ABSTRACT
Nutritional authorities state that both total carbohydrates and low glycemic index (GI) diets affect glycemic control. High hydrostatic pressure (HHP) processing reduced the GI response of mango pulp when consumed by healthy adults. Present work evaluated the effects of HHP processing on concentrations of mango pulp dietary fiber sub-fractions (AOAC 2011.25 method), pectin methyl esterase (PME) activity, and established links with reduction of GI responses in healthy adults. Processing stability of mango pulp micronutrients (carotenoids, phenolics, ascorbic acid, and antioxidant activity) were also assessed. HHP-processing caused fiber inter-conversions from insoluble to soluble dietary fiber, the latter being significantly higher. Concentrations of non-digestible oligosaccharides and PME activities were also higher in treated pulps. In addition, HHP-processing preserved relevant mango phytonutrients such as carotenoids and phenolic compounds. Micronutrient retention and dietary fiber modifications suggested that HHP-processing improved mango pulp benefits for healthy subjects and potentially for patients with chronic metabolic diseases.
RESUMEN
Los lineamientos nutricionales establecen que el contenido de carbohidratos totales y el consumo de dietas con bajo índice glucémico (IG) tienen impacto sobre el control glucémico. Se ha reportado que el procesado de pulpa de mango con alta presión hidrostática (HHP) disminuye la respuesta de IG del fruto en adultos sanos. El objetivo del presente trabajo se centró en estudiar los efectos del tratamiento con HHP sobre las concentraciones de las sub-fracciones de fibra dietaria de la pulpa de mango (método AOAC 2011.25), la actividad de la enzima pectin metil esterasa (PME), y el establecimiento de relaciones con la reducción IG en adultos sanos. Paralelamente, se caracterizó la estabilidad de los micronutrientes de la pulpa de mango (carotenoides, fenólicos, ácido ascórbico y capacidad antioxidante) al procesado con HHP. La presurización con HHP generó la inter-conversión de la fibra dietaria insoluble en soluble, incrementando signficativamente sus niveles. La actividad de PME se incrementó significativamente en las muestras tratadas con HHP. Los carotenoides y compuestos fenólicos, se mantuvieron constantes después del procesado con HHP. Los resultados de retención de micronutrientes y modificaciones de sub-fracciones de fibra dietaria indicaron que el procesamiento con HHP mejora los beneficios de la pulpa de mango para individuos sanos y potencialmente para pacientes con enfermedades crónico-degenerativas.
1. Introduction
The role of dietary fiber in promotion of health and disease prevention has now become related to metabolic pathways and to overall metabolism in humans. Soluble fibers have been widely studied for their roles in glucose (Weickert & Pfeiffer, Citation2018) and cholesterol metabolisms (Ho et al., Citation2016). The impact of food processing technologies on preservation of the functional properties of dietary fiber to enhance its health benefits has also been described. For instance, a recent review on pectin, as a soluble dietary fiber, focused on summarizing structural modifications that can be attained by traditional thermal and novel non-thermal processing technologies, such as the use of high hydrostatic pressure (HHP) (Christiaens et al., Citation2016).
The commercial use of non-thermal processing technologies, such as HHP, has been growing world-wide as an alternative to pasteurize a wide variety of products without compromising their flavor and fresh-like characteristics, including fruit pulps, vegetable juices, smoothies, and refrigerated products like hams, cheeses, and prepared meals, among others. However, knowledge on the effects of this non-traditional pasteurization technology on the macronutrients and micronutrients of foods is still a growing field of nutritional research. For instance, HHP treatment at commercial conditions (592 MPa for 3 min) reduced glycemic index (GI) of mango pulp of healthy humans (Elizondo-Montemayor et al., Citation2015). Clinical observations were found to be associated with rheological changes in the mango pulp matrix, such as higher viscosity and higher cell-wall water-holding capacity for the HHP treated pulps, however the authors did not observe significant changes in the dietary fiber components. Similarly, other works have also described no HHP-induced inter-conversions in the dietary fiber compositions using older fiber analysis methodologies (Hernandez-Carrion, Vazquez-Gutierrez, Hernando, & Quiles, Citation2014; Rodríguez-Garayar, Martín-Cabrejas, & Esteban, Citation2017; Vega-Galvez et al., Citation2014). On the contrary, other authors have reported inter-conversion of insoluble dietary fiber (IDF) into soluble dietary fiber (SDF) in HHP-treated by-products from plant sources, but, noteworthy, without studying their clinical implications (Mateos-Aparicio, Mateos-Peinado, & Rupérez, Citation2010; Tejada-Ortigoza, Garcia-Amezquita, Serna-Saldivar, & Welti-Chanes, Citation2017). Prior authors, which observed fiber inter-conversions, differed in the plant food matrixes used and in the fiber analysis methodologies. Mateos-Aparicio et al. (Citation2010) reported an 8-fold increase in the SDF contents of okara from soybean after HHP processing (400MPa and 60°C) when compared with the unprocessed sample (control). Similarly, Tejada-Ortigoza et al. (Citation2017) treated fruit peels with HHP and observed increases in SDF that ranged from 2 to 59% depending on processing conditions and type of plant matrix (Tejada-Ortigoza et al., Citation2017). Discrepancies among prior works on the effect of HHP on dietary fibers can be attributed, in part, to the use of prior official AOAC fiber analysis methodologies AOAC 985.29 (Prosky et al., Citation1985) and AOAC 991.43 (Lee, Prosky, & DeVries, Citation1992) that were less accurate in the quantification of IDF, SDF, and its sub-fractions than the subsequently proposed integrated fiber analysis method AOAC 2011.25 (McCleary et al., Citation2012). The newer method, named the integrated fiber analysis methodology, incorporated improvements to simulate human digestion, remove components that cause overestimation and allows for quantification of resistant starch and the non-digestible oligosaccharides (NDOs) contained in a sub-fraction of SDF. Quantification of NDOs, as soluble dietary fibers, has gained importance in recent years due to their contributions to human metabolism and promotion of a healthy microbiome (Bindels et al., Citation2015; Bruno-Barcena & Azcarate-Peril, Citation2015).
Mango pulp was selected for the present work, since it is a highly consumed fruit by the general population as well as by diabetic patients worldwide, with considerable concerns by consumers regarding its sugar content and the potential impact on blood sugar levels (Elizondo-Montemayor et al., Citation2015). Furthermore, mango is a rich source of provitamin A carotenoids and other relevant antioxidants such as vitamin C and phenolics (Ibarra-Garza, Ramos-Parra, Hernández-Brenes, & Jacobo-Velázquez, Citation2015; Xiao, Citation2016), which contribute important health benefits.
The present work focused on studying the effects of HHP processing on pectin methyl esterase (PME) activities, concentrations of mango pulp dietary fiber sub-fractions (assessed by AOAC 2011.25 integrated official method), and evaluated potential associations with previously reported reductions in GI responses of healthy adults after consumption of HHP-processed mango pulp. Likewise, the present work aimed to characterize the carotenoids, phenolics, ascorbic acid and the antioxidant activity of mango pulp after the HHP treatment to address concerns on the stability of relevant mango micronutrients under commercially used conditions.
2. Materials and methods
2.1. High hydrostatic pressure (HHP) processing
Ripe mangos (Mangifera indica L. cv. Tommy Atkins) were obtained from a local market. Mango fruits (120 kg) were selected by maturity stage, peel color, shape and size. Fruits were washed with 0.5 mL/L sodium hypochlorite (6%), manually peeled and homogenized in a blender (Torrey LP12, San Nicolas de los Garza, N.L., México). Individual mango pulp packets (200 g) were vacuum packaged in plastic bags and maintained in a cold-water bath (<3ºC) prior to processing. Samples were processed under similar commercial processing conditions as those described by Jacobo-Velázquez, Ramos-Parra, and Hernández-Brenes (Citation2010), which included pressurization at 592 MPa, 3 min, 25ºC in a High Pressure Processing System QUINTUS® Type QFP 350L-600 (Avure Technologies, Kent, WA, USA), using water as the pressure-transmitting fluid. The time required to reach 592 MPa was 3.5 min. The decompression time was 2.75 min. Afterwards, HHP treated mango pulps and the unprocessed controls were stored at −80°C until further phytochemical evaluations were conducted.
2.2. Chemicals
Sodium hydroxide (NaOH), sodium chloride (NaCl), sulfuric acid (H2SO4), sodium tetraborate (Na2B4O7), potassium hydroxide (KOH), ascorbic acid, phenolphthalein, isopropanol (HPLC grade), acetone, and ethanol were obtained from Desarrollo de Especialidades Químicas (San Nicolas de los Garza, N.L., México). Ethylenediaminetetraacetic acid calcium disodium (Na2Ca-EDTA), m-hydroxydiphenyl, citrus pectin, bromothymol blue, fluorescein, butylated hydroxytoluene (BHT) 2,2´-azobis-(2-methylpropionamidine) dihydrochloride (AAPH); D-sorbitol, D-glucose, D-galacturonic acid, trans-β-apo-8ʹ-carotenal, and all-trans-β-carotene were obtained from Sigma-Aldrich (St. Louis, MO, USA). All-trans-lutein was obtained from Indofine Chemical Company, Inc (Hillsborough, N.J, USA). HPLC grade solvents, methyl tert-butyl ether (MTBE) and methanol (MeOH), were obtained from Fisher Scientific Int. (Winnipeg, MB., Canada). Petroleum ether and diethyl ether were acquired from CTR Scientific (Monterrey, N.L., México).
