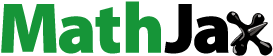
ABSTRACT
Bacterial cellulose films were combined with glycerol and chitosan to improve the UV-barrier and mechanical properties. In part 1, the moisture content and mechanical properties (tensile and puncture properties) were analysed by response surface methodology. The calculated values of the mechanical properties were in the range of 1.23–39.28 MPa for tensile strength, 2.28–25.34% for percentage of elongation, 11.72–1579.59 MPa for Young’s modulus, 0.22–1.92 MJ/m3 for toughness, 43.74–1067.35 g for burst strength and 0.39–3.12 mm for distance to burst. In general, a plasticizing and a reinforcing effect on the bacterial cellulose films was observed by glycerol and chitosan addition. Glycerol provided adhesive properties, which can be an advantage for application on foods, such as fruit or vegetables, as a wrapping material.
RESUMEN
Se combinaron películas de celulosa bacteriana con glicerol y quitosano para mejorar las propiedades de barrera a la radiación UV y las propiedades mecánicas. En la parte 1, se analizaron el contenido de humedad y las propiedades mecánicas (propiedades de tracción y punción) mediante la metodología de superficie de respuesta. Los valores calculados de las propiedades mecánicas estuvieron en el rango de 1.23-39.28 MPa para resistencia a la tracción, 2.28-25.34% para porcentaje de alargamiento, 11.72-1579.59 MPa para módulo de Young, 0.22-1.92 MJ/m3 para tenacidad, 43.74-1067.35 g para resistencia a la punción y 0.39-3.12 mm para la elasticidad de punción. En general, se observó un efecto plastificante y reforzante sobre las películas de celulosa bacteriana mediante la adición de glicerol y quitosano. El glicerol proporcionó propiedades adhesivas, que pueden ser una ventaja para su aplicación en alimentos, como frutas o verduras, como material de envoltura.
1. Introduction
Food packaging is constantly evolving due to the current consumer demand to find sustainable, minimally processed, fresh, and safe products while keeping high quality and organoleptic properties. Besides, consumer concerns about environmental problems derived from the high consumption and uncontrolled management of synthetic plastics are growing. Consumers demand biodegradable materials from natural sources. Driven by the current demand and trend in food industry in the last decades, innovative biodegradable and edible food packaging materials with active functional properties have been developed. These are not limited to be only a physical barrier to protect food from external factors (Janjarasskul & Suppakul, Citation2018; Khan et al., Citation2020; Wyrwa & Barska, Citation2017).
The interest in developing active packaging systems has grown to attend the current market demand, as a result of the simultaneous increase in food demand and the new consumer trends. In literature, the concept of active packaging is defined as packaging in which subsidiary constituents have been deliberately included in or on either the packaging material or the package headspace to enhance the performance of the package system (Robertson, Citation2012). Accordingly, an active packaging is a system where the packaging material, the packed product, and the environment interact to extend the shelf life, keeping its sensory and quality properties (Pang et al., Citation2019; Wyrwa & Barska, Citation2017).
Among the main potential biopolymers to develop new biodegradable materials of natural origin is the bacterial cellulose (BC). BC is a unique natural material with interesting properties, such as a 3D structure, biodegradability, non-toxic and biocompatibility. Recently, it has been published a novel in-situ process to coat 3D objects by the adhesion of cellulose-secreting bacteria on selected object forming a hydrogel with high water content with application in biomedical field (Rühs et al., Citation2020). However, there is a significant lack of studies evaluating the potential of this material to develop active packaging films for application in food or as a vehicle for other active components.
Unlike vegetable cellulose, BC is synthesized in pure form, without the presence of vegetable remains, such as lignin, hemicellulose or pectin. The BC purity makes its production and purification an easier process that do not require intensive treatments, aggressive chemicals or high energy input as compared to the extraction of cellulose from plants. In addition, BC can be used directly, without a dissolution step during the film production. The BC obtained process allows maintaining its 3D structure and crystallinity index above 85% (Azeredo et al., Citation2019; Gromovykh et al., Citation2020; Jozala et al., Citation2016; Keshk, Citation2014; Tsouko et al., Citation2015). The large amount of hydroxyl groups along the BC chains promote strong physical interactions resulting in a rigid structure in dried samples, driving to high Young’s modulus and low elongation values (Cazon et al., Citation2019; Sun et al., Citation2018). The poor elastic properties of dried BC make it a brittle material that limits its suitability for many applications (Sun et al., Citation2018). However, its high porosity and 3D structure make easy to modify its properties ex-situ after the fermentation bioprocess, by dipping in baths with plasticizers or soluble polymer solutions. This post-modification method has been also studied for regenerated cellulose-based films (Cazon et al., Citation2018b, Citation2020c). However, the unique structure of BC and its capability for ex-situ properties modification offer a great advantage to reach a higher number of interactions between the components (Reiniati et al., Citation2017). In this way, polymer blending is a good strategy to overcome the brittleness of BC and to improve the functional properties (Lin et al., Citation2013; Sun et al., Citation2018; Ul-Islam et al., Citation2011). The improvement of the mechanical properties of the BC to obtain easier handling films could allow expanding its potential applications as a biodegradable material.
Glycerol is a well-known biocompatible polyol, commonly used as a food additive authorized by the European Food Safety Authority (EFSA) Mortensen et al., Citation2017. Due to its properties, such as non-volatility, cheap and low toxicity, glycerol is extensively used as a plasticizer in polysaccharide-based films, such as cellulose (Sirviö et al., Citation2018; Sun et al., Citation2018).
In literature, previous studies demonstrated the plasticizing effect of glycerol on BC films. Results indicated that glycerol interacts with the BC polymer chains, reducing the intra- and inter-molecular hydrogen bonds in the BC fibers, decreasing the stiffness and brittleness of the dried BC samples and improving their elasticity (Cazon et al., Citation2019a, Citation2020b; Cielecka et al., Citation2019; Sun et al., Citation2018).
On the other hand, chitosan is a natural polymer that has received attention for its commercial applications in medical, nutrition and food technology fields. Chitosan was accepted as Generally Recognized As Safe (GRAS) in 2005, and it has been approved as food additive to preserve many food products in Japan and Korea (Montilla et al., Citation2013). Chitosan has several properties suitable for combining it with other materials and obtain composites with improved functional properties. Among the main properties, chitosan has film-forming ability (Khemir et al., Citation2020). Chitosan is obtained from renewable sources, primarily from the shellfish industry waste, being inexpensive and commercially available (Aider, Citation2010; Cazon & Vázquez, Citation2020; Morin-Crini et al., Citation2019).
Previous works have evaluated and characterized BC-chitosan films for application as a food packaging, obtaining improved mechanical resistance properties, but showing poor elasticity values (Ul-Islam et al., Citation2011). BC-chitosan-polyvinyl alcohol composite films were analyzed to evaluate the effect of chitosan and polyvinyl alcohol on the BC structure and the final properties of the composite. Results indicated that chitosan and polyvinyl alcohol manifested a reinforcing and plasticizing effect on the BC structure, improving the mechanical, optical and thermal properties of the samples compared with the pure polymer (Cazon et al., Citation2019). However, polyvinyl alcohol produced an increase in the transmittance values of the samples in the UV-light region. Considering the results obtained in previous works and the interest in developing films with antimicrobial and UV radiation barrier properties, the combination of BC-chitosan-glycerol could be a good alternative as biodegradable films for food packaging. Glycerol could manifest a desired plasticizing effect on BC films while improving its UV-light barrier properties. Chitosan could promote a reinforcing effect of the matrix, improving the functionality of the films thanks to its extensively evaluated antimicrobial properties (Rabea et al., Citation2003).
