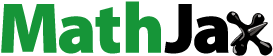
ABSTRACT
The aim of this study was to evaluate the antioxidant activity of exopolysaccharides (EPS) from Lactobacillus kimchi SR8. The EPS exhibited strong scavenging activities for 1,1-diphenyl-2-picrylhydrazyl and hydroxyl radicals. Two EPS fractions (EPS-1 and EPS-3) exhibited higher antioxidant activities than EPS-2. Low-dose CEPS could significantly increase the activities of catalase (CAT) and glutathione peroxidase (GSH-Px) in the serum and the activities of CAT, GSH-Px and superoxide dismutase (SOD) and total antioxidant activity in the liver. Medium- and high-dose CEPS could significantly increase the activities of CAT and GSH-Px and total antioxidant activity in the serum and the activities of CAT, GSH-Px, and SOD and total antioxidant activity in the liver. High-dose CEPS significantly increased the liver index of mice and the activity of SOD in the serum. CEPS could inhibit the formation of malondialdehyde (MDA) in the serum and liver. This suggests that EPS from Lactobacillus kimchi SR8 possesses potential antioxidant activities.
Resumen
El objetivo del presente estudio fue evaluar la actividad antioxidante de los exopolisacáridos (EPS) de Lactobacillus kimchi SR8. Estos mostraron una fuerte actividad de eliminación de los radicales 1,1-difenil-2-picrilhidrazilo e hidroxilo. Dos fracciones de EPS (EPS-1 y EPS-3) exhibieron mayor actividad antioxidante que EPS-2. Las dosis bajas de CEPS elevaron significativamente la actividad de la catalasa (CAT) y la glutatión peroxidasa (GSH-Px) en el suero, la actividad de la CAT, la GSH-Px y la superóxido dismutasa (SOD) y la actividad antioxidante total en el hígado. Las dosis medias y altas de CEPS incrementaron de manera significativa la actividad de la CAT y la GSH-Px, la actividad antioxidante total en el suero, la actividad de la CAT, la GSH-Px y la SOD, así como la actividad antioxidante total en el hígado. Las dosis altas de CEPS aumentaron significativamente el índice hepático de los ratones y la actividad de la SOD en el suero. El CEPS pudo inhibir la formación de malondialdehído (MDA) en el suero y el hígado. Estos resultados dan cuenta de que el EPS de Lactobacillus kimchi SR8 posee actividades antioxidantes potenciales.
1. Introduction
Exopolysaccharides (EPS) are high molecular weight biopolymers that are produced and secreted into the environment by a variety of microorganisms, such as lactic acid bacteria, yeast, fungi, and others (Zannini et al., Citation2016). Among the EPS-producing lactic acid bacteria, these include lactobacillus, bifidobacterium, leuconostoc, Pediococcus, and streptococcus (Kim et al., Citation2018; Matsuzaki et al., Citation2017; Pachekrepapol et al., Citation2017; Shin et al., Citation2016; Yan et al., Citation2017). In recent years, EPS from lactic acid bacteria have received much attention for being generally recognized as safe (GRAS) of food-grade status (Ahmed et al., Citation2013; Nadkarni et al., Citation2014; Zhang et al., Citation2017). The EPS-producing lactic acid bacteria are important in a number of industries, such as developing functional products and as starters to produce fermented products such as yoghurt (Mende et al., Citation2016; Zannini, Citation2015; Zannini et al., Citation2016), cheese (Liu et al., Citation2013), Chinese sauerkraut (Lu et al., Citation2017), and bread products (Lynch et al., Citation2018). In fermented products, lactic acid bacteria produce EPS in situ, and may improve the physical and chemical characteristics of fermented products, such as texture, rheology, flocculating activity, oil binding, and water-holding capacity (Gentes et al., Citation2016; Han et al., Citation2016; Rajoka et al., Citation2018; Z Xu et al., Citation2018). Moreover, these EPS have been reported to possess immunomodulatory properties (Kukihi & Afiati, Citation2016), antioxidant activity (Wang et al., Citation2017), antibacterial activity (Jeong et al., Citation2017), antitumor activity (Rajoka et al., Citation2018), cholesterol-lowering capacity (Seesuriyachan et al., Citation2011), and probiotic effects (Salazar et al., Citation2016).
