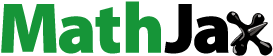
ABSTRACT
Interaction of pectin with starch modified by heat-moisture treatment (HMT) was evaluated. HMT preserved the presence of starch fractions with k1 and k2 (digestion rate constants at rapid and slow phase, respectively) being 4.5 and 0.42 × 10−2 min−1. Compared to the native starch, HMT starch had lower k1 and k2 probably due to the change in crystalline type. HMT increased total digestible starch from 1.02% in native to 27.23% in HMT starch. The decrease in k1 and/or k2 with the increase in pectin might be linked to the effect of viscosity or pectin–amylase interaction, while the decrease in digestible starch at rapid phase (C1∞) could be attributed to pectin–starch interaction. Pectin affected significantly water absorption capacity, syneresis, cohesiveness and gumminess of HMT starch but did not alter those of native starch. The effect of HMT on functional, textural and pasting properties was more remarkable than the effect of pectin.
RESUMEN
El presente estudio evaluó la interacción de la pectina con almidón modificado por tratamiento de humedad térmica (HMT). El HMT preservó la presencia de fracciones de almidón con k1 y k2 (constantes de la tasa de digestión en la fase rápida y la fase lenta, respectivamente) siendo estas 4.5 y 0.42 × 10−2 min−1. En comparación con el almidón nativo, el almidón del HMT presentó menos k1 y k2, lo que probablemente fue ocasionado por el cambio en el tipo cristalino. El HMT elevó el total de almidón digerible de 1.02% en el almidón nativo a 27.23% en el almidón HMT. La disminución de las constantes k1 y/o k2 al aumentar la pectina podría estar relacionada con el efecto de viscosidad o con la interacción pectina-amilasa, mientras que la disminución del almidón digerible en la fase rápida (C1∞) podría atribuirse a la interacción pectina-almidón. Se pudo comprobar que la pectina afectó considerablemente la capacidad de absorción de agua, la sinéresis, la cohesividad y la gomosidad del almidón HMT, aunque no alteró las capacidades del almidón nativo. Se concluyó que el efecto del HMT sobre las propiedades funcionales, texturales y de pegado fue más notable que el efecto de la pectina.
1. Introduction
Starch digestibility in the human small intestine can vary from rapid digestion to resistant starch (Lehmann & Robin, Citation2007). Starch is often modified thermally, chemically or enzymatically to adapt to industrial application. These modifications have been reported to change not only physicochemical properties but also starch digestibility (Handarini et al., Citation2020b; Jiang et al., Citation2014; Marta, Cahyana, Arifin et al., Citation2019; Quintero-Castaño et al., Citation2020). In food products, non-starch components can interact with starch and affect digestibility and physicochemical properties of starch (Cahyana et al., Citation2020; Handarini et al., Citation2020a; Kim & BeMiller, Citation2012; G. Li & Zhu, Citation2017; Marta, Cahyana, Djali et al., Citation2019). Pectin is one of the non-starch components containing at least 65% by weight of galacturonic acid-base units and it is commonly used in the food industry for thickening and gelling purposes.
Numerous studies have been carried out to reveal the effect of pectin on starch digestibility (Bi et al., Citation2017; Sasaki & Kohyama, Citation2012; Sasaki et al., Citation2015; Varela et al., Citation2016). Sasaki et al. (Citation2015) carried out a study by adding pectin to gelatinised starch suspension. The result revealed the role of pectin in gelatinised starch digestibility. Another study conducted by Bi et al. (Citation2017) has been carried out by comparing the digestibility of starch and flour.
Most previous studies were performed using the Englyst method to examine starch digestibility by which starch is classified into rapidly and slowly digestible starch (Englyst et al., Citation1992). The extent of starch digestibility (slowly or rapidly digestible starch) is often reported but digestion rate constant which is another important parameter is rarely reported. Furthermore, most studies on pectin have been conducted using native starch. The effect of pectin on digestibility and physicochemical properties of modified starch is rarely studied.
In this study interaction of pectin with banana starch modified by heat-moisture treated (HMT) was carried out. LOS-plot method was applied to determine starch digestibility. Using this method, both the extent and the digestion rate constants can be estimated (Butterworth et al., Citation2012; Edwards et al., Citation2014; Marta et al., Citation2020; Patel et al., Citation2014).
The advantage of using HMT starches is that HMT starch possesses higher content of slowly digestible starch and exhibits a change in starch structure (Cahyana et al., Citation2019). The change in starch structure could alter the interaction of pectin with starch and consequently would change digestibility and functional properties. Study on HMT starch application in food product such as biscuit demonstrated an encouraging result in terms of postprandial glucose response (Cahyana et al., Citation2020). This result shows that HMT application in food product would be interesting. HMT starch can be used in other food applications where pectin is also used. Therefore, studying the interaction of pectin and HMT starch will provide important information including the pectin effect on digestibility, functional and pasting properties of starch. Moreover, to the best of my knowledge, information on digestibility and physicochemical properties of pectin–HMT starch interaction using LOS plot is unavailable.
2. Materials and methods
2.1. Materials
Green banana “Kapas” cultivar (triploid genotypes Musa AAB) of 8– 10 weeks after flowering was obtained from local farmer (Jati Gede, Indonesia). Pectin from the citrus peel (galacturonic acid ≥74.0% db) from Sigma Aldrich (P9135).