BC-glycerol-chitosan composites were previously evaluated as artificial dura mater candidates for head trauma (Angtika et al., Citation2018). They evaluated the effect of the addition of glycerol to the culture media of Acetobacter xylinum bacteria. The BC-glycerol samples were soaked in a chitosan solution (0.5%). Results showed that the increase of glycerol concentration in the culture media composition increased the tensile strength properties from 29.40 to 142.24 MPa. However, the glycerol did not improve significantly the elastic properties of the samples, showing a slight increase from 1.97 to 2.99% (Angtika et al., Citation2018). BC-glycerol-chitosan and BC-glycerol composite films were mainly evaluated for medical application. No studies have evaluated its potential applications as an active food packaging with UV radiation barrier properties.
Considering the lack of a complete characterization of the properties of these films to be applied as a food packa-ging material and based on previous research, the present study was focused on analyzing the physicochemical and functional properties of BC-glycerol-chitosan composite films. The effect of the presence of each component and their interactions was studied and compared with those of raw BC films. In part 1, the effect of the ratio of glycerol and chitosan was analyzed. Mathematical models were obtained to assess the effect of chitosan and glycerol on equilibrium moisture content, tensile properties (tensile strength, percentage of elongation, Young’s modulus and toughness) and puncture properties (burst strength and distance to burst) of BC samples.
2. Materials and methods
Raw BC films were elaborated using Komagataeibacter xylinus from the “Colección Española de Cultivos Tipo” (CECT). D(+)-glucose monohydrate (99% extra pure), sodium hydroxide (98%), extra pure anhydrous sodium bromide (99%), chitosan (Mw 100000–300000) and acetic acid (99.5%) were purchased from Acros organics (Geel, Belgium) and yeast extract was provided by Scharlau Microbiology (Barcelona, Spain). Glycerol was purchased from Fisher Scientific (Pittsburgh, PA, US).
2.1. Preparation of films
The elaboration of raw BC films has been described in detail elsewhere (Cazon et al., Citation2019b, Citation2019). Washed BC films were combined with glycerol and chitosan solutions, after removing the excess water from the BC samples using filter paper sheets to obtain the composite films. The combination of the films in different baths was carried out sequentially. A glycerol solution bath with concentrations ranging between 0 and 5% w/w and another aqueous chitosan solution bath at several concentration ranging between 0 and 1% w/w with acetic acid 1% (v/v) were prepared. BC-glycerol and BC-chitosan samples were obtained by dipping and soaking for 1 h pure BC pellicles into the glycerol or chitosan solution baths with a specific composition as indicated in the experimental design (). Then, the composite films were placed in a Petri dish and dried at room temperature for 48 h, easing the evaporation of the solvent to obtain the final sample. BC-glycerol-chitosan samples were elaborated from the BC-glycerol samples to facilitate the diffusion of the plasticizer into the BC matrix before blending with chitosan. BC samples were immersed first in a glycerol bath with a specific concentration for 1 h. These samples were dried at room temperature for 24 h to facilitate the partial evaporation of the solvent. Then, the BC-glycerol samples were immersed in a chitosan bath with a specific concentration for 1 h to obtain the BC-glycerol-chitosan films. Finally, BC-glycerol-chitosan samples were dried for 48 h to get samples ready for conditioning and subsequent measurement. The overall elaboration process of BC films combined with glycerol and chitosan is shown in .
Table 1. Experimental results for equilibrium moisture content and tensile properties (TS, tensile strength; %E, percentage of elongation; YM, Young’s modulus; T, toughness).
Tabla 1. Resultados experimentales para el contenido de humedad en equilibrio y propiedades de tracción (TS, resistencia a la tracción; % E, porcentaje de alargamiento; YM, módulo de Young; T, tenacidad)
Figure 1. Overall process of the elaboration of bacterial cellulose films combined with glycerol and chitosan. RT = room temperature.
Figura 1. Proceso general de elaboración de películas de celulosa bacteriana combinadas con glicerol y quitosano. RT = temperatura ambiente
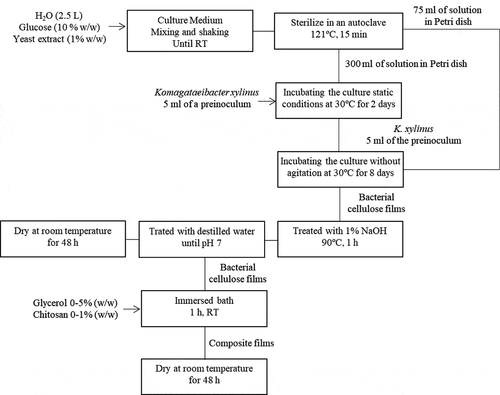
The composite films were cut to specific sizes depending on the test. The thickness (mm) was measured at five random locations using a thickness meter ET115S (Etari GmbH, Stuttgart, Germany). The samples were stored in desiccators with a saturated sodium bromide solution or silica gel for 5 days, as required for each test.
2.2. Equilibrium moisture content
The equilibrium moisture content was calculated by measuring the differences between the weights of conditioned and dried samples. Three samples (3 × 3 cm2) of each formulation were stored in desiccators at 57% relative humidity of equilibrium (RHeq) using a solution of sodium bromide saturated for 5 days. The weight of conditioned samples (initial weight) was measured using a precision balance. Then, the samples were dried at 105°C in a vacuum oven for 24 h until to reach constant weight and the dry weight was measured. Equilibrium moisture content (%) was calculated using EquationEquation 1(1)
(1) (Pinotti et al., Citation2007):
2.3. Mechanical properties analysis
The mechanical properties of the developed films were analyzed by tensile and puncture tests. Tensile strength (TS, MPa), percentage of elongation at break (%E, %), Young’s Modulus (YM, MPa), toughness (T, MJ/m3), burst strength (BS, g) and distance to burst (DB, mm) were measured as described elsewhere (Cazon et al., Citation2018a, Citation2019).
2.4. Statistical analysis
The experimental design denoted the independent variables glycerol and chitosan content as A and B, respectively. The effect of the glycerol and chitosan content on the dependent variables (moisture content, TS, %E, YM, T, BS, DB) was calculated and evaluated following a complete factorial design. Cook’s distance was used to detect outliers (Cook, Citation1977) and the Box-Cox data transformation technique was used to reduce anomalies (Sakia, Citation1992). The statistical analysis was accomplished using Design Expert® 10.0.6 software (Stat-Ease, Inc., Minneapolis, MN, USA).
3. Results
BC-glycerol-chitosan composite samples were obtained by dipping in baths with several concentrations of glycerol (0–5%) and chitosan (0–1%). The average thickness of the samples ranged between 2.10 · 10−2 and 2.98 · 10−2 mm. The thickness of the samples increased when the chitosan and glycerol content increased.
