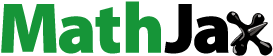
ABSTRACT
This study examined the potential of incorporating stearic acid in starch-based edible coating formulations in order to increase the quality and shelf-life of fresh-cut papaya (“Sekaki” variety). Glycerol was employed as plasticizer and stearic acid was incorporated in proportions of; 0 %, 1%, 2%, and 3% (w/w). Film with 30% glycerol, 1% stearic acid, 5 g of starch, and 5% aloe Vera was chosen because it had low film solubility and water vapour permeability (29.54 ± 0.21, 2.99 ± 0.21), respectively. Furthermore, this film had slightly darker L* value (78.19 ± 1.21) which is an advantage because it will be able to reduce the loss of ascorbic acid (AA) which is sensitive to light and acceptable level of mechanical property The properties of the fresh-cut papayas were analysed during 12 days of storage at 10°C. The results obtained showed that the edible coating (i.e. film with 30% glycerol, 1% stearic acid, 5 g of starch, and 5% aloe Vera) significantly improved the physiochemical properties of fresh-cut papaya in terms of reduced weight loss, better firmness, better colour retention, and a slight decrease in AA content during storage. Furthermore, the microbial analysis showed that, the coating was able to extend the shelf life of fresh-cut papaya up to 12 days at refrigerated conditions.
RESUMEN
El presente estudio examinó el potencial que tiene la incorporación de ácido esteárico en las formulaciones de recubrimientos comestibles a base de almidón para aumentar la calidad y la vida útil de la papaya variedad “Sekaki” recién cortada. Con este objetivo se empleó glicerol como plastificante, incorporándose ácido esteárico en proporciones de 0%, 1%, 2% y 3% (p/p). Se seleccionó una película que contenía 30% de glicerol, 1% de ácido esteárico, 5 g de almidón y 5% de aloe vera porque tiene baja solubilidad y baja permeabilidad al vapor de agua (29.54 ± 0.21, 2.99 ± 0.21, respectivamente). Además, esta película posee un nivel aceptable de propiedades mecánicas y mostró un valor L* ligeramente más oscuro (78.19 ± 1.21), lo que representa una ventaja pues, dado que el ácido ascórbico es sensible a la luz, podrá reducir su pérdida. Las propiedades de las papayas recién cortadas se analizaron durante su almacenamiento por 12 días a 10°C, constatándose que el recubrimiento comestible (es decir, la película con 30% de glicerol, 1% de ácido esteárico, 5 g de almidón y 5% de aloe vera) mejoró significativamente sus propiedades fisicoquímicas en términos de escasa pérdida de peso, mejor firmeza, mejor retención de color y ligera disminución del contenido de ácido ascórbico durante el almacenamiento. Asimismo, el análisis microbiano dio cuenta de que el recubrimiento puede extender la vida útil de la papaya recién cortada hasta 12 días en condiciones de refrigeración.
Introduction
In recent years, the production and consumption of minimally processed fruits have been gaining popularity (Falah, Nadine, & Suryandono, Citation2015). This is because of the fact that consumers are becoming more health conscious and fruits are becoming part of their daily diet. Thus, fruits in the form of fresh-cut are preferred compared to whole fruit due to its convenience, freshness and quality which adds value to it (Qadri, Yousuf, & Srivastava, Citation2015). Generally, fresh-cut fruits are packaged and stored at chilled temperature for consumption (Moreíra, Tomadoni, Martín-Belloso, & Fortuny, Citation2015).
Papaya (Carica papaya L.), is a climacteric tropical fruit which is becoming important internationally both as fresh and processed products (Lasekan & Abbas, Citation2012). Consumption of papaya fruit is rising because it contains important nutrients which provide various health benefits such as vitamin C, polysaccharides, phenolic and carotenoids, which have a wide range of biological activities (John, Yang, Liu, Jiang, & Yang, Citation2018). However, the commercialization of fresh-cut fruits have been restricted due to their short shelf life which is reflected through weight loss, softening of pulp and rapid growth of microbes (Albertini et al., Citation2016). For instance, the metabolic processes that occur during fruit ripening and fruit development causes sensory and compositional changes in fresh-cut papaya (Cáez-Ramírez, Alamilla-Beltrán, & Gutiérrez-López, Citation2018).To overcome this issue, many methods have been adopted in extending the shelf life of fresh-cut fruits. One of these methods is the application of edible coatings. Edible coating has been optimized and explored widely due to its simple and environmental friendly nature. An edible film or coating is referred to as any material used for wrapping foods to extend the shelf-life of the product that may be eaten together with the food with or without further removal (Brasil et al, Citation2012).
Among many polysaccharides, starch-based materials have been widely studied since they are edible, food compatible, low cost, renewable resource, widely available and possess film forming capacity (Gutiérrez, Herniou-Julien, Álvarez, & Alvarez, Citation2018). In this study, Chickpea starch was used because the legume contains high amylose content and this contributed to its film forming capacity. Furthermore, it has been reported that permeability for oxygen in high amylose starch films was found to be virtually zero despite the addition of plasticizers that were known to increase permeability (Lasekan, Laskan, Idowu, & Ojo, Citation1996; Pavlath & Orts Citation2009). In addition, there are very few publications on the use of chickpea starch as edible coating (Bashir & Haripriya, Citation2016). Nonetheless, starches generally have some drawbacks which are; poor mechanical strength and high hydrophilic property (Gutiérrez et al., Citation2018). The drawbacks can be overcome by incorporating glycerol as plasticizer (Gheribi et al., Citation2018). Furthermore, the addition of fatty acid component such as stearic acid can act as a moisture barrier for the film (Thakur et al., Citation2016). Moreover, aloe vera gel, which has antimicrobial property and simple preparation, make it feasible to be use as an edible coating to extend the shelf life of fruit (Benítez, Achaerandio, Pujolà, & Sepulcre, Citation2015; Sui Chin, Han Lyn, & Nur Hanani, Citation2017). Currently, studies have centred on the multicomponent edible coatings (Hassan, Chatha, Hussain, Zia, & Akhtar, Citation2018).
Therefore, the main objective of this study was to develop composite-starch based coating to improve the shelf life and quality of fresh-cut papaya (Carica papaya L.) using appropriate formulation. The specific objectives of this study are (1) to determine suitable cowpea starch-base formulation(s) for coating fresh-cut papaya, (2) To measure the effectiveness of coating in improving the quality of fresh-cut papaya and (3) To determine the shelf life of fresh-cut papaya through microbial analysis.
