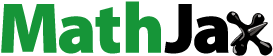
ABSTRACT
Oxidative stress and mitochondrial dysfunction play an important role in the onset of neurodegenerative diseases; thus, the antioxidant use could be a promising preventive or therapeutic intervention. The food and agricultural industries generate huge quantities of by-products rich in biophenolic compounds. If, on the one hand, they represent a serious environmental problem, on the other hand, are valuable natural sources of antioxidants. In the present work, the total phenolic content and the antioxidant power of the wastewater derived from the production of four Sicilian extra virgin olive oils are shown. Four biophenols, hydroxytyrosol and tyrosol alcohols and caffeic acid (CA) and p-coumaric acids, were identified and quantified and their antioxidant ability determined. Their effects in counteracting Abeta1-42 oligomer oxidative damage in LAN5 cell culture were investigated. Results indicated that CA was the most effective compound in counteracting the cell damages induced by Abeta1-42 oligomers.
RESUMEN
El estrés oxidativo y la disfunción mitocondrial juegan un rol importante en el comienzo de ciertas enfermedades neurodegenerativas; en estos casos, el uso de antioxidantes puede ser una intervención preventiva o terapéutica prometedora. Las industrias alimentarias y agrícolas producen enormes cantidades de subproductos ricos en compuestos biofenólicos. Si bien por un lado representan un grave problema ambiental, por el otro constituyen valiosas fuentes naturales de antioxidantes. En el presente estudio se analizan el contenido fenólico total y el poder antioxidante de las aguas residuales (WW) derivadas de la producción de cuatro aceites de oliva virgen extra (AOVE) de Sicilia. Para el efecto, Se identificaron y cuantificaron cuatro biofenoles, alcoholes hidroxitirosol (HT) y tirosol (TY), además de ácidos cafeico (CA) y p-cumárico (CU), y se determinó su capacidad antioxidante. Asimismo, se investigaron sus efectos para contrarrestar el daño oxidativo provocado por el oligómero Abeta1-42 en cultivos celulares LAN5, constatándose que el CA fue el más efectivo para contrarrestar el daño celular inducido por oligómeros Abeta1-42.
Introduction
Oxidative stress is one of the major cellular features in the pathophysiology of many severe human illnesses, among which the neurodegenerative Alzheimer’s disease (AD). It occurs when there is an excessive generation of free radicals, normally produced during metabolic processes in the cell, and physiologically counteracted by the antioxidative defense system (Kulawik, Ozogul, Glew, & Özogul, Citation2013). Thus, free radical excess can induce lipid and protein oxidation, cell membrane integrity disruption and DNA damages causing serious pathologies (Blasiak, Glowacki, Kauppinen, & Kaarniranta, Citation2013; Sharma, Jha, Dubey, & Pessarakli, Citation2012). Hence, application of external source of antioxidants can give a substantial help in fighting cell oxidative stress and its consequences. Since synthetic antioxidants have been reported to be dangerous for human health (Gould, Citation1995), the use of the so-called functional foods, that is, foods playing a great contribution to the prevention and reduction of risk factors for several diseases or improving the physiological functions, has become an important practice in the current nutrition theory (Ballard, Mallikarjunan, Zhou, & O’Keefe, Citation2009; Panusa, Zuorro, Lavecchia, Marrosu, & Petrucci, Citation2013). Moreover, several experimental evidences have suggested that the use of antioxidants could have a role in prevention and onset of several pathologies including neurodegenerative diseases (Sgarbossa, Giacomazza, & Di Carlo, Citation2015).
AD is the most common form of dementia in the elderly. It impairs higher brain functions such as memory, thinking and personality. It is characterized by neuronal cell loss and increasing accumulation of neurofibrillary tangles in neurons, and amyloid fibers in neuritic plaques and in the walls of blood vessels (Serrano-Pozo, Frosch, Masliah, & Hyman, Citation2011).
Amyloid beta peptide (Abeta) (39–43 residues), derived from the larger transmembrane protein called amyloid precursor protein (APP) (Wilqueta & De Strooper, Citation2004), is the main component of the amyloid plaques present in the brain of Alzheimer-affected patients. Accumulation of Abeta is hypothesized to initiate a pathogenic cascade that eventually leads to AD (Hardy & Selkoe, Citation2002).
Biophenols are a heterogeneous class of chemical compounds widely diffused in plants. They are secondary metabolism molecules derived by the pentose phosphate, shikimate and phenylpropanoid pathways (Balasundram, Sundram, & Samman, Citation2006). Based on their solubility properties, they can be divided into lipophilic and hydrophilic. Tocopherols (or vitamin E), representing the lipophilic phenol fraction, can be found in different types of vegetable oils; on the contrary, some hydrophilic phenols are a prerogative of extra virgin olive oil (EVOO) (El Riachy, Priego-Capote, Leon, Rallo, & Luque De Castro, Citation2011). The antioxidant activity of biophenols present in EVOO is responsible for its prolonged shelf-life because they avoid lipid oxidation through a variety of mechanisms based on radical scavenging, hydrogen atom transfer and metal-chelation (El Riachy et al., Citation2011). Despite their low concentration, phenolic compounds have been related with the healthy beneficial effects derived from EVOO intake (Casamenti & Stefani, Citation2017; Galli, Citation2006).
Many factors affect the final composition and stability of EVOO. Among these factors an important role is played by (i) cultivation altitude (Garcia-Martinez, Mazzaglia, Sanchez-Ortiz, Ocana-Peinado, & Rivas, Citation2014), (ii) olive cultivar (Obied, Bedgood, Mailer, Prenzler, & Robards, Citation2008), (iii) harvesting time (Giuffrè, Piscopo, Sicari, & Poiana, Citation2010), (vi) irrigation (Gómez-Rico et al., Citation2007; Servili et al., Citation2007) and (v) milling technology (Ben Sassi, Boularbah, Jaouad, Walker, & Boussaid, Citation2006).
During the EVOO production, in the so-called two-phase method, the last centrifugation step allows the separation of the oil from the wet paste. Contrarily to the three-phase method, the two-phase processing does not require water, added to facilitate the separation between oil and paste. The two-phase method enhances the bouquet of the EVOOs by allowing the maintenance of a higher percentage of natural antioxidants, thus ensuring a great quality of the final product (Kiritsakis, Citation1998).