3.1. Equilibrium moisture content
Samples were conditioned at 57% RHeq before carrying out the mechanical and optical tests. The moisture content may depend on its composition due to the composite formulations (glycerol:chitosan ratio) and the hydrophilic characteristics of these components. The moisture content variation could have an important effect on the functional properties of films, such as the mechanical properties (Cazon et al., Citation2020a). Therefore, the initial analysis of the moisture content of each formulation is important when interpreting subsequent results.
The calculated equilibrium moisture content of the samples conditioned at 57% RHeq for 5 days ranged from 1.82 to 240.53%. indicates the average moisture content values for each experimental composition. Data were fitted using a natural logarithmic equation to obtain better fitted, as recommended the Box-Cox plot. The obtained mathematical model was significative, indicating that the moisture content of the samples depended on their formulation. The F-value and p-value of the model were 19.12 and 0.0036, respectively. The p-values of the model terms indicated that the linear and quadratic effect of glycerol, A and A2, respectively, were the only significative terms (p < .005). The linear (B) and quadratic (B2) effect of the chitosan concentration were no significant terms (p-value > 0.1000) (). According to the p-values, the model was reduced to the terms A, A2 and B in order to improve the model fit and the statistical parameters. The F-values of the terms allow determining which component has higher effect on the response. The linear effect of the glycerol concentration (F-value = 16.67) produced a strong effect on the moisture content properties, followed by the quadratic effect of glycerol concentration (F-value = 2.28).
Table 2. Analysis of variance (ANOVA) for moisture content and tensile properties (TS, tensile strength; %E, percentage of elongation; YM, Young’s modulus; T, toughness). A and B refer to glycerol (% w/w) and chitosan (% w/w), respectively.
Tabla 2. Análisis de varianza (ANOVA) para contenido de humedad y propiedades de tracción (TS, resistencia a la tracción; % E, porcentaje de alargamiento; YM, módulo de Young; T, tenacidad). A y B se refieren a glicerol (% p/p) y quitosano (% p/p), respectivamente
The fit statistics results indicated a r2 of 0.92. The adjusted r2 is used to compare the goodness-of-fit for regression models that contain differing numbers of independent variables. Predicted r2 measures how well the model predicts a response value. The difference between adjusted r2 and predicted r2 should be within 0.20, approximately to be in reasonable agreement. In this case, the predicted r2 (0.71) was in reasonable agreement with the adjusted r2 (0.87). Adequate precision is a signal/noise ratio used to compare the range of the predicted values at the design points to average prediction error. Ratio greater than 4 indicates adequate model discrimination. In this case, the adequate precision obtained was 10.39, implying an adequate signal ().
Table 3. Fit statistics of the obtained model for moisture content and tensile properties (TS, tensile strength; %E, percentage of elongation at break; YM, Young’s Modulus; T, toughness).
Tabla 3. Ajuste estadístico del modelo obtenido para contenido de humedad y propiedades de tracción (TS, resistencia a la tracción; % E, porcentaje de elongación a la rotura; YM, módulo de Young; T, tenacidad)
EquationEquation 2(2)
(2) predicts the moisture content response in terms of actual factors of glycerol and chitosan concentrations.
shows the response surface plot of the moisture content of BC given by EquationEquation 2(2)
(2) . Note that the samples suffered a drastic increase of the water content by adding glycerol. A sharp increase of the water content was observed at low glycerol content (2.5% w/w), from 1.82% (BC samples) up to 87.72% (BC-glycerol 2.5% w/w), followed by a soft increase of water content at higher glycerol concentration, reaching 91.10% for BC-glycerol 5% w/w.
Figure 2. Response surface graph of the predicted effect of glycerol and chitosan concentration on the equilibrium moisture content of bacterial cellulose films.
Figura 2. Gráfico de superficie de respuesta del efecto previsto de la concentración de glicerol y quitosano en el contenido de humedad de equilibrio de las películas de celulosa bacteriana
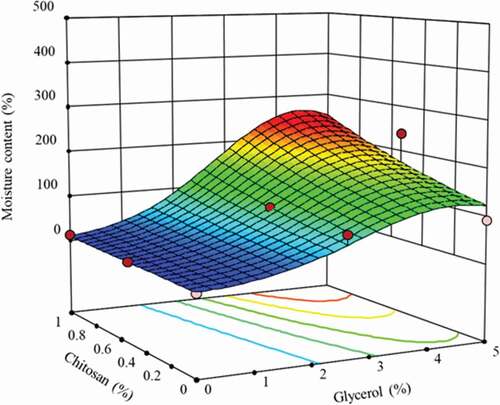
On the other side, the presence of chitosan promoted a lower water content than glycerol, reaching a maximum value of 12.55% at the higher chitosan concentration (BC-chitosan 1% w/w). The response of the equilibrium water content changed completely by the presence of both components, due to the physical interaction between chitosan-glycerol. The presence of glycerol in samples with chitosan produced a sharp increase of the moisture content values of the BC-glycerol-chitosan samples. However, a decrease of the moisture values was observed by increasing the chitosan concentration and keeping constant the glycerol content. Although both components present hydrophilic properties, glycerol is a known hygroscopic component with a stronger affinity by the surrounding environment water molecules than chitosan. Previous studies of adsorption isotherms of chitosan-glycerol (Monte et al., Citation2018), starch-glycerol mixtures (Enrione et al., Citation2007) microfibritalled cellulose-glycerol (Minelli et al., Citation2010) and BC-polyvinyl alcohol-glycerol (Cazon et al., Citation2019b) observed that the presence of glycerol resulted in a significant increase of the moisture content of the films. Besides, chitosan possesses free hydroxyl and amine groups which give it hydrophilic properties. In presence of glycerol, most of these groups interact with the components of the samples. Hence, they were not available thereafter for interacting with water molecules, resulting in a decrease of the moisture content. Similar behavior was observed in previous studies of chitosan films incorporated with citric acid and glycerol (Priyadarshi et al., Citation2018).
In the literature, pure BC samples showed a moisture content of 1.76% at 11% RHeq (Cazon et al., Citation2020a). Chitosan:glycerol samples with a ratio composition of 60:40 (w/w) showed a moisture content value of 24.3% after being stored for 3 weeks at 98% RHeq (Suyatma et al., Citation2005). The values obtained in the present study for BC-glycerol-chitosan composite films were significantly higher. The porous and 3D structure that forms the cellulose fibers resulted in a matrix with a greater contact surface. This structure allowed a better interaction among BC-glycerol-chitosan and polymer blend-water molecule, observing higher moisture contents than those of pure BC and chitosan:glycerol films.
3.2. Evaluation of the mechanical properties
The modification of the mechanical properties of BC films as a function of glycerol and chitosan was evaluated through tensile and puncture tests. shows the mean values of tensile properties and shows the mean values of the puncture properties.