Materials and methods
Chickpea starch preparation
Chickpea starch was extracted by using the method of Huang et al. (Citation2007) with slight modification. Chickpeas were obtained from Tesco, Shah Alam, Malaysia. The Chickpeas were steeped in distilled water at 4°C for 24 h. The steep water was decanted, and the softened legume was ground in a blender for 3 min at low speed. The ground slurry was sieved and the residue on the sieve was ground again for another 3 min in the blender with distilled water, and sieved again. The starch suspension was collected and allowed to settle overnight at 4°C. The supernatant was drained off, and the upper non-white layer was removed. The starch layer was resuspended in cold 0.2% NaOH and kept at 4°C for 2 h and after 2 h the solution was neutralized with 2 mol/L of HCl to pH 6. This procedure helps to neutralize the starch. Next, the solution was centrifuged at 1500 g at 4°C. The starch layer was suspended in deionized water and centrifuged twice. The starch was recovered by filtration, and dried at 40°C for 72 h.
Preparation of aloe vera gel
Aloe vera (Aloe barbadensis Miller) obtained from a local farm at Gombak, Malaysia was washed thoroughly in distilled water and the aloe Vera rind was removed to expose the flesh. The gel was subsequently removed with a clean spatula and stored below 5°C until further use (Sui Chin et al., Citation2017).
Film preparation
The film preparation was done according to the reported method of Schmidt, Porto, Laurindo, and Menegalli (Citation2013) with slight modification. The films were prepared by casting it from a suspension of 5 g of chickpea starch and 100 g distilled water, with heating to 65± °C. Then, analytical grade glycerol (20% and 30% w/w) were separately added to different concentrations of stearic acid (0%, 1%, 2%, 3% w/w) (Wilmer, Scienfield Expertise, Malaysia) and aloe vera gel (5% w/w). The suspension was added to the chickpea starch slurry and stirred for 5 min to disperse the stearic acid, and was subsequently heated to 80°C and maintained at this temperature for 5 min for gelatinization to occur. The gelatinized suspension was homogenized while still hot in an Ultra-Turrax for 10 min with a stirring frequency of 200 Hz (12,000 rpm). The suspension was vacuum cooled at 750 mmHg to remove the bubbles and excess air which dissolved in the suspension. Around 20 g of the emulsion was then spread on petri plate and dried for 24 h in an oven at 40°C. The films were conditioned at 50 ± 5% RH and at a temperature of 23 ± 2°C for at least 48 h in a dry cabinet prior to testing.
Coating preparation
Mature green papaya “Sekaki” variety of colour index 5 (yellow with trace of green) based on the Federal Agricultural Marketing Authority (FAMA) colour index were obtained from a local fruit wholesaler at Selangor, Malaysia. Fruits of uniform sizes (800–1200 g), shape, maturity and free from any indication of mechanical injury, insect damage/infestation or pathogenic infection were selected for the experiment. Papayas were peeled by using sharp peelers and cut into two equal halves. Seeds were scraped off from the halves. The halves were further processed by preparing uniform cube (2.5 cm) with the help of cube mould. Selected coating formulation was applied by dipping the cut papaya into selected solution and distilled water as control for 10 s at room temperature (23 ± 2°C). After dipping, they were air dried with the aid of a fan for 10 min. Then, they were packed in Polypropylene (PP) trays and transparent polyethylene (PE) wrapping films. Later they were kept in chiller at 4 ± 1°C and 85% RH for 12 days. (Alves, Gonçalves, & Rocha, Citation2017).
Film analysis
Thickness
The film thickness was determined with a digital micrometre (Mitutoyo Absolute, Tester Sangyo Co. Ltd., Japan). The thickness was measured at 10 randomly selected locations on each film and then an average value was calculated (Maryam Adilah, Jamilah, & Nur Hanani, Citation2018).
Water solubility
Water solubility was determined using the gravimetric method as reported by Maryam Adilah et al. (Citation2018). To determine the initial dry mass, the film samples were cut into square strips (2 × 2 cm2) and dried at 100 ± 5°C for 24 h. It was then submerged in 50 mL distilled water for 24 h at 23 ± 2°C. The soaked sample was then re-dried at 100 ± 5°C for 24 h to get the final dry mass. The water solubility of the film was calculated using Equation (1):
Water vapour permeability
Water vapour permeability (WVP) of films was carried out using the method reported by Gennadios, Weller, and Gooding (Citation1994). A permeation cell (acrylic cup) with an internal diameter of 4.4 cm and an external diameter of 8.4 cm and a depth of 3.5 cm was used. The cell contained CaCl2 (RH; 0 %: zero water vapour pressure). The film was placed between the cell and the acrylic ring cover and screwed to the cup allowing a 7 mm gap between the film and the CaCl2 layer. The whole assemblage was placed in a temperature and RH controlled chamber (Ibertest, Espania). A temperature of 25°C and a RH of 70% (approximately 2288 Pa) for 24 h when a stationary water vapour transmission rate was attained. Subsequently, the changes in weight of the cell (in triplicate) were carried out for 6 days. The WVP was calculated using corrected WVP method of Gennadios et al. (Citation1994).
Mechanical properties
Films’ mechanical properties such as tensile strength (TS), and elongation at break (EAB) were examined with an INSTRON 4302 Series IX machine (Instron Co., Canton, MA, USA). The films with lengths of 10 mm and widths of 5 mm were first conditioned at 23 ± 2°C and 50% RH for 48 h before analysis. The film was held between the molars of the grips with 50 mm separation and 50 mm/min cross head speed. A tensile load of 5 kN was employed (Maryam Adilah et al., Citation2018).
Colour properties
Measurement was carried out in a Minolta colorimeter (Minolta CM-508d, Tokyo, Japan) using an opening of 1.5 cm diameter. In order to prevent light trapping effect, the exposed area was made greater relative to the illuminated area. The L, a and b parameters were measured according to the test method (ASTM E1925, Citation1995) from five different points. Colour parameters ranges from L = 0 (black) to L = 100 (White), −a (greenness) to +a (redness), and −b (blueness) to +b (yellowness). The machine was calibrated using a standard white tile. The same method was adopted for monitoring the colour of fresh-cut papaya. Whiteness index (W) was calculated using the Gooch (Citation2011) Equation (2):
Physiochemical analysis of fresh-cut papaya
Weight loss
The weight loss was obtained by taking the difference between the initial weight of the fresh-cut papaya and the one obtained at the end of each storage time. The % weight loss was calculated using Equation (3).
Firmness
The firmness was measured with a texture analyser (TAXT2i, Stable Micro System Ltd, England). A cylindrical probe with the following; pre-test speed of 4 mm s−1, post-test speed of 8 mm s−1, test speed of 2 mm s−1 and penetration depth of 5 mm was used. The results were expressed in Newton (N) (Cortez-Vega, Pizato, De Souza, & Prentice, Citation2014).