2.3. Physicochemical analyses
Mango pulps were directly analyzed using a refractometer (Master-α, Atago, Japan) for the determination of their soluble solid contents (°Brix). Moisture content was determined using 5 g of mango pulp, which was dried at 60°C for 48 h in an aluminum dish (Ibarra-Garza et al., Citation2015). Acidity (%) and pH were determined as described by Ibarra-Garza et al. (Citation2015).
2.4. Dietary fiber determination
TDF (Total Dietary Fiber), SDF and IDF dietary fiber concentrations were determined by AOAC 991.43 and AOAC 985.29 methods (Lee et al., Citation1992; Prosky et al., Citation1985, Citation1994) using the total dietary fiber assay kit from Megazyme (K-TDFR) (Wicklow, Ireland). Analyses were performed in duplicate according to the “Total Dietary Fiber” official method and the results were expressed as grams (g) of dietary fiber per 100 g of sample (dry weight).
A second dietary fiber determination was performed by the integrated TDF method AOAC 2011.25 (McCleary et al., Citation2012) to analyze IDF, SDF and two sub-fractions of SDF with higher- and lower molecular weights (SDFP and SDFS, respectively). As described in the AOAC 2011.25 method, SDFP precipitated at a concentration of 78% (v/v) ethanol and SDFS was soluble at 78% (v/v) ethanol and contained oligosaccharides that are not digested by humans (NDOs). SDF was quantified as the sum of SDFP and SDFS, whereas total dietary fiber (TDF) was quantified as the sum of SDF and IDF. Quantifications of the SDFS fraction were performed as described by McCleary et al. (Citation2012) and Tejada-Ortigoza et al. (Citation2017); using a Waters 515 HPLC system equipped with a refractive index detector (Waters Co., Milford, MA, USA). Chromatographic separation of SDFS was conducted in a Bio-Rad Aminex® column (300 x 7.8 mm) at 90°C, using Na2Ca-EDTA (50 mg L−1) as mobile phase at a flow-rate of 0.5 mL·min−1 Standard calibration curves were prepared using D-sorbitol (internal standard) or D-glucose. Separations and quantifications were performed in quadruplicate and the results were expressed as grams (g) of dietary fiber per 100 g of sample.
2.4.1. Uronic acid determination
Aliquots of the SDFS sub-fractions (20 µL) from the control and HHP treated mango puree samples were assayed for uronic acid content by the m-hydroxydiphenyl method of Kintner and Van Buren (Citation1982) with slight modifications. Calibration curve was prepared with galacturonic acid standard (0–60 μg/mL). Analytical standards and SDFS aliquots were adjusted to volume (500 µl) and placed in an ice-water bath. Afterwards, 3 mL of a H2SO4/Na2B4O7 solution (0.0125 M) were added to each tube in an ice-water bath and then samples homogenized. Tubes were then heated in a water-bath (100°C, 5 min) and immediately placed in the ice-water bath. A solution of 0.15% m-hydroxydiphenyl (50 μL) was then added to all SDFS sub-fractions samples and standard solutions. Absorbance measurements (520 nm, 25°C) were recorded using a Beckman Coulter DU-800 spectrophotometer (Brea, CA, USA).
2.5. Pectin methyl esterase (PME) activity
PME enzymatic activities of mango puree extracts were determined according to Hagerman and Austin (Citation1986), with slight modifications. Enzyme extracts were obtained by homogenization of mango pulp samples (2 g) with a NaCl solution (8.8%, 4 mL) at 4°C. Homogenates were centrifuged (18,000 x g per 15 min) and supernatants were collected. Assay solutions for PME activity determination included citrus pectin as substrate (0.5%, 2 mL), distilled water (0.7 mL) and bromothymol blue (0.01%, 0.2 mL), adjusted at pH 7.5. For the actual assay, an aliquot of the enzyme extract (0.1 mL) was added; and PME activity units were determined in a Beckman Coulter DU-800 spectrophotometer (Brea, CA, USA) at 620 nm and 25°C through demethylation kinetics of the substrate (μmol of acid released per min).
2.6. Antioxidant analyses
Mango pulp (3 g) extracts analyzed for phenolic compounds and antioxidant capacities were prepared by homogenization with aqueous methanol (80%, 3mL) using an Ultra-turrax homogenizer (IKA Works, Inc., Wilmington, NC), then centrifuged at 12,175 x g for 15 min (4°C). Total phenolics were estimated by the Folin-Ciocalteu method adapted to a 96-well microplate format; and the plate was read at 725 nm using a plate reader (Synergy HT, Bio Tek, Winooski, USA). The results were expressed as mg gallic acid equivalents (GAE) per 100 g of mango fresh weight (FW). Antioxidant capacity values were determined by the oxygen radical absorbing capacity (ORAC) method as described by Ibarra-Garza et al. (Citation2015). Fluorescein was used as fluorophore, and AAPH as free-radical generator. Results were expressed as µM of trolox equivalents (TroloxE) per 100 g sample FW. Total ascorbic acid was analyzed using the method reported by Gillespie and Ainsworth (Citation2007), and adapted to a 96-well microplate format. Results were expressed as mg ascorbic acid per 100 g FW.
2.7. Carotenoids analyses
2.7.1. Extraction of mango carotenoids
Carotenoid extractions were performed as described by Ibarra-Garza et al. (Citation2015). All extraction solvents contained BHT (0.1%). Isolations were conducted in the dark, and at room temperature (25°C). Trans-β-apo-8ʹ-carotenal was added to the mango pulp (2 g) as internal standard and samples were homogenized with acetone (10 mL). Thereafter, unprocessed and the HHP treated mango sample extracts were filtered under vacuum through Whatman No.1 filter paper (New Jersey, USA) and acetone extracts were recovered. Extraction was repeated three times, and acetone extracts were concentrated under vacuum using a Buchi Rotavapor R-215 (Flawil, Switzerland) set at 37°C, 100 rpm and 300 mbar. Concentrated extracts were saponified with 9M KOH (1 mL) prepared in water-ethanol (1:1 v/v) and with ascorbic acid (0.5 g) as antioxidant. Saponification reaction was performed for 12 h at room temperature in a close system under N2. Afterwards, carotenoids were recovered in a separation funnel with petroleum ether and diethyl ether mixture (1:1 v/v, 30mL). Then, water was added (10 mL) in order to remove the alkali, using phenolphthalein as indicator. If emulsion between the organic and aqueous phases occurred, a 30% NaCl solution (3 mL) was added. Carotenoid extracts were concentrated to dryness in a rotary evaporator (37°C, 100 rpm, 700 mbar for 10 min); and samples were re-suspended in isopropanol (1 mL). Extracts were filtered through polytetrafluoroethylene membranes (0.45 µm) (MillexTM, Millipore, Billerica, MA, USA) prior to HPLC analysis.
2.7.2. Carotenoid identification and quantification by HPLC-DAD
Carotenoid separation was performed with a HPLC system (Agilent Technologies 1260 Series, Santa Clara, CA, USA) coupled with a diode array detector (DAD). The stationary phase was a C30 reverse-phase column, 4.6 mm x 150 mm, 3 µm, (YMC carotenoid, Milford, MA, USA); using two mobile phases for chromatographic separation: phase A was composed of methanol/water (96:4 v/v) and phase B consisted of MTBE. Gradient elution consisted in 0/5, 10/10, 40/45, 45/75, 50/100, 55/100, 57/5, 75/5 (min/%phase B) with a flow rate of 0.75 mL/min. The analysis was performed at 450 nm. Peak identification was performed using three methods as described in Ibarra-Garza et al. (Citation2015): A) comparison of reported UV–Visible spectra and wavelengths of maximum absorption; (B) comparison of the retention time and UV–Visible spectra characteristics according to commercial standards; (C) reported chromatographic order of elution. Quantification was conducted with standard curves of all-trans-β-carotene, trans-β-apo-8ʹ-carotenal, and all-trans-lutein.
2.8. Statistical analysis
Data represents the mean value of repetitions and their standard error. Total dietary fiber using AOAC 991.43 and AOAC 985.29 was determined in duplicate following the official methodology, whereas the determinations of fiber using the more resent integrated TDF method AOAC 2011.25 were determined in quadruplicate. Likewise, physicochemical analysis, uronic acid, PEM activity, antioxidant and carotenoid determination were determined in triplicate. Analysis of variance (ANOVA) was conducted using JMP statistical version 12.0 (SAS Institute Inc., USA) and mean separation was performed using LSD student t-tests (p < 0.05).