The estimated TS values ranged between 1.23 and 39.28 MPa. Data were fitted to a square root model as recommended the Box-Cox plot. The F-value of 76.87 and p-value of 0.0023 indicated that the model was significant. The p-values showed that the linear effect of glycerol (A) and chitosan (B) and the quadratic effect to glycerol (B2) content had a significant effect on the response property. The F-values showed that glycerol content had a higher effect on the tensile response (F-value = 270.82), followed by the quadratic effect of glycerol content (F-value = 62.83) and the linear effect of chitosan content (F-value = 49.15). The glycerol–chitosan interactions and the quadratic effect of chitosan content had a negligible effect on the tensile response, as shown by their F-values (). The fit statistics values indicated a r2 value of 0.99. The predicted r2 value of 0.94 and the adjusted r2 value of 0.98 were in reasonable agreement and the adequate precision was 23.45.
EquationEquation 3(3)
(3) forecasts the TS values of the BC composite samples as a function of the percentage of glycerol and chitosan concentration. shows the response surface graph of the TS given by EquationEquation 3
(3)
(3) .
Figure 3. Response surface graph of the predicted effect of glycerol and chitosan concentration on tensile properties of bacterial cellulose films. A) Tensile strength (TS), B) Percentage of elongation at break (%E), C) Young’s Modulus (YM) and D) Toughness (T).
Figura 3. Gráfico de superficie de respuesta del efecto previsto de la concentración de glicerol y quitosano sobre las propiedades de tracción de las películas de celulosa bacteriana. A) Resistencia a la tracción (TS), B) Porcentaje de elongación a la rotura (% E), C) Módulo de Young (YM) y D) Tenacidad (T)
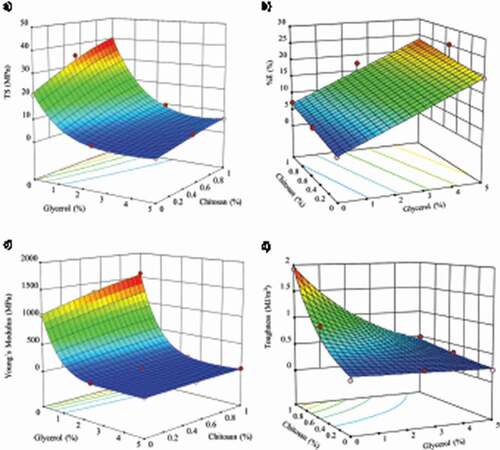
The results showed a drastic decrease in the TS values by the addition of glycerol. Low concentrations of glycerol were enough to produce a drastic drop of TS values from 20.76 MPa (BC samples) to 2.50 MPa (BC-glycerol 2.5% w/w). By contrast, chitosan helped to increase the TS values of BC films, doubling the resistance to break, until reaching values of 39.28 MPa (BC-chitosan 1% w/w).
The analysis of the elongation properties of the composite films showed %E values of the BC-glycerol-chitosan samples ranging between 2.28 and 25.34%. Data was well fitted to a linear model. Trial 4 was disregarded because it was identified as an outlier by the Cook’s distance test. The F-value of model was 30.67 and p-value was 0.0016, meaning the model was significant. Note that the p-values of the model terms indicated that only the glycerol concentration (p-value = 0.0006) was significant. The fit statistics values were r2 of 0.93, predicted r2 of 0.83 and adjusted r2 of 0.90. The values of predicted and adjusted r2 were in reasonable agreement, and adequate precision (12.75) indicated an adequate signal.
The elongation properties of the BC-glycerol-chitosan composite films are predicted using EquationEquation 4(4)
(4) . The corresponding response surface graph is shown in .
The response surface revealed that chitosan and glycerol improved the elongation properties of BC films. Chitosan produced a slightly increased of %E values from 2.28% to 7.08%. On the other hand, the glycerol effect on the elongation properties of the samples was much more significant and drastic, reaching values up to 25.34% by dipping in glycerol 2.5% (w/w) bath. However, the increase of glycerol content up to 5% (w/w) produced a slightly decreased of the elongation down to 19.21%. The plasticizing effect of glycerol predominated in the mechanical behavior of the composite films in samples with both components, glycerol and chitosan, reaching a value of 25.21% for samples combined with chitosan 0.5% (w/w) and glycerol 5% (w/w).
The calculated Young’s modulus (YM) values of the developed samples ranged from 11.72 to 1579.59 MPa. Data were fitted to a square root as recommended by the Box-Cox graph. The statistical analysis indicated that the model had an F-value of 2108.10 and p-value < 0.0001, implying the model was significant. According to the p-values, all terms were significant, except the quadratic effect of the chitosan content (p-value of 0.1380).
EquationEquation 5 predicts the YM values of the BC-glycerol-chitosan composite films and the corresponding response surface graph is shown in .
YM data and response surface graph followed a similar trend to TS response of the BC composite films by adding glycerol and/or chitosan. The presence of chitosan resulted in a raise of the YM values from 1043.88 MPa (BC films) to 1579.59 MPa (BC-chitosan 1% w/w). This behavior changed and suffered a sharp drop of YM values by adding glycerol, reaching a value of 13.43 MPa for BC-glycerol 2.5% (w/w) and a minimum value of 11.72 MPa in samples with glycerol 5% (w/w). In samples containing both components, the effect of glycerol predominated in YM values of the composite films.
The toughness values calculated ranged between 0.22 and 1.92 MJ/m3. Experiment 5 was ignored because it was identified as an outlier by the Cook’s distance test. The Box-Cox plot recommended transforming the experimental values to a natural logarithmic of T and a two-factor interaction mathematical model was used to fit the data. The F-value of the model was 8.79 and p-value was less than 0.0500, being significant. The p-values of the glycerol (p-value = 0.0367) and chitosan (p-value = 0.0108) content indicated that these terms were significant. Note that chitosan effect (F-value = 15.67) was higher than glycerol effect (F-value = 8.01) on the measured response. The p-value of glycerol–chitosan interaction (p-value = 0.1622) indicated that the glycerol–chitosan interaction was not significant for the toughness response. Regards the fit statistics values, the r2 was 0.95 and the predicted r2 (0.7639) was in reasonable agreement with the adjusted r2 (0.9104).
EquationEquation 6 estimates the T response as a function of the glycerol and chitosan concentration on the BC films. shows the resulted response surface graph.
The addition of glycerol produced no significant variation on the T values, resulting in a slight variation of the values from 0.26 MJ/m3 (BC samples) to 0.31 MJ/m3. The energy absorption capacity before the rupture increased due to the addition of chitosan, reaching a maximum value of 1.92 MJ/m3 for samples immersed in a 1% (w/w) chitosan bath.
Regarding the puncture test, BS and DB properties provide information about the resistance and elongation of the samples under a puncture force deformation. BS values () ranged from 43.74 to 1067.35 g and data were fitted to a two-factor interaction model with F-value of 16.50 and p-value of 0.0050. Only the concentration of chitosan (p-value 0.0014) was the significant term (). The r2 value was 0.91 and the predicted r2 (0.75) was in reasonable agreement with the adjusted r2 (0.85). The ratio of the adequate precision (10.90) was higher than 4, indicating an adequate signal ().
Table 4. Experimental results for puncture properties (burst strength, BS; distance to burst, DB).
Tabla 4. Resultados experimentales para las propiedades de perforación (resistencia al estallido, BS; distancia al estallido, DB)
Table 5. Analysis of variance (ANOVA) for puncture properties (BS, burst strength; DB, distance to burst). A and B refer to glycerol (% w/w) and chitosan (% w/w), respectively.