Total soluble solid and pH
The measurements of soluble solids and pH were done according to AOAC (Citation2000). In case of total soluble solid, the direct readings were taken using manual refractometer and expressed in brix. The pH was measured using a digital pH meter.
Ascorbic acid (AA) content
AA content was determined according to Malaterre, Stanislas, Douraguia, and Gonthier (Citation2016) with slight modification. Briefly, 5 g of fruit sample were dissolved in 10 mL of metaphosphoric acid/acetic acid solution and 10 mL distilled water. The solution was titrated with 0.01% (v/v) of 2,6-dichloro-phenol-indophenol solution. The final point was considered when the solution had a pink colour for 15 s. AA (Vitamin C) contents was expressed as mg AA equivalent/100 g of fresh weight.
Microbial analysis
Microbiological analysis of fresh-cut papaya were performed by determining the total aerobic mesophilic plate count and yeast and mould (YM) populations based on the methods reported by Koh, Noranizan, Nur Hanani, Karim, and Rosli (Citation2017). Fresh-cut papaya (15 g) was homogenized for 2 min with 0.1% sterile peptone water in a ratio of 1:10 (sample: peptone water, w/v) using a Bag Mixer 400 stomacher lab blender under sterile conditions. Serial dilutions of the homogenates using sterile peptone water were plated onto plate count agar (Merck, Darmstadt, Germany) for total plate count and incubated for 48 h at 37 ± 1°C. Potato dextrose agar (Merck, Darmstadt, Germany) was used for determination of the YM count with incubation at 25 ± 1°C for 5 days. The results were expressed in log cfu g−1.
Statistical analysis
Two-way and One-way analysis of variance (ANOVA) was performed to compare mean values with Tukey’s test was performed using Minitab 16 software.
Results and discussion
Film analysis
Thickness
In terms of thickness, there was no significant difference found between and within the films (P > .05) (). This might be due to the emulsion which gives good coverage and homogeneous films. In addition, the emulsion probably allowed for a better distribution of the components in the polymer matrix, and the reduction of the formation of pores and regions with accumulations of materials (Schmidt et al., Citation2013). Similar result was found by Hashemi, Mousavi Khaneghah, Ghaderi Ghahfarrokhi, and Eş (Citation2017) with Basel seed gum and oil, and with mucilage plasticized with different polyols (Gheribi et al., Citation2018).
Table 1. Thickness, tensile strength, elongation at break, water vapour permeability and water solubility of chickpea-starch based films.
Tabla 1. Grosor, resistencia a la tracción, alargamiento de rotura, permeabilidad al vapor de agua y solubilidad en agua de películas a base de almidón de garbanzos.
Mechanical property
TS and EAB are the main parameters used for mechanical strength of composite films which indicate the maximum tensile stress and their flexibility (Sui Chin et al., Citation2017). In control films (i.e. films with 0% stearic acid) when glycerol concentration was increased from 20% to 30%, the TS decreased (P > .05) from 3.15 to 2.7 Mpa (). The observed decrease in TS was probably due to the fact that the plasticizer formed an hydrogen bond interaction with the starch and thus weakened the interaction of starch molecules (Rompothi, Pradipasena, Tananuwong, Somwangthanaroj, & Janjarasskul, Citation2017). Meanwhile, the EAB was increased when glycerol concentration was increased in control film, from 12.0% to 14.5% (P < .05) (data not shown). When glycerol was incorporated into the starch network, competition for hydrogen bonding occurred. As a result, direct interactions between the starch chains were partly reduced due to hydrogen bond formation with glycerol, which gave the polymer chains more freedom of motion. Thus, the starch film became more flexible as the plasticizer concentration was increased, due to greater possibilities for chain displacement (Bertuzzi, Gottifredi, & Armada, Citation2012). Furthermore, the effectiveness of glycerol was most likely due to its smaller size and lower molecular weight. A smaller molecule of glycerol (molecular weight = 92) could fit between the starch chains, interrupt hydrogen bonds and influence film mechanical properties more readily. Similar result was found by Yunos and Rahman (Citation2011)
Meanwhile, when stearic acid (SA) was added in increasing manner from 1% to 2%, the TS increased significantly (P < .05) as compared to the control. Increasing the SA to 3% resulted in a further increase (P < .05) in TS. For example, when 30% glycerol and 3% SA were incorporated into the starch network, a film of the highest TS was produced (). This is due to the fact that SA exhibited more complex formation than unsaturated fatty acid. The formation of the amylose-lipid complex may have given greater strength to the composite films during the film forming process, which led to an increase in the network resistance (Mohammad Amini, Razavi, & Zahedi, Citation2015).
On the other hand, when SA was added in increasing proportion from 1% to 3%, the EAB decreased significantly (P < .05) for both 20% and 30% glycerol films. In addition, the films became more rigid as the concentration of SA was increased (Fabra, Pérez-Masiá, Talens, & Chiralt, Citation2011). Similar observation was found by Schmidt et al. (Citation2013) where, the EAB decreases when SA was added in starch film. Furthermore, Jiménez, Fabra, Talens, and Chiralt (Citation2012) opined that lipids introduce discontinuities in the polymer matrix which contribute to a reduction in the polymer cohesion forces and, thus, the film’s resistance to break. The authors also suggested that, size and distribution of solid particles in the polymer matrix also affected its mechanical properties. If fatty acid is better dispersed as nanoparticles instead of micro/macro particles, they could act as a lubricant for the polymer chain slipping during the film stretching, thus inducing an increase in stretch ability and a slight change, in TS and elastic modulus.
3.3. Water vapour permeability
WVP is an important characteristic of packaging material. Ideally, interactions between the wrapped product and the environment should be minimal to prolong the shelf life of the products. WVP is defined as the transmission rate of water to the driving force of vapour pressure.
In control films, when glycerol concentration was increased, it increased the permeability of water (). Increasing permeability of films has been attributed to interference of plasticizer in intermolecular bonds of polymer chains, thus increasing inter-chain spacing and molecular mobility Mohammad Amini et al. (Citation2015). As mentioned earlier, glycerol is a relatively small hydrophilic molecule, which can be entrapped between adjacent polymeric chains, decreasing intermolecular attractions, increasing molecular mobility, and facilitating migration of water vapour (Souza et al., Citation2012). Similar results were obtained by Isotton, Bernardo, Baldasso, Rosa, and Zeni (Citation2015) in etherified starch films containing glycerol as plasticizer. Moreover, Santacruz et al. (Citation2015) also showed that, starch and chitosan composite film has high WVP due to glycerol’s small molecular size.