2.2. Banana starch extraction
The green banana starch was extracted using the method of Cahyana et al. (Citation2018) with some modifications. Green banana was peeled and sliced into ±2 cm thick, rinsed immediately for 15 min in water. The banana slices were blended in a waring blender for 2 min. The resultant slurry was sieved through filter cloth and washed until the water was clear then precipitated for 4 h at room temperature. The resultant starch was washed again with water and centrifuged at 2050 ×g for 15 min. The starch was dried in a convection oven at 50°C for 24 h, ground in miller machine and sieved using 100 mesh screen.
2.3. Heat-moisture treated banana starch preparation
The heat-moisture-treated banana starch was prepared using method of Cahyana et al. (Citation2019). The starch was adjusted to 30% moisture content then put into a covered pan and equilibrated at 4°C for 24 h in a refrigerator. The starch was heated at 120°C for 8 h and dried at 50°C for 24 h in convection oven. After drying, starch was milled using miller machine and sieved using 100 mesh screen.
2.4. InVitro starch digestion
Pectin concentration used in this study referred to the concentration used in previous studies (Sasaki & Kohyama, Citation2012; Varela et al., Citation2016), while the starch concentration was in accordance with a study carried out by Butterworth et al. (Citation2012). Starch digestion was performed using previous method (Marta et al., Citation2020) with slight modification. Pectin (1%, 3% and 5% w/w based on starch weight) was added to 15 mL of deionized water in the Eppendorf tube and homogenized using vortex mixer to ensure complete dissolution. Then, the starch suspension (5 mg mL−1) was poured into the tube. The resulting mixture was homogenized using a vortex mixer and placed in a mixing rotator inside an incubator at 37°C. The solution of α-amylase was added to each tube to digest starch during which 600 μL of aliquots was withdrawn at timed intervals until 120 min. Each aliquot was transferred into an Eppendorf tube on ice containing 600 μL of stop solution. All tubes containing the aliquot were frozen (−20°C) for later use in the Prussian blue assay for the determination of maltose content (Marta et al., Citation2020; Slaughter et al., Citation2001).
2.5. Logarithm of slope analysis
Logarithm of Slope (LOS) analysis referred to Butterworth et al. (Citation2012). Starch digestibility curves can be fitted to a first-order equation (EquationEq. (1(1)
(1) ))
where Ct is the concentration of product or reactant at time (t), C∞ is the concentration of product at the end point, and k is the digestion rate constant.
The first derivative of the first-order equation in logarithmic form gives a Logarithm of Slope (LOS) plot (EquationEq. (2)(2)
(2) ).
A linear plot between ln(dC/dt) and amylolysis t can be obtained with a slope of – k and the intercept on y-axis equals ln(C∞k) from which C∞ can be calculated. When starch has two different fractions with different reactivities to amylase, the slope of each distinct phase gives a distinct rate constant. The digestion rate constants in rapid and slow phase (k1 and k2 respectively) allow estimating the end-point of starch amylolysis in rapid (C1∞) and slow phase (C2∞). The sum of C1∞ and C2∞ is total digested starch (total C∞).
2.6. Swelling volume measurement
Swelling volume was measured according to a previous method (Handarini et al., Citation2020b). Starch (0.35 g) was mixed with pectin (1%, 3% and 5% w/w, based on starch weight). The mixture was then transferred into 12.5 mL of distilled water in a centrifuge tube, then covered to prevent water loss. All samples were mixed well using a vortex mixer and heated at 92.5°C for 30 min in a water bath with stirring regularly. After heating, the samples were cooled by dipping in ice water for 1 min and centrifuged at 2050 ×g for 15 min. The resultant supernatant was poured and weighed. Volume of swelling granule was calculated by difference between volume of distilled water and supernatant. Swelling volume is the ratio of the volume of swollen granules (mL) to dry weight of sample (g).
2.7. Water absorption capacity (WAC) measurement
A 1 g of sample containing starch mixed with 0%, 1%, 3% and 5% w/w of pectin was added into 10 g of distilled water in a centrifuge tube. The suspension was mixed well using a vortex mixer, left at room temperature for 1 h. Then, the sample was centrifuged at 2050 ×g for 30 min to separate supernatant. The supernatant was decanted carefully and weighed. Water absorbed was calculated by difference between weight of distilled water and supernatant. WAC is the ratio of water absorbed (g) to dry weight of sample (g) (Marta, Cahyana, Arifin et al., Citation2019).
2.8. Freeze-thawing stability (Syneresis) measurement
All samples containing starch-pectin mixture with several concentrations (0%, 1%, 3% and 5% w/w of pectin) were transferred into distilled water in centrifuge tubes to obtain 8% w/v of starch suspension. The sample was heated to 95°C for 30 min in water bath with stirring regularly, cooled to room temperature (26 ± 2°C). The starch paste (10 g) was weighed and transferred into a centrifuge tube, stored at 4°C for 24 h, frozen at −20°C for 48 h and thawed for 3 h at room temperature. After thawing, the sample was centrifuged at 2050 ×g for 15 min to separate liquid. Syneresis is percentage of liquid separated (g) per weight of starch paste (g) (Handarini et al., Citation2020b).
2.9. Pasting properties analysis
Pasting properties of sample were analyzed using Rapid Visco Analyzer (RVA StarchMaster 2, Warriewood, Australia). 25 g of distilled water and 3 g of sample (starch containing 1%, 3% and 5% w/w pectin) were transferred into an RVA canister to make the starch suspension (14% moisture basis) with a total mass of 28 g. Before running the test, the sample mixture was homogenized by stirring. The standard heating program 1 was used. The starch slurry was maintained at 50°C for 1 min, heated to 95°C at a constant rate of 12°C min−1 and held at 95°C for 2.5 min. After heating, the starch paste was cooled to 50°C at the same rate and held at 50°C for 2 min.