Tabla 5. Análisis de varianza (ANOVA) para propiedades de perforación (BS, resistencia al estallido; DB, distancia al estallido). A y B se refieren a glicerol (% p/p) y quitosano (% p/p), respectivamente
Table 6. Fit statistics of the obtained model for puncture properties (BS, burst strength; DB, distance to burst).
Tabla 6. Estadísticas de ajuste del modelo obtenido para las propiedades de perforación (BS, resistencia al estallido; DB, distancia al estallido)
EquationEquation 7(7)
(7) estimates the response on the BS as a function of the concentration of glycerol and chitosan on the BC composite films.
shows the response surface graph plotted using EquationEquation 7(7)
(7) . The incorporation of chitosan produced an important rise of the BS values from 58.88 g (BC samples) to a maximum value of 1067.35 g (BC-chitosan 1% w/w). On the other hand, the puncture resistance to break depended on the concentration of glycerol. A low glycerol content promoted a slight increase of the puncture resistance values to 115.37 g for BC-glycerol 2.5% w/w. Unlike the highest glycerol concentrations, it manifested a decrease in puncture resistance, diminishing to values of 43.74 g.
Figure 4. Response surface graph of the predicted effect of glycerol and chitosan concentration on puncture properties of bacterial cellulose films. A) Burst strength (BS) and B) Distance to burst (DB).
Figura 4. Gráfico de superficie de respuesta del efecto previsto de la concentración de glicerol y quitosano sobre las propiedades de punción de las películas de celulosa bacteriana. A) Resistencia al estallido (BS) y B) Distancia al estallido (DB)
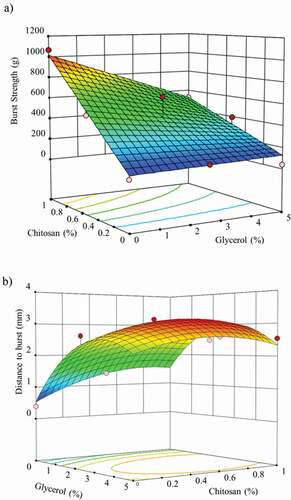
The elongation values of the samples under puncture forces ranged from 0.39 to 3.12 mm. The DB data fitted well to a quadratic model with an F-value of 9.69 implying that the model was significant. There was only a 4.53% chance (p-value of 0.0453) that an F-value this large could occur due to noise. The linear (p-value of 0.0218) and quadratic effect (p-value of 0.0211) of glycerol concentration were significant terms of the model, and both had a similar effect on the DB response, showing similar F values, being 19.35 and 19.84, respectively. The linear (p-value of 0.1016) and quadratic (p-value of 0.2184) effect of chitosan concentration and the interactions glycerol-chitosan (p-values of 0.3241) were not significant. The value of r2 was 0.94 and the predicted r2 (0.31) was not as close to the adjusted r2 (0.85) as one might normally expected. However, the value of adequate precision indicated an adequate signal-to-noise ratio. EquationEquation 8(8)
(8) predicts the DB response on the BC composite films as a function of the glycerol and chitosan concentrations.
shows the response surface graph resulted from EquationEquation 8(8)
(8) . Adding chitosan and glycerol improved the elongation properties of the films. DB values suffered a higher rise due to the effect of glycerol, from 0.39 mm (BC samples) to 2.60 mm (BC-glycerol 2.5% w/w) and due to the glycerol–chitosan interactions, reaching a maximum value of 3.12 mm (BC-glycerol 2.5% w/w – chitosan 0.5% w/w). Results indicated that the combination of plasticizing effect of glycerol at low concentrations and the reinforcing effect of chitosan resulted in a higher rise of the elongation properties of the BC films.
4. Discussion
The hydrophilic nature of chitosan and glycerol increased the equilibrium moisture content of the films when the glycerol and chitosan content. Samples stored at the same RHEq conditions showed different moisture content depending on their formulation, as discussed previously. Water molecules are a well-known plasticizer for polysaccharide-based films and they can modify their properties (Aguirre-Loredo et al., Citation2016; Cazon et al., Citation2019b; Meriçer et al., Citation2017; Sothornvit & Krochta, Citation2005). Previous study evaluating the effect of the moisture on BC films (Cazon et al., Citation2020a) reported that the active groups of the adsorbed water molecules interact with the cellulose fibers by hydrogen bonds, reducing the BC-BC interactions and promoting the swelling of the polymeric matrix. These changes resulted in a reorganization of the polymeric chains increasing the free volume within the BC matrix (Bedane et al., Citation2015; Cazon et al., Citation2020a; Chinnan & Park, Citation1995; Roy et al., Citation2000; Su et al., Citation2010). Therefore, a softening of the polysaccharide-based material and a loss of stiffness were observed due to the increase of the moisture content. Generally, it results in an increase of the elongation (%E and DB), a reduction of the mechanical resistance of the material (TS, T and BS values), and an increase of the deformation capability (low YM values) (Cazon et al., Citation2019b, Citation2020, Citation2020a, Citation2020c; Sirviö et al., Citation2018). The addition of glycerol and chitosan increased the moisture content enhancing the plasticizing effect.
Overall, the results of the mechanical properties suggested that glycerol mainly acted as a plasticizing agent and chitosan acted as a reinforcing agent. Based on the literature, cellulose fibers are secreted by K. xylinus in the liquid medium forming a 3D network of fibers. During the drying process, BC film loses water within the network structure, resulting in a more compact structure due to the large amounts of hydrogen bonds formed between the BC fibers (Cazon et al., Citation2020b). Hence, the inter- and intra-molecular interactions between cellulose fibers hinder the movement between the fibers, resulting in a strong but brittle material (Sun et al., Citation2018). TS values of pure BC films showed a wide range between 5 and 165 MPa (Cai & Kim, Citation2010; Phisalaphong & Jatupaiboon, Citation2008; Saibuatong & Phisalaphong, Citation2010; Sun et al., Citation2018; Szymańska-Chargot et al., Citation2017). Unlike TS values, the elongation values were in a similar narrow range between 0.6 and 5% (Cai & Kim, Citation2010; Sun et al., Citation2018). Pure BC films showed BS values ranging from 145 to 338 g and DB values ranging between 0.39 and 1.86 mm, depending on the moisture content (Cazon et al., Citation2020a). The good resistance to deformation (TS and BS) and low elongation properties (%E and DB) revealed the brittleness of the material. The wide range of the reported TS values could be due to several factors involved during the production and subsequent treatment of BC films. These factors have an impact on the thickness and on the assembly of the BC network, causing variations in the mechanical properties of the cellulose-based polymer (Cazon et al., Citation2019; Lin et al., Citation2013; Wang et al., Citation2019).