However, the addition of stearic acid resulted in films with lower WVP compared to control films (). When 1% SA was added, the WVP decreased (P < .05) significantly compared to the control and a further increase in SA reduced WVP (P > .05). The reduction in WVP can be due to the hydrophilic–hydrophobic ratio in the film structure. Fatty acid in film matrix imparts the hydrophobic character on the film and these results in morphological alterations in the film structure. At low fatty acid concentration water molecules permeate the hydrophilic matrix through a straight path. However, permeation becomes difficult at high fatty acid concentration with dominated hydrophobicity of film (Thakur et al., Citation2016). This behaviour can be explained by the higher hydrophobicity and lower mobility of long chain fatty acids of stearic acid compared to short chain fatty acids (Seyedi, Koocheki, Mohebbi, & Zahedi, Citation2015). Other than that, Jiménez et al. (Citation2012) also found that the addition of SA in starch film reduced WVP because stearic acid were homogeneously distributed in the film matrix and they are solid at the control temperature, which contributed to a reduction in the water transfer rate. However, reduction of moisture and gas transfer from fruit to the environment is desirable, extremely impermeable coatings may induce anaerobic conditions that can lead to a decrease in aroma production (Mattheis & Fellman, Citation2000). Other authors such as Chiumarelli and Hubinger (Citation2014) on cassava starch, Liu, Sun, Hou, and Dong (Citation2016) on potato starch, Fernández, De Apodaca, Cebrián, Villarán, and Maté (Citation2007) on whey protein isolate (WPI) film, Thakur et al. (Citation2016) on starch-I carrageenan have all reported that addition of stearic acid reduced the WVP in films.
Water solubility
Film solubility (FS) is an important property of edible films used in food protection. This is notably important when the film is in contact with water during the processing of the coated food. Generally, higher solubility would indicate lower water resistance of films (Thakur et al., Citation2016). The solubility of film in the control sample increased significantly (P < .05) as the glycerol concentration was increased (). This is mainly due to its hydrophilic character. When glycerol is added, it interacts with the film matrix by increasing the space between the chains, facilitating the water diffusion and, consequently, increased film solubility (Chiumarelli & Hubinger, Citation2014).
Meanwhile, when SA was added the solubility decreased as compared with the control films. When 1% of SA was added, the solubility decreased significantly (P < .05) in both 20% and 30% added glycerol films. This might be attributed to the better dispersion of stearic acid in the starch matrix (Oyeyinka, Singh, & Amonsou, Citation2017). Further increase in SA concentration only resulted in a slight decrease in water solubility. In addition, the films were immersed in water for 24 h they were able to retain structural integrity. Furthermore, according to Adjouman et al. (Citation2017), the reduction in WVP is due to improved barrier effectiveness as the non-polar part of the molecule increases, reducing the solubility and, therefore, the transfer of water molecules through the film. Other than that, lower values for solubility in water would be the result of greater interaction between network components (Schmidt et al., Citation2013). Similar result was reported by Seyedi et al. (Citation2015), Zahedi et al. (Citation2010) in starch and protein incorporated with stearic acid films.
Colour properties of films
The colour of the packaging films has crucial importance in improving the product appearance, and thus they directly influence the consumer acceptability. Generally, the desirable characteristics in edible film packaging and coating are high transparency and lightness (Zhang, Zhao, & Shi, Citation2016). However, darker films are more appropriate for packaging foods that are sensitive to light (Maryam Adilah et al., Citation2018).
Three parameters that were taken into consideration were lightness (L*), green-red (a*) and blue-yellow (b*) colour, were used as the index to show the colour differences between the samples and control. The results showed that for control (i.e. 20% or 30% glycerol without stearic acid films) there was a significant difference (P < .05) between the whiteness index of the films (). When glycerol concentration was increased, the whiteness index (or L value) increased significantly (P < .05). However, there was no significant difference obtained in the “a” and “b” values, respectively. This is probably due to the colourless nature of glycerol and the enhanced reflection of light on film surface (Ramos et al., Citation2013). A similar observation was reported by Farahnaky, Saberi, and Majzoobi (Citation2013).
Table 2. Effect of stearic acid and glycerol addition on the colour parameters of the chickpea-starch based films.
Tabla 2. Efecto de la adición de ácido esteárico y glicerol en los parámetros de color de las películas basadas en almidón de garbanzos.
The combination of stearic acid and glycerol in the films produced significant differences (P < .05) in the colour properties of the films. For instance, when stearic acid concentration of 1% was used, the whiteness index decreased significantly (P < .05) compared to control (). However, when steric acid concentration was further increased from 1% to 3%, the whiteness index increased significantly (P < .05). This may be attributed to the physical state of the fatty acid which is solid at room temperature. Moreover, glycerol may have reduced the density of the starch–starch interactions by enhancing polymer chain movements, thereby increasing the free volume and lowering the Tg, which allows more light to pass through the film matrix (Liaotrakoon & Raviyan, Citation2018). Furthermore, Jiménez, Fabra, Talens, and Chiralt (Citation2010) found that the decrease in the lipid layer size of emulsion increases the opportunity for light transmission which promotes film transparency. A similar result was reported by Zahedi et al. (Citation2010) in which they observed significant increases in film opacity when fatty acid was increased in pistachio globulin protein. In terms of a values and b values there were no significant differences (P > .05).
Physiochemical properties of fresh-cut papaya
From the film analysis, it was concluded that WVP and water solubility were the most important characteristics. Subsequently, films made using 30% glycerol and 1% stearic acid with 5 g of starch and 5% of aloe vera gel were selected for the coating experiment. Furthermore, since papaya has high content of AA which is light sensitive, this formulation which is slightly darker was more beneficial. Moreover, the mechanical properties are also satisfactory.
Weight loss
One of the main objectives of coating application is to reduced fruit weight loss during storage (Alves et al., Citation2017). Fresh plant tissues tend to lose moisture and thus lose weight when stored at fixed temperatures and in an environment with relatively low water vapour pressure due to transpiration rate (Hashemi et al., Citation2017; Liplap, Vigneault, Toivonen, Charles, & Raghavan, Citation2013). Moreover cutting of fruit causes increase in wound-induced ethylene production, water activity and surface area per unit volume leading to water loss (Narsaiah et al., Citation2015). In the current study, there was a progress weight loss (P < .05) among uncoated papaya fruits stored from day 0 to day 12 (). On the other hand the coated fruits had no significant weight loss (P > .05) during storage. This result is not unexpected since the edible coating would have formed an extra layer around the fruits which in turn helps to coat the stomata. This would therefore result in the reduction of transpiration rate and the subsequent reduction in weight loss. Furthermore, the coating creates a water loss barrier, causing high relative humidity in the surrounding atmosphere of the sliced fruits and thus reducing the gradient to the exterior (Hashemi et al., Citation2017). Guerreiro, Gago, Faleiro, Miguel, and Antunes (Citation2017) also reported that, fresh-cut fruit which is coated with lipid has less weight loss due to lipid’s hydrophobic character.