3. Results
3.1. Chemical composition of unprocessed and HHP treated mango pulp
Chemical composition of the unprocessed and HHP treated mango pulp samples are included in . Based on its composition, mangoes used in the study were classified to be at a commercial maturity stage (Ibarra-Garza et al., Citation2015). A slight increase in the concentrations of glucose (3%), fructose (3%), and sucrose (4%) (p < 0.05) resulted from HHP processing.
Table 1. Effect of high hydrostatic pressure on chemical characterization, total phenolic compounds, ascorbic acid, and antioxidant capacity of mango pulp (Mangifera indica L. cv. Tommy atkins) HHP = high hydrostatic pressure; MPa = megapascals.
Tabla 1. Efecto de la alta presión hidrostática sobre la composición química de pulpa de mango (Mangifera indica L. cv. Tommy atkins), incluyendo el contenido de compuestos fenólicos totales, ácido ascórbico y capacidad antioxidante. HHP = alta presión hidrostática; MPa = megapascales
3.2. Effects of HHP processing on dietary fiber
The concentrations of TDF, using the conventional official AOAC fiber analysis methodology (Prosky et al., Citation1985, Citation1994) (), were lower when compared with TDF values obtained with the more recent integrated fiber method (McCleary et al., Citation2012) (). However, results from both fiber analysis methodologies indicated that mango TDF concentrations were not significantly affected (p > 0.05) by the HHP treatment itself ( and ). Even though HHP did not affect TDF concentrations, the fiber methodologies generated different experimental results for the quantification of IDF and SDF. Results obtained with the fiber analysis kit, based on the conventionally used fiber AOAC methodology (shown in ) indicated that IDF and SDF concentrations in unprocessed and HHP-treated mango pulps were not significantly different. However, as shown in (fiber data measured by integrated fiber assay), a significant 22% increase in the SDF concentrations of mango pulp was observed following HHP treatment when compared with the unprocessed treatments. The increase in the SDF fraction was mainly due to the contribution of low-molecular weight soluble dietary fiber (SDFS) sub-fraction, which presented twice the concentrations of the unprocessed mango pulp (. (1). Quantification of the SDFS sub-fraction by HPLC, following the integrated fiber methodology, included NDOs, such as resistant maltodextrins, fructo- and/or galacto-oligosaccharides, and plant cell-wall components with a lower degree of polymerization (between 3–9 monomers), measured by the refractive index detector.
Figure 1. Characterization of dietary fiber contents of mango pulp untreated and treated with high hydrostatic pressure (HHP, 592 MPa for 3min), using two different AOAC official fiber analysis methods. (a) Concentrations of soluble dietary fiber (SDF), insoluble dietary fiber (IDF) and the sum of both as total dietary fiber (TDF), determined by a combination of the AOAC 985.29 (Prosky et al., Citation1985) and AOAC 991.43 (Lee et al., Citation1992) methods. (b.1) Concentrations of SDF, IDF and TDF determined by the integrated fiber analysis method AOAC 2011.25 (McCleary et al., Citation2012); and its graph inset (b) Concentrations of sub-fractions of SDF, which included lower-molecular weight soluble dietary fiber (SDFS) and higher-molecular weight soluble dietary fiber (SDFP), also determined by the fiber analysis method AOAC 2011.25 (McCleary et al., Citation2012). 1Concentrations with different letters for each dietary fiber fraction, and for the same dietary fiber method, indicate that the unprocessed and HHP-treated samples were found to be significantly different (student t-test, p < 0.05).
Figura 1. Caracterización del contenido de fibra de pulpa de mango, tratada y no tratada con alta presión hidrostática (HHP, 592 MPa por 3min), empleando dos métodos oficiales AOAC de análisis de fibra. A) Concentraciones de fibra dietética soluble (SDF), fibra dietética insoluble (IDF) y la suma de ambas expresada como fibra dietética total (TDF), determinadas por una combinación de los métodos AOAC 985.29 (Prosky et al., Citation1985) y AOAC 991.43 (Lee et al., Citation1992). B) Concentraciones de SDF, IDF y TDF determinadas por el método integrado de análisis de fibra AOAC 2011.25 (McCleary et al., Citation2012); y su gráfica insertada B.1) Concentraciones de las sub-fracciones de SDF, que incluyen fibra dietética soluble de bajo peso molecular (SDFS) y fibra dietética soluble de alto peso molecular (SDFP), también determinadas por el método de análisis de fibra AOAC 2011.25 (McCleary et al., Citation2012). 1Las concentraciones con diferentes letras para cada fracción de fibra dietética, y para el mismo método de análisis, indican diferencias significativas (prueba t-Student, p < 0.05) entre las muestras tratadas y no tratadas con HHP
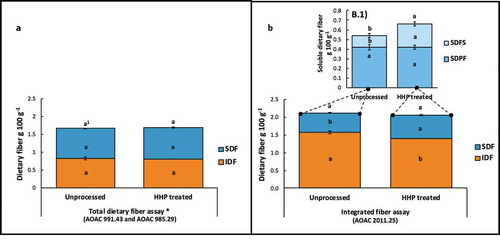
3.3. Pectin methyl esterase (PME) activity of mango pulp and galacturonic acid determination in the low-molecular weight soluble dietary fiber sub-fractions (SDFS)
PME activity of mango pulp increased by 38% after HHP treatment compared with the unprocessed control (). Galacturonic acid content, which is the monomer of pectin chains, was determined in the SFDS sub-fractions to assess the presence of pectin oligosaccharides in that low-molecular weight SDF sub-fraction. HPLC methodology used for the quantification of NDOs, as part of the integrated fiber method, considers only those derived from resistant starch and other plant cell wall components that contain sugar monomers, but not those derived from pectin. Results shown in indicated that galacturonic acid was detected in the SDFS sub-fractions from both treatments (unprocessed and HHP-processed mango pulps), suggesting the presence of galacturonic oligosaccharides. As mentioned, the chromatographic procedure used in the integrated fiber method does not allow for their quantification. Thus, even though colorimetric methods can be less accurate than chromatographic ones, galacturonic acid spectrophotometric quantification was conducted to estimate the levels of pectin-derived oligosaccharides (shown in ). However, concentrations were found to be non-significantly different between the unprocessed and HHP-treated mango pulps (p = .1597).
Table 2. Pectin methyl esterase (PME) activities of unprocessed and high hydrostatic pressure (HHP) processed mango pulp and of galacturonic acid contents of their low-molecular weight dietary soluble fiber (SDFS) sub-fractions.
Tabla 2. Actividad de la pectin metil esterasa (PME) en la pulpa de mango tratada y no tratada con alta presión hidrostática (HHP) y contenido de ácido galacturónico en las sub-fracciones de su fibra dietética soluble de bajo peso molecular (SDFS)
3.4. Effect of HHP processing on total phenolics, total ascorbic acid, and antioxidant capacity (ORAC value) of mango pulp
Preservation of the initial levels of total phenolic compounds, vitamin C, and antioxidant activity of mango pulp was achieved following HHP treatment. As shown in , no significant differences (p > 0.05) were observed between the unprocessed and HHP-treated mango pulps.
3.5. Carotenoid analysis
A representative HPLC chromatogram for mango pulp cv. Tommy Atkins is shown in , containing 21 peaks with the characteristic carotenoid UV–Vis spectrum. Chemical identities for each peak were assigned based on the criteria summarized in . As observed, the identities were more certain for peaks that were quantified with existing analytical standards and that agreed with previously reported analytical data. Mango carotenoid profile contained 6 xanthophylls and 10 carotenes. The main mango pulp carotenoids, including their percent contribution to the total concentration (shown in parenthesis) were all-trans-β-carotene (50%), all-trans-α-carotene (7%), all-trans-lutein (5%), 9-cis-α-carotene (4%) and all-trans-violaxanthin (2%).
Table 3. Carotenoid concentrations of mango pulp (cv. Tommy Atkins) quantified by HPLC-DAD in unprocessed and high hydrostatic pressure (HHP) treated samples.
Tabla 3. Concentración de carotenoides de la pulpa de mango (cv. Tommy Atkins) tratada y no tratada con alta presión hidrostática (HHP) cuantificada por HPLC-DAD
Figure 2. Mango pulp (cv. Tommy Atkins) carotenoid chromatographic profiles (HPLC-DAD, collected at 450 nm) as affected by high hydrostatic pressure (HHP) processing (592 MPa for 3 min).
Figura 2. Efecto del procesamiento con alta presión hidrostática (HHP, 592 MPa por 3 min) sobre el perfil cromatográfico de carotenoides de la pulpa de mango (cv. Tommy Atkins) determinado por HPLC-DAD a 450 nm
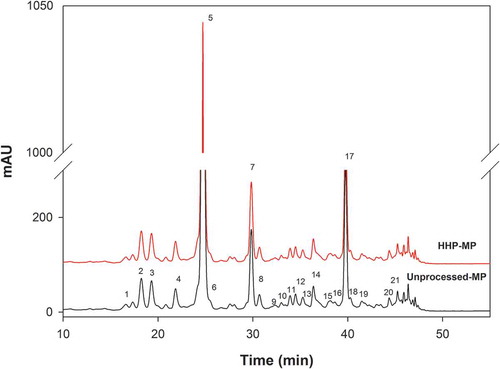
3.5.1. Effect of high hydrostatic pressure (HHP) on carotenoid contents in mango pulp
As shown in and , neither the characteristic carotenoid profile nor the concentrations of unprocessed mango pulp were significantly (p < 0.05) affected by HHP processing. Concentrations of the main pro-vitamin-A carotenoid, all-trans-β-carotene (9.14 ± 0.72 mg per kg), were therefore also not affected by HHP processing ().