The addition of glycerol promoted structural changes that resulted in a plasticizing effect. Glycerol could work as internal “lubricant” that facilitated the mobility of the cellulose chains by reducing friction forces between fibers (Sothornvit & Krochta, Citation2005; Suyatma et al., Citation2005). The hydroxyl groups of glycerol could form hydrogen bonds with the BC fibers, breaking the polymer–polymer interactions and forming new polymer-plasticizer bonds. Therefore, glycerol could prevent the establishment of hydrogen bonds between the cellulose fibers which form a packed and rigid structure. Reducing the cellulose chain interactions could also increase the space among the fibers improving the elongation properties (%E and DB) and decreasing the resistance to deformation and rupture (TS, T and BS) (Cazon et al., Citation2020b; Sothornvit & Krochta, Citation2005; Sun et al., Citation2018; Suyatma et al., Citation2005). Previous studies have evaluated the effect of two plasticizers on BC samples depending on the hydroxyl group content and the hydrogen bond interaction (Sun et al., Citation2018). They observed that at higher hydroxyl group content, more hydrogen bonds are formed between plasticizer-BC. The presence of the plasticizer increases the deformation capability of the polymer (lower TS, YM, T and BS values) and eases the relative movement of the chains (higher% E and DB values) (Sirviö et al., Citation2018; Sun et al., Citation2018). Similar modifications of the mechanical properties by the presence of glycerol were observed in hydroxyethyl cellulose-based films (Sirviö et al., Citation2018), BC-based films (Cazon et al., Citation2020b; Cielecka et al., Citation2019; Sun et al., Citation2018), and chitosan (Priyadarshi et al., Citation2018), among other polysaccharide-based films.
Chitosan mainly had a reinforcing effect, increasing the mechanical and deformation resistance of the film. Hence, the TS, YM, T and BS values increased along with a noticeable improvement in the elastic properties. The final effect of chitosan on the BC matrix was the result of several factors. First, chitosan penetrated inside the BC matrix between the fibers, decreasing the porosity and increasing the film density. Chitosan has film-forming capacity, and it could create a continuous network interlocking chains within the BC matrix. The active groups of the chitosan can also interact with the cellulose chains and glycerol molecules, forming new hydrogen bonding. Previous work observed a decrease of the crystallinity of BC-chitosan composite compared to pure BC, implying that the interactions between the chitosan molecules with the BC chains by hydrogen bonds. As a result, when a deformation force is applied to the composite samples, the reinforcing effect of the chitosan network resulted in a composite material with an increased deformation resistance compared to pure BC samples (Cazon et al., Citation2019; Ul-Islam et al., Citation2011). The observed effect of chitosan and data obtained are in agreement with those obtained in previous studies of BC-chitosan (Ul-Islam et al., Citation2011) or BC-chitosan-polyvinyl alcohol films (Cazon et al., Citation2019).
Samples containing glycerol and chitosan showed a simultaneous plasticizing and reinforcing effect, respectively, being the effect of glycerol the predominant one. Consequently, despite the presence of chitosan, the values of resistance to mechanical rupture and deformation suffered a sharp decrease. Due to its high availability of active groups, glycerol interacted with cellulose fibers and chitosan molecules through hydrogen bonds. The new BC-glycerol-chitosan interactions resulted in composite films with higher elastic values (up to 25.21%) and higher resistance to rupture and mechanical deformation properties than those of BC-glycerol samples. When increasing the glycerol content, the chitosan–glycerol interactions also increased resulting in higher elongation values while maintaining the values of the mechanical resistance properties. Besides, BC-glycerol-chitosan samples showed the highest values of the moisture content, that acted as a plasticizer agent promoting the structural modifications and hydrogen bond interactions.
5. Conclusions
The incorporation of glycerol into the matrix following a simple immersion method was enough to observe a significant plasticizing effect on BC matrix, as shown by the mechanical results. The new plasticizer – polymer interactions, reducing the polymer – polymer interactions, produced a reduction in the stiffness of the structure, increasing the elongation of the film. Consequently, the plasticizing effect of glycerol produced a drastic reduction in the breaking strength of the samples. Chitosan promoted the formation of a connected internal network resulting in a reinforcing effect of the film which allowed obtaining more resistant films. The combination of the effect of both components in the BC matrix allowed developing more flexible biodegradable films with improved mechanical resistance properties. In presence of glycerol, the samples showed higher equilibrium moisture values that provided adhesive properties. The adhesive behavior can be an advantage for easy application on foods, such as fruit or vegetables, as a wrapping material.
Acknowledgments
A grant to author Patricia Cazón from CONACYT (Mexico) is gratefully acknowledged.
Disclosure statement
The authors declare that they have no conflicts of interest.
Additional information
Funding
References
- Aguirre-Loredo, R. Y., Rodríguez-Hernández, A. I., Morales-Sánchez, E., Gómez-Aldapa, C. A., & Velazquez, G. (2016). Effect of equilibrium moisture content on barrier, mechanical and thermal properties of chitosan films. Food Chemistry, 196, 560–566. https://doi.org/https://doi.org/10.1016/j.foodchem.2015.09.065
- Aider, M. (2010). Chitosan application for active bio-based films production and potential in the food industry: Review. LWT - Food Science and Technology, 43(6), 837–842. https://doi.org/https://doi.org/10.1016/j.lwt.2010.01.021
- Angtika, R. S., Widiyanti, P., & Aminatun. (2018). Bacterial cellulose-chitosan-glycerol biocomposite as artificial dura mater candidates for head trauma. Journal of Biomimetics, Biomaterials and Biomedical Engineering, 36, 7–16. https://doi.org/https://doi.org/10.4028/www.scientific.net/JBBBE.36.7
- Azeredo, H., Barud, H., Farinas, C. S., Vasconcellos, V. M., & Claro, A. M. (2019). Bacterial cellulose as a raw material for food and food packaging applications. Frontiers in Sustainable Food Systems, 3, 7. https://doi.org/https://doi.org/10.3389/fsufs.2019.00007
