stable DPPH radical is usually used to evaluate the compound’s scavenging activity, which has a characteristic absorption at 517 nm and disappears upon reduction by the compounds occurring antioxidant capacity. The test was conducted according to the reports by Zhang, Shen, et al. (Citation2016) and the result was calculated using the following equation:
where Ai, absorbance of the sample; A0, absorbance of the blank.
2.5.3. Hydroxyl radical-scavenging activity
The hydroxyl radical-scavenging activity was carried out using the method described by Rad, Alfatemi, Rad, and Iriti (Citation2014). Results were determined using the following equation:
where Ai, absorbance of the sample; Aj, absorbance of sample without H2O2; A0, absorbance of the blank.
2.6. Statistical analysis
All experiments were repeated three times and determinations were performed in triplicate. The results were statistically analyzed by calculating the mean and standard deviation, and presented as means ± standard deviations. The interpretation of the results was performed with Excel 2010 and one-way of ANOVA followed by Data Processing System (DPS). The differences were considered statistically significant at p < 0.05. PCA was using the Unscrambler Data Analysis,which is a multivariate statistical method that examines correlations among variables, and explains what samples are similar or dissimilar to each other.
3. Results and discussion
3.1. Chemical compositions of BWC and DWC
The results indicate that the moisture contents are 6.36% and 2.99% for the samples of BWC and DWC, respectively. The main compositions were determined and shown in . Obviously, the most representative compound is amygdalin (D-mandelonitrile-β-D-gentiobioside), and the content is 33.48 ± 0.04 and 23.89 ± 0.03 (g/100 dry base weight) for the samples of BWC and DWC, respectively. Generally, the content of amygdalin is about 5.5 g/100 g in the apricot kernels (Femenia et al., Citation1995), and it may leak from the skins and kernels into the water during the blanching and debitterizing procedures, being the origin of amygdalin in the BWC and DWC. In the meantime, the amygdalin in water might degrade, especially under the involvement of enzymes in the kernels, which explained the significant differences of the content between the BWC, DWC, and the original apricot kernels. Considering the great numbers of amygdalin in the samples of BWC and DWC, these by-products should be recycled and utilized as a good source of amygdalin in the processing industry of apricot kernels.
Table 1. Chemical compositions of BWC and DWC.
Tabla 1. Composiciones químicas de BWC y DWC.
Apart from the amygdalin, there are also relatively high contents of protein, reducing sugar and polysaccharides in the samples, which are 4.17, 4.59, 23.55 and 10.32, 7.14, 14.34 (g/100 dry base weight) for the BWC and DWC, respectively. The higher protein content in DWC may be related to its higher content (23.74–25.70%) in the apricot kernels itself and more transferring during debitterizing of longer contact with water. As for the total phenolics and flavonoids both in BWC and DWC, the contents are significantly lower than those of other compounds such as amygdalin, protein, reducing sugar, and polysaccharides, which are only 1.38, 2.48 and 0.84, 0.52 (g/100 dry base weight) for the BWC and DWC, respectively.
3.2. HPLC analysis of phenolic compounds in BWC and DWC
As mentioned in the previous section, the BWC and DWC contain some active phenolics and flavonoids. In order to fully understand the specific component, HPLC was employed to separate and identify the phenolic compounds in samples, and a typical combined chromatogram is shown in . Obviously, six phenolic compounds could be identified in the sample of BWC and three in DWC by matching the spectra and retention time with those of the standards. shows the regression equation, coefficient and linear range for the six standards conducted by the HPLC analysis. The results demonstrate that the content of the most predominate compound (protocatechuic aldehyde) is 2.69 mg/g (dry base) and 1.24 mg/g in BWC and DWC, respectively. In comparison, the contents of the other identified compounds in BWC are a bitter lower, which is 0.34 mg/g, 0.20 mg/g, 0.57 mg/g, 0.44 mg/g, and 1.01 mg/g for the procyanidin B1, EGC, protocatechuic acid, catechin and chlorogenic acid, respectively. To the sample of DWC, three peaks were identified as the compounds of procyanidin B1 (0.29 mg/g), catechin (0.23 mg/g), and protocatechuic aldehyde (1.24 mg/g).