Manufacturing of the EVOO, produced either through the two-phase or three-phase processes, causes the formation of two main by-products: a solid waste (pomace) and an aqueous liquid product [olive mill wastewater (WW)]. Both by-products create serious disposal problem because of their phytotoxic content (Capasso, Cristinzio, Evidente, & Scognamiglio, Citation1992; El-Abassi, Kiai, & Hafidi, Citation2012) mainly due to the high concentration of the same biophenols present in EVOO (Tsagaraki, Lazarides, & Petrotos, Citation2007). Furthermore, from the whole ecosystem point of view, 1 m3 of WW has been reported to be correspondent to 200 m3 of domestic sewage (Visioli et al., Citation1999).
If produced through the three-phase method, the olive mill WW and the pomace are both present; while in the case of the two-phase process only the solid waste is obtained. After centrifugation, the pomace can be further separated as WW and solid waste. In the latter method, the WW amount is mainly related to the water content in olives whose variability is influenced by cultivar type, biodiversity of geographic region as well as irrigation methods (Aganchich et al., Citation2008; Giuffrè, Citation2017; Kılıçkan & Güner, Citation2008)
The recovery of phenolic antioxidants from EVOO WW, in addition to their pharmacological and beneficial applications, constitutes a valid alternative for valorizing this problematic by-product (Reboredo-Rodriguez et al., Citation2016).
In the present work, we quantified the phenolic content and the antioxidant ability of four WWs derived from four Sicilian EVOOs, produced with the two-phase method. The total biophenol content of each WW was compared to that one of related EVOO. Furthermore, four biophenols consisting of one aromatic ring and one or more hydroxylic (–OH) groups, the hydroxytyrosol (HT) and tyrosol (TY) alcohols and the caffeic acid (CA) and p-coumaric (CU) acid, were identified and quantified in every single oil WW phenolic extract by high-performance liquid chromatography (HPLC) analysis. The antioxidant ability of the four biophenols, representing a large fraction of the total biophenols, was determined by oxygen radical absorbance capacity (ORAC) assay. Finally, at the best of our knowledge, for the first time, the four biophenols were compared to each other in relation to their effect in counteracting the oxidative damage induced by addition of Abeta oligomer in LAN5 cell culture. Results indicated that CA was the most effective compound in counteracting the cell damages induced by Abeta oligomer injury, allowing almost the complete defense of the cell viability and morphology.
Materials and methods
Geographical, microclimatic and agronomical data of the olive cultivations
Four WWs and four related EVOOs were kindly supplied in 2015 by Sicilian producers of the Sciacca area (Trapani, Sicily, Italy, Long. 13.039708, latitude 37.591930; 60 m a.s.l.), in the frame of the European Fund for Regional Development project. All the WW samples were obtained at the end of the production of the corresponding EVOOs. The samples were produced from Biancolilla (EVOO1 and WW1), Cerasuola (EVOO2 and WW2; EVOO3 and WW3) and Nocellara del Belice (EVOO4 and WW4) cultivars. All samples were from the same geographical area. The production techniques were the same for all samples. The Nocellara del Belice and Cerasuola olive fruits were roundish and large size with a mean weight between 5 and 7 g, while Biancolilla had an elliptical shape with a weight that not exceed 4 g.
In the Sciacca area, the annual temperature range was from 5°C (February) to 38°C (in August), the total rain was about 700 mm/year. The average healthy tree age was about 40, and the distance among the plants was about 6 m. The soil type was calcareous and clayey. The trees were fertilized with a complex manure containing high nitrogen content. Dry-farmed (rainfed) olive trees were pruned from October to March every 2 years. Plants were opportunely treated against Lytta vesicatoria (Spanish fly), Bactrocera oleae (oil fly), Spilocaea oleaginea (peacock eye) and Colletotrichum gloeosporioides (olive anthracnose).
Two-phase EVOO production steps
After harvesting, the olives were stored in the mill for no more than 48 h. Once washed, the olives were moved in the hammer crusher (Pieralisi) that usually works at 2900 rpm. In this step, the temperature of the sample increases of about 4–10°C with respect to the room temperature. Then, the olive pits were eliminated by the stoner operating at 500–600 rpm. The obtained olive paste was then transferred in the kneading tanks (Molinova, Pieralisi) for the malaxation step. The time required for this step is 40–60 min at the temperature of 25°C. The oil production ended in the two-phase decanter (Pieralisi, 3600 rpm) and centrifuge (Pieralisi, about 5000 rpm). In the last step, the WW is obtained together with the solid pomace and separated by centrifugation. At the end of the process, the EVOO was filtered through a coarse filter. Time necessary for the whole process did not exceed 24 h.
Materials and chemicals
Each WW was obtained at the end of the two-phase production of the related EVOO, after centrifugation of the solid pomace obtained after oil production. The identification code of each sample is reported in .
Table 1. Identification code of the four EVOO and WW samples.
Tabla 1. Código de identificación de las cuatro muestras AOVE y WW. Las muestras 1, 2 y 4 fueron producidas en la misma parcela agrícola.
All samples were stored in the dark at 4°C. Methanol (HPLC grade), acetonitrile (HPLC grade), phosphoric acid 85%, Folin–Ciocalteu solution, sodium carbonate, fluorescein, 2,2′-Azobis(2-amidinopropane) dihydrochloride (AAPH), (±)-6-hydroxy-2,5,7,8-tetramethylchromane-2-carboxylic acid (trolox), 2-(4-hydroxyphenyl)ethanol TY, HT, gallic acid, syringic acid, vanillin, ferulic acid, vanillic acid, CA and CU acid, dichlorofluorescin diacetate (DCFH-DA) were purchased from Sigma-Aldrich (Milan, Italia). CellTiter 96® Aqueous One Solution Assay (MTS) was purchased from Promega. The water used in all experiments was Millipore MilliQ.