2.10. Texture characteristics measurement
The sample paste formed from RVA was poured into a pipe container (2.5 cm diameter and 3 cm depth) and then left at 4°C for 24 h in a refrigerator. The gel formed was measured using a texture analyser (TA-XT2 Stable Micro System, Surrey, UK) with texture profile analysis (TPA) project. An aluminum cylinder probe (P36R) with a 2 kg load cell was used to press starch gel at a speed of 5 mm s−1. Starch gel texture characteristics including hardness, springiness, cohesiveness, and gumminess were determined by the exponent lite express software.
2.11. Statistical analysis
Data were expressed as mean ± SD triplicate. One-way analysis of variance (ANOVA) was applied for the data of digestibility and physicochemical properties of HMT banana starches, followed by Duncan test, at a significance level of 5% (P < .05). Physicochemical and starch digestibility of native starches were analyzed using two-sample T-test (independent-samples T-test). All Data were analyzed using SPSS 21.0 Statistical Software Program.
3. Result and discussion
3.1. InVitro starch digestibility
Digestibility curves of interaction between pectin with native and HMT banana starches are presented in . The digestibility of HMT starches either in the absence or presence of 3% pectin was higher than that of native starches. This might be attributed to the change in starch structure following HMT. Several studies have reported that HMT starch exhibited shorter double helices and a decrease in crystallinity compared to its native starch (Y. Chen et al., Citation2017; Marta et al., Citation2020; Tan et al., Citation2017). Addition of pectin to HMT starch decreased its digestibility within 120 min of experiment. Although the effect of HMT and pectin on digestibility is clear from the curve, but the curve fails to detect whether or not different starch fractions possessing different reactivities toward amylase are present. In order to elucidate such parameters, LOS-plot was applied.
3.2. LOS analysis
The LOS-plot () was derived from the digestibility curve and the values of the first-order reaction are summarized in . The LOS-plot curves for all native and HMT banana starches showed discontinuity, indicating the presence of starch fractions digested with different reactivities to amylase, i.e. rapid and slow fraction. Our finding on the presence of different starch fractions in native starch is in line with our previous study (Marta et al., Citation2020) and also with other studies on pea and wheat starches (Butterworth et al., Citation2012); wheat, potato, pea, rice and maize starches (Patel et al., Citation2014). Furthermore, HMT starches also exhibited two differently digestible starch fractions although the starch had been thermally modified. Therefore, HMT modification can preserve the presence of different fractions of starch normally present in native starch probably due to the granular intactness. This finding is in line with our previous study (Marta et al., Citation2020). The intact granule of HMT banana starch has been previously reported in other studies (Cahyana et al., Citation2019; Marta et al., Citation2020).
Table 1. Parameters of starch digestibility estimated from the LOS-plot in native and HMT banana starches in the absence or presence of pectin.
Tabla 1. Parámetros de digestibilidad del almidón estimados a partir del gráfico LOS en almidones nativos y de plátanos HMT en ausencia o presencia de pectina
Figure 2. Typical Logarithm of Slope (LOS)-plots obtained from digestibility data of interaction between pectin with native and HMT banana starches. HMT = Heat-moisture treatment.
Figura 2. Típico logaritmo de la pendiente (LOS) - gráficos obtenidos a partir de datos de digestibilidad de la interacción entre la pectina con los almidones nativos y el HMT banana. HMT = Tratamiento de humedad por calor
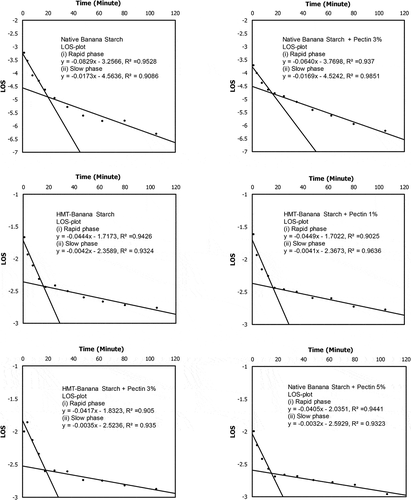
Values of k1 and k2 in HMT starch were lower than those of native starch. The lower digestion rate constant of HMT starch particularly k2 compared to that of native starch has been suggested to link to the change in crystalline type of starch. HMT banana starch exhibited an A crystalline type with a denser-packed structure through which the amylase might diffuse more slowly than in B type, leading to a lower digestion rate constant (Marta et al., Citation2020).
Discontinuity in the LOS-plot occurred within 15–20 min of incubation under digestion condition used in the experiment. The total digestible starch (total C∞) in native banana starch was around 1% consisting of 0.41% digestible starch in the rapid phase (C1∞) and 0.61% in slow phase (C2∞). The rate constants of k1 and k2 were, respectively, around 8.49 × 10−2 min−1 and 1.62 × 10−2 min−1. The low total digestible starch in native banana starch using the LOS-plot method was also found in potato starch of approximately 2.6% (Patel et al., Citation2014). The total C∞ value of banana starch increased to 27.23% when HMT was applied. The increase in total C∞ can be attributed to the increase in both digestible starches in rapid (C1∞) and slower phase (C2∞). The starch fraction in the slower phase however increased incredibly by HMT suggesting that HMT is a suitable treatment to augment the content of digestible starch at slower phase, i.e. slowly digestible starch.