Due to the biotechnological and functional properties of EPS produced by lactic acid bacteria, many researchers are focusing on the screening, isolation, characterization, biosynthesis, rheological and functional properties of the EPS produced by lactic acid bacteria (Saravanan & Shetty, Citation2016; Z Xu et al., Citation2018; Zannini et al., Citation2016). Thus far, the main EPS-producing lactic acid bacteria strains were Lactobacillus (Lb.) plantarum, Lb. rhamnosus, Lb. helveticus, Lb. delbrueckii subsp. Bulgaricus, Lb. kefiranofaciens, Lb. Kimchi, Lb. acidophilus, Lb. paracasei, Lactococcus lactis subsp. Lactis, Bifidobacterium bifidum, Leuconostoc mesenteroides, Leuconostoc citreum, Leuconostoc garlicum, Pediococcus pentosaceus, Weissella cibaria, and Streptococcus thermophilus (Adesulu-Dahunsi et al., Citation2018; Balzaretti et al., Citation2017; Cheirsilp et al., Citation2018; Domingos-Lopes et al., Citation2017; El-Deeb et al., Citation2018; Hong et al., Citation2017; Matsuzaki et al., Citation2017; Zhang et al., Citation2017). Most studies have focused on EPS produced by Lb. plantarum, Lb. rhamnosus, Lb. delbrueckii subsp. Bulgaricus, and Pediococcus pentosaceus (Ng & Xue, Citation2017; Sasikumar et al., Citation2017; Zhang et al., Citation2017). We previously reported the factors that affect EPS production of Lactobacillus (Lb.) kimchi SR8 and its 1,1-diphenyl-2-picrylhydrazyl (DPPH) radical scavenging activity (Zhang et al., Citation2017). However, no published studies have characterized the purification of EPSs from Lb. kimchi and measured its in vivo or in vitro antioxidant activity.
Sour meat is a commonly consumed fermented meat product in China, which is naturally fermented by microorganisms with fresh pork as the raw material and spices, such as rice flour and salt (Zhang et al., Citation2020). The Lb. kimchi SR8 was previously isolated from sour meat and was shown to have EPS-producing properties (Zhang et al., Citation2017). In this study, the purification of EPS from Lb. kimchi SR8 was carried out by DEAE-Cellulose Fast Flow ion-exchange chromatography and the in vitro and in vivo antioxidant activities were measured.
2. Materials and methods
2.1. Bacterium and media
The EPS-producing bacterium, Lactobacillus (Lb.) kimchi SR8, previously isolated from fermented sour meat of the Dong of China (Zhan et al., Citation2013), was used in this study. The strain was maintained at −80°C in MRS broth supplemented with 20% (v/v) glycerol for long-term preservation and at 4°C in MRS broth for short-term storage, and was regrown twice at 37°C for 18–20 h prior to experiments. According to the results of our medium optimization for EPS yield, the medium was prepared with the same components as MRS broth except that sucrose (30 g/L) was used in place of glucose. Subsequently, this medium was used for EPS quantification and enrichment.
2.2. Extraction, spectroscopic analysis, and purification of EPS
Fresh medium was inoculated (3%, v/v) with Lb. kimchi SR8 from logarithmic growth phase culture suspensions and incubated at 40°C for 24 h. After the incubation, CEPS were extracted according to the method described by Yang et al. (Citation2018). The method was modified by using a final trichloroacetic acid (TCA) concentration of 4% for protein removal, and by using three-fold volumes of absolute ethanol for exopolysaccharide precipitation at 4°C for 24 h. Then, CEPS were freeze-dried using an FD-1A-50 vacuum freeze dryer (Beijing Biocool Lab Equipment Co., Ltd., China), and the freeze-dried CEPS were stored at 4°C. EPS was quantified in accordance with phenol-sulfuric acid method using glucose as a standard (Saravanan & Shetty, Citation2016).