Sample preparation
The sample preparation for the determination of biophenols in EVOO and WW was based on the method proposed by Goldsmith, Stathopoulos, Golding, and Roach (Citation2014). Five grams of WW were added to 15 mL of methanol:water (80:20 v/v) mixture. Samples were vortexed for 2 min before extraction in an ultrasonic bath for 15 min at 4°C and centrifuged at 3000 g for 25 min at 4°C in a Beckman Avanti 30 Compact centrifuge (Beckman Coulter, Italy). The supernatant phase was then taken and filtered through a 0.45 μm nylon PVF filter (Grace Alltec) before further analysis.
Determination of total phenolic compounds: Folin–Ciocalteu method
The Folin–Ciocalteu assay was done in agreement with Hrncirik and Frische (Citation2004) with a few minor modifications. A standard curve was developed using gallic acid, which is linear between 62.5 ÷ 250 mg/mL. The biophenol methanolic solution was diluted 1:100 with methanol:water (80:20 v/v). Then, 0.2 mL were added to 4.8 mL of water to a total volume of 5 mL, followed by the addition of 0.5 mL Folin–Ciocalteu reagent. After 3 min, 1 mL sodium carbonate solution (20%, w/v) was added to the reaction mixture, which was finally mixed and diluted with water to 10 mL total volume. The absorbance of the solution was measured against a blank sample after 2 h on a Shimadzu Spectrophotometer at the wavelength of 765 nm.
HPLC experiments
The methanol:water (80:20 v/v) extracts were analyzed using a Shimadzu HPLC system LC-2010 AT equipped with an UV-vis photodiode array detector (SPD-M20A) and a 20-µL sample loop. A Supelco Discovery C18 (250 mm × 4 mm i.d. × 5 μm particle size) column was used with detection at 280 nm. The column was maintained at 25°C, the flow rate was 1 mL/min. Three solvents were used for the mobile phase (A, B and C), and the programmed elution gradient was applied, as reported in .
Table 2. Gradient applied during the HPLC experiments for the identification of the biophenolic fraction of the wastewater.
Tabla 2. Gradiente aplicado durante los experimentos de cromatografía líquida de alta eficacia (HPLC) para la identificación de la fracción biofenólica del agua residual.
The solvent composition reported in the last row of has been maintained for further 3 min to the aim of equilibrating the column for the next sample injection. The recognition of the biophenols and the determination of their concentrations have been done through the use of standards.
ORAC assay
The method reported by Ninfali, Bacchiocca, Biagiotti, Servili, and Montedoro (Citation2002), slightly modified, was applied. The reaction mixtures were prepared in a 96-well black microplate as follows: 160 μL of 0.04 μM fluorescein in 0.075 M Na-K phosphate buffer pH 7.0, 20 μL of appropriately diluted phenolic extract or 20 μL single methanol:water (80:20 v/v) standard solution (10 μg/mL) or 20 μL of 100 μM Trolox, the latter was used as standard reference. The mixtures were kept 10 min at 37°C in the dark, and the reaction was started with the addition of 20 μL of 40 mM 2-2ʹ-azobis-2-methyl-propanimidamide, dihydrochloride (AAPH). The control was the methanol solution (80:20 v/v) properly diluted in 0.075 M Na-K phosphate buffer (pH 7.0). The fluorescence decay was measured at 37°C every 5 min at 485 nm excitation and 538 nm emission, using a Thermo Scientific Fluoroskan Ascent F2 Microplate. The ORAC value refers to the net area under the curve of fluorescein decay in the presence of WW phenolic extract or single standard solution or Trolox, subtracted of the blank area. The activity of the sample was expressed as μmol of Trolox Equivalents (TE)/g of WW by using the following equation:
where k is the total dilution of the methanolic extract, a is the ratio between the volume (L) of the phenolic extract and grams of WW and in the case of standards it corresponds to their initial concentration, h is the final concentration of Trolox expressed as μmol/L and S is the area under the curve of fluorescein in the presence of sample, Trolox, or buffer solution.
Cell cultures and treatments
LAN5 neuroblastoma cells were cultured with RPMI 1640 medium (Celbio srl, Milan, Italy) supplemented with 10% heat-inactivated fetal bovine serum (Gibco-Invitrogen, Milan, Italy), 2 mM l-glutamine and 1% penicillin, 1% streptomycin (50 mg/mL). Cells were maintained in a humidified 5% CO2 atmosphere at 37 ± 0.1°C. For experiments, HT and TY alcohols and CA and CU acids were separately added to the LAN5 cell culture in a range 12.5–100 μM for 24 h with or without recombinant Abeta1-42 oligomers (40 μM) obtained as in Carrotta et al. (Citation2006). The four antioxidants were initially prepared in ethanol and diluted at final concentration in culture medium. A microscope (Zeiss Axio Scope) with a camera (Axiocam) was utilized to analyze the morphology of the cells.
Determination of cell viability
Cell viability was measured by MTS assay (PROMEGA). 1 × 106/mL LAN5 cells were plated in 96-well plate and after 24 h were untreated (control) or treated as described above. MTS [3-(4,5-dimethylthiazol-2-yl)-5-(3-carboxymethoxyphenyl)-2-(4-sulphopheyl)2H-tetrazolium] was used according to the manufacturer’s instructions. After cell treatments, 20 μL of the MTS solution were added to each well and incubated with cells for 4 h at 37°C, 5% CO2. The absorbance was read at 490 nm with the Microplate reader WallacVictor 2 1420 Multilabel Counter (Perkin Elmer). Results were expressed as the percentage of MTS reduction relative to the control.
Analysis of the generation of reactive oxygen species (ROS)
To assess ROS generation by fluorimetric analysis, LAN5 cells (6 × 105 cells/mL) were plated in a 96-well optical bottom white microplate or in a 96-well transparent plate for the microscope fluorescence analysis. After the treatments, the cells were incubated with 1 μM DCFH-DA in PBS for 10 min at room temperature in the dark. The conversion from non-fluorescent DCFH-DA to the highly fluorescent compound 2ʹ,7ʹ-dichlorofluorescin (DCF), due to the presence of ROS, is used for monitoring the oxidative burst in the cells. The emitted fluorescence is proportional to the concentration of ROS inside the cell. Therefore, after washing in PBS the cells were analyzed by fluorimeter (Microplate reader WallacVictor 2 1420 Multilabel Counter, PerkinElmer, Inc.) and fluorescence microscope (Zeiss Axio Scope 2). The excitation filter was set at 485 nm and the emission filter at 530 nm.