Table 2. Regression equation, coefficient and linear range for the standard compounds of protocatechuic acid, procyanidin B1, EGC, protocatechuic aldehyde, catechin, chlorogenic acid by HPLC.
Tabla 2. Ecuación de regresión, coeficiente y rango lineal de los compuestos estándar de ácido protocatecuico, procianidina B1, EGC, aldehído protocatecuico, catequina, ácido clorogénico a partir de HPLC.
Figure 1. Combined HPLC chromatogram of phenolic compounds in the samples. Peak number: 1. protocatechuic acid, 2. procyanidin B1, 3. EGC, 4. protocatechuic aldehyde, 5. catechin 6. chlorogenic acid.
Figura 1. Cromatograma combinado HPLC de los compuestos fenólicos en las muestras. Número pico: 1. ácido protocatecuico; 2. procianidina B1; 3. EGC; 4. aldehído protocatecuico; 5. catequina; 6. ácido clorogénico.
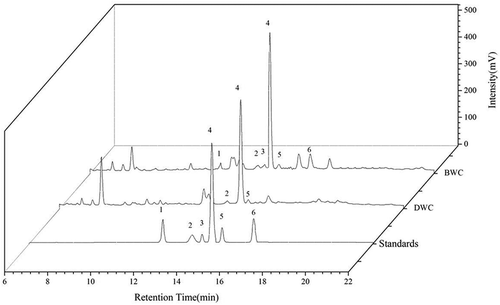
3.3. Antioxidant activity of the BWC and DWC
Recent years, researchers have pointed out that the antioxidants can delay or restrain the oxidation of molecules by inhibiting the initiation of the free radical-induced chain reactions (Esfahlan et al., Citation2010). Due to the chemical complexity of the sample, often a mixture of compounds with different polarities, chemical behaviors, and functional groups, the results of the antioxidant tests are usually scattered, closely depending on the assay employed. Thus, it is oversimplified and inappropriate to select only one single method to evaluate the antioxidant capacity. Therefore, several assays were conducted to provide a more reliable result about the antioxidant and radical-scavenging potential of the BWC and DWC.
As shown in (a), both the BWC and DWC illustrate the perfect reducing power with the increase of concentration, i.e. an obvious dose-effect manner is exerted between either the BWC or the DWC and the reducing power. In the reducing power assay, with the reduction of the Fe3+/ferricyanide complex to the ferrous form, the original yellow color of the solution turns into the green/blue, and the intensity depends on the reducing power of the antioxidants existing in the solution, so the Fe2+ can be monitored by the absorbance at 700 nm (Ferreira, Baptista, Vilas-Boas, & Barros, Citation2007). Regarding the results, the strong reducing ability may suggest the by-products’ function of oxidation resistance, furthermore, the BWC and DWC may donate electrons to free radicals or end up the free radical-mediated chain reactions due to the presence of the phenolic compounds.
Figure 2. Antioxidant capacity evaluation of BWC and DWC, (a) reducing power, (b) scavenging effect of DPPH free radical, (c) scavenging effect of OH free radical.
Figura 2. Evaluación de la capacidad antioxidante de BWC y DWC, (a) Poder reductor, (b) Efecto de eliminar el radical libre DPPH, (c) Efecto de eliminar el radical libre OH.
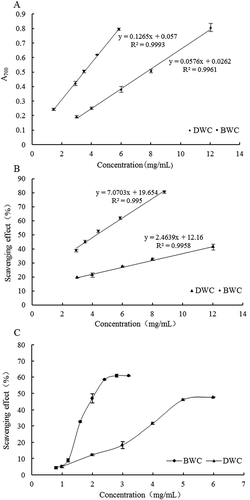
Due to the DPPH radical perfect hydrogen-donating ability, the test of scavenging the DPPH radical by antioxidants is widely employed (Kalaivani & Mathew, Citation2010). As depicted in (b), both the BWC and DWC show a concentration-dependent scavenging manner of DPPH radical, i.e. the scavenging capacity of the samples toward the DPPH radical was highly correlated with the concentration. In comparison, the BWC has a stronger DPPH radical-scavenging capacity than that of the DWC at a given concentration, which can also be proved by the IC50 value, namely, the IC50 value of BWC and DWC is 4.24 mg/mL and 14.77 mg/mL, respectively. The IC50 is defined as the amount of the antioxidants necessary to decrease the initial DPPH radical concentration by 50%. Generally, the lower the IC50 value, the stronger the scavenging capacity of the compound. The lower IC50 value of BWC can be attributed to the higher content of phenolic compounds as analyzed earlier.