Quantification of protein and nucleic acid components in the crude exopolysaccharides was performed by measuring the UV spectrum from 200 to 400 nm using a quartz cuvette with a 1 cm path length using a SpectraMax 190 Microplate Reader (SpectraMax M2e, Molecular Devices, Sunnyvale, CA)
Subsequently, 0.1 g of freeze-dried CEPS powder was dissolved in 2 mL deionized water and subjected to a diethylaminoethyl (DEAE)-Cellulose column (diameter 1.6 cm × 70 cm). The column was initially equilibrated with 200–300 mL deionized water and then EPS fractions were prepared in stepwise elution with 0, 0.2, 0.5, and 1.0 mol/L sodium chloride solution at a flow rate of 1.0 mL/min, respectively. Samples containing 6 mL per tube were collected with a fraction collector and the polysaccharide content was determined by the phenol-sulfuric acid method.
2.3. Measuring antioxidant activity in vitro
2.3.1. 1,1-diphenyl-2-picrylhydrazyl (DPPH) radical scavenging activity
A series of samples and ascorbic acid (Vc) solutions with different concentrations of crude exopolysaccharides (CEPS) (0, 1, 2, 4, and 8 mg/mL) and 81 μg/mL CEPS, E1, E2, and E3 solutions were prepared, respectively. The 1,1-diphenyl-2-picrylhydrazyl (DPPH) radical scavenging activity was measured according to the method described by Du et al. (Citation2018) with slight modification. Briefly, 4 mL of each sample solution was mixed with 4 mL of 0.2 mmol/L DPPH radical-ethanol solution. The mixture was left for 30 min at room temperature in the dark. Then, the absorbance of the sample solution (Asample) was measured against a blank containing only ethanol (Ablank) at 517 nm. The DPPH radical scavenging activity was calculated using the following equation:
2.3.2. Hydroxyl radical scavenging activity
Hydroxyl radical scavenging activity was measured using Fenton’s reaction in accordance with the method of Li et al. (Citation2014) in triplicate. Briefly, the reaction system was prepared by mixing several solutions into 2 mL of phosphate buffer solution (pH 7.4) in the following order: 1 mL of water, 1 mL of 1,10-phenanthroline ethanol solution (0.75 mmol/L), 1 mL of ferrous sulfate (0.75 mmol/L), and 1 mL of hydrogen peroxide (0.01%). The final mixture was incubated at 37°C for 90 min. Afterward, the absorbance of the blank solution (Ablank, 1,10-phenanthroline, and ferrous sulfate), the control solution (Acontrol, containing 1,10-phenanthroline, ferrous sulfate, and hydrogen peroxide), and the sample solution (Asample, containing the sample) were measured at 536 nm. The scavenging activity was calculated using the following equation:
2.4. Animal experiments
2.4.1. Experimental animals
A total of 60 healthy SPF-grade male Kunming mice were kept to acclimatize under the same condition for a week. The animals were housed in clean cages and maintained under the same standard laboratory conditions of humidity (60 ± 5%), temperature (22–25°C), and controlled environment (natural light/dark cycle). They had free access to water and standard pellet. All experimental procedures were carried out on the basis of the guidelines of the Institutional Animal Ethics Committee (IAEC).
2.4.2. Experimental design
After the acclimatization period, 60 experimental mice reaching 18 g – 22 g, were randomly divided into six groups, with ten mice in each group. The experimental mice in each group were treated according to the method of Luo et al. (Citation2012) with the following modifications:
Group I – fed on pellet and normal saline (Normal control);
Group II – received 200 mg/kg body weight of 100 mg/mL D-galactose and an equivalent volume of normal saline orally for four weeks (Model group);
Group III – received 200 mg/kg body weight of 100 mg/mL D-galactose and 50 mg/kg body weight of 10 mg/mL CEPS orally for four weeks (Low-dose group);
Group IV – received 200 mg/kg body weight of 100 mg/mL D-galactose and 100 mg/kg body weight of 10 mg/mL CEPS orally for four weeks (Medium-dose group);
Group V – received 200 mg/kg body weight of 100 mg/mL D-galactose and 200 mg/kg body weight of 10 mg/mL CEPS orally for four weeks (High-dose group);
Group VI – received 200 mg/kg body weight of 100 mg/mL D-galactose and 100 mg/kg body weight of 10 mg/mL Vc orally for four weeks (Positive control)
2.4.3. Measurement of body weight
The initial and final body weights of the experimental animals were measured with an electronic balance before and after the experiment. The weight gain was calculated as the difference of the initial and final weight values.