Table 3. Physicochemical properties of film-coated and uncoated fresh-cut papaya.
Tabla 3. Propiedades fisicoquímicas de la papaya recién cortada recubierta con película y sin revestir.
Acidity and total soluble solid
It can be seen that, from day 0 to day 12 the pH increased significantly (P < .05) among the uncoated papaya fruits and coated fruits (). The increase in pH occurred due to the ripening and senescence of the fruits and this probably resulted in the decrease in the acid content of the fruit since acids are important substrates for respiratory metabolism. The higher the metabolic respiration, the higher would be the decline in acidity of the fruit and vice versa. Therefore, the change in acid content is an indicator for monitoring ripening stages (Narsaiah et al., Citation2015). Similar result was reported by Cortez-Vega et al. (Citation2014) on fresh-cut papaya, and Li et al. (Citation2017) on fresh-cut kiwi.
Total soluble solid expressed in brix is an indicator that reflects the ripening of fruits. In the current study, the total soluble solid increased (P < .05) progressively in the uncoated papaya fruits compared with the coated fruits (). The increase in the total soluble solid in the uncoated papaya fruits might be due to increase degradation or biosynthesis of polysaccharides into simple sugars. Furthermore, it also increases due to moisture loss because of the accumulation of sugars in the tissues. However, the coated sample was able to demonstrate minimal increase in the values of total soluble solid. Edible coatings are able to modify the internal atmosphere by elevating CO2 and/or reducing O2, thus retarding the respiration rate and suppressing ethylene production. Slow respiration rates in turn, delays the synthesis and the use of metabolites that results in lowering the soluble solid concentration. Similar results were reported by Li et al. (2017) on edible coating for fresh-cut kiwi, and Cortez-Vega et al. (Citation2014) on fresh-cut papaya.
Firmness
Texture is also a critical factor for fruit quality and reflects metabolic changes and changes in water content. The maintenance of the firmness is an important factor for increasing shelf -life of fresh-cut products (Musacchi & Serra, Citation2018). From , it can be seen that, the reduction in firmness among uncoated fruits were significantly lower (P < .05) than coated samples (P > .05). Moreover, there was a significant difference (P < .05) between the coated and uncoated papaya from day 6 to day 12. The decrease in firmness is due to fruit ripening and softening. Softening is due to progressive cell wall modification and disassembly by enzyme action, leading to the solubilisation and de-polymerization of pectin and hemicelluloses. Furthermore, it also related to weight loss of fresh-cut papaya (Guerreiro et al., Citation2017).This result is in agreement with Cortez-Vega et al. (Citation2014), who coated fresh-cut papaya with protein isolate. According to their results, the firmness values were; 15 N and17.5 N for uncoated and coated fruits, respectively. However, in this study the firmness value for coated sample was higher (23.83N).
Colour properties of fresh – cut papaya
Colour is an important sensory attribute for consumer acceptability. Three parameters that were taken into consideration were lightness (L*), green-red (a*) and blue-yellow (b*) colour, and these were used as the index to show the colour difference between the coated and uncoated papaya (Sui Chin et al., Citation2017).There was a significant (P < .05) decrease in “L” values among uncoated papaya as well as coated samples with storage, indicating ripening. Moreover, from day 6 to day 12 both uncoated and coated papaya fruits decreased significantly (P < .05). It can be seen that, the L value which is lightness of the fresh-cut papaya decreased (P < .05) significantly while the coated fresh-cut papaya showed minimum decrease in L value. Similar decreases in “L” values with storage have been reported in the case of fresh-cut papaya (Waghmare & Annapure, Citation2013). Likewise, “b” values which represent yellowness when positive, decreased significantly (P < .05) with storage for uncoated papaya and insignificantly (P > .05) for coated samples. Meanwhile, “a” values which represent redness when positive increased significantly (P < .05) in uncoated papaya and insignificantly (P > .05) in coated samples. Moreover, after day 6 it was found that the values of b and a were significantly different (P < .05). Slower colour change in the coated samples could be attributed to the slow respiration rate which in turn delayed ripening and the senescence. Similar trends have been reported by Brasil, Gomes, Puerta-Gomez, Castell-Perez, and Moreira (Citation2012) on fresh-cut papaya coated with polysaccharide.
Ascorbic acid
AA also referred to as vitamin C is among some of the vital water-soluble vitamins, and it is an important vitamin in papaya (Magwaza, Mditshwa, Tesfay, & Opara, Citation2017). On day 0, the AA contents of fresh-cut papaya were; 72 mg/100 g and 73.5 mg/100 g for both uncoated and coated fresh-cut papaya. However, it was found that the AA values decreased gradually in both coated and uncoated fresh-cut papaya during storage. However, by the end of the storage period (day 12) the coated papaya fruits were noticeably better preserved than uncoated papaya fruits (). This is due to the reduced oxygen access to the tissues of the coated fresh-cut papaya compared to that of the uncoated samples. It is a known fact that AA degradation is dependent on oxygen concentration (dos Santos Caetano et al., Citation2018). Furthermore, according to USDA National database standard, papaya should contain 60.9 mg/100 g of AA. Thus, the coated fresh-cut papaya AA amount was within the range. A similar result was observed by Brasil et al. (Citation2012) on fresh-cut papaya coated with polysaccharide
Microbial analysis
Food spoilage microorganisms are the main causes of fresh fruit deterioration (Guerreiro et al., Citation2017). ) displays the growth of Total plate count (TPC) and ) displays YM in uncoated and coated fresh-cut papaya fruits during 12 days refrigerated storage. During the storage period, increases in microbial count were observed in all the samples. According to the Institute of Food Science and Technology 10^6 CFU/g is considered as the limit of microbial load acceptance for fruit-based products (Azarakhsh, Osman, Ghazali, Tan, & Mohd Adzahan, Citation2014). Based on this limit, the coated fruit has significantly (P < .05) lower total plate count than the uncoated papaya. It can be seen that, the total plate count in coated sample reached the limit on day 12 while the uncoated papaya attained the limit much earlier (i.e. on the 8th, day).
Figure 1. Microbial analyses of fresh-cut papaya during 12 days of storage. (a) Total plate count and (b) yeast and mould count.
Figura 1. Análisis microbianos de la papaya recién cortada durante 12 días de almacenamiento. (a) Recuento total en placa y (b) conteo de levadura y moho.