The scavenging activity of hydroxyl radical is an important index for measuring antioxidant capacity (Singh & Rajini, Citation2004). Hydroxyl-free radicals-scavenging capacity of BWC and DWC is depicted in . Obviously, the hydroxyl-free radicals can be scavenged from 4.3–60.9% by the sample of BWC within the tested concentrations, and a significantly increasing trend was shown about the hydroxyl-free radicals-scavenging ability within the tested concentrations of 0.8–2.4 mg/mL. Similarly, the sample of DWC also demonstrated a concentration-dependent scavenging activity on the hydroxyl-free radicals at the range of 1–5 mg/mL. It is interesting that both BWC and DWC have a very limited scavenging capacity on the hydroxyl-free radicals, since the increasing trend is not obvious with the further increase of concentrations, namely, exceeding 2.4 mg/mL and 5 mg/mL for the BWC and DWC, respectively.
In a word, the BWC and DWC have a good antioxidant capacity including the higher reducing power, and the higher scavenging ability on the DPPH and hydroxyl-free radicals, which may suggest the great potential applied in cosmetics or functional food to be acted as the natural antioxidants in absorbing and neutralizing the free radicals produced in the metabolism of human body (Kim, Sim, & Choi, Citation2010), since the safety of the synthetic antioxidants is so severely concerned by the consumers.
3.4. Validation of the effect of amygdalin on scavenging the DPPH radical
As mentioned in the sections earlier, amygdalin is the most representative compound in both BWC and DWC, as the dominant compound in the by-products, we would like to know whether the amygdalin contributes to the antioxidant capacity or not. According to the reports available, the amygdalin was considered to have the function of scavenging the DPPH-free radical. To be specific, Dong, Yin, Zhang, Yang, and Chen (Citation2007) reported that the amygdalin extracted from apricot blossom pollen could scavenge the DPPH-free radical under the tested conditions. In addition, Zhao (Citation2010) also presented her results about the good DPPH radical-scavenging ability of amygdalin extracts from the loquat core. illustrates the scanned spectral about the effect of standard amygdalin on the DPPH radical-scavenging capacity; interestingly, the absorption at 517 nm did not decrease, but increased slightly. That is to say, the standard amygdalin has no impact on DPPH radical-scavenging capacity, so we can infer that the DPPH-free radical-scavenging capacity of BWC and DWC cannot be attributed to the amygdalin contained in them. Generally, the DPPH radical solution would become colorless or pale yellow when neutralized, and the scavenged radicals can be counted from the changes of the absorption at 517 nm (Sharma & Bhat, Citation2009). Regarding the results reported by Dong et al. (Citation2007) and Zhao (Citation2010), the reason might be the fact that the extracts of amygdalin are not pure enough, and some phenolic compounds may be included in the extracts since the blossom pollen or loquat core also contains the antioxidant phenolics.