2.4.4. Preparation of serum and liver homogenate supernatant
At the end of the experiment, all experimental animals were required to fast overnight (12 h) and then sacrificed by cervical dislocation following collection of eyeball blood. The serum was separated from the blood by centrifugation at 3000 rpm for 10 min. After the experimental animals were sacrificed, the livers were separated, weighed, homogenized for 10% liver homogenate with normal saline through ultrasonic pulverization under ice-bath condition and finally centrifuged at 3000 rpm for 10 min to collect the homogenate supernatant. The levels of the following antioxidant parameters were measured from the serum and liver homogenate supernatant: total antioxidant capacity (T-AOC), catalase (CAT), glutathione peroxidase (GSH-Px), superoxide dismutase (SOD) and malondialdehyde (MDA).
Liver protein content was measured in accordance with the method described by Bradford (Citation1976) using bovine serum albumin as a standard. Briefly, 0.1 mL of sample solution was mixed with 5 mL of Coomassie brilliant blue G250 solution. The mixture was left for 10 min at room temperature. Then, the absorbance of the sample solution (Asample) was measured against a blank (sample replaced with normal saline) at 595 nm.
2.4.5. Determination of liver index
The liver index was calculated on the basis of liver weight and body weight using the following equation:
2.4.6. Total antioxidant capacity
The total antioxidant capacity (T-AOC) of EPS was measured in triplicate using a total antioxidant capacity assay kit with the Ferric Reducing Ability of Plasma (FRAP) method (Beyotime Institute of Biotechnology, Haimen, China), described by Sun et al. (Citation2014). A 100 mmol/L ferrous sulfate solution was prepared using 200 mg of Green vitriol. A working solution was freshly prepared by mixing the DL-dithiothreitol (TPTZ) diluent, the TPTZ solution and the detection buffer in a ratio of 10:1:1 (v/v), respectively, and maintained at 37°C before experimental use. A 5 µL aliquot of the sample (the serum and the liver homogenate supernatant, respectively) was used to react with 180 uL of the FRAP working solution for 5 min at 37°C. The absorbance of the mixture was then measured at 593 nm. Distilled water was used instead of a sample for the blank and a standard curve was generated using ferrous sulfate in a concentration range of 0.15–1.5 mmol/L. The results were expressed as ferrous sulfate values calculated in accordance with the standard curve.
2.4.7. Enzymatic measurement
The serum and the liver homogenate supernatant were prepared for catalase (CAT), glutathione peroxidase (GSH-Px) and superoxide dismutase (SOD) assay. Catalase activity of the serum and liver tissue were measured using a catalase (CAT) assay kit (Nanjing Jiancheng Bioengineering Institute, Catalog no. A007-1-1, China) according to the manufacturer’s instructions. GSH-Px activity was analyzed using a GSH-Px assay kit (Nanjing Jiancheng Bioengineering Institute, Catalog no. A005, China) according to the manufacturer’s instructions. SOD activity was measured using a SOD assay kit (Nanjing Jiancheng Bioengineering Institute, Catalog no. A001-1-1, China) according to the manufacturer’s instructions.
2.4.8. Measurement of malondialdehyde
The content of malondialdehyde (MDA) in the serum and the liver were analyzed using an MDA assay kit (Nanjing Jiancheng Bioengineering Institute, Catalog no. A003-1, China) according to the manufacturer’s instructions. MDA can form an adduct (MDA-TBA) with thiobarbituric acid (TBA) in a 1:2 ratio. The adduct was measured at 530 nm using an UNICAM Helyos spectrophotometer. The level of thiobarbituric acid reactive substances (TBARS) was determined from a MDA equivalence standard. The MDA value was expressed as nmol/mg total protein (nmol/mL).
2.5. Statistical analysis
All data were analyzed with the Analysis of Variance (ANOVA) using SPSS 16.0 software (version 16.0, SPSS Inc., USA). All experimental results were presented as mean ± standard deviation (n = 3). Probability values (p = .05 and p = .01) were considered significant to indicate differences. The Duncan’s multiple comparison (p = .05 and p = .01) were used to indicate significant difference.