In order to visualize results, carotenoids were also classified based on their chemical structures in two major groups that included carotenes and xanthophylls. shows the concentrations of the latter two groups, and their sum, which resulted in the total carotenoids (shown in mg Kg−1 and in retinol activity equivalents (RAE). RAE calculations were conducted considering the concentrations of the mango pro-vitamin A carotenoids, which included all-trans-β-cryptoxanthin, 13-cis-β-carotene, 15-cis-β-carotene, all-trans- α-carotene, 9-cis-α-carotene, all-trans-β-carotene, and 9-cis-β-carotene. Since the HHP treatment did not significantly affect the concentrations of the two main carotenoid groups, it did not modify the pro-vitamin A concentrations of mango pulp ().
Figure 3. Effect of high hydrostatic pressure (HHP) treatment on the concentrations of mango pulp (Mangifera indica L. cv. Tommy Atkins) carotenoids grouped in two major groups (xanthophylls and carotenes, shown in yellow and orange colors, respectively), and on their sum as total carotenoids (expressed in mg kg−1) and in retinol activity equivalents (RAE), shown in red and green colors, respectively. 1Similar letters for the same carotenoid group indicated that the unprocessed and HHP-processed mango pulps were not significantly different by the LSD test (p > 0.05). RAE = Retinol activity equivalents; HHP = High hydrostatic pressure, processed at 592 MPa for 3 min. Data represents the mean ± standard error of three independent determinations.
Figura 3. Efecto del tratamiento con alta presión hidrostática (HHP) sobre la concentración de carotenoides de la pulpa de mango (Mangifera indica L. cv. Tommy Atkins) clasificados en los grupos principales (xantófilas y carotenos, mostrados en color amarillo y naranja, respectivamente), y la suma mostrada como carotenoides totales (expresados en mg kg−1) y actividad en equivalentes de retinol (RAE), mostrados en color rojo y verde, respectivamente. 1Letras similares para el mismo grupo de carotenoids indican que la muestra de mango procesada y no procesada con HHP no son significativamente diferentes por la prueba LSD (p > 0.05). RAE = Actividad en equivalentes de retinol; HHP = Alta presión hidrostática, procesado a 592 MPa por 3 min. Los datos representan el promedio ± el error estándar de tres determinaciones independientes
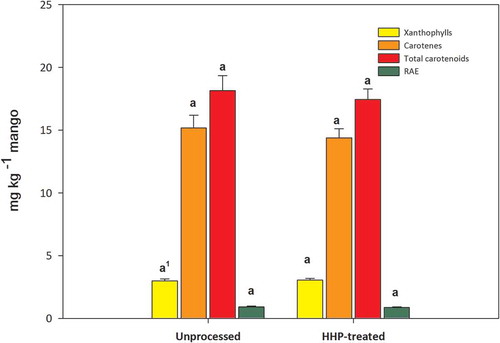
4. Discussion
4.1. Insights into mango pulp dietary fibers
Concentrations of TDF are shown in . TDF levels were not significantly (p > 0.05) affected by the HHP treatment, although different fiber analysis methodologies were used for their determination. However, concentrations of TDF measured by the conventional fiber methodologies (Prosky et al., Citation1985, Citation1994) were lower than those obtained by the integrated fiber assay (McCleary et al., Citation2012). The newer methodology introduced different enzymatic conditions and incubation at 37°C to simulate human digestion. In agreement with our findings, Garcia-Amezquita, Tejada-Ortigoza, Heredia-Olea, Serna-Saldívar, and Welti-Chanes (Citation2018) reported higher TDF concentrations for mango peel and pulp (cultivar Ataulfo) with the integrated fiber method when compared with the conventional fiber methodology. Similarly, other authors have shown higher TDF concentrations for other plant matrices when comparing both methodologies (McCleary, Citation2007; Prosky et al., Citation1985). These observations might be attributed to improvements in the breakdown of starch and plant cell walls introduced by the newer methodology, which facilitated the extraction of the dietary fibers. The confirmation that the mango pulp itself, regardless HHP treatment, contained 1.3-fold higher TDF concentrations was a desirable result with a relevant clinical implication, since a portion of 165 g (1-cup) of mango can contribute 3.5 g of TDF to the human diet. This amount represents 14% and 9% of the recommended daily allowance (RDA) of dietary fiber for adult females and males in a 2000 kcal intake basis, respectively (Trumbo, Yates, Schlicker, & Poos, Citation2001).
4.2. Insights into HHP induced inter-conversions of dietary fibers and their possible clinical impact on healthy subjects and patients with cardiometabolic diseases
Although the HHP treatment did not affect TDF concentrations, quantification of IDF and SDF by the integrated fiber method provided new insight into their inter-conversions caused by the pressure treatment (). SDF increased (by 22%) in the HHP treated mango pulps in reference to the unprocessed controls, an increment that was caused by higher concentrations of NDOs, such as resistant maltodextrins and other oligosaccharides generated by cell-wall breakdown (quantified as SDFS, shown in ). Therefore, our results indicated that HHP treatments generated higher levels of soluble fibers with a lower-degree of polymerization according with the integrated method (>3 and up to 9 monomers, NDOs by definition), from a list of possible oligosaccharides such as resistant maltodextrins, fructo- and galacto-oligosaccarides and other breakdown products of cell walls (McCleary et al., Citation2012). The integrated method does not quantify galacturonic oligosaccharides derived from pectin. Therefore, the presence of galacturonic acid in the SDFS sub-fraction was measured in the present study to assess the presence of pectin-derived oligosaccharides within the SDF fraction. Results from our spectrophotometric assessment confirmed the presence of pectin-derived oligosaccharides (), which allowed us to identify an important limitation of the official integrated dietary fiber method AOAC 2011.25 (McCleary et al., Citation2012). Although the integrated method allowed us to observe fiber inter-conversions and to obtain a more clinically relevant quantification of fibers, based on our observations we would recommend further improvements to the integrated fiber method to include quantification of other NDOs by HPLC, particularly those derived from pectin. The quantification of galacturonic oligosaccharides can represent a significant improvement for the SDF quantification of pectin containing fruits, such as mango.
Nevertheless, improvements in the analysis of dietary fiber and dietary fiber sub-fractions conducted in the present study allowed for a deeper explanation of the observed clinical effects after HHP treatment with respect to unprocessed mango as shown the Supplementary Data Figure S1, in which several subjects presented a high glycemic index response after ingestion of unprocessed mango pulp, while none of the subjects did after ingestion of the HHP-processed mango pulp (Elizondo-Montemayor et al., Citation2015). HHP treatment not only increased the SDF, but also doubled the amount of low molecular weight soluble dietary fiber (SDFS) (). The increased SDF after HHP treatment of mango might have an important clinical impact. Although not evaluated in this study, SDF has been shown to delay gastric emptying and to slower glucose absorption rate both in healthy subjects and in patients with type 2 diabetes (Chen et al., Citation2016). Likewise, SDF has been associated with satiety induction in humans by modulating the gut microbiome and the neurotransmitters and hormones that act directly on the gut-brain-axis (Zhao et al., Citation2018). In addition to postprandial glucose levels, other cardiometabolic risk factors have also been shown to be responsive to SDF supplementation in the diet. SDF consumption in patients with type 2 diabetes has demonstrated to decrease postprandial blood glucose and insulin levels (Chen et al., Citation2016; Yao et al., Citation2014), as well as fasting blood glucose, triglycerides, and LDL cholesterol levels (Yao et al., Citation2014).
4.3. Insights into HHP induced pectin methyl esterase (PME) activation and its effect on pectin structural changes
In addition to the inter-conversion of the soluble fiber sub-fractions induced by the HHP treatment of mango pulp, we also observed an increase in PME activity and the presence of galacturonic acid in the SDFS sub-fractions (). In agreement with our results, an increase in PME activity after HHP has been described in other fruit pulps and juices, such as apple juice at 650MPa/25°C/10 min (Baron, Dénes, & Durier, Citation2006), peach juice at 400MPa/25°C/15 min (Rao et al., Citation2014), mango at 300/15min, 400/5min and 500MPa/2.5min (Liu, Wang, Li, Bi, & Liao, Citation2014), carrot at 400–1,000MPa/2min (Sun, Kang, Chen, Liao, & Hu, Citation2019), and capsicum pulp at 100-300MPa/25°C/2 min (Castro, Loey, Saraiva, Smout, & Hendrickx, Citation2006).