The increase of pectin concentration decreased k1, C1∞, and k2 of HMT banana starches. The addition of at least 3% pectin in HMT starch significantly reduced starch digestibility parameters particularly k1 and k2 from, respectively, 4.5 and 0.42 × 10−2 min−1 to 4.2 and 0.35 × 10−2 min−1.
Rate constants were affected by the addition of 3% pectin but the amount of digestible starch remained unchanged. As pectin concentration increased to 5%, value of C1∞ decreased while value of C2∞ did not change. Several factors can be proposed to explain its underlying mechanism one of which is viscosity.
Further investigation on the viscosity in this study, it was found that the viscosities of milieu with 0%, 1%, 3%, and 5% pectin were, respectively, 23.7, 40, 42, 45 mPas suggesting that pectin increased the milieu viscosity. Pearson correlation between viscosities with starch digestibility parameters indicated that the correlation was significant for values of k1 and k2 (Supplementary Table 1). This finding suggests that pectin is more effective on reducing rate constant than the amount of digestible starch. The reduction of digestion rate constants might stem from the increase in viscosity which ultimately led to lower amylase diffusion to hydrolyze digestible α-glucan chains. At 3% pectin concentration the reducing rate constants might also result from the reduction of amylase concentration due to its binding with pectin. The binding between enzyme and pectin was observed in another previous study (Bai et al., Citation2017).
As pectin concentration increased to 5%, the amount of digestible starch reduced which is probably due to pectin interaction with starch. The interaction might form a physical barrier on the granular surface hence lowering digestible starch at rapid phase as suggested by Sasaki and Kohyama (Citation2011).
Overall it can be proposed that the role of pectin in starch digestion is first of all to reduce the rate constants with k1 being more sensitive than k2. This reduction may be because of the increase in viscosity or amylase–pectin interaction. As the pectin concentration increases, starch–pectin interaction takes place leading to a lower amount of digestible starch.
3.3. Functional properties
Functional properties including swelling volume (SV), water absorption capacity (WAC) and syneresis of native and HMT banana starches are presented in . HMT decreased SV and WAC but increased syneresis of banana starch. The increased syneresis of HMT banana starch was also reported in other studies (Cahyana et al., Citation2019; Marta, Cahyana, Arifin et al., Citation2019). It is observed that the decrease in SV is in concomitant with the decrease in WAC therefore the decrease in SV might be related to the decrease in WAC. The change in WAC may result from the rearrangement of molecular chains during HMT which strengthens the structure of granule thereby limiting the absorption of water into granule. The decrease in SV may also because of a decrease in granular stability (Olayinka et al., Citation2008), an increase in interaction between amylose and amylopectin and formation of amylose-lipids complexes in starch granules (Adebowale et al., Citation2005; X. Chen et al., Citation2015; Olayinka et al., Citation2008; Xiao et al., Citation2017).
Table 2. Functional and texture properties of native and HMT banana starches in the absence or presence of pectin.
Tabla 2. Propiedades funcionales y de textura de los almidones de los plátanos nativos y HMT en ausencia o presencia de pectina
In terms of pectin effect, addition of 3% pectin to native starch did not have any significant effect on SV, WAC, and syneresis of native banana starch. Whereas in HMT starch, pectin addition significantly decreased WAC and syneresis. The decrease in SV from 12.84 mL g−1 to 10.97 mL g−1 was observed when 5% pectin was mixed with HMT starch. Pectin affected functional properties more remarkably in HMT starch than in native-starch. It suggests that interaction of pectin in HMT starch might be more favorable than in native starch, leading to a more pronounced change in functional properties.
The addition of 1% to 5% pectin significantly reduced WAC of HMT banana starch from 1.47 to 1.16 g g−1 db. This result is in agreement with another study on wheat/potato starch mixed with 1.5% and 3% pectin (Varela et al., Citation2016). The reduction in WAC might be linked to the hydration competition between starch granule and pectin molecules for water. The decrease may be also caused by wrapping starch granule by pectin during heating (Zhang et al., Citation2018). Pectin addition at 5% decreased SV. The decrease in SV with the addition of pectin and other hydrocolloids was also reported in other studies (Chaisawang & Suphantharika, Citation2006; H.-m. Chen et al., Citation2015; C.-C. Huang, Citation2009; Varela et al., Citation2016).
Upon thawing, bulk phase water is released from the starch gel, a phenomenon known as syneresis. The amount of syneresis is directly related to starch retrogradation (Bai et al., Citation2017) and is used to evaluate the stability of starch gels during freezing and thawing.
Contrary to the effect of HMT, pectin addition reduced syneresis. The addition of pectin significantly reduced syneresis of HMT starch from 30.90% to 12.46%, suggesting that pectin addition improves freeze-thaw stability. The finding is in line with previous studies (Varela et al., Citation2016; Zhang et al., Citation2018). Other hydrocolloids such as arabic gum, xanthan gum, locust bean gum and konjac-glucomannan have been reported to improve stability of various starch gels (Charoenrein et al., Citation2011; Funami et al., Citation2008; H.-m. Chen et al., Citation2015; Muadklay & Charoenrein, Citation2008). The decrease in syneresis is related to an increase in intracellular and intermolecular hydrogen bonds (Muadklay & Charoenrein, Citation2008; Phimolsiripol et al., Citation2011), phase separation or interactions between hydrocolloids and amylose molecules (Shi & BeMiller, Citation2002).