3. Results
3.1. Extraction, purification, and spectroscopic analysis of EPS
CEPS isolated from culture supernatant of Lactobacillus kimchi SR8 were prepared by protein removal using 80% TCA and ethanol precipitation. The CEPS were obtained after 24 h incubation in optimized medium at 40°C, with a total concentration of 627.50 mg/L, and a CEPS extraction yield of 342.30 mg/L. Spectroscopic analysis of CEPS indicated no nucleic acids in the sample (). Afterward, the CEPS were purified on a DEAE-Cellulose ion-exchange chromatography (). The elution profile showed three relatively symmetrical CEPS peaks. All fractions corresponding to major peaks eluted with 0 (EPS-1), 0.2 (EPS-2) and 0.5 mol/L (EPS-3) sodium chloride and were found to contain polysaccharides. The EPS-1 fraction was eluted with deionized water, suggesting the fraction was comprised of neutral polysaccharides. The EPS-2 and EPS-3 fractions eluted with increasing concentrations of sodium chloride solution, respectively, indicating the presence of negatively charged, acidic polysaccharides, when compared with EPS-1.
Figure 1. The UV spectrum of crude exopolysaccharides (CEPS) from Lactobacillus kimchi SR8.
Figura 1. Espectro UV de los exopolisacáridos crudos (CEPS) de Lactobacillus kimchi SR8
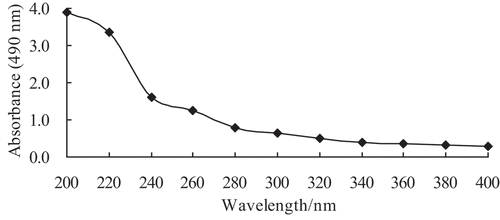
Figure 2. The elution profile of crude exopolysaccharides (CEPS) from Lactobacillus kimchi SR8 on a DEAE Cellulose ion-exchange column chromatography with a gradient of sodium chloride solution (0, 0.2, and 0.5 mol/L). Each elution peak represents a kind of exopolysaccharide (EPS) fraction. CEPS are purified and divided into three EPS fractions (named as EPS-1, EPS-2, and EPS-3).
Figura 2. Perfil de elución de los exopolisacáridos crudos (CEPS) de Lactobacillus kimchi SR8 en una columna de intercambio iónico de celulosa DEAE con un gradiente de solución de cloruro de sodio (0, 0.2 y 0.5 mol/L). Cada pico de elución representa un tipo de fracción de exopolisacáridos (EPS). Los CEPS se purifican y se dividen en tres fracciones de EPS (denominadas EPS-1, EPS-2 y EPS-3)
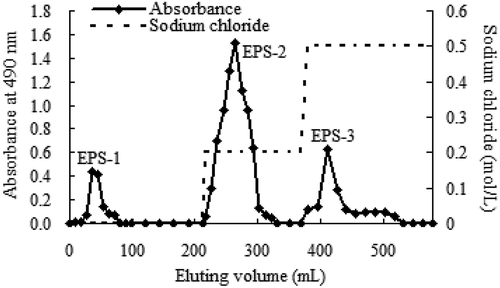
3.2. EPS antioxidant activity in vitro
3.2.1. DPPH radical scavenging activity
The DPPH radical scavenging activities of CEPS, EPS-1, EPS-2, and EPS-3 are shown in . The DPPH radical scavenging activity of CEPS increased with the concentration of polysaccharides, although the scavenging activity of CEPS was lower than that of ascorbic acid (Vc). At a concentration of 8 mg/mL, the scavenging activity of CEPS increased to 50.41%, and the IC50 value was 7.37 mg/mL. At a concentration of 81 μg/mL, scavenging activities of CEPS, EPS-1, EPS-2, and EPS-3 were 9.52%, 12.90%, 6.18%, and 7.16%, respectively, indicating that EPS-1 possessed higher scavenging activity on DPPH radicals than CEPS, EPS-2, and EPS-3 (p < .05).