3.5 Principal component analysis about the correlation between phenolic compounds and antioxidant capacity in BWC and DWC
To determine the correlation between the content of phenolic compounds and the antioxidant capacity in BWC and DWC, the principal component analysis (PCA) and the correlation coefficient were applied in this study. The correlation coefficient of the antioxidant capacity and the identified phenolic compounds are shown in . The result of PCA contains 4 of the predefined plots that are available, scores plot and loadings plot are the most significant, which can show that which samples are similar or dissimilar, and positive or negative relationships, respectively. In the scores plot, the closer the samples, the smaller the difference, and the sample closer to the center is an average one. displays that the sample of BWC1, BWC2, DWC1, and DWC2 can be discriminated each other (1 and 2 represent the different concentrations of BWC and DWC samples, respectively). demonstrates the correlation loadings plot about the samples between the individual phenolic compounds and the antioxidant activity. Obviously, the and suggest that significantly positive correlations exist between all the individual phenolic compounds and the antioxidant capacity, i.e. regarding the protocatechuic acid, chlorogenic acid, and EGC, they are more closely with the DPPH radical-scavenging activity than the reducing power and the hydroxyl radical-scavenging activity, the correlation coefficients also illustrate this point, which means that the protocatechuic acid, chlorogenic acid, and EGC have the stronger DPPH radical-scavenging activity. At the same time, it also explains the results in that the BWC has the stronger antioxidant capacity than that of the DWC within the tested concentrations, especially the DPPH radical-scavenging activity, since these three compounds were not detected in the sample of DWC. Protocatechuic aldehyde highly correlated with the reducing power and OH radical-scavenging capacity, so were catechin and procyanidin B1.
Table 3. The correlation coefficient of antioxidant capacity and identified phenolic compounds.
Tabla 3. Coeficiente de correlación de la capacidad antioxidante y los compuestos fenólicos identificados.
4. Conclusions
The results indicate that the by-products (BWC and DWC) still contain high valuable compounds like protein, reducing sugar, and polysaccharides. Especially for the amygdalin, as the most abundant component, its separation from other bioactive compounds is quite important. Antioxidant tests have clearly demonstrated the radical-scavenging capacity of BWC and DWC as well as its potential use, which highly correlated with the identified phenolic compounds, not the abundant amygdalin in the samples. In summary, the bioactive compounds in the by-products generated during the apricot kernels processing industry should be recycled, entailing financial and environmental benefits for the different branches of the industry.
Disclosure statement
No potential conflict of interest was reported by the authors.
Additional information
Funding
References
- Abud, M., Maiella, G., Nicodemi, F., Pettorino, R., & Yoshida, K. (2013). Antioxidant and photoprotective effects of blanch water, a byproduct of the almond processing industry. Molecules, 18(10), 12426–12440.
- Chang, H.-K., Shin, M.-S., Yang, H.-Y., Lee, J.-W., Kim, Y.-S., Lee, M.-H., … Kim, C.-J. (2006). Amygdalin induces apoptosis through regulation of Bax and Bcl-2 expressions in human DU145 and LNCaP prostate cancer cells. Biological & Pharmaceutical Bulletin, 29(8), 1597–1602.
- Curcic, M. G., Stankovic, M. S., Radojevic, I. D., Stefanovic, O. D., Comic, L. R., Topuzovic, M. D., … Markovic, S. D. (2012). Biological effects, total phenolic content and flavonoid concentrations of fragrant yellow onion (Allium flavum L.). Medicinal Chemistry, 8, 46–51.
- Dong, J., Yin, C., Zhang, H. C., Yang, L. C., & Chen, C. L. (2007). Research on antioxidative activities of amygdlin from almond pollen. Food Science, 28, 65–68. ( (In Chinese)).
- Eleazu, C. O., & Eleazu, K. C. (2012). Determination of the proximate composition, total carotenoid, reducing sugars and residual cyanide levels of flours of 6 new yellow and white cassava (Manihot esculenta Crantz) varieties. American Journal of Food Technology, 7, 642–649.
- Esfahlan, A. J., Jamei, R., & Esfahlan, R. J. (2010). The importance of almond (Prunus amygdalus L.) and its by-products. Food Chemistry, 120(2), 349–360.
- Femenia, A., Rossello, C., Mulet, A., & Canellas, J. (1995). Chemical composition of bitter and sweet apricot kernels. Journal of Agricultural and Food Chemistry, 43, 356–361.
- Ferreira, I. C. F. R., Baptista, P., Vilas-Boas, M., & Barros, L. (2007). Free-radical scavenging capacity and reducing power of wild edible mushrooms from northeast Portugal: Individual cap and stipe activity. Food Chemistry, 100, 1511–1516.
- Garrido, I., Monagas, M., Gómez-Cordovés, C., & Bartolomé, B. (2008). Polyphenols and antioxidant properties of almond skins: Influence of industrial processing. Journal of Food Science, 73(2), 106–115.