3.4. Texture characteristics
Texture characteristics of native and HMT banana starches are presented in . The texture characteristics including hardness, springiness, cohesiveness, and gumminess were observed. HMT reduced all texture parameters of native starch. The hardness decreased from 583.39 gF in native starch to 94.17 gF following HMT. A similar trend was observed in the presence of 3% pectin.
HMT has been reported to alter starch structure including crystallinity and crystalline type (Cahyana et al., Citation2019), molecular weight and lamellar structure (Tan et al., Citation2017), and birefringence pattern (S. Li et al., Citation2011). HMT also decreased gelatinization enthalpy (ΔH) (T.-T. Huang et al., Citation2016; Tan et al., Citation2017). These structural changes apparently affected the interaction of intermolecular starch chains possibly hydrogen bonds formed from hydroxyl groups. HMT rendered such interactions weaker during starch retrogradation and consequently the hardness reduced. HMT starch also exhibited a lower springiness, cohesiveness and gumminess. As gumminess is a secondary property that is dependent on hardness and cohesiveness, the reduction in gumminess could be attributed to the decrease in both hardness and cohesiveness.
The addition of pectin to native banana starch did not have any significant effect on all texture parameters except on hardness. The hardness of native and HMT starch decreased, respectively, from 583.39 gF and 94.17 gF to 464.21 gF and 61.59 gF when mixed with 3% pectin. Zhang et al. (Citation2018) proposed that pectin could penetrate the interior of starch granules, which led to an increase in the distance between starch molecular chains and a decrease in hydrogen bonds. This might lead to a decrease in hardness.
Neither springiness of HMT starch nor that of native starch was affected by pectin. The increase in pectin increased cohesiveness but decreased gumminess which is in agreement with a previous study (Zheng et al., Citation2019). Given that gumminess depends on hardness and cohesiveness, it can be concluded that the decrease in gumminess in the presence of pectin may be attributed to the reduction in hardness but not related to the change in cohesiveness.
3.5. Pasting properties
Pasting properties of native and HMT banana starches are presented in and . HMT decreased viscosity of native starch paste and resulted in a more stable viscosity during heating and mixing compared to native starch. However, pasting curve of HMT banana starches did not show any clear peak viscosity; therefore, parameters such as peak viscosity (PV) and breakdown (BD) could not be determined. This was also observed in previous study (Tan et al., Citation2017).
Table 3. Pasting properties of native and HMT banana starches in the absence or presence of pectin.
Tabla 3. Propiedades de pegado de los almidones de plátano nativos y HMT en ausencia o presencia de pectina
Figure 3. Pasting properties of interaction between pectin with native and HMT banana starches. HMT = Heat-moisture treatment.
Figura 3. Propiedades de la interacción de la pectina con los almidones de los plátanos nativos y HMT. HMT = Tratamiento de humedad por calor
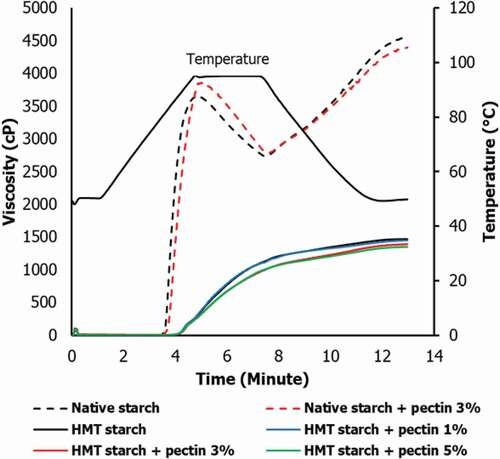
HMT increased pasting temperature (PT) but decreased final viscosity (FV), hold viscosity (HV) and setback (SB) compared to the native. Our finding is in line with previous studies (Cahyana et al., Citation2019; Marta, Cahyana, Arifin et al., Citation2019; Puncha-arnon & Uttapap, Citation2013; Tan et al., Citation2017). The increase in the PT after HMT could be related to the limited swelling volume as observed in this study and in other studies (Cahyana et al., Citation2019; Sun et al., Citation2013).
Neither PT of native starch nor HMT starch was affected by pectin. Addition of 3% pectin to native starch increased PV to 3872 cP but decreased FV and SB. Meanwhile, the same pectin concentration decreased HV and FV of HMT starch. The increase in the PV might be related to the increase in viscosity due to pectin addition (Dartois et al. (Citation2010)).
Comparing the effect of pectin with HMT on pasting properties of starch it is clear that the effect of HMT on the changes in pasting properties is more preponderant than the effect of pectin. The change due to pectin addition is much smaller than due to HMT so that when pectin is mixed with HMT starch, the effect of pectin on pasting properties could be neglected.
Overall when parameters of functional properties were related to texture and pasting properties in HMT starch using Pearson correlation it was found that SV and WAC were positively correlated with hardness, gumminess, and hold viscosity but negatively correlated with cohesiveness (supplementary Table 2). This may be related to the change in moisture content in the starch system which can be indicated from the change in WAC.
4. Conclusion
Heat-moisture treatment preserved the presence of different fractions of starch normally observed in native starch as indicated by the discontinuity in LOS-plot. HMT increased remarkably the amount of digestible starch at slower phase (C2∞) to approximately 23% of starch. The lower k2 of HMT starch compared to that of native starch may be attributed to the difference in the density of structure packing where the A crystalline type in HMT starch with a denser-packed structure might slow down the amylase diffusion thereby reducing digestion rate constant.