Figure 3. The 1,1-diphenyl-2-picrylhydrazyl (DPPH) radical scavenging ability of exopolysaccharides (EPSs) from Lactobacillus kimchi SR8. (a) Effect of crude exopolysaccharides (CEPS) and ascorbic acid (Vc) on DPPH radical scavenging ability; (b) Comparison of DPPH radical scavenging abilities of CEPS, EPS-1, EPS-2 and EPS-3, and different lower cases (a, b, and c) showed significant difference (p < 0.05).
Figura 3. Capacidad de eliminación de radicales 1,1-difenil-2-picrilhidrazilo (DPPH) de los exopolisacáridos (EPS) de Lactobacillus kimchi SR8. (a) Efecto de los exopolisacáridos crudos (CEPS) y del ácido ascórbico (Vc) en la capacidad de eliminación del radical DPPH; (b) Comparación de las capacidades de eliminación del radical DPPH de los CEPS, EPS-1, EPS-2 y EPS-3; las distintas letras minúsculas (a, b, y c) son significativamente diferentes (p < 0.05)
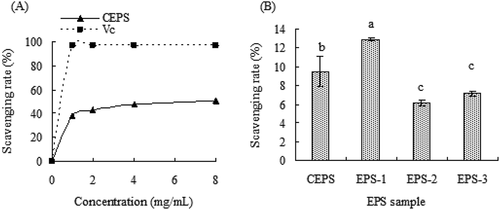
3.2.2. Hydroxyl radical scavenging activity
As shown in , both CEPS and ascorbic acid (Vc) exhibited strong scavenging activity towards hydroxyl radicals in a concentration-dependent manner. Although the hydroxyl radical scavenging activity of CEPS gradually increased in a concentration-dependent manner and the scavenging activity of Vc increased sharply at a concentration of 0 to 1 mg/mL, the scavenging activity of CEPS was lower than that of Vc. CEPS hydroxyl radical scavenging activity reached 71.39% and 96.58% at concentration of 1.0 mg/mL and 8.0 mg/mL, respectively. However, at a concentration of 81 μg/mL, scavenging activities of CEPS, EPS-1, EPS-2, and EPS-3 were 7.05%, 12.34%, 2.23%, and 38.51%, respectively, revealing that EPS-3 possessed significantly higher hydroxyl radical scavenging activity than those of CEPS, EPS-1 and EPS-2 (p < .05). Additionally, the EPS-1 fraction showed higher hydroxyl radical scavenging activity than that of the EPS-2 fraction.
Figure 4. Hydroxyl radical scavenging ability of exopolysaccharides (EPSs) from Lactobacillus kimchi SR8. (a) Effect of crude exopolysaccharides (CEPS) and ascorbic acid (Vc) on hydroxyl radical scavenging ability; (b) Comparison of hydroxyl radical scavenging abilities of CEPS, EPS-1, EPS-2 and EPS-3, and different lower cases (a, b, and c) showed significant difference (p < 0.05).
Figura 4. Capacidad de eliminación de radicales hidroxilo de los exopolisacáridos (EPS) de Lactobacillus kimchi SR8. (a) Efecto de los exopolisacáridos crudos (CEPS) y del ácido ascórbico (Vc) en la capacidad de eliminación de los radicales hidroxilos; (b) Comparación de las capacidades de eliminación de los radicales hidroxilos de los CEPS, EPS-1, EPS-2 y EPS-3; las distintas letras minúsculas (a, b, y c) son significativamente diferentes (p < 0.05)
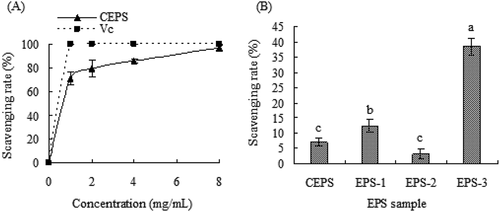
3.3. EPS antioxidant activity in vivo
3.3.1. Body weight and liver index of mice
The initial and final body weights and the liver index of the experimental mice are presented in . All of the experimental animals that received Lactobacillus kimchi SR8 extract increased in body weight, indicating EPS from the strain was safe and had no effect on the growth and health of experimental animals. However, the group receiving 100 mg/kg Lactobacillus kimchi SR8 extract displayed the highest percentage weight gain (74.51%). Liver index was calculated on the basis of the body weight and the liver weight, and increased significantly in mice from the high-dose group and the positive control compared to the normal control and model groups (p < .01). This result indicated that EPS was non-toxic and harmless to the liver. The difference in liver index between the high-dose and positive control group was not significant (p > .05).