Analysis of mitochondrial superoxide generation
The mitochondrial production of superoxides was analyzed by fluorescence using the MitoSOX™ Red reagent. MitoSOX™ Red reagent permeates viable cells where it selectively targets mitochondria. It is rapidly oxidized by superoxide (·O2) but not by other ROS and reactive nitrogen species. To assess superoxide generation by fluorimeter analysis, LAN5 cells (6 × 105 cell/mL) were plated in a 96-well optical bottom white microplate or in a 96-well transparent plate for microscopic fluorescence. After the treatments the cells were washed with PBS and incubated with 5 μM MitoSOX™ reagent working solution for 10 min at 37°C in the dark. At the end of the incubation the cells were washed in PBS and analyzed by fluorimeter (Microplate reader WallacVictor 2 1420 Multilabel Counter, PerkinElmer, Inc.) and fluorescence microscopy (Zeiss Axio Scope 2 microscope). Ex/Em: 510/580 nm.
Statistical analysis
Values obtained for the cellular analysis were reported as the mean of, at least, three independent experiments ± standard deviation (SD). Results were compared using one-way analysis of variance with pairwise comparisons among treatments made using Tukey’s HSD test. The analyses were performed using the OriginPro 8.1 statistical program (OriginLab Corp., Northampton, MA, USA), and the level of significance was set to P < 0.05.
Results and discussion
Biophenol total amount and antioxidant ability in EVOO and WW
A major environmental concern in the Mediterranean countries is the disposal and/or treatment of the large quantities of olive mill WW produced during olive oil processing. WW constitutes a serious problem with severe negative impact on soil and water quality, and thus on agriculture, environment and public health, due to the presence of compounds with biostatic activity, such as phenolic compounds (Rusan, Albalasmeh, & Malkawi, Citation2016). The recovery of such bioactive compounds with high antioxidant activity, usable for pharmacological and beneficial applications, would allow for the reuse of this by-product (Giuffrè, Sicari, Piscopo, & Louadj, Citation2012).
We analyzed the total biophenol content in four WWs (WW1, WW2, WW3 and WW4) and in the related Sicilian EVOO (EVOO1, EVOO2, EVOO3 and EVOO4). EVOO1 and WW1 samples came from Biancolilla cultivar; EVOO2, WW2, EVOO3 and WW3 from Cerasuola cultivar, and EVOO4 and WW4 from Nocellara del Belice cultivar. Samples exhibited a total phenol concentration dependent on the cultivar type. The difference observed in the two Cerasuola samples could be due to a different olive ripening degree (). Significant decrease of total phenol amount has indeed been found with the fruit maturity progress (Abdallah, Marzocco, Adesso, Zarrouk, & Guerfel, Citation2018; Bouaziz, Jemai, Khabou, & Sayadi, Citation2010). also shows that WW total biophenol values were from 10 to 15 times higher than the ones measured in the respective oils. In the first steps of the oil extraction process, when a multiphasic system called olive paste is obtained (Ryan & Robards, Citation1998), thermodynamic determines the fate of the phenolic molecules: their presence depends on their affinity towards solid, oil or water phases. Phenolic compounds are partially present in the lipidic phase during oil production but the main fraction is transferred to the WW due to their higher affinity for the aqueous medium (Mulinacci et al., Citation2001).
Table 3. Comparison between the total biophenol content in EVOO and in the corresponding WW.
Tabla 3. Comparación entre el contenido biofenólico total en el AOVE y en la correspondiente WW. Los valores de concentración se expresan en mg/kg.
In , the chromatographic profile of one of the WW samples (WW3) is reported. The peak of the HT dominates the HPLC chromatogram reaching a very high absorbance value if compared with other biophenol molecules at 280 nm. In addition, other three biophenols are most represented and they were identified as TY, and the CA and CU acid by using standard solutions. Their concentrations measured in WW3 sample are reported in the inset of . A similar chromatogram was obtained for the other WW samples (data not shown).
Figure 1. Chromatographic profile of the WW3 sample. The peaks of the hydroxytyrosol (1), tyrosol (2), caffeic (3) and p-coumaric (4) acids are indicated. Wavelength 280 nm. In the inset, the concentrations of the same compounds are reported. Data are expressed as mg/g.
Figura 1. Perfil cromatográfico de la muestra WW3. Se indican los picos del hidroxitirosol (1), tirosol (2), y ácidos cafeico (3) y p-cumárico (4). Longitud de onda: 280 nm. En el recuadro se indican las concentraciones de los mismos compuestos. Los datos se expresan como mg/g.
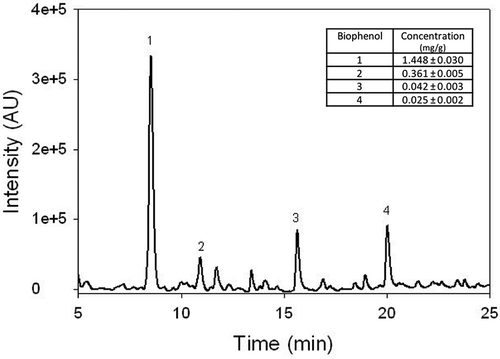
In order to test the antioxidant ability of the WW total phenolic content, the ORAC assay was performed (). The ORAC values are reported in the inset of . It can be observed that the ORAC value is strictly correlated with the total biophenol content. The test has been used to determine the antioxidant power of pure compounds (Davalos, Gomez-Cordoves, & Bartolomé, Citation2004) and food such as fruit juices, beverages and olive oils (Davalos et al., Citation2004; Ninfali et al., Citation2002; Wang, Cao, & Prior, Citation1996). Furthermore, the method has also been tested to reveal the increase of antioxidants in human plasma after intake of antioxidant-rich foods (Cao & Prior, Citation2000; Ninfali & Aluigi, Citation1998). In the present study, the ORAC test was also performed on solutions in which HT, TY, CA and CU standards were individually present to analyze their antioxidant ability. The ORAC values of each phenolic compound are reported in , in which is also represented the molecular structure of the studied antioxidants. The results show that the scavenging ability of the four molecules against free radicals is as follows: TY ≈ HT > CU > CA.