The increase in pectin decreased digestion rate constants. The reduction may be attributed to the increase in viscosity or interaction of pectin and amylase. The lower digestible starch particularly C2∞ with the increase in pectin concentration may be linked to pectin–starch interaction. HMT decreased SV and WAC but increased syneresis of the starch. HMT also increased pasting temperature but decreased final, hold and setback viscosity compared to the native. In terms of texture characteristics hardness, springiness, cohesiveness, and gumminess were lower in HMT starch than in native starch.
The effect of pectin on functional properties such as SV, WAC and syneresis depends on the starch structure or granule morphology. In the presence of pectin, functional properties of HMT starch were more sensitive to change than those of the native starch suggesting the more favorable pectin–HMT starch interaction than pectin–native starch interaction. Moreover, pectin did not affect pasting temperature of starch which suggests that pasting temperature might be related to the change in starch structure and granule morphology. Pectin did not affect the springiness of starch. Hardness and gumminess reduced but cohesiveness increased with the increase in pectin. By and large, the effect of HMT on the changes in pasting properties is more preponderant than the effect of pectin. A similar effect can be seen on digestibility and functional properties except syneresis. In food application, when HMT starch is used for its high slowly digestible starch content, the product is expected to have poor storage stability due to retrogradation. Pectin addition is recommended to improve freeze-thaw stability without changing pasting and other properties remarkably.
Supplemental Material
Download MS Word (14.6 KB)Disclosure statement
The authors have no conflicts of interest to declare.
Supplementary material
Supplemental data for this article can be accessed on the publisher’s website.
Additional information
Funding
References
- Adebowale, K. O., Afolabi, T. A., & Olu-Owolabi, B. I. (2005). Hydrothermal treatments of finger millet (Eleusine coracana) starch. Food Hydrocolloids, 19(6), 974–983. https://doi.org/https://doi.org/10.1016/j.foodhyd.2004.12.007
- Bai, Y., Wu, P., Wang, K., Li, C., Li, E., & Gilbert, R. G. (2017). Effects of pectin on molecular structural changes in starch during digestion. Food Hydrocolloids, 69, 10–18. https://doi.org/https://doi.org/10.1016/j.foodhyd.2017.01.021
- Bi, Y., Zhang, Y., Jiang, H., Hong, Y., Gu, Z., Cheng, L., Li, Z., & Li, C. (2017). Molecular structure and digestibility of banana flour and starch. Food Hydrocolloids, 72(Suppl. C), 219–227. https://doi.org/https://doi.org/10.1016/j.foodhyd.2017.06.003
- Butterworth, P. J., Warren, F. J., Grassby, T., Patel, H., & Ellis, P. R. (2012). Analysis of starch amylolysis using plots for first-order kinetics. Carbohydrate Polymers, 87(3), 2189–2197. https://doi.org/https://doi.org/10.1016/j.carbpol.2011.10.048
- Cahyana, Y., Rangkuti, A., Siti Halimah, T., Marta, H., & Yuliana, T. (2020). Application of heat-moisture-treated banana flour as composite material in hard biscuit. CyTA - Journal of Food, 18(1), 599–605. https://doi.org/https://doi.org/10.1080/19476337.2020.1805514
- Cahyana, Y., Titipanillah, R., Mardawati, E., Sukarminah, E., Rialita, T., Andoyo, R., Djali, M., Hanidah, -I.-I., Setiasih, I. S., & Handarini, K. (2018). Non-starch contents affect the susceptibility of banana starch and flour to ozonation. Journal of Food Science and Technology, 55(5), 1726–1733. https://doi.org/https://doi.org/10.1007/s13197-018-3085-2
- Cahyana, Y., Wijaya, E., Halimah, T. S., Marta, H., Suryadi, E., & Kurniati, D. (2019). The effect of different thermal modifications on slowly digestible starch and physicochemical properties of green banana flour (Musa acuminata colla). Food Chemistry, 274, 274–280. https://doi.org/https://doi.org/10.1016/j.foodchem.2018.09.004
- Chaisawang, M., & Suphantharika, M. (2006). Pasting and rheological properties of native and anionic tapioca starches as modified by GG and xanthan gum. Food Hydrocolloids, 20(5), 641–649. https://doi.org/https://doi.org/10.1016/j.foodhyd.2005.06.003
- Charoenrein, S., Tatirat, O., Rengsutthi, K., & Thongngam, M. (2011). Effect of konjac glucomannan on syneresis, textural properties and the microstructure of frozen rice starch gels. Carbohydrate Polymers, 83(1), 291–296. https://doi.org/https://doi.org/10.1016/j.carbpol.2010.07.056
- Chen, H.-M., Fu, X., & Luo, Z.-G. (2015). Effect of gum arabic on freeze-thaw stability, pasting and rheological properties of tapioca starch and its derivatives. Food Hydrocolloids, 51, 355–360. https://doi.org/https://doi.org/10.1016/j.foodhyd.2015.05.034