Table 1. Effect of CEPS on body weight and liver index of mice in each treatment group.
Tabla 1. Efecto del CEPS en el peso corporal y el índice hepático de los ratones en cada grupo de tratamiento
3.3.2. Total antioxidant capacity of the serum and liver
The total antioxidant activity of the serum and liver are shown in . The total antioxidant activities of serum in the medium- and high-dose groups was significantly higher than the model group (p < .01), but showed no significant difference when compared to the positive control group (p > .05). Thus, the total antioxidant activity of high-dose group was significantly higher than that of the normal control group (p < .01). The antioxidant activity in the liver of groups receiving Lactobacillus kimchi SR8 extract was significantly higher than that of the model group (p < .01), but significantly lower than that of the positive control (p > .05).
Figure 5. Determination of total antioxidant capacity (T-AOC) of the serum and liver of mice in each treatment group. Different lower cases showed significant difference (p < 0.05), and different upper cases showed extremely significant difference (p < 0.01).
Figura 5. Determinación de la capacidad antioxidante total (T-AOC) del suero y el hígado de ratones de cada grupo de tratamiento. Las distintas letras minúsculas son significativamente diferentes (p < 0.05), y las distintas letras mayúsculas representan diferencias sumamente significativas (p < 0.01)
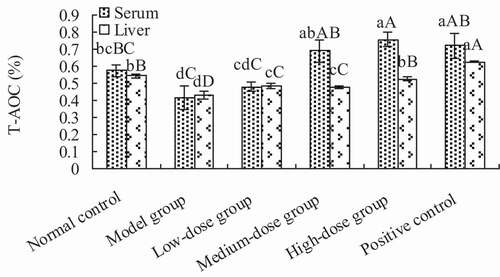
3.3.3. Levels of CAT, GSH-Px, and SOD in serum
The results obtained from the measurement of serum levels of CAT, GSH-Px, and SOD are presented in . There was a significant increase in the levels of CAT, GSH-Px, and SOD in all the groups receiving the extract when compared with the model group (p < .05), with the highest increase observed in the high-dose group (200 mg/kg). Compared with the normal control, there was a significant increase in the level of CAT (p < .01) and a significant increase in the level of GSH-Px (p < .05). Under the same dose, the levels of CAT, GSH-Px, and SOD of the positive group were significantly higher than those of the medium-dose group (p < .01). However, there was no significant difference in the levels of CAT, GSH-Px, and SOD between the low and medium-dose groups (p > .05), but there was a slight, yet not significant increase in the level of CAT in the medium-dose group when compared to the normal group.
Table 2. Levels of CAT, GSH-Px, and SOD in the serum of mice in each treatment group.
Tabla 2. Niveles de CAT, GSH-Px y SOD en el suero de los ratones de cada grupo de tratamiento
3.3.4. Levels of CAT, GSH-Px, and SOD in liver tissue
The activity level of the antioxidant enzymes in the different groups is shown in . The activities of CAT, GSH-Px, and SOD of groups receiving the extract and ascorbic acid significantly increased when compared to the model group (p < .01). There was no significant increase in the level of CAT in the liver of the high-dose group (200 mg/kg) when compared to the normal control group (p > .05). The activity of GSH-Px of the high-dose group was significantly higher than that of the normal control (p < .05), and there was a significant increase in the level of SOD in the liver of groups receiving the extract as compared to the normal control (p < .05), especially that of the high-dose group when compared to the normal control (p < .01). Furthermore, the activity of SOD in the high-dose group was slightly higher than that of the positive group, although the difference was not significant.
Table 3. Levels of CAT, GSH-Px, and SOD in the liver of mice in each treatment group.