Our results showed that the activation of pectic enzymes by HHP, particularly PME, generated the de-methylated pectin substrate for polyglucoronidase (PG) to act upon and cause pectin hydrolysis. HHP treatments have been reported to cause PG activation in some cultivars of persimmon fruit (Rodríguez-Garayar et al., Citation2017). Previous studies have also reported stimulation of other hydrolytic enzymes on IDF in response to HHP, such as xylanase (Oliveira, Figueiredo, Evtuguin, & Saraiva, Citation2012), xylosidase (Cao et al., 2106), arabinofuranosidases [34], and methyl glucoronosidases (Houben, Citation2013). In addition, the activation of hydrolytic enzymes on both IDF and SDF resulted in increased production of NDOs, as shown in . The observed degradation of IDF and SDF into SDFS, therefore, yielded sugar derivatives for microflora fermentation, which are known to be clinically relevant since microflora fermentation produces short chain fatty acids that can reduce cholesterol synthesis by inactivating the hydroxymethyl-glutaryl-Co-A-synthase (Hara, Haga, Aoyama, & Kiriyama, Citation1999). This modification in the mango fiber sub-fractions might highly improve health-promoting properties, particularly regarding glucose and cholesterol homeostasis.
The observed activation of PME by the HHP treatment, in addition to providing substrates for further pectin hydrolysis, caused relevant structural modifications in the pectin chains. De-methylated pectins have been shown to contain more free-carboxyl groups which in turn are available to form complexes with calcium and other metal ions, changing the rheology of the food matrix (Christiaens et al., Citation2016; Willats, Knox, & Mikkelsen, Citation2006). In the mango pulp matrix of this study, HHP treatment increased the viscosity and hydration properties, as previously reported by our group (Elizondo-Montemayor et al., Citation2015). In addition, the higher viscosity induced by HHP treatment decreased the glucose absorption rate into the bloodstream, reduced the glycemic index response and maintained more stable circulating blood glucose levels in healthy adults (Elizondo-Montemayor et al., Citation2015). Among the nutritional recommendations to maintain glucose homeostasis both in healthy subjects and in people with diabetes, the selection of low glycemic index carbohydrates sources over those with high glycemic index is referenced (Atkinson, Foster-Powell, & Brand-Miller, Citation2008). Considering further potential health benefits of HHP processing of foods in health promotion and disease prevention, it has been reported that the consumption of high viscosity fruits can decrease cardiometabolic risk factors such as total and LDL cholesterol levels in people with obesity and insulin resistance (Vuksan et al., Citation2000). Likewise, a recent meta-analysis including adults with overweight or obesity concluded that viscous fiber supplementation can significantly lower systolic and diastolic blood pressure (Khan et al., Citation2018).
The observed HHP-induced modifications on mango pectins resulted in a relevant functional modification, a reduction of the glycemic index. Among the soluble fibers, pectin has been described as the most abundant subtype in the majority of tropical fruits (Mahattanatawee et al., Citation2006). In addition, pectin intake has shown a decrease in gastric emptying by two-fold in healthy subjects, which in turn has been associated to a reduced tendency to over-eat and thus, with potential to prevent overweight or obesity (Sanaka, Yamamoto, Anjiki, Nagasawa, & Kuyama, Citation2007). Likewise, patients with obesity have reported an increased sensation of satiety associated to a reduced gastric emptying rate after consumption of meals supplemented with pectin (Di Lorenzo, Williams, Hajnal, & Valenzuela, Citation1988). Moreover, in mildly hypercholesterolemic subjects, supplementation with pectin from apples and citrus peel was shown to significantly reduce LDL-cholesterol levels (Brouns et al., Citation2012). Our results show that HHP treatment modifies pectin structures of mango pulp, which might potentiate the health benefits associated with pectin intake in healthy subjects, and based on previous reports, in patients with obesity, diabetes, hypercholesterolemia or the metabolic syndrome as well.
4.4. Insights into the relevance of the retention of phenolic compounds, vitamin C, antioxidant capacity, and carotenoids in the mango pulps processed by high-hydrostatic pressure (HHP)
Total phenolic and vitamin C concentrations, as well as the antioxidant capacity of the HHP treated mango pulps were not altered when compared to the unprocessed samples. Similar results have been reported for mango cv. Amrapali, in which 85%, 92% and 90% of the ascorbic acid, total phenolics and antioxidant capacity (DPPH value) were retained, respectively, after HHP processing (Kaushik, Kaur, Rao, & Mishra, Citation2014). Likewise, in other plant foods such as strawberry, blackberry, litchi, and longan, the total phenolic content was not affected by HHP processing (Patras, Brunton, Da Pieve, & Butler, Citation2009; Prasad, Yang, Yi, Zhao, & Jiang, Citation2009a; Prasad, Yang, Zhao, Wang, Chen, & Jiang, Citation2009b). Diets rich in phenolic compounds have been associated with prevention of cancer (Nemec et al., Citation2017), cardiovascular diseases, and type 2 diabetes (Xiao, Citation2016). In the present study, the natural content of polyphenols was not modified after HHP treatment of mango pulp, a phenolics rich fruit, therefore mango might be included as a part of a health-promoting diet even after HHP processing. Venancio et al. (Citation2018) reported beneficial effects of the consumption of mango phytochemicals on the amelioration of constipation and chronic inflammation in humans; in their clinical study, the authors administered an equivalent amount of fiber to subjects and concluded that mango phytonutrients such as phenolic compounds may also contribute to the observed beneficial health effects beyond fiber. The mango matrix consumed in our clinical study was also rich in both fiber and polyphenolics; and although our work focused on glycemic responses and fiber characterization, it is worth mentioning that mango phyto/micronutrients are also relevant for healthy metabolisms and microbiomes (Venancio et al., Citation2018).
Retention of fresh mango fruit concentrations of vitamin C after HHP processing () was also a desirable outcome for the use of pressure for the pasteurization of foods. Other studies have also reported no changes in vitamin C contents following the application of HHP-treatments of orange juice (Butz et al., Citation2003) and guava nectar (Yen & Lin, Citation1996), while small losses (6%) were reported in strawberry puree processed at 600 MPa for 15 min (Patras et al., Citation2009).
Regarding antioxidant capacity (ORAC) value, our results were also in agreement with previous studies that have evaluated the stability of ORAC values during processing in food matrixes such as tomato pulp (Butz et al., Citation2002), orange, apple, peaches, mixed citrus juice, carrots, tomatoes, raspberries (Butz et al., Citation2003), and broccoli (McInerney, Seccafien, Stewart, & Bird, Citation2007).
Many of the clinical benefits associated to fruits and vegetable consumption are explained by the antioxidant activity of the vitamins, minerals, and nutraceuticals that they contain (Kaur & Kapoor, Citation2001). Among the water-soluble vitamins, vitamin C is characterized for its strong ability to protect against oxidative stress (Padayatty et al., Citation2003). Increased oxidative stress has been associated with cancer, cardiovascular diseases, diabetes, and obesity, while an inverse relationship between vitamin C levels and the incidence of these diseases has been reported (Pearson et al., Citation2017). Moreover, supplementation with vitamin C has shown to significantly reduce blood glucose levels and glycosylated hemoglobin in patients with type 2 diabetes (Dakhale, Chaudhari, & Shrivastava, Citation2011). Although some fruits and vegetables are excellent sources of vitamin C, high losses of this vitamin are frequently induced after food processing because of its water-solubility and instability (Rameen, Citation2015). Our results show that after HHP treatment, vitamin C content in mango pulp remained constant, maintaining the health benefits of mango fruit associated to this vitamin. HHP processing might thus be considered a suitable food processing technology for mango and possible for other vitamin C rich foods.
In addition, mango has proven to be an excellent source of pro-vitamin A carotenoids. One portion of processed or unprocessed mango pulp (355 g which correspond to one mango) can provide 47% to 37% of the daily vitamin A recommended dietary allowance (RDA) for women and men, respectively (Trumbo et al., Citation2001). Our results agree with prior authors that have evaluated the HHP processing stability of mango carotenoids of a different cultivar (cv. Tainong) (Liu et al., Citation2014). Other authors have also documented stability in other fruits such as persimmon, orange, avocado, and papaya, among others de (de Ancos, Gonzalez, & Cano, Citation2000; Escobedo-Avellaneda, Gutiérrez-Uribe, Valdez-Fragoso, Torres, & Welti-Chanes, Citation2015; Hernández-Brenes, Ramos-Parra, Jacobo-Velázquez, Villarreal-Lara, & Díaz de la Garza, Citation2013).
The clinical impact of carotenoids on health and disease has been widely described. Carotenoids have been shown to protect against age-related macular degeneration and cataracts (El-Sayed, Ghareeb, Talat, & Sarhan, Citation2013), cancer (Kucuk et al., Citation2001) and cardiovascular diseases (Riccioni et al., Citation2010). These functional properties have been attributed mainly to the antioxidant capacity of carotenoids. Noteworthy, in a recent 10-year prospective cohort study including 37,846 participants, consumption of diets high in β-carotene and α-carotene were found to significantly decrease the incidence of type 2 diabetes mellitus in healthy adults (Sluijs et al., Citation2015). Our results showed that HHP treatment preserved the contents of the different carotenoids of mango (). Therefore, HHP preservation of the carotenoid content of mango and potentially of other foods rich in carotenoids might have a relevant clinical impact by decreasing the incidence of type 2 diabetes and in reducing the cardiometabolic risk factors in healthy subjects, as well as in those patients who already present such diseases (as proposed in ).