Table 4. ORAC values of the four antioxidant biophenols.
Tabla 4. Valores ORAC de los cuatro biofenoles antioxidantes. Los datos se expresan como μmol de equivalentes de Trolox (TE)/g de solución.
Figure 2. Fluorescence decay curves obtained by ORAC test of four wastewaters obtained during EVOO production. Blank (empty triangles), Trolox (empty circles), WW1 (dot–dot dashed line), WW2 (solid line), WW3 (dashed line) and WW4 (dotted line). In the inset are reported the ORAC value expressed as μmol of Trolox Equivalents (TE)/g of WW.
Figura 2. Curvas de pérdida de fluorescencia obtenidas mediante la evaluación ORAC de cuatro aguas residuales derivadas de la producción de AOVE. Vacío (triángulos vacíos), Trolox (círculos vacíos), WW1 (línea de punto-punto-raya), WW2 (línea sólida), WW3 (línea discontinua) y WW4 (línea punteada). En el recuadro se indica el valor ORAC expresado como μmol de equivalentes de Trolox (TE)/g de WW.
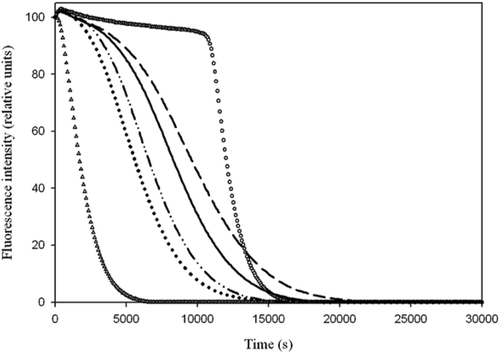
Phenolic antioxidants are not cytotoxic
Since HT, TY, CA and CU constitute a large amount of the biophenolic fraction extracted from the WW and, even more, for their very similar chemical structure, the corresponding standard molecules were used to measure their effect on LAN5 cell line viability through the dose-dependent experiments. HT, TY, CA or CU phenolic molecules were tested in the range 12.5–100 μM for a period of 24 h on LAN5 neuroblastoma cells. Cell viability was measured by MTS assay. No significant cytotoxic effect was revealed for all the tested phenolic antioxidants at concentrations up to 50 μM ()), while the administration of the highest concentration presented a decrease of the cell viability in HT- and CU-treated LAN5 cells. Microscopic inspections confirmed that no morphological changes occurred up to 50 μM ()). The data indicated that the analyzed substances did not modify the cellular physiological status.
Figure 3. (a) Viability tests on LAN5 neuroblastoma cell line performed in the range 12.5–100 μM after 24-h incubation with HT (circles and solid line), TY (squares and solid line), CA (diamonds and dashed line) and CU (triangles and dashed line). The data are shown as means ± SD. P < 0.05 vs. untreated cells. (b) Microscopic inspection of the untreated cells (Control) and after individual addition of the four antioxidants, HT, TY, CA and CU at the concentration of 50 μM kept in the culture medium for 24 h.
Figura 3. (a) Pruebas de viabilidad de una línea celular del neuroblastoma LAN5 realizadas en el rango de 12.5 – 100 μM tras una incubación de 24 horas con HT (círculos y línea sólida), TY (cuadros y línea sólida, CA (rombos y línea discontinua) y CU (triángulos y línea punteada). Los datos se expresan como medias ± DE. P < 0.05 vs. células sin tratamiento. (b) Inspección microscópica de las células sin tratamiento (control) y después de la adición individual de los cuatro antioxidantes, HT, TY, CA y CU, a una concentración de 50 μM mantenida en el medio de cultivo durante 24 horas.
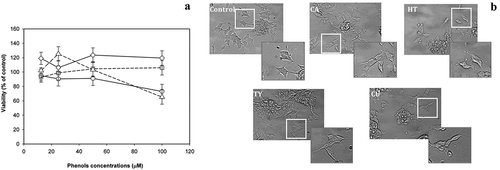
Phenolic antioxidants play a neuroprotective role
Phenolic compounds possess ideal structural chemistry for free radical-scavenging and metal-chelating activities, all properties that could counteract and reduce the risk of age-dependent neurodegeneration including AD. Extracellular deposits of Abeta, a small fragment originated by the enzymatic cleavage of the large transmembrane APP, are hypothesized to be the fundamental cause of this devastating disease (Hardy & Selkoe, Citation2002). In particular, the early Abeta aggregates, namely soluble oligomers, are the major cause of neuronal damage in AD (Busche & Konnerth, Citation2016; Di Carlo, Citation2010) and many experimental data have proved that oxidative stress plays a key role in cell damage after exposure to the early aggregates (Butterfield, Swomley, & Sultana, Citation2013; Di Carlo, Giacomazza, Picone, Nuzzo, & San Biagio, Citation2012; Viola & Klein, Citation2015).
To assess the neuroprotective role of the analyzed WW biophenol compounds, LAN5 cells were treated for 24 h with Abeta oligomers (Carrotta et al., Citation2006) alone or in the presence of HT, TY, CA and CU at two different concentrations (25 and 50 μM), selected on the basis of the viability test results shown in , and compared with untreated cells. The effects of the four antioxidants against toxicity induced by Abeta oligomers are shown in . It can be observed that CU did not show any significant effect in protecting cells from Abeta damages. Viability test and microscopic observation indicated that the treatment with HT or TY was able to reduce the injury caused by Abeta oligomer addition while after addition of CA an almost complete protection was observed.
Figure 4. Viability tests (a–d) performed on LAN5 neuroblastoma cells after addition of 40 μM Abeta oligomers alone or with 25 or 50 μM of CA (a), HT (b), TY (c) and CU (d). Microscopic observations (e) of the cell morphology for control, Abeta treatment (40 μM) and addition of Abeta (40 μM) and CA, HT, TY or CU at the higher concentration tested (50 μM). The treatments were prolonged for 24 h. The data are shown as means ± SD. **P < 0.02; *P < 0.05.