- Chen, X., He, X., Fu, X., & Huang, Q. (2015). In vitro digestion and physicochemical properties of wheat starch/flour modified by heat-moisture treatment. Journal of Cereal Science, 63, 109–115. https://doi.org/https://doi.org/10.1016/j.jcs.2015.03.003
- Chen, Y., Yang, Q., Xu, X., Qi, L., Dong, Z., Luo, Z., Lu, X., & Peng, X. (2017). Structural changes of waxy and normal maize starches modified by heat moisture treatment and their relationship with starch digestibility. Carbohydrate Polymers, 177(Suppl. C), 232–240. https://doi.org/https://doi.org/10.1016/j.carbpol.2017.08.121
- Dartois, A., Singh, J., Kaur, L., & Singh, H. (2010). Influence of guar gum on the in vitro starch digestibility—Rheological and microstructural characteristics. Food Biophysics, 5(3), 149–160. https://doi.org/https://doi.org/10.1007/s11483-010-9155-2
- Edwards, C. H., Warren, F. J., Milligan, P. J., Butterworth, P. J., & Ellis, P. R. (2014). A novel method for classifying starch digestion by modelling the amylolysis of plant foods using first-order enzyme kinetic principles. Food & Function, 5(11), 2751–2758. https://doi.org/https://doi.org/10.1039/c4fo00115j
- Englyst, H. N., Kingman, S. M., & Cummings, J. (1992). Classification and measurement of nutritionally important starch fractions. European Journal of Clinical Nutrition, 46(Suppl 2), S33–50. https://pubmed.ncbi.nlm.nih.gov/1330528/
- Funami, T., Nakauma, M., Noda, S., Ishihara, S., Asai, I., Inouchi, N., & Nishinari, K. (2008). Effects of some anionic polysaccharides on the gelatinization and retrogradation behaviors of wheat starch: Soybean-soluble polysaccharide and gum arabic. Food Hydrocolloids, 22(8), 1528–1540. https://doi.org/https://doi.org/10.1016/j.foodhyd.2007.10.008
- Handarini, K., Hamdani, J. S., Cahyana, Y., & Setiasih, I. S. (2020a). Functional and pasting properties of a starch–lipid complex formed with gaseous ozone and palm oil. International Journal of Food Properties, 23(1), 1361–1372. https://doi.org/https://doi.org/10.1080/10942912.2020.1801723
- Handarini, K., Hamdani, J. S., Cahyana, Y., & Setiasih, I. S. (2020b). Gaseous ozonation at low concentration modifies functional, pasting, and thermal properties of arrowroot starch (Maranta arundinaceae). Starch - Stärke, 72(5–6), 1900106. https://doi.org/https://doi.org/10.1002/star.201900106
- Huang, -C.-C. (2009). Physicochemical, pasting and thermal properties of tuber starches as modified by guar gum and locust bean gum. International Journal of Food Science & Technology, 44(1), 50–57. https://doi.org/https://doi.org/10.1111/j.1365-2621.2007.01634.x
- Huang, -T.-T., Zhou, D.-N., Jin, Z.-Y., Xu, X.-M., & Chen, H.-Q. (2016). Effect of repeated heat-moisture treatments on digestibility, physicochemical and structural properties of sweet potato starch. Food Hydrocolloids, 54(Part A), 202–210. https://doi.org/https://doi.org/10.1016/j.foodhyd.2015.10.002
- Jiang, H., Miao, M., Ye, F., Jiang, B., & Zhang, T. (2014). Enzymatic modification of corn starch with 4-α-glucanotransferase results in increasing slow digestible and resistant starch. International Journal of Biological Macromolecules, 65, 208–214. https://doi.org/https://doi.org/10.1016/j.ijbiomac.2014.01.044
- Kim, H.-S., & BeMiller, J. N. (2012). Effects of hydrocolloids on the pasting and paste properties of commercial pea starch. Carbohydrate Polymers, 88(4), 1164–1171. https://doi.org/https://doi.org/10.1016/j.carbpol.2012.01.060
- Lehmann, U., & Robin, F. (2007). Slowly digestible starch – Its structure and health implications: A review. Trends in Food Science & Technology, 18(7), 346–355. https://doi.org/https://doi.org/10.1016/j.tifs.2007.02.009
- Li, G., & Zhu, F. (2017). Physicochemical properties of quinoa flour as affected by starch interactions. Food Chemistry, 221(Suppl. C), 1560–1568. https://doi.org/https://doi.org/10.1016/j.foodchem.2016.10.137
- Li, S., Ward, R., & Gao, Q. (2011). Effect of heat-moisture treatment on the formation and physicochemical properties of resistant starch from mung bean (Phaseolus radiatus) starch. Food Hydrocolloids, 25(7), 1702–1709. https://doi.org/https://doi.org/10.1016/j.foodhyd.2011.03.009
- Marta, H., Cahyana, Y., Arifin, H. R., & Khairani, L. (2019). Comparing the effect of four different thermal modifications on physicochemical and pasting properties of breadfruit (Artocarpus altilis) starch. International Food Research Journal, 26(1), 269–276. http://www.ifrj.upm.edu.my/26%20(01)%202019/(30).pdf
- Marta, H., Cahyana, Y., & Djali, M. (2020). Densely packed-matrices of heat moisture treated-starch determine the digestion rate constant as revealed by logarithm of slope plots. Journal of Food Science and Technology. https://doi.org/https://doi.org/10.1007/s13197-020-04734-6