Figure 4. Suggested Mechanism of effects of High Hydrostatic Pressure (HHP) Processing on dietary fiber and nutraceutical composition of mango and associated clinical outcomes. Green outlined boxes, green filled boxes, and green colored text indicate results are from the present study. Brown outlined boxes and brown colored text indicate results are from previous studies (Cao et al., Citation2011; Houben, Citation2013; Oliveira et al., Citation2012). (a) HHP processing of fruit pulp increases the activity of pectinases such as pectin methyl esterase (PME) and polyglucoronidase (PG), therefore increasing low molecular weight soluble fiber, and resulting in increased viscosity. (b1) Once ingested by healthy subjects, higher viscosity of the food matrix decreases glucose absorption rate into the bloodstream, reducing glycemic index and maintaining more stable circulating blood glucose levels. (c) HHP processing maintains initial content of relevant vitamins and nutraceuticals such as carotenoids, vitamin C, and phenolic compounds. (b2) Besides promoting glucose homeostasis, other clinical benefits associated to soluble fiber, vitamins and nutraceuticals contained in mango have been previously reported (Brouns et al., Citation2012; El-Sayed et al., Citation2013; Ho et al., Citation2016; Kucuk et al., Citation2001; Riccioni et al., Citation2010; Sluijs et al., Citation2015; Vuksan et al., Citation2000).
Figura 4. Mecanismo sugerido para los efectos del procesamiento con Alta Presión Hidrostática (APH) sobre la fibra dietética, la composición nutracéutica de mango y los efectos clínicos asociados. Los cuadros contorneados en verde, los cuadros con relleno verde y el texto en color verde indican que los resultados provienen del presente estudio. Los cuadros delineados en marrón y el texto en color marrón indican que los resultados son de estudios previos (Cao et al., Citation2011; Houben, Citation2013; Oliveira et al., Citation2012). (A) El procesamiento de la pulpa de la fruta con HHP incrementa la actividad de pectinasas como la pectin metil esterasa (PME) y poligalacturonidasa (PG), incrementando la fibra dietética soluble de bajo peso molecular, y resultando en un incremento en la viscosidad. (B1) Una vez ingerida por sujetos saludables, el incremento en viscosidad de la matriz alimenticia disminuye la velocidad de absorción de glucosa en el torrente sanguíneo, lo cual reduce el índice glicémico y mantiene los niveles de glucosa en sangre circulante más estable. (C) El procesamiento con HHP retiene el contenido inicial de vitaminas y nutacéuticos relevantes como los carotenoides, vitamina C, y compuestos fenólicos. (B2) Además de promover la homeostasis de la glucosa, se han reportado previamente otros beneficios clínicos asociados con la fibra soluble, vitaminas y nutracéuticos presentes en el mango (Brouns et al., Citation2012; El-Sayed et al., Citation2013; Ho et al., Citation2016; Kucuk et al., Citation2001; Riccioni et al., Citation2010; Sluijs et al., Citation2015; Vuksan et al., Citation2000)
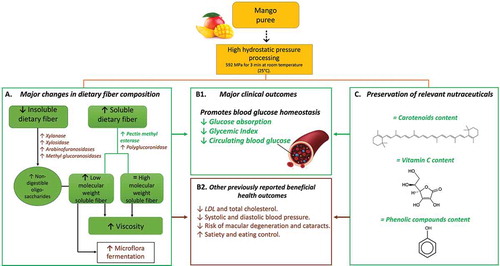
The present work contains several strength points, such as the use of improvements in the methodology for the characterization of the mango fibers, which gave us insight in to the HHP-induced modifications, which prior authors did not observe in studies that used conventional methodologies. Characterization of the stability of relevant mango micronutrients, with high resolution of individual components, also strengthened the reliability of the conclusions of this work on the vitamin and phytonutrient contents after HHP processing. Although relevant advances were made on the characterization of mango fibers, further improvements in the methodology were identified, particularly the need to include the quantification of pectin oligosaccharides in the integrated official AOAC method. The present work also generated important observations for future research, particularly on the understanding of structural changes and health-related properties of HHP-modified mango pectins.
5. Conclusions
HHP treatment at commercial conditions (592 MPa for 3 min) increased the soluble fiber dietary fraction. The integrated fiber method assay allowed for a more accurate quantification of low- and high- molecular weight soluble fiber sub-fractions, when compared with the traditional fiber assay. HHP treatment increased PME activity, as well as the contents of NDOs of mango when contrasted with unprocessed mango. The increases of dietary fiber inter-conversion, especially of the SDFS sub-fraction, offered insight to understand the relevant outcome of a lower glycemic index of healthy adults that consumed HHP-treated of mango pulp. Increases of PME activity induced by pressurization and presence of pectin oligosaccharides in the soluble fiber sub-fraction also offered insights into the rheological changes of the mango matrix.
In addition, HHP treatment of mango pulp resulted in preservation of the total carotenoid, ascorbic acid, phenolic contents, and antioxidant capacity, which could also enhance health-promoting properties both for healthy subjects and for patients with diabetes mellitus, obesity and other conditions characterized by oxidative stress. Furthermore, the modifications of pectin structures as a result of the HHP treatment of mango, might benefit not only healthy subjects but also populations with diabetes, obesity, hypercholesterolemia, and metabolic syndrome as well. Future studies should explore if patients with alterations in glucose and cholesterol homeostasis might also benefit from consuming HHP treated fruit in contrast to unprocessed fruit.
Supplemental Material
Download TIFF Image (26.2 MB)Acknowledgments
We also are grateful to M.Sc. Aratza M. Rosas-Pérez for her technical support for vitamin C analyses.
Disclosure statement
No potential conflict of interest was reported by the authors.
Supplementary material
Supplemental data for this article can be accessed Publisher’s website.
Additional information
Funding
References
- Atkinson, F. S., Foster-Powell, K., & Brand-Miller, J. C. (2008). International tables of glycemic index and glycemic load values: 2008. Diabetes Care, 31(12), 2281–2283. doi:10.2337/dc08-1239
- Baron, A., Dénes, J. M., & Durier, C. (2006). High-pressure treatment of cloudy apple juice. LWT - Food Science and Technology, 39, 1005–1013. doi:10.1016/j.lwt.2006.02.016
- Bindels, L. B., Neyrinck, A. M., Salazar, N., Taminiau, B., Druart, C., Muccioli, G. G., … Delzenne, N. M. (2015). Non digestible oligosaccharides modulate the gut microbiota to control the development of leukemia and associated cachexia in mice. PLoS One, 10(6), e0131009. doi:10.1371/journal.pone.0131009
- Brouns, F., Theuwissen, E., Adam, A., Bell, M., Berger, A., & Mensink, R. P. (2012). Cholesterol-lowering properties of different pectin types in mildly hyper-cholesterolemic men and women. European Journal of Clinical Nutrition, 66(5), 591–599. doi:10.1038/ejcn.2011.208
- Bruno-Barcena, J. M., & Azcarate-Peril, M. A. (2015). Galacto-oligosaccharides and colorectal cancer: Feeding our intestinal probiome. Journal of Functional Foods, 12, 92–108. doi:10.1016/j.jff.2014.10.029
- Butz, P., Edenharder, R., Fernandez Garcia, A., Fister, H., Merkel, C., & Tauscher, B. J. F. (2002). Changes in functional properties of vegetables induced by high pressure treatment. Food Research International, 35(2–3), 295–300. doi:10.1016/S0963-9969(01)00199-5
- Butz, P., Fernández Garcı́a, A., Lindauer, R., Dieterich, S., Bognár, A., & Tauscher, B. (2003). Influence of ultra high pressure processing on fruit and vegetable products. Journal of Food Engineering, 56(2), 233–236. doi:10.1016/S0260-8774(02)00258-3
- Cao, X., Zhang, Y., Zhang, F., Wang, Y., Yi, J., & Liao, X. (2011). Effects of high hydrostatic pressure on enzymes, phenolic compounds, anthocyanins, polymeric color and color of strawberry pulps. Journal of the Science of Food and Agriculture, 91(5), 877–885. doi:10.1002/jsfa.v91.5
- Castro, S. M., Loey, A. V., Saraiva, J. A., Smout, C., & Hendrickx, M. (2006). Inactivation of pepper (Capsicum annuum) pectin methylesterase by combined high-pressure and temperature treatments. Journal of Food Engineering, 75(1), 50–58. doi:10.1016/j.jfoodeng.2005.03.050
- Chen, C., Zeng, Y., Xu, J., Zheng, H., Liu, J., Fan, R., … Wang, J. (2016). Therapeutic effects of soluble dietary fiber consumption on type 2 diabetes mellitus. Experimental and Therapeuctic Medicine, 12(2), 1232–1242. doi:10.3892/etm.2016.3377
- Christiaens, S., Van Buggenhout, S., Houben, K., Jamsazzadeh Kermani, Z., Moelants, K. R., Ngouemazong, E. D., … Hendrickx, M. E. (2016). Process-structure-function relations of pectin in food. Critical Reviews in Food Science and Nutrition, 56(6), 1021–1042. doi:10.1080/10408398.2012.753029
- Dakhale, G. N., Chaudhari, H. V., & Shrivastava, M. (2011). Supplementation of vitamin C reduces blood glucose and improves glycosylated hemoglobin in type 2 diabetes mellitus: A randomized, double-blind study. Advance in Pharmacological Sciences, 2011, 195271. doi:10.1155/2011/195271
- de Ancos, B., Gonzalez, E., & Cano, M. P. (2000). Effect of high-pressure treatment on the carotenoid composition and the radical scavenging activity of persimmon fruit purees. Journal of Agricultural and Food Chemistry, 48(8), 3542–3548. doi:10.1021/jf990911w
- Di Lorenzo, C., Williams, C. M., Hajnal, F., & Valenzuela, J. E. (1988). Pectin delays gastric emptying and increases satiety in obese subjects. Gastroenterology, 95(5), 1211–1215. doi:10.1016/0016-5085(88)90352-6
- Elizondo-Montemayor, L., Hernandez-Brenes, C., Ramos-Parra, P. A., Moreno-Sanchez, D., Nieblas, B., Rosas-Perez, A. M., & Lamadrid-Zertuche, A. C. (2015). High hydrostatic pressure processing reduces the glycemic index of fresh mango puree in healthy subjects. Food & Function, 6(4), 1352–1360. doi:10.1039/C4FO01005A
- El-Sayed, M. M., Ghareeb, D. A., Talat, H. A., & Sarhan, E. M. (2013). High fat diet induced insulin resistance and elevated retinol binding protein 4 in female rats; treatment and protection with Berberis vulgaris extract and vitamin A. Pakistan Journal of Pharmaceutical Science, 26(6), 1189–1195.