Figura 4. Pruebas de viabilidad (a-d) realizadas en las células neuroblastomas LAN5 después de la adición de 40 μM oligómeros Abeta, solos o con 25 o 50 μM de CA (a), HT (b), TY (c) y CU (d). Observaciones microscópicas (e) de la morfología celular del control, el tratamiento Abeta (40 μM) y la adición de Abeta (40 μM) y CA, HT, TY o CU a la concentración más alta probada (50 μM). Los tratamientos se prolongaron durante 24 horas. Los datos se expresan como medias ± DE. **P < 0.02; *P < 0.05.
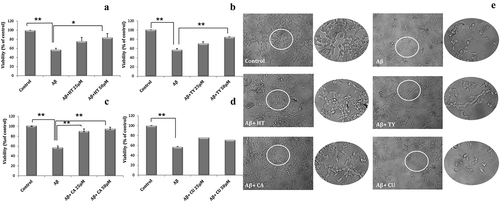
On the basis of the obtained results, only HT, TY and CA were used to investigate their protective role against Abeta-induced oxidative stress. LAN5 cells were treated with Abeta oligomers, alone or in the presence of HT, TY and CA and submitted to DCFH-DA assay. Results shown in indicated that the levels of ROS observed in the presence of Abeta oligomers were reduced by the treatment with HT, TY and CA. Furthermore, after the same treatment LAN5 cells were submitted to MitoSOX assay that reveals exclusively mitochondrial superoxide formation. A significant increase of fluorescence in LAN5 cells treated with Abeta oligomers was observed; instead a reduced fluorescence was observed in the presence of HT or TY and especially in the presence of CA. These results indicated that the biophenolic compounds were able to reduce ROS and particularly superoxides, thus playing a neuroprotective role. This result seems in contrast with data found by ORAC experiments, in which the value of CA was lower than other biophenols. It is noteworthy that ORAC experiments do not account for any biological activity but rather for the mere chemical ability to counteract the free-radical compounds. Biological systems are considerably more complex than simple chemical mixture, and antioxidants may operate via multiple mechanisms. Oxidative stress and mitochondrial dysfunction are believed to be crucial for the onset and progression of neurodegeneration observed in elderly as well as demented brain (Ames, Shigenaga, & Hagen, Citation1993; Angelova & Abramov, Citation2018; Di Carlo, Giacomazza, & San Biagio, Citation2012). Modification of cellular functions due to oxidative damage, coupled with depletion of antioxidant defense, is, indeed, considered a hallmark in the onset and progression of many pathological disorders. This suggests that biological activities could involve different molecular mechanisms and activation of some intracellular signaling events. It has been demonstrated that the increase in the nuclear translocation of NF-κB from the cytoplasma to the nucleus after Abeta exposure was attenuated in the presence of HT or TY (Saint-Laurent-Thibault, Arseneault, Longprè, & Ramassamy, Citation2011). NF-κB is a transcription factor widely expressed in neuronal cells and involved in activation of APP and presenilin genes (Chami et al., Citation2012; Picone et al., Citation2015). NF-κB is stimulated by oxidative stress, and we may hypothesize that biophenols restore cellular homeostasis by inhibiting its activation.
Figure 5. Oxidative stress assays (a–d) performed on LAN5 neuroblastoma cells after addition of 40 μM Abeta alone or in presence of CA, HT and TY at 50 μM. DCFH-DA assay (a, b) and MitoSOX assay (c, d). The data are shown as means ± SD. **P < 0.02; *P < 0.05.
Figura 5. Ensayos de estrés oxidativo (a-d) realizados en células de neuroblastoma LAN5 tras la adición de 40 μM de Abeta sola o en presencia de CA, HT y TY a 50 μM. Ensayos DCFH-DA (a-b) y ensayo MitoSOX (c-d). Los datos de se expresan como medias ± DE. **P < 0.02; *P < 0.05.
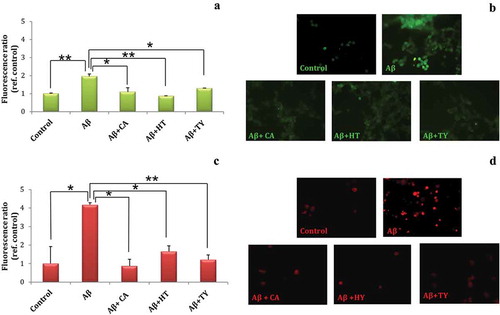
Conclusions
Our data indicate that olive oil WW contains total biophenolic concentration from 10 to 15 times higher than the one present in the corresponding EVOO. Among the four biophenols detected in the WW biophenol extracts, HT is in far greater concentration than TY, and CA and CU. Only HT, TY and CA showed a neuroprotective effect against Abeta toxicity, reducing both cellular ROS production and mitochondrial superoxide generation induced by the presence of Abeta oligomers in LAN5 cell line. In particular, CA showed a more significant protective effect on the cell viability following co-incubation with Abeta when compared to the other biophenols investigated. The discrepancy between ORAC assay results for each biophenol and biological tests can arise from the very complex nature of living cells in which different routes can be run.
Olive mill WW is therefore a natural source of useful substances that extracted and purified could be used in food and pharmaceutical industries. Moreover, its recycling would help in reducing its negative impact on the environment.
Our results confirm once more the beneficial effects of the Mediterranean diet, a feeding regime with high antioxidant content.
Acknowledgment
The authors thank Mr Fulvio Ferrante for his valuable technical help.
Disclosure statement
No potential conflict of interest was reported by the authors.
Additional information
Funding
References
- Abdallah, M., Marzocco, S., Adesso, S., Zarrouk, M., & Guerfel, M. (2018). Olive oil polyphenols extracts inhibit inflammatory markers in J774A.1 murine macrophages and scavenge free radicals. Acta Histochemica, 120(1), 1–10.
- Aganchich, B. ., El Antari, A., Wahbi, S., Tahi, H., Wakrim, R., & Serraj, R. (2008). Fruit and oil quality of mature olive trees under partial rootzone drying in field conditions. Grasas Y Aceites, 59, 225–233. ISSN: 0017-3495.
- Ames, B. N., Shigenaga, M. K., & Hagen, T. M. (1993). Oxidants, antioxidants and the degenerative diseases of aging. Proceedings of the National Academy of Sciences, 90(17), 7915–7922.