- Bedane, A. H., Eić, M., Farmahini-Farahani, M., & Xiao, H. (2015). Water vapor transport properties of regenerated cellulose and nanofibrillated cellulose films. Journal of Membrane Science, 493, 46–57. https://doi.org/https://doi.org/10.1016/j.memsci.2015.06.009
- Cai, Z., & Kim, J. (2010). Bacterial cellulose/poly(ethylene glycol) composite: Characterization and first evaluation of biocompatibility. Cellulose, 17(1), 83–91. https://doi.org/https://doi.org/10.1007/s10570-009-9362-5
- Cazon, P., & Vázquez, M. (2020). Mechanical and barrier properties of chitosan combined with other components as food packaging film. Environmental Chemistry Letters, 18, 257–267. Springer Verlag. https://doi.org/https://doi.org/10.1007/s10311-019-00936-3
- Cazon, P., Vazquez, M., & Velazquez, G. (2018a). Composite films of regenerate cellulose with chitosan and polyvinyl alcohol: Evaluation of water adsorption, mechanical and optical properties. International Journal of Biological Macromolecules, 117, 235–246. https://doi.org/https://doi.org/10.1016/j.ijbiomac.2018.05.148
- Cazon, P., Vazquez, M., & Velazquez, G. (2018b). Novel composite films based on cellulose reinforced with chitosan and polyvinyl alcohol: Effect on mechanical properties and water vapour permeability. Polymer Testing, 69(June), 536–544. https://doi.org/https://doi.org/10.1016/j.polymertesting.2018.06.016
- Cazon, P., Vázquez, M., & Velazquez, G. (2019a). Composite films with UV-barrier properties of bacterial cellulose with glycerol and poly(vinyl alcohol): Puncture properties, solubility, and swelling degree. Biomacromolecules, 20(8), 3115–3125. https://doi.org/https://doi.org/10.1021/acs.biomac.9b00704
- Cazon, P., Vázquez, M., & Velazquez, G. (2019b). Environmentally friendly films combining bacterial cellulose, chitosan, and polyvinyl alcohol: Effect of water activity on barrier, mechanical, and optical properties. Biomacromolecules, 21(2), 753–760. https://doi.org/https://doi.org/10.1021/acs.biomac.9b01457
- Cazon, P., Vázquez, M., & Velázquez, G. (2020). Regenerated cellulose films with chitosan and polyvinyl alcohol: Effect of the moisture content on the barrier, mechanical and optical properties. Carbohydrate Polymers, 236, 116031. https://doi.org/https://doi.org/10.1016/j.carbpol.2020.116031
- Cazon, P., Velázquez, G., & Vázquez, M. (2019). Characterization of bacterial cellulose films combined with chitosan and polyvinyl alcohol: Evaluation of mechanical and barrier properties. Carbohydrate Polymers, 216, 72–85. https://doi.org/https://doi.org/10.1016/j.carbpol.2019.03.093
- Cazon, P., Velázquez, G., & Vázquez, M. (2020a). Bacterial cellulose films: Evaluation of the water interaction. Food Packaging and Shelf Life, 25, 100526. https://doi.org/https://doi.org/10.1016/j.fpsl.2020.100526
- Cazon, P., Velázquez, G., & Vázquez, M. (2020b). Characterization of mechanical and barrier properties of bacterial cellulose, glycerol and polyvinyl alcohol (PVOH) composite films with eco-friendly UV-protective properties. Food Hydrocolloids, 99, 105323. https://doi.org/https://doi.org/10.1016/j.foodhyd.2019.105323
- Cazon, P., Velázquez, G., & Vázquez, M. (2020c). Regenerated cellulose films combined with glycerol and polyvinyl alcohol: Effect of moisture content on the physical properties. Food Hydrocolloids, 103, 105657. https://doi.org/https://doi.org/10.1016/j.foodhyd.2020.105657
- Chinnan, M. S., & Park, H. J. (1995). Effect of plasticizer level and temperature on water vapor transmission of cellulose-based films. Journal of Food Process Engineering, 18(4), 417–429. https://doi.org/https://doi.org/10.1111/j.1745-4530.1995.tb00375.x
- Cielecka, I., Szustak, M., Kalinowska, H., Gendaszewska-Darmach, E., Ryngajłło, M., Maniukiewicz, W., & Bielecki, S. (2019). Glycerol-plasticized bacterial nanocellulose-based composites with enhanced flexibility and liquid sorption capacity. Cellulose, 26(9), 5409–5426. https://doi.org/https://doi.org/10.1007/s10570-019-02501-1
- Cook, R. D. (1977). Detection of influential observations in linear regression. Technometrics,19(1), 15–18. https://doi.org/https://doi.org/10.2307/1268249
- Enrione, J. I., Sandra, E., Hill, A., & Mitchell, J. R. (2007). Sorption Behavior of Mixtures of Glycerol and Starch. J. Agric. Food Chem. https://doi.org/https://doi.org/10.1021/JF062186C
- Gromovykh, T. I., Pigaleva, M. A., Gallyamov, M. O., Ivanenko, I. P., Ozerova, K. E., Kharitonova, E. P., Bahman, M., Feldman, N. B., Lutsenko, S. V., & Kiselyova, O. I. (2020). Structural organization of bacterial cellulose: The origin of anisotropy and layered structures. Carbohydrate Polymers, 237, 116140. https://doi.org/https://doi.org/10.1016/j.carbpol.2020.116140
- Janjarasskul, T., & Suppakul, P. (2018). Active and intelligent packaging: The indication of quality and safety. Critical Reviews in Food Science and Nutrition, 58(5), 808–831. https://doi.org/https://doi.org/10.1080/10408398.2016.1225278
- Jozala, A. F., de Lencastre-novaes, L. C., Lopes, A. M., de Carvalho Santos-ebinuma, V., Mazzola, P. G., Pessoa-Jr, A., ... & Chaud, M. V. (2016). Bacterial nanocellulose production and application: A 10-year overview. Applied microbiology and biotechnology, 100(5), 2063–2072. https://doi.org/https://doi.org/10.1007/s00253-015-7243-4
- Keshk, S. M. (2014). Bacterial cellulose production and its industrial applications. Journal of Bioprocessing & Biotechniques, 04(2), 1–10. https://doi.org/https://doi.org/10.4172/2155-9821.1000150
- Khan, M. R., Sadiq, M. B., & Mehmood, Z. (2020). Development of edible gelatin composite films enriched with polyphenol loaded nanoemulsions as chicken meat packaging material. CYTA - Journal of Food, 18(1), 137–146. https://doi.org/https://doi.org/10.1080/19476337.2020.1720826
- Khemir, M., Besbes, N., Khemis, I. B., Di Bella, C., Lo Monaco, D., & Sadok, S. (2020). Determination of shelf-life of vacuum-packed sea bream (Sparus aurata) fillets using chitosan-microparticles-coating. CYTA - Journal of Food, 18(1), 51–60. https://doi.org/https://doi.org/10.1080/19476337.2019.1696893
- Lin, W.-C., Lien, -C.-C., Yeh, H.-J., Yu, C.-M., & Hsu, S. (2013). Bacterial cellulose and bacterial cellulose–chitosan membranes for wound dressing applications. Carbohydrate Polymers, 94(1), 603–611. https://doi.org/https://doi.org/10.1016/j.carbpol.2013.01.076
- Meriçer, Ç., Minelli, M., Giacinti Baschetti, M., & Lindström, T. (2017). Water sorption in microfibrillated cellulose (MFC): The effect of temperature and pretreatment. Carbohydrate Polymers, 174, 1201–1212. https://doi.org/https://doi.org/10.1016/j.carbpol.2017.07.023
- Minelli, M., Baschetti, M. G., Doghieri, F., Ankerfors, M., Lindström, T., Siró, I., & Plackett, D. (2010). Investigation of mass transport properties of microfibrillated cellulose (MFC) films. Journal of Membrane Science, 358(1–2), 67–75. https://doi.org/https://doi.org/10.1016/J.MEMSCI.2010.04.030