- Escobedo-Avellaneda, Z., Gutiérrez-Uribe, J., Valdez-Fragoso, A., Torres, J. A., & Welti-Chanes, J. (2015). High hydrostatic pressure combined with mild temperature for the preservation of comminuted orange: Effects on functional compounds and antioxidant activity. Food and Bioprocess Technology, 8(5), 1032–1044. doi:10.1007/s11947-014-1465-9
- Garcia-Amezquita, L. E., Tejada-Ortigoza, V., Heredia-Olea, E., Serna-Saldívar, S. O., & Welti-Chanes, J. (2018). Differences in the dietary fiber content of fruits and their by-products quantified by conventional and integrated AOAC official methodologies. Journal of Food Composition and Analysis, 67, 77–85. doi:10.1016/j.jfca.2018.01.004
- Gillespie, K. M., & Ainsworth, E. A. (2007). Measurement of reduced, oxidized and total ascorbate content in plants. Nature Protocols, 2, 871–874. doi:10.1038/nprot.2007.101
- Hagerman, A. E., & Austin, P. J. (1986). Continuous spectrophotometric assay for plant pectin methyl esterase. Journal of Agricultural and Food Chemistry, 34, 440–444. doi:10.1021/jf00069a015
- Hara, H., Haga, S., Aoyama, Y., & Kiriyama, S. (1999). Short-chain fatty acids suppress cholesterol synthesis in rat liver and intestine. Journal of Nutrition, 129(5), 942–948. doi:10.1093/jn/129.5.942
- Hernández-Brenes, C., Ramos-Parra, P. A., Jacobo-Velázquez, D. A., Villarreal-Lara, R., & Díaz de la Garza, R. I. (2013). High hydrostatic pressure processing as a strategy to increase carotenoid contents of tropical fruits. in tropical and subtropical fruits: Flavors, color, and health benefits. ACS Symposium Series, 1129, 29–42.
- Hernandez-Carrion, M., Vazquez-Gutierrez, J. L., Hernando, I., & Quiles, A. (2014). Impact of high hydrostatic pressure and pasteurization on the structure and the extractability of bioactive compounds of persimmon “Rojo Brillante”. Journal of Food Science, 79(1), C32–38. doi:10.1111/jfds.2014.79.issue-1
- Ho, H. V., Sievenpiper, J. L., Zurbau, A., Blanco Mejia, S., Jovanovski, E., Au-Yeung, F., … Vuksan, V. (2016). The effect of oat beta-glucan on LDL-cholesterol, non-HDL-cholesterol and apoB for CVD risk reduction: A systematic review and meta-analysis of randomised-controlled trials. British Journal of Nutrition, 116(8), 1369–1382. doi:10.1017/S000711451600341X
- Houben, K. (2013). Thermal and high-pressure stability of pectinmethylesterase, polygalacturonase, β-galactosidase and α-arabinofuranosidase in a tomato matrix: Towards the creation of specific endogenous enzyme populations through processing. Food and Bioprocess Technology, 6(12), 3368–3380. doi:10.1007/s11947-012-0984-5
- Ibarra-Garza, I. P., Ramos-Parra, P. A., Hernández-Brenes, C., & Jacobo-Velázquez, D. A. (2015). Effects of postharvest ripening on the nutraceutical and physicochemical properties of mango (Mangifera indica L. cv Keitt). Postharvest Biology and Technology, 103, 45–54. doi:10.1016/j.postharvbio.2015.02.014
- Jacobo-Velázquez, D. A., Ramos-Parra, P. A., & Hernández-Brenes, C. (2010). Survival analysis applied to the sensory shelf‐life dating of high hydrostatic pressure processed avocado and mango pulps. Journal of Food Science, 75(6), S286–S291. doi:10.1111/j.1750-3841.2010.01656.x
- Kaur, C., & Kapoor, H. C. (2001). Antioxidants in fruits and vegetables – The millennium’s health. International Journal of Food Science and Technology, 36(7), 703–725. doi:10.1046/j.1365-2621.2001.00513.x
- Kaushik, N., Kaur, B. P., Rao, P. S., & Mishra, H. N. (2014). Effect of high pressure processing on color, biochemical and microbiological characteristics of mango pulp (Mangifera indica cv. Amrapali). Innovative Food Science and Emerging Technologies, 22, 40–50. doi:10.1016/j.ifset.2013.12.011
- Khan, K., Jovanovski, E., Ho, H. V. T., Marques, A. C. R., Zurbau, A., Mejia, S. B., … Vuksan, V. (2018). The effect of viscous soluble fiber on blood pressure: A systematic review and meta-analysis of randomized controlled trials. Nutrition, Metabolism and Cardiovascular Diseases, 28(1), 3–13. doi:10.1016/j.numecd.2017.09.007
- Kintner, P. K., & Van Buren, J. P. (1982). Carbohydrate interference and its correction in pectin analysis using the m-hydroxydiphenyl method. Journal of Food Science, 47(3), 756–759. doi:10.1111/jfds.1982.47.issue-3
- Kucuk, O., Sarkar, F. H., Sakr, W., Djuric, Z., Pollak, M. N., Khachik, F., … Wood, D. P., Jr. (2001). Phase II randomized clinical trial of lycopene supplementation before radical prostatectomy. Cancer Epidemiology Biomarkers and Prevention, 10(8), 861–868.