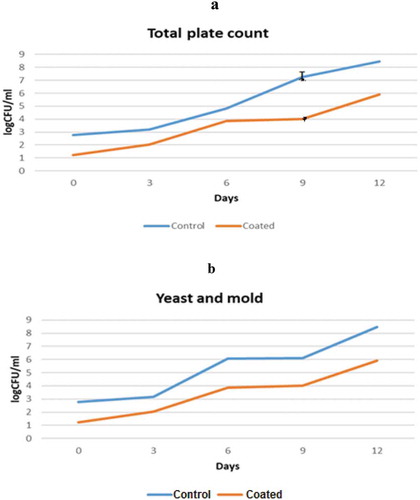
In terms of YM count the coated papaya has significantly slower increment (P < .05) than uncoated sample. The coated papaya reached the maximum limit on day 12 and uncoated sample reached maximum limit on day 6. Another reason for the longer shelf life of the coated fresh-cut papaya might be due to the aloe vera incorporated in the coating. In particular, aloe vera compounds such as; saponins, acemannan, and anthraquinones derivatives have been reported to be responsible for antibacterial activity (Radi, Firouzi, Akhavan, & Amiri, Citation2017). Similar result was reported by Benítez et al. (Citation2015) on fresh slice kiwi coated with aloe vera, and on strawberry coated with aloe vera (Radi, Firouzi, Akhavan, & Amiri, Citation2017).
Conclusion
In conclusion, the results obtained in this study showed that the composite starch-based edible coating has improved the physiochemical properties of fresh-cut papaya in terms of reduced weight loss, better firmness (23.83 N), minimal increase in total soluble solid (11.03), better colour retention, and lower decrease in AA content (65.24 mg/100 g) during storage. Furthermore, the microbial analysis showed that, the coating was able to extend the shelf life of fresh-cut papaya up to 12 days at refrigerated condition. Thus, this edible coating has the potential to extend the shelf life of fresh-cut fruits and will be able to reduce fruit wastage.
Conflict of Interest
The authors declare that they have no conflicting interest.
Acknowledgements
The authors are grateful for the extensive financial support received from the University Putra Malaysia research scheme grant (9478500).
Additional information
Funding
References
- Adjouman, Y. D., Nindjin, C., Tetchi, F. A., Dalcaq, A. C., Amani, N. G., & Sindic, M. (2017). Water vapor permeability of edible films based on improved Cassava (Manihot esculenta Crantz) native starches. Journal of Food Process Technol, 8(665). doi:10.4172/2157-7110.1000665
- Albertini, S., Lai Reyes, A. E., Trigo, J. M., Sarriés, G. A., & Spoto, M. H. F. (2016). Effects of chemical treatments on fresh-cut papaya. Food Chemistry, 190, 1182–1189.
- Alves, M. M., Gonçalves, M. P., & Rocha, C. M. R. (2017). Effect of ferulic acid on the performance of soy protein isolate-based edible coatings applied to fresh-cut apples. LWT - Food Science and Technology, 80, 409–415.
- AOAC (2000). The official methods of analysis (17th ed.). Maryland, USA: Association of Official Analytical Chemists.
- ASTM E1925. (1995). Standard test method for yellowness idnex of plasticss. Philadelphia, PA: American society for Testing and Materials.
- Azarakhsh, N., Osman, A., Ghazali, H. M., Tan, C. P., & Mohd Adzahan, N. (2014). Lemongrass essential oil incorporated into alginate-based edible coating for shelf-life extension and quality retention of fresh-cut pineapple. Postharvest Biology and Technology, 88, 1–7.
- Bashir, M., & Haripriya, S. (2016). Physicochemical and structural evaluation of alkali extracted chickpea starch as affected by γ-irradiation. International Journal of Biological Macromolecules, 89, 279–286. doi:10.1016/j.ijbiomac.2016.04.048
- Benítez, S., Achaerandio, I., Pujolà, M., & Sepulcre, F. (2015). Aloe vera as an alternative to traditional edible coatings used in fresh-cut fruits: A case of study with kiwifruit slices. LWT - Food Science and Technology, 61(1), 184–193.
- Bertuzzi, M. A., Gottifredi, J. C., & Armada, M. (2012). Mechanical properties of a high amylose content corn starch based film, gelatinized at low temperature. Brazilian Journal of Food Technology, 15(3), 219–227. doi:10.1590/S1981-67232012005000015
- Brasil, I. M., Gomes, C., Puerta-Gomez, A., Castell-Perez, M. E., & Moreira, R. G. (2012). Polysaccharide-based multilayered antimicrobial edible coating enhances quality of fresh-cut papaya. LWT - Food Science and Technology, 47(1), 39–45. doi:10.1016/j.lwt.2012.01.005
- Cáez-Ramírez, G., Alamilla-Beltrán, L., & Gutiérrez-López, G. F. (2018). Morphometric analysis and tissue structural continuity evaluation of senescence progression in fresh cut papaya (Carica papaya L.). Journal of Food Engineering, 216, 107–119. doi:10.1016/j.jfoodeng.2017.08.004
- Chiumarelli, M., & Hubinger, M. D. (2014). Evaluation of edible films and coatings formulated with cassava starch, glycerol, carnauba wax and stearic acid. Food Hydrocolloids, 38, 20–27. doi:10.1016/j.foodhyd.2013.11.013
- Cortez-Vega, W. R., Pizato, S., De Souza, J. T. A., & Prentice, C. (2014). Using edible coatings from Whitemouth croaker (Micropogonias furnieri) protein isolate and organo-clay nanocomposite for improve the conservation properties of fresh-cut “Formosa” papaya. Innovative Food Science and Emerging Technologies, 22, 197–202. doi:10.1016/j.ifset.2013.12.007
- dos Santos Caetano, K., Almeida Lopes, N., Haas Costa, T.M., Brandelli, A., Rodrigues, E., Hickmann Flores, S., & Cladera-Olivera, F. (2018). Characterization of active biodegradable films based on cassava starch and natural compounds. Food Packaging and Shelf Life, 16, 138–147.