- Angelova, P. R., & Abramov, A. Y. (2018). Role of mitochondrial ROS in the brain: From physiology to neurodegeneration. FEBS Letters, 592, 692–702.
- Balasundram, N., Sundram, K., & Samman, S. (2006). Phenolic compounds in plants and agri-industrialby-products: Antioxidant activity, occurrence, and potential uses. Food Chemistry, 99(1), 191–203.
- Ballard, T. S., Mallikarjunan, P., Zhou, K., & O’Keefe, S. F. (2009). Optimizing the extraction of phenolic antioxidants from peanut skins using response surface methodology. Journal of Agricultural and Food Chemistry, 57(8), 3064–3072.
- Ben Sassi, A., Boularbah, A., Jaouad, A., Walker, G., & Boussaid, A. (2006). A comparison of olive oil mill wastewaters (OMW) from three different processes in Morocco. Process Biochemistry, 41(1), 74–78.
- Blasiak, J., Glowacki, S., Kauppinen, A., & Kaarniranta, K. (2013). Mitochondrial and nuclear DNA damage and repair in age-related macular degeneration. International Journal of Molecular Sciences, 14(2), 2996–3010.
- Bouaziz, M., Jemai, H., Khabou, W., & Sayadi, S. (2010). Oil content, phenolic profiling and antioxidant potential of Tunisian olive drupes. Journal of the Science of Food and Agriculture, 90(10), 1750–1758.
- Busche, M. A., & Konnerth, A. (2016). Impairments of neural circuit function in Alzheimer’s disease. Philosophical Transactions of the Royal Society B, Biological Sciences, 371, 1700.
- Butterfield, D. A., Swomley, A. M., & Sultana, R. (2013). Amyloid β-peptide (1-42)-induced oxidative stress in Alzheimer disease: Importance in disease pathogenesis and progression. Antioxidants & Redox Signaling, 19(8), 823–835.
- Cao, G., & Prior, R. L. (2000). Postprandial increases in serum antioxidant capacity in older women. Journal of Applied Physiology, 89(3), 877–883.
- Capasso, R., Cristinzio, G., Evidente, A., & Scognamiglio, F. (1992). Isolation, spectroscopy and selective phytotoxic effects of polyphenols from vegetable waste waters. Phytochemistry, 31(12), 4125–4128.
- Carrotta, R., Di Carlo, M., Manno, M., Montana, G., Picone, P., Romancino, D., & San Biagio, P. L. (2006). Toxicity of recombinant β-amyloid prefibrillar oligomers on the morphogenesis of the sea urchin Paracentrotus lividus. FASEB Journal, 20(11), 1916–1917.
- Casamenti, F., & Stefani, M. (2017). Olive polyphenols: New promising agents to combat aging-associated neurodegeneration. Expert Review of Neurotherapeutics, 17(4), 345–358.
- Chami, L., Buggia-Prevot, V., Duplan, E., Del Prete, D., Chami, M., Peyron, J. F., & Checler, F. (2012). Nuclear factor-kappa B regulates beta APP and beta- and gamma-secretases differently at physiological and supraphysiological Abeta concentrations. Journal of Biological Chemistry, 287(29), 24573–24584.
- Davalos, A., Gomez-Cordoves, C., & Bartolomé, B. (2004). Extending applicability of the oxygen radical absorbance capacity (ORAC-Fluorescein) assay. Journal of Agricultural and Food Chemistry, 52(1), 48–54.
- Di Carlo, M. (2010). Beta amyloid peptide: From different aggregation forms to the activation of different biochemical pathways. European Biophysics Journal, 39, 877–888.
- Di Carlo, M., Giacomazza, D., Picone, P., Nuzzo, D., & San Biagio, P. L. (2012). Are oxidative stress and mitochondrial dysfunction the key players in the neurodegenerative diseases? Free Radical Research, 46(11), 1327–1338.
- Di Carlo, M., Giacomazza, D., & San Biagio, P. L. (2012). Alzheimer’s disease: Biological aspects, therapeutic perspectives and diagnostic tools. Journal of Physics: Condensed Matter, 24(24), 244102.
- El Riachy, M., Priego-Capote, F., Leon, L., Rallo, L., & Luque de Castro, M. D. (2011). Hydrophilic antioxidants of virgin olive oil. Part 1: Hydrophyilic phenols: A key factor for virgin olive oil quality. European Journal of Lipid Science and Technology, 113(6), 678–691.
- El-Abassi, A., Kiai, H., & Hafidi, A. (2012). Phenolic profile and antioxidant activities of olive mill wastewater. Food Chemistry, 132(1), 406–412.
- Galli, C. (2006). Bioactive minor compounds of extra-virgin olive oil: The ibuprofen-like activity of oleocanthal. European Journal of Lipid Science and Technology, 108(2), 95–96.
- Garcia-Martinez, O., Mazzaglia, G., Sanchez-Ortiz, A., Ocana-Peinado, F. M., & Rivas, A. (2014). Phenolic content of Sicilian virgin olive oils and their effect on MG-63 human osteoblastic cell proliferation. Grasas Y Aceites, 65(3). doi:10.3989/gya.0111141
- Giuffrè, A. M. (2017). Biometric evaluation of twelve olive cultivars under rainfed conditions in the region of Calabria, South Italy. Emirates Journal of Food and Agriculture, 29(9), 696–709.
- Giuffrè, A. M., Piscopo, A., Sicari, V., & Poiana, M. (2010). The effects of harvesting on phenolic compounds and fatty acids content in virgin oil (cv. Roggianella). Rivista Italiana Delle Sostanze Grasse, 87, 14–23.
- Giuffrè, A. M., Sicari, V., Piscopo, A., & Louadj, L. (2012). Antioxidant activity of olive oil mill wastewater obtained from different thermal treatments. Grasas Y Aceites, 63(2), 209–213.
- Goldsmith, C. D., Stathopoulos, C. E., Golding, J. B., & Roach, P. D. (2014). Fate of the phenolic compounds during olive oil production with the traditional press method. International Food Research Journal, 21(1), 101–109.