- Lee, S. C., Prosky, L., & DeVries, J. W. (1992). Determination of total, soluble and insoluble dietary fiber in foods. Enzymatic-gravimetric method, MES-TRIS buffer: Collaborative study. Journal of AOAC International, 75, 395–416. doi:10.1093/jaoac/75.3.395
- Liu, F., Wang, Y., Li, R., Bi, X., & Liao, X. (2014). Effects of high hydrostatic pressure and high temperature short time on antioxidant activity, antioxidant compounds and color of mango nectars. Innovative Food Science and Emerging Technologies, 21, 35–43. doi:10.1016/j.ifset.2013.09.015
- Mahattanatawee, K., Manthey, J. A., Luzio, G., Talcott, S. T., Goodner, K., & Baldwin, E. A. (2006). Total antioxidant activity and fiber content of select Florida-grown tropical fruits. Journal of Agricultural and Food Chemistry, 54(19), 7355–7363. doi:10.1021/jf060566s
- Mateos-Aparicio, I., Mateos-Peinado, C., & Rupérez, P. (2010). High hydrostatic pressure improves the functionality of dietary fibre in okara by-product from soybean. Innovative Food Science and Emerging Technologies, 11(3), 445–450. doi:10.1016/j.ifset.2010.02.003
- McCleary, B. V. (2007). An integrated procedure for the measurement of total dietary fibre (including resistant starch), non-digestible oligosaccharides and available carbohydrates. Analytical and Bioanalytical Chemistry, 389(1), 291–308. doi:10.1007/s00216-007-1389-6
- McCleary, B. V., DeVries, J. W., Rader, J. I., Cohen, G., Prosky, L., Mugford, D. C., & Okuma, K. (2012). Determination of insoluble, soluble, and total dietary fiber (CODEX definition) by enzymatic-gravimetric method and liquid chromatography: Collaborative study. Journal of AOAC International, 95(3), 824–844. doi:10.5740/jaoacint.CS2011_25
- McInerney, J. K., Seccafien, C. A., Stewart, C. M., & Bird, A. R. (2007). Effects of high pressure processing on antioxidant activity, and total carotenoid content and availability, in vegetables. Innovative Food Science and Emerging Technologies, 8(4), 543–548. doi:10.1016/j.ifset.2007.04.005
- Nemec, M. J., Kim, H., Marciante, A. B., Barnes, R. C., Hendrick, E. D., Bisson, W. H., … Mertens-Talcott, S. U. (2017). Polyphenolics from mango (Mangifera indica L.) suppress breast cancer ductal carcinoma in situ proliferation through activation of AMPK pathway and suppression of mTOR in athymic nude mice. The Journal of Nutritional Biochemistry, 41, 12–19. doi:10.1016/j.jnutbio.2016.11.005
- Oliveira, S. C., Figueiredo, A. B., Evtuguin, D. V., & Saraiva, J. A. (2012). High pressure treatment as a tool for engineering of enzymatic reactions in cellulosic fibres. Bioresource Technology, 107, 530–534. doi:10.1016/j.biortech.2011.12.093
- Padayatty, S. J., Katz, A., Wang, Y., Eck, P., Kwon, O., Lee, J. H., … Levine, M. (2003). Vitamin C as an antioxidant: Evaluation of its role in disease prevention. Journal of the American College and Nutrition, 22(1), 18–35. doi:10.1080/07315724.2003.10719272
- Patras, A., Brunton, N. P., Da Pieve, S., & Butler, F. (2009). Impact of high pressure processing on total antioxidant activity, phenolic, ascorbic acid, anthocyanin content and colour of strawberry and blackberry purées. Innovative Food Science and Emerging Technologies, 10(3), 308–313. doi:10.1016/j.ifset.2008.12.004
- Pearson, J. F., Pullar, J. M., Wilson, R., Spittlehouse, J. K., Vissers, M. C. M., Skidmore, P. M. L., … Carr, A. C. (2017). Vitamin C status correlates with markers of metabolic and cognitive health in 50-year-olds: Findings of the CHALICE cohort study. Nutrients, 9(8), E831. doi:10.3390/nu9080831
- Prasad, K. N., Yang, E., Yi, C., Zhao, M., & Jiang, Y. (2009a). Effects of high pressure extraction on the extraction yield, total phenolic content and antioxidant activity of longan fruit pericarp. Innovative Food Science and Emerging Technologies, 10(2), 155–159. doi:10.1016/j.ifset.2008.11.007
- Prasad, N. K., Yang, B., Zhao, M., Wang, B. S., Chen, F., & Jiang, Y. (2009b). Effects of high-pressure treatment on the extraction yield, phenolic content and antioxidant activity of litchi (Litchi chinensis Sonn.) fruit pericarp. International Journal of Food Science and Technology, 44(5), 960–966. doi:10.1111/ifs.2009.44.issue-5
- Prosky, L., Asp, N. G., Furda, I., DeVries, J. W., Schweizer, T. F., & Harland, B. F. (1985). Determination of total dietary fiber in foods and food products: Collaborative study. Journal-Association of Official Analytical Chemists, 68(4), 677–679.
- Prosky, L., Asp, N. G., Schweizer, T. F., DeVries, J. W., Furda, I., & Lee, S. C. (1994). Determination of soluble dietary fiber in foods and food products: Collaborative study. Journal of AOAC International, 77, 690–694. doi:10.1093/jaoac/77.3.690
- Rameen, D. (2015). Food processing and impact on nutrition. Scholars Journal of Agriculture and Veterinary Sciences, 2, 304–311.
- Rao, L., Guo, X., Pang, X., Tan, X., Liao, X., & Wu, J. (2014). Enzyme activity and nutritional quality of peach (Prunus persica) juice: Effect of high hydrostatic pressure. International Journal of Food Properties, 17(6), 1406–1417. doi:10.1080/10942912.2012.716474
- Riccioni, G., D’Orazio, N., Speranza, L., Di Ilio, E., Glade, M., Bucciarelli, V., … Bucciarelli, T. (2010). Carotenoids and asymptomatic carotid atherosclerosis. Journal of Biological Regulators and Homeostasis Agents, 24(4), 447–452.
- Rodríguez-Garayar, M., Martín-Cabrejas, M. A., & Esteban, R. M. (2017). High hydrostatic pressure in astringent and non-astringent persimmons to obtain fiber-enriched ingredients with improved functionality. Food and Bioprocess Technology, 10(5), 854–865. doi:10.1007/s11947-017-1870-y
- Sanaka, M., Yamamoto, T., Anjiki, H., Nagasawa, K., & Kuyama, Y. (2007). Effects of agar and pectin on gastric emptying and post-prandial glycaemic profiles in healthy human volunteers. Clinical and Experimental Pharmacology and Physiology, 34(11), 1151–1155. doi:10.1111/j.1440-1681.2007.04706.x
- Sluijs, I., Cadier, E., Beulens, J. W., van der A, D. L., Spijkerman, A. M., & van der Schouw, Y. T. (2015). Dietary intake of carotenoids and risk of type 2 diabetes. Nutrition, Metabolism and Cardiovascular Diseases, 25(4), 376–381. doi:10.1016/j.numecd.2014.12.008
- Sun, Y., Kang, X., Chen, F., Liao, X., & Hu, X. (2019). Mechanisms of carrot texture alteration induced by pure effect of high pressure processing. Innovative Food Science and Emerging Technology, 54, 260–269. doi:10.1016/j.ifset.2018.08.012
- Tejada-Ortigoza, V., Garcia-Amezquita, L. E., Serna-Saldivar, S. O., & Welti-Chanes, J. (2017). The dietary fiber profile of fruit peels and functionality modifications induced by high hydrostatic pressure treatments. Food Science and Technology International, 23(5), 396–402. doi:10.1177/1082013217694301
- Trumbo, P., Yates, A. A., Schlicker, S., & Poos, M. (2001). Dietary reference intakes: Vitamin A, Vitamin K, arsenic, boron, chromium, copper, iodine, iron, manganese, molybdenum, nickel, silicon, vanadium, and zinc. Journal of the American Dietetic Association, 101(3), 294–301. doi:10.1016/S0002-8223(01)00078-5
- Vega-Galvez, A., Miranda, M., Nunez-Mancilla, Y., Garcia-Segovia, P., Ah-Hen, K., Tabilo-Munizaga, G., & Perez-Won, M. (2014). Influence of high hydrostatic pressure on quality parameters and structural properties of aloe vera gel (Aloe barbadensis Miller). Journal of Food Science and Technology, 51(10), 2481–2489. doi:10.1007/s13197-012-0769-x
- Venancio, V. P., Kim, H., Sirven, M. A., Tekwe, C. D., Honvoh, G., Talcott, S. T., & Mertens-Talcott, S. U. (2018). Polyphenol-rich mango (Mangifera indica L.) ameliorate functional constipation symptoms in humans beyond equivalent amount of fiber. Molecular Nutrition and Food Research, 62, 1701034, 1-8. doi:10.1002/mnfr.v62.12
- Vuksan, V., Sievenpiper, J. L., Owen, R., Swilley, J. A., Spadafora, P., Jenkins, D. J., … Novokmet, R. (2000). Beneficial effects of viscous dietary fiber from Konjac-mannan in subjects with the insulin resistance syndrome: Results of a controlled metabolic trial. Diabetes Care, 23(1), 9–14. doi:10.2337/diacare.23.1.9
- Weickert, M. O., & Pfeiffer, A. F. H. (2018). Impact of dietary fiber consumption on insulin resistance and the prevention of type 2 diabetes. Journal of Nutrition, 148(1), 7–12. doi:10.1093/jn/nxx008
- Willats, W. G. T., Knox, J. P., & Mikkelsen, J. D. (2006). Pectin: New insights into an old polymer are starting to gel. Trends in Food Science and Technology, 17(3), 97–104. doi:10.1016/j.tifs.2005.10.008
- Xiao, J. (2016). Phytochemicals in food and nutrition. Critical Reviews in Food Science and Nutrition, 56(sup1), S1–S3. doi:10.1080/10408398.2015.1111074
- Yao, B., Fang, H., Xu, W., Yan, Y., Xu, H., Liu, Y., … Zhao, Y. (2014). Dietary fiber intake and risk of type 2 diabetes: A dose-response analysis of prospective studies. European Journal of Epidemiology, 29(2), 79–88. doi:10.1007/s10654-013-9876-x
- Yen, G.-C., & Lin, H.-T. (1996). Comparison of high pressure treatment and thermal pasteurization effects on the quality and shelf life of guava puree. International Journal of Food Science and Technology, 31(2), 205–213. doi:10.1111/ifs.1996.31.issue-2
- Zhao, L., Zhang, F., Ding, X., Wu, G., Lam, Y. Y., Wang, X., … Zhang, C. 1. (2018). Gut bacteria selectively promoted by dietary fibers alleviate type 2 diabetes. Science, 359(6380), 1151–1156. doi:10.1126/science.aao5774