- Górnaś, P., Dwiecki, K., Siger, A., & Polewski, K. (2016). Contribution of phenolic acids isolated from green and roasted boiled-type coffee brews to total coffee antioxidant capacity. European Food Research and Technology, 242(5), 641–653.
- Górnaś, P., Mišina, I., Grāvīte, I., Soliven, A., Kaufmane, E., & Segliņa, D. (2015). Tocochromanols composition in kernels recovered from different apricot varieties: RP-HPLC/FLD and RP-UPLC-ESI/MSn study. Natural Product Research, 29, 1222–1227.
- Kalaivani, T., & Mathew, L. (2010). Free radical scavenging activity from leaves of acacia nilotica (L.) wild. ex Delile, an Indian medicinal tree. Food and Chemical Toxicology, 48, 298–305.
- Kim, S. I., Sim, K. H., & Choi, H.-Y. (2010). A comparative study of antioxidant activity in some Korean medicinal plant used as food materials. Molecular & Cellular Toxicology, 6, 279–285.
- Liu, Z., Gonzalez, J. S., Wang, H., Gunasekaran, S., & Runge, T. (2015). Dairy manure protein analysis using UV-vis based on the Bradford method. Analytical Methods, 7, 2645–2652.
- Mandalari, G., Tomaino, A., Arcoraci, T., Martorana, M., Turco, V. L., Cacciola, F., … Dugo, P. (2010). Characterization of polyphenols, lipids and dietary fibre from almond skins (Amygdalus communis L.). Journal of Food Composition and Analysis, 23(2), 166–174.
- Martorana, M., Arcoraci, T., Rizza, L., Cristani, M., Bonina, F. P., Saija, A., … Tomaino, A. (2013). In vitro antioxidant and in vivo photoprotective effect of pistachio (Pistacia vera L., variety Bronte) seed and skin extracts. Fitoterapia, 85, 41–48.
- Rad, J. S., Alfatemi, S. M. H., Rad, M. S., & Iriti, M. (2014). Free radical scavenging and antioxidant activities of different parts of nitraria schoberi L. Journal of Biologically Active Products from Nature, 4, 44–51.
- Rover, M. R., Johnston, P. A., Lamsal, B. P., & Brown, R. C. (2013). Total water-soluble sugars quantification in bio-oil using the phenol-sulfuric acid assay. Journal of Analytical and Applied Pyrolysis, 104, 194–201.
- Rudzińska, M., Górnaś, P., Raczyk, M., & Soliven, A. (2017). Sterols and squalene in apricot (Prunus armeniaca L.) kernel oils: The variety as a key factor. Natural Product Research, 31, 84–88.
- Sharma, O. P., & Bhat, T. K. (2009). DPPH antioxidant assay revisited. Food Chemistry, 113, 1202–1205.
- Singh, N., & Rajini, P. S. (2004). Free radical scavenging activity of an aqueous extract of potato peel. Food Chemistry, 85, 611–616.
- Smeriglio, A., Monteleone, D., & Trombetta, D. (2014). Health effects of Vaccinium myrtillus L.: Evaluation of efficacy and technological strategies for preservation of active ingredients. Mini Reviews in Medicinal Chemistry, 14(7), 567–584.
- Yada, S., Lapsley, K., & Huang, G. (2011). A review of composition studies of cultivated almonds: Macronutrients and micronutrients. Journal of Food Composition and Analysis, 24, 469–480.
- Zhang, Q.-A., Shen, Y., Fan, X.-H., & García Martín, J. F. (2016). Preliminary study of the effect of ultrasound on physicochemical properties of red wine. CyTA-Journal of Food, 14, 55–64.
- Zhang, Q.-A., Song, Y., Wang, X., Zhao, W.-Q., & Fan, X.-H. (2016). Mathematical modeling of debittered apricot (Prunus armeniaca L.) kernels during thin-layer drying. CyTA-Journal of Food, 14, 509–517.
- Zhao, J. (2010). Extraction, purification and antioxidant activity of amygdalin in Loquat seed ( Master thesis of Nanjing Agricultural University, pp. 48–49). (In Chinese).