- Fabra, M. J., Pérez-Masiá, R., Talens, P., & Chiralt, A. (2011). Influence of the homogenization conditions and lipid self-association on properties of sodium caseinate based films containing oleic and stearic acids. Food Hydrocolloids, 25(5), 1112–1121. doi:10.1016/j.foodhyd.2010.10.008
- Falah, M. A. F., Nadine, M. D., & Suryandono, A. (2015). Effects of storage conditions on quality and shelf-life of fresh-cut melon (Cucumis Melo L.) and papaya (Carica Papaya L.). Procedia Food Science, 3, 313–322. doi:10.1016/j.profoo.2015.01.034
- Farahnaky, A., Saberi, B., & Majzoobi, M. (2013). Effect of glycerol on physical and mechanical properties of wheat starch edible films. Journal of Texture Studies, 44(3), 176–186. doi:10.1111/jtxs.2013.44.issue-3
- Fernández, L., De Apodaca, E. D., Cebrián, M., Villarán, M. C., & Maté, J. I. (2007). Effect of the unsaturation degree and concentration of fatty acids on the properties of WPI-based edible films. European Food Research and Technology, 224(4), 415–420. doi:10.1007/s00217-006-0305-1
- Gennadios, A., Weller, C. L., & Gooding, C. H. (1994). Measurement errors in water vapor permeability of highly permeable, hydrophilic edible films. Journal of Food Engineering, 21, 395–409. doi:10.1016/0260-8774(94)90062-0
- Gheribi, R., Puchot, L., Verge, P., Jaoued-Grayaa, N., Mezni, M., Habibi, Y., & Khwaldia, K. (2018). Development of plasticized edible films from Opuntia ficus-indica mucilage: A comparative study of various polyol plasticizers. Carbohydrate Polymers, 190, 204–211. doi:10.1016/j.carbpol.2018.02.085
- Gooch, J. W. (2011). Whiteness index. In J. W. Gooch (Ed.), Encyclopedic dictionary of polymers. New York, NY: Springer.
- Guerreiro, A. C., Gago, C. M. L., Faleiro, M. L., Miguel, M. G. C., & Antunes, M. D. C. (2017). The effect of edible coatings on the nutritional quality of ‘Bravo de Esmolfe’ fresh-cut apple through shelf-life. LWT - Food Science and Technology, 75, 210–219. doi:10.1016/j.lwt.2016.08.052
- Gutiérrez, T. J., Herniou-Julien, C., Álvarez, K., & Alvarez, V. A. (2018). Structural properties and in vitro digestibility of edible and pH-sensitive films made from guinea arrowroot starch and wastes from wine manufacture. Carbohydrate Polymers, 184, 135–143. doi:10.1016/j.carbpol.2017.12.039
- Hashemi, S. M. B., Mousavi Khaneghah, A., Ghaderi Ghahfarrokhi, M., & Eş, I. (2017). Basil-seed gum containing Origanum vulgare subsp. viride essential oil as edible coating for fresh cut apricots. Postharvest Biology and Technology, 125, 26–34. doi:10.1016/j.postharvbio.2016.11.003
- Hassan, B., Chatha, S. A. S., Hussain, A. I., Zia, K. M., & Akhtar, N. (2018). Recent advances on polysaccharides, lipids and protein based edible films and coatings: A review. International Journal of Biological Macromolecules, 109, 1095–1107. doi:10.1016/j.ijbiomac.2017.11.097
- Huang, J., Schols, H. A., van Soest, J. J. G., Jin, Z., Sulmann, E., & Voragen, A. G. J. (2007). Physicochemical properties and amylopectin chain profiles of cowpea, chickpea and yellow pea starches. Food Chemistry, 101(4), 1338–1345. doi:10.1016/j.foodchem.2006.03.039
- Isotton, F. S., Bernardo, G. L., Baldasso, C., Rosa, L. M., & Zeni, M. (2015). The plasticizer effect on preparation and properties of etherified corn starchs films. Industrial Crops and Products, 76, 717–724. doi:10.1016/j.indcrop.2015.04.005
- Jiménez, A., Fabra, M. J., Talens, P., & Chiralt, A. (2010). Effect of lipid self-association on the microstructure and physical properties of hydroxypropyl-methylcellulose edible films containing fatty acids. Carbohydrate Polymers, 82(3), 585–593. doi:10.1016/j.carbpol.2010.05.014
- Jiménez, A., Fabra, M. J., Talens, P., & Chiralt, A. (2012). Effect of re-crystallization on tensile, optical and water vapour barrier properties of corn starch films containing fatty acids. Food Hydrocolloids, 26(1), 302–310. doi:10.1016/j.foodhyd.2011.06.009
- John, A., Yang, J., Liu, J., Jiang, Y., & Yang, B. (2018). The structure changes of water-soluble polysaccharides in papaya during ripening. International Journal of Biological Macromolecules, 115, 152–156. doi:10.1016/j.ijbiomac.2018.04.059
- Koh, P. C., Noranizan, M. A., Nur Hanani, Z. A., Karim, R., & Rosli, S. Z. (2017). Application of edible coatings and repetitive pulsed light for shelf life extension of fresh-cut cantaloupe (Cucumis melo L. reticulatus cv. Glamour). Postharvest Biology and Technology, 129, 64–78. doi:10.1016/j.postharvbio.2017.03.003
- Lasekan, O., & Abbas, K. A. (2012). Distinctive exotic flavor and aroma compounds of some exotic tropical fruits and berries: A review. Critical Reviews in Food Science and Nutrition, 52, 726–735. doi:10.1080/10408398.2010.507910
- Lasekan, O, Lasekan, W, Idowu, M, & Ojo, A. (1996). Effect of extrusion cooking conditions on the nutritional value, storage stability and sensory characteristics of a maize-based snack food. Journal Of Cereal Science, 24, 79–85.
- Li, S., Zhang, L., Liu, M., Wang, X., Zhao, G., & Zong, W. (2017). Effect of poly-e-lysine incorporated into alginate-based edible coatings on microbial and physicochemical properties of fresh-cut kiwifruit. Postharvest Biology and Technology, 134, 114–121.
- Liaotrakoon, V., & Raviyan, P. (2018). Modifying the properties of whey protein isolate edible film by incorporating palm oil and glycerol. Songklanakarin Journal of Science and Technology, 40(1), 243–249.