Tabla 3. Niveles de CAT, GSH-Px y SOD en el hígado de los ratones de cada grupo de tratamiento
3.3.5. Levels of MDA in the serum and liver
The levels of MDA in the serum and liver in groups receiving the extract are shown in . In the serum, the levels of MDA in the medium- and high-dose groups were significantly higher than that of the model group (p < .01). The level of MDA in the high-dose group was slightly lower than that of the normal control group, although the difference was not significant. The level of MDA in the positive control group was lower than that of the high-dose group. In the liver, the level of MDA in the high-dose group was significantly lower than the model group (p < .01). The levels of MDA in the low-dose, medium-dose, and positive control groups were statistically similar. The level of MDA in the high-dose group was lower than that of the normal control group, and significantly lower than that of the positive control group (p < .05).
Table 4. Level of MDA in the serum and liver of mice in each treatment group.
Tabla 4. Nivel de MDA en el suero y el hígado de los ratones de cada grupo de tratamiento
4. Discussion
The EPS produced by lactic acid bacteria could exhibit bioactivity (antioxidant activity, antitumor activity, and cholesterol-lowering activity, as well as immunomodulatory properties, etc.) and functional properties (texture, rheology, and flocculating activity, etc.) (Kukihi & Afiati, Citation2016; Mende et al., Citation2016; Rajoka et al., Citation2018; Seesuriyachan et al., Citation2011; Wang et al., Citation2017). Many researchers pay much attention to the application of these EPS for improving the quality and function of products in food industry, such as dextran, levan, and kefiran (Duboc & Mollet, Citation2001; Mende et al., Citation2016; Moradi et al., Citation2020; Zannini et al., Citation2016). Previous studies have reported that EPS production is mainly influenced by the strain of lactic acid bacteria, components in the medium, and the culture conditions (Seesuriyachan et al., Citation2014; Wang et al., Citation2017), and that EPS extract production is influenced by extracting conditions, such as the concentration of TCA, ethanol volume, and duration of ethanol-precipitation (Liao et al., Citation2017). Imran et al. (Citation2016) reported that the EPS production by Lactobacillus plantarum NTMI05 and NTMI20 increased from 197 mg/L to 956 mg/L and from 187 mg/L to 827 mg/L under optimum conditions, respectively. Wang et al. (Citation2017) also reported that the production of EPS from Lactobacillus plantarum KX041 increased from the original production of less than 200 mg/L to the optimized production of 599.52 mg/L. In this study, the maximum amount of EPS produced by Lactobacillus Kimchi SR8 was up to 627.50 mg/L, and was higher than the value reported by Wang et al. (Citation2017). For EPS produced by Lactobacillus Kimchi SR8, three fractions were purified from ion-exchange chromatography, including a neutral polysaccharide (EPS-1) and two acidic polysaccharides (EPS-2 and EPS-3). Interestingly, X Zhang et al. (Citation2012) reported that EPS produced by Streptococcus thermophilus CH9 also contained a neutral polysaccharide and two acidic polysaccharides. Both EPS-1 and EPS-3 showed strong scavenging activities on DPPH radical and hydroxyl radical. The EPS produced by Weissella cibaria SJ14 were reported to contain three acidic polysaccharides (EPS1, EPS2, and EPS3), among which both EPS1 and EPS3 exhibited reducing strength and strong scavenging activities of DPPH, hydroxyl, and superoxide radicals (Zhu et al., Citation2018). A previous study reported that EPS of the royal sun medicinal mushroom (Agaricus brasiliensis) exhibited scavenging effects on DPPH, hydroxyl radicals and reducing power, and one of the EPS fractions showed higher DPPH and hydroxyl radical scavenging activity (32.96%, 96.26%) at a dose of 200 mg/L EPS when compared to those of CEPS (J Zhang et al., Citation2016). R Xu et al. (Citation2011) reported the effects of EPS fractions from Bifidobacterium animalis RH on the activities of anti-lipid peroxidation, DPPH, hydroxyl, and superoxide anion radicals, and found high antioxidant activity. EPSs from yoghurt starter (Lactobacillus delbrueckii subsp. bulgaricus and Streptococcus salivarius subsp. thermophilus) exhibited DPPH radical scavenging activity (24.25%) at 100 μg/mL, which was lower than that of Vc (67.79%) at the same concentration (Ghalem, Citation2017). Differences in the antioxidant activity of EPS may be due to the source, composition, and structure