- Marta, H., Cahyana, Y., Djali, M., Arcot, J., & Tensiska, T. (2019). A comparative study on the physicochemical and pasting properties of starch and flour from different banana (Musa spp.) cultivars grown in Indonesia. International Journal of Food Properties, 22(1), 1562–1575. https://doi.org/https://doi.org/10.1080/10942912.2019.1657447
- Muadklay, J., & Charoenrein, S. (2008). Effects of hydrocolloids and freezing rates on freeze–thaw stability of tapioca starch gels. Food Hydrocolloids, 22(7), 1268–1272. https://doi.org/https://doi.org/10.1016/j.foodhyd.2007.06.008
- Olayinka, O. O., Adebowale, K. O., & Olu-Owolabi, B. I. (2008). Effect of heat-moisture treatment on physicochemical properties of white sorghum starch. Food Hydrocolloids, 22(2), 225–230. https://doi.org/https://doi.org/10.1016/j.foodhyd.2006.11.004
- Patel, H., Day, R., Butterworth, P. J., & Ellis, P. R. (2014). A mechanistic approach to studies of the possible digestion of retrograded starch by α-amylase revealed using a log of slope (LOS) plot. Carbohydrate Polymers, 113(Suppl. C), 182–188. https://doi.org/https://doi.org/10.1016/j.carbpol.2014.06.089
- Phimolsiripol, Y., Siripatrawan, U., & Henry, C. J. K. (2011). Pasting behaviour, textural properties and freeze–thaw stability of wheat flour–crude malva nut (Scaphium scaphigerum) gum system. Journal of Food Engineering, 105(3), 557–562. https://doi.org/https://doi.org/10.1016/j.jfoodeng.2011.03.022
- Puncha-arnon, S., & Uttapap, D. (2013). Rice starch vs. rice flour: Differences in their properties when modified by heat–moisture treatment. Carbohydrate Polymers, 91(1), 85–91. https://doi.org/https://doi.org/10.1016/j.carbpol.2012.08.006
- Quintero-Castaño, V. D., Castellanos-Galeano, F. J., Álvarez-Barreto, C. I., Bello-Pérez, L. A., & Alvarez-Ramirez, J. (2020). In vitro digestibility of octenyl succinic anhydride-starch from the fruit of three Colombian Musa. Food Hydrocolloids, 101, 105566. https://doi.org/https://doi.org/10.1016/j.foodhyd.2019.105566
- Sasaki, T., & Kohyama, K. (2011). Effect of non-starch polysaccharides on the in vitro digestibility and rheological properties of rice starch gel. Food Chemistry, 127(2), 541–546. https://doi.org/https://doi.org/10.1016/j.foodchem.2011.01.038
- Sasaki, T., & Kohyama, K. (2012). Influence of non-starch polysaccharides on the in vitro digestibility and viscosity of starch suspensions. Food Chemistry, 133(4), 1420–1426. https://doi.org/https://doi.org/10.1016/j.foodchem.2012.02.029
- Sasaki, T., Sotome, I., & Okadome, H. (2015). In vitro starch digestibility and in vivo glucose response of gelatinized potato starch in the presence of non-starch polysaccharides. Starch - Stärke, 67(5–6), 415–423. https://doi.org/https://doi.org/10.1002/star.201400214
- Shi, X., & BeMiller, J. N. (2002). Effects of food gums on viscosities of starch suspensions during pasting. Carbohydrate Polymers, 50(1), 7–18. https://doi.org/https://doi.org/10.1016/S0144-8617(01)00369-1
- Slaughter, S. L., Ellis, P. R., & Butterworth, P. J. (2001). An investigation of the action of porcine pancreatic α-amylase on native and gelatinised starches. Biochimica Et Biophysica Acta (BBA) - General Subjects, 1525(1), 29–36. https://doi.org/https://doi.org/10.1016/S0304-4165(00)00162-8
- Sun, Q., Wang, T., Xiong, L., & Zhao, Y. (2013). The effect of heat moisture treatment on physicochemical properties of early indica rice. Food Chemistry, 141(2), 853–857. https://doi.org/https://doi.org/10.1016/j.foodchem.2013.03.077
- Tan, X., Li, X., Chen, L., Xie, F., Li, L., & Huang, J. (2017). Effect of heat-moisture treatment on multi-scale structures and physicochemical properties of breadfruit starch. Carbohydrate Polymers, 161(Suppl. C), 286–294. https://doi.org/https://doi.org/10.1016/j.carbpol.2017.01.029
- Varela, M. S., Navarro, A. S., & Yamul, D. K. (2016). Effect of hydrocolloids on the properties of wheat/potato starch mixtures. Starch - Stärke, 68(7–8), 753–761. https://doi.org/https://doi.org/10.1002/star.201400257
- Xiao, Y., Liu, H., Wei, T., Shen, J., & Wang, M. (2017). Differences in physicochemical properties and in vitro digestibility between tartary buckwheat flour and starch modified by heat-moisture treatment. LWT - Food Science and Technology, 86(Suppl. C), 285–292. https://doi.org/https://doi.org/10.1016/j.lwt.2017.08.001
- Zhang, B., Bai, B., Pan, Y., Li, X.-M., Cheng, J.-S., & Chen, H.-Q. (2018). Effects of pectin with different molecular weight on gelatinization behavior, textural properties, retrogradation and in vitro digestibility of corn starch. Food Chemistry, 264, 58–63. https://doi.org/https://doi.org/10.1016/j.foodchem.2018.05.011
- Zheng, J., Liu, M., Zhang, M., Kan, J., & Zhang, F. (2019). Effects of pectin on the pasting, rheological, and textural properties of lotus root starch. Starch - Stärke, 71(3–4), 1700347. https://doi.org/https://doi.org/10.1002/star.201700347