- Gómez-Rico, A., Salvador, M. D., Moriana, A., Pérez, D., Olmedilla, N., Ribas, F., & Fregapane, G. (2007). Influence of different irrigation strategies in a traditional Cornicabra cv. Olive orchard on virgin olive oil composition and quality. Food Chemistry, 100(2), 568–578.
- Gould, G. W. (1995). Biodeterioration of foods and an overview of preservation in the food and dairy industries. International Biodeterioration & Biodegradation, 36, 267–277.
- Hardy, J., & Selkoe, D. J. (2002). The amyloid hypothesis of Alzheimer’s disease: Progress and problems on the road to therapeutics. Science, 297(5580), 353–356.
- Hrncirik, K., & Frische, S. (2004). Comparability and reliability of different techniques for the determination of phenolic compounds in virgin olive oil. European Journal of Lipid Science and Technology, 106(8), 540–549.
- Kılıçkan, A., & Güner, M. (2008). Physical properties and mechanical behavior of olive fruits (Olea europaea L.) under compression loading. Journal of Food Engineering, 87, 222–228.
- Kiritsakis, A. K. (1998). Flavor component of olive oil – A review. Journal of the American Oil Chemists’ Society, 75(6), 673–681.
- Kulawik, P., Ozogul, F., Glew, R., & Özogul, Y. (2013). Significance of antioxidants for seafood safety and human health. Journal of Agricultural and Food Chemistry, 61(3), 475–491.
- Mulinacci, N., Romani, A., Galardi, C., Pinelli, P., Giaccherini, C., & Vincieri, F. F. (2001). Polyphenolic content in olive oil waste waters and related olive samples. Journal of Agricultural and Food Chemistry, 49(8), 3509–3514.
- Ninfali, P., & Aluigi, G. (1998). Variability of Oxygen Radical Absorbance Capacity (ORAC) in different animal species. Free Radical Research, 29(5), 399–408.
- Ninfali, P., Bacchiocca, M., Biagiotti, E., Servili, M., & Montedoro, G. (2002). Validation of the Oxygen Radical Absorbance Capacity (ORAC) parameter as a new index of quality and stability of virgin olive oil. Journal of the American Oil Chemists’ Society, 79(10), 977–982.
- Obied, H. K, Bedgood, D, Mailer, R, Prenzler, P. D, & Robards, K. (2008). Impact of cultivar, harvesting time, and seasonal variation on the content of biophenols in olive mill waste. Journal Of Agricultural and Food Chemistry, 56(19), 8851-8. doi: 10.1021/jf801802k
- Panusa, A., Zuorro, A., Lavecchia, R., Marrosu, G., & Petrucci, R. (2013). Recovery of natural antioxidants from spent coffee grounds. Journal of Agricultural and Food Chemistry, 61(17), 4162–4168.
- Picone, P., Nuzzo, D., Caruana, L., Messina, E., Barera, A., Vasto, S., & Di Carlo, M. (2015). Metformin increases APP expression and processing via oxidative stress, mitochondrial dysfunction and NF-κB activation: Use of insulin to attenuate metformin’s effect. Biochimica Et Biophysica Acta, 1853(5), 1046–1059.
- Reboredo-Rodriguez, P., Valli, E., Bendini, A., Di Lecce, G., Simal-Gandara, J., & Gallina Toschi, T. (2016). A widely used spectrophotometric assay to quantify olive oil biophenols according to the health claim (EU Reg. 432/2012). European Journal of Lipid Science and Technology, 118(10), 1593–1599.
- Rusan, M. J. M., Albalasmeh, A. A., & Malkawi, H. I. (2016). Treated olive mill wastewater effects on soil properties and plant growth. Water Air Soil Pollut, 227, 135.
- Ryan, D., & Robards, K. (1998). Phenolic compounds in olives. Analyst, 123, 31R–44R.
- Saint-Laurent-Thibault, C., Arseneault, M., Longprè, F., & Ramassamy, C. (2011). Tyrosol and hydroxytyrosol, two main components of olive oil, protect N2a cells against amyloid-β-induced toxicity. Involvement of the NF-κB signaling. Current Alzheimer Research, 8(5), 543–551.
- Serrano-Pozo, A., Frosch, M. P., Masliah, E., & Hyman, B. T. (2011). Neuropathological alterations in Alzheimer disease. Cold Spring Harbor Perspectives in Medicine, 1(1), a006189.
- Servili, M., Taticchi, A., Esposto, S., Urbani, S., Selvaggini, R., & Montedoro, G. (2007). Effect of olive stoning on the volatile and phenolic composition of virgin olive oil. Journal of Agricultural and Food Chemistry, 55(17), 7028–7035.
- Sgarbossa, A., Giacomazza, D., & Di Carlo, M. (2015). Ferulic acid: A hope for Alzheimer’s disease therapy from plants. Nutrients, 7(7), 5764–5782.
- Sharma, P., Jha, A. B., Dubey, R. M., & Pessarakli, M. (2012). Reactive oxygen species, oxidative damage, and antioxidative defense mechanism in plants under stressful conditions. Journal of Botany, 2012, 1–26. 26. Art. ID 217037.
- Tsagaraki, E, Lazarides, H, & Petrotos, K. (2007). Olive mill wastewater. In (Eds.), Utilisation of by-products and treatment of waste in the food industry (pp. 133-157). New York, NY: Springer.
- Viola, K. L., & Klein, W. L. (2015). Amyloid β oligomers in Alzheimer’s disease pathogenesis, treatment, and diagnosis. Acta Neuropathologica, 129(2), 183–206.
- Visioli, F., Romani, A., Mulinacci, N., Zarini, S., Conte, D., Vincieri, F. F., & Galli, C. (1999). Antioxidant and other biological activities of olive mill waste waters. Journal of Agricultural and Food Chemistry, 47(8), 3397–3401.
- Wang, H., Cao, G., & Prior, R. L. (1996). Total antioxidant capacity of fruits. Journal of Agricultural and Food Chemistry, 44(3), 701–705.
- Wilqueta, V., & De Strooper, B. (2004). Amyloid-beta precursor protein processing in neurodegeneration. Current Opinion in Neurobiology, 14(5), 582–588.