- Monte, M. L., Moreno, M. L., Senna, J., Arrieche, L. S., & Pinto, L. A. A. (2018). Moisture sorption isotherms of chitosan-glycerol films: Thermodynamic properties and microstructure. Food Bioscience, 22, 170–177. https://doi.org/https://doi.org/10.1016/j.fbio.2018.02.004
- Montilla, A., Ruiz-Matute, A. I., & Corzo, N. (2013). Biological effects and extraction processes used to obtain marine chitosan. In Blanca Hernández‐Ledesma & Miguel Herrero (Eds.), Bioactive compounds from marine foods (pp. 193–217). John Wiley & Sons, Ltd.https://doi.org/https://doi.org/10.1002/9781118412893.ch10
- Morin-Crini, N., Lichtfouse, E., Torri, G., & Crini, G. (2019). Applications of chitosan in food, pharmaceuticals, medicine, cosmetics, agriculture, textiles, pulp and paper, biotechnology, and environmental chemistry. Environmental Chemistry Letters, 17, 1667–1692. https://doi.org/https://doi.org/10.1007/s10311-019-00904-x
- Mortensen, A., Aguilar, F., Crebelli, R., Di Domenico, A., Dusemund, B., Frutos, M. J., Galtier, P., Gott, D., Gundert-Remy, U., Leblanc, J.-C., Lindtner, O., Moldeus, P., Mosesso, P., Parent-Massin, D., Oskarsson, A., Stankovic, I., Waalkens-Berendsen, I., Woutersen, R. A., & Lambré, C., & (ANS), E. P. on F. A. and N. S. added to F.. (2017). Re-evaluation of glycerol (E 422) as a food additive. EFSA Journal, 15(3), e04720. https://doi.org/https://doi.org/10.2903/j.efsa.2017.4720
- Pang, M., Cao, L., Cao, L., Sheb, Y., & Wang, H. (2019). Properties of nisin incorporated ZrO2/poly (vinyl alcohol) - wheat gluten antimicrobial barrier films. CyTA - Journal of Food, 17(1), 400–407. https://doi.org/https://doi.org/10.1080/19476337.2019.1587517
- Phisalaphong, M., & Jatupaiboon, N. (2008). Biosynthesis and characterization of bacteria cellulose–chitosan film. Carbohydrate Polymers, 74(3), 482–488. https://doi.org/https://doi.org/10.1016/j.carbpol.2008.04.004
- Pinotti, A., García, M. A., Martino, M. N., & Zaritzky, N. E. (2007). Study on microstructure and physical properties of composite films based on chitosan and methylcellulose. Food Hydrocolloids, 21(1), 66–72. https://doi.org/https://doi.org/10.1016/j.foodhyd.2006.02.001
- Priyadarshi, R., Sauraj, Kumar, B., & Negi, Y. S. (2018). Chitosan films incorporated with citric acid and glycerol as an active packaging material for extension of green chilli shelf life. Carbohydrate Polymers, 195(December 2017), 329–338. https://doi.org/https://doi.org/10.1016/j.carbpol.2018.04.089
- Rabea, E. I., Badawy, M. E. T., Stevens, C. V., Smagghe, G., & Steurbaut, W. (2003). Chitosan as antimicrobial agent: Applications and mode of action. Biomacromolecules, 4(6), 1457–1465. https://doi.org/https://doi.org/10.1021/bm034130m
- Reiniati, I., Hrymak, A. N., & Margaritis, A. (2017). Recent developments in the production and applications of bacterial cellulose fibers and nanocrystals. Critical Reviews in Biotechnology, 37(4), 510–524. Taylor and Francis Ltd. https://doi.org/https://doi.org/10.1080/07388551.2016.1189871
- Robertson, G. L. (2012). Food Packaging. Principles and Practice (3rd Editio ed.). CRC Press. https://doi.org/https://doi.org/10.1201/b21347
- Roy, S., Gennadios, A., Weller, C. L., & Testin, R. F. (2000). Water vapor transport parameters of a cast wheat gluten film. Industrial Crops and Products, 11(1), 43–50. https://doi.org/https://doi.org/10.1016/S0926-6690(99)00032-1
- Rühs, P. A., Malollari, K. G., Binelli, M. R., Crockett, R., Balkenende, D. W. R., Studart, A. R., & Messersmith, P. B. (2020). Conformal bacterial cellulose coatings as lubricious surfaces. ACS Nano, 14(4), 3885–3895. https://doi.org/https://doi.org/10.1021/acsnano.9b09956
- Saibuatong, O., & Phisalaphong, M. (2010). Novo aloe vera–bacterial cellulose composite film from biosynthesis. Carbohydrate Polymers, 79(2), 455–460. https://doi.org/https://doi.org/10.1016/j.carbpol.2009.08.039
- Sakia, R. M. (1992). The Box-Cox Transformation Technique: A Review. The Statistician, 41(2), 169. https://doi.org/https://doi.org/10.2307/2348250
- Sirviö, J. A., Visanko, M., Ukkola, J., & Liimatainen, H. (2018). Effect of plasticizers on the mechanical and thermomechanical properties of cellulose-based biocomposite films. Industrial Crops and Products, 122, 513–521. https://doi.org/https://doi.org/10.1016/j.indcrop.2018.06.039
- Sothornvit, R., & Krochta, J. M. (2005). Plasticizers in edible films and coatings. Innovations in Food Packaging, 403–433. https://doi.org/https://doi.org/10.1016/B978-012311632-1/50055-3
- Su, J. F., Huang, Z., Zhao, Y. H., Yuan, X. Y., Wang, X. Y., & Li, M. (2010). Moisture sorption and water vapor permeability of soy protein isolate/poly(vinyl alcohol)/glycerol blend films. Industrial Crops and Products, 31(2), 266–276. https://doi.org/https://doi.org/10.1016/j.indcrop.2009.11.010
- Sun, Y., Meng, C., Zheng, Y., Xie, Y., He, W., Wang, Y., Qiao, K., & Yue, L. (2018). The effects of two biocompatible plasticizers on the performance of dry bacterial cellulose membrane: A comparative study. Cellulose, 25(10), 5893–5908. https://doi.org/https://doi.org/10.1007/s10570-018-1968-z
- Suyatma, N. E., Tighzert, L., Copinet, A., & Coma, V. (2005). Effects of hydrophilic plasticizers on mechanical, thermal, and surface properties of chitosan films. Journal of Agricultural and Food Chemistry, 53(10), 3950–3957. https://doi.org/https://doi.org/10.1021/jf048790+
- Szymańska-Chargot, M., Chylińska, M., Cybulska, J., Kozioł, A., Pieczywek, P. M., & Zdunek, A. (2017). Simultaneous influence of pectin and xyloglucan on structure and mechanical properties of bacterial cellulose composites. Carbohydrate Polymers, 174, 970–979. https://doi.org/https://doi.org/10.1016/j.carbpol.2017.07.004
- Tsouko, E., Kourmentza, C., Ladakis, D., Kopsahelis, N., Mandala, I., Papanikolaou, S., ... & Koutinas, A. (2015). Bacterial cellulose production from industrial waste and by-product streams. International Journal of Molecular Sciences, 16(7), 14832–14849. https://doi.org/https://doi.org/10.3390/ijms160714832
- Ul-Islam, M., Shah, N., Ha, J. H., & Park, J. K. (2011). Effect of chitosan penetration on physico-chemical and mechanical properties of bacterial cellulose. Korean Journal of Chemical Engineering, 28(8), 1736–1743. https://doi.org/https://doi.org/10.1007/s11814-011-0042-4
- Wang, J., Tavakoli, J., & Tang, Y. (2019). Bacterial cellulose production, properties and applications with different culture methods – A review. Carbohydrate Polymers, 219, 63–76. https://doi.org/https://doi.org/10.1016/j.carbpol.2019.05.008
- Wyrwa, J., & Barska, A. (2017). Innovations in the food packaging market: Active packaging. European Food Research and Technology, 243(10), 1681–1692. https://doi.org/https://doi.org/10.1007/s00217-017-2878-2