- Liplap, P., Vigneault, C., Toivonen, P., Charles, M. T., & Raghavan, G. S. V. (2013). Effect of hyperbaric pressure and temperature on respiration rates and quality attributes of tomato. Postharvest Biology and Technology, 86, 240–248. doi:10.1016/j.postharvbio.2013.07.002
- Liu, P., Sun, S., Hou, H., & Dong, H. (2016). Effects of fatty acids with different degree of unsaturation on properties of sweet potato starch-based films. Food Hydrocolloids, 61, 351–357. doi:10.1016/j.foodhyd.2016.05.033
- Magwaza, L. S., Mditshwa, A., Tesfay, S. Z., & Opara, U. L. (2017). An overview of preharvest factors affecting vitamin C content of citrus fruit. Scientia Horticulturae, 216, 12–21. doi:10.1016/j.scienta.2016.12.021
- Malaterre, A. S., Stanislas, G., Douraguia, E., & Gonthier, M. P. (2016). Evaluation of nutritional and antioxidant properties of the tropical fruits banana, litchi, mango, papaya, passion fruit and pineapple cultivated in Réunion French Island. Food Chemistry, 212, 225–233. doi:10.1016/j.foodchem.2016.05.147
- Maryam Adilah, Z. A., Jamilah, B., & Nur Hanani, Z. A. (2018). Functional and antioxidant properties of protein-based films incorporated with mango kernel extract for active packaging. Food Hydrocolloids, 74, 207–218. doi:10.1016/j.foodhyd.2017.08.017
- Mattheis, J. P., & Fellman, J. K. (2000). Impacts of modified atmosphere packaging and controlled atmosphere on aroma, flavor, and quality of horticultural commodities. HortTechnology, 10, 507–510. doi:10.21273/HORTTECH.10.3.507
- Mohammad Amini, A., Razavi, S. M. A., & Zahedi, Y. (2015). The influence of different plasticisers and fatty acids on functional properties of basil seed gum edible film. International Journal of Food Science and Technology, 50(5), 1137–1143. doi:10.1111/ijfs.12765
- Moreíra, M. R., Tomadoni, B., Martín-Belloso, O., & Fortuny, R. S. (2015). Preservation of fresh-cut apple quality attributes by pulsed light in combination with gellan gum-based prebiotic edible coatings. LWT - Food Science and Technology, 64(2), 1130–1137. doi:10.1016/j.lwt.2015.07.002
- Musacchi, S., & Serra, S. (2018). Apple fruit quality: Overview on pre-harvest factors. Scientia Horticulturae, 234, 409–430. doi:10.1016/j.scienta.2017.12.057
- Narsaiah, K., Wilson, R. A., Gokul, K., Mandge, H. M., Jha, S. N., Bhadwal, S., & Vij, S. (2015). Effect of bacteriocin-incorporated alginate coating on shelf-life of minimally processed papaya (Carica papaya L.). Postharvest Biology and Technology, 100, 212–218. doi:10.1016/j.postharvbio.2014.10.003
- Oyeyinka, S., Singh, S., & Amonsou, E. (2017). Physicochemical and mechanical properties of Bambara Groundnut starch films modified with stearic acid. Journal of Food Science, 82, 118–123.
- Pavlath, A. E., & Orts, W. (2009). Edible films and coatings: Why, what, and how?. In Hubert, K., Embuscado, M (Eds.), Edible films and coatings for food applications. New York, NY: Springer.
- Qadri, O. S., Yousuf, B., & Srivastava, A. K. (2015). Fresh-cut fruits and vegetables: Critical factors influencing microbiology and novel approaches to prevent microbial risk.A review. Cogent Food & Agriculture, 1(1). doi:10.1080/23311932.2015.1121606
- Radi, M., Firouzi, E., Akhavan, H., & Amiri, S. (2017). Effect of gelatin-based edible coatings incorporated with aloe vera and black and green tea extracts on the shelf-life of fresh-cut oranges. Journal Of Food Quality, 2017. doi:10.1155/2017/9764650
- Ramos, Ó. L., Reinas, I., Silva, S. I., Fernandes, J. C., Cerqueira, M. A., Pereira, R. N., … Malcata, F. X. (2013). Effect of whey protein purity and glycerol content upon physical properties of edible films manufactured therefrom. Food Hydrocolloids, 30(1), 110–122. doi:10.1016/j.foodhyd.2012.05.001
- Rompothi, O., Pradipasena, P., Tananuwong, K., Somwangthanaroj, A., & Janjarasskul, T. (2017). Development of non-water soluble, ductile mung bean starch based edible film with oxygen barrier and heat sealability. Carbohydrate Polymers, 157, 748–756. doi:10.1016/j.carbpol.2016.11.031
- Santacruz, S., Rivadeneira, C., & Castro, M. (2015). Edible films based on starch and chitosan. Effect of starch source and concentration, plasticizer, surfactant's hydrophobic tail and mechanical treatment. Food Hydrocolloids, 49, 89–94.
- Schmidt, V. C. R., Porto, L. M., Laurindo, J. B., & Menegalli, F. C. (2013). Water vapor barrier and mechanical properties of starch films containing stearic acid. Industrial Crops and Products, 41(1), 227–234. doi:10.1016/j.indcrop.2012.04.038
- Seyedi, S., Koocheki, A., Mohebbi, M., & Zahedi, Y. (2015). Improving the physical and moisture barrier properties of Lepidium perfoliatum seed gum biodegradable film with stearic and palmitic acids. International Journal of Biological Macromolecules, 77, 151–158. doi:10.1016/j.ijbiomac.2015.03.005
- Souza, A. C., Benze, R., Ferrão, E. S., Ditchfield, C., Coelho, A. C. V., & Tadini, C. C. (2012). Cassava starch biodegradable films: Influence of glycerol and clay nanoparticles content on tensile and barrier properties and glass transition temperature. LWT - Food Science and Technology, 46(1), 110–117. doi:10.1016/j.lwt.2011.10.018
- Sui Chin, S., Han Lyn, F., & Nur Hanani, Z. A. (2017). Effect of Aloe vera (Aloe barbadensis Miller) gel on the physical and functional properties of fish gelatin films as active packaging. Food Packaging and Shelf Life, 12, 128–134. doi:10.1016/j.fpsl.2017.04.008
- Thakur, R., Saberi, B., Pristijono, P., Golding, J., Stathopoulos, C., Scarlett, C., … Vuong, Q. (2016). Chraacterization of rice starch-ι-carrageenan biodegradable edible film. Effect of stearic acid on the film properties. International Journal of Biological Macromolecules, 93, 952–960. doi:10.1016/j.ijbiomac.2016.09.053
- Waghmare, R. B., & Annapure, U. S. (2013). Combined effect of chemical treatment and/or modified atmosphere packaging (MAP) on quality of fresh-cut papaya. Postharvest Biology and Technology, 85, 147–153. doi:10.1016/j.postharvbio.2013.05.010
- Yunos, M. Z. B., & Rahman, W. A. W. A. (2011). Effect of glycerol on performance rice straw/starch based polymer. Journal of Applied Sciences, 11(13), 2456–2459. doi:10.3923/jas.2011.2456.2459
- Zahedi, Y., Ghanbarzadeh, B., & Sedaghat, N. (2010). Physical properties of edible emulsified films based on pistachio globulin protein and fatty acids. Journal of Food Engineering, 100(1), 102–108. doi:10.1016/j.jfoodeng.2010.03.033
- Zhang, P., Zhao, Y., & Shi, Q. (2016). Characterization of a novel edible film based on gum ghatti: Effect of plasticizer type and concentration. Carbohydrate Polymers, 153, 345–355. doi:10.1016/j.carbpol.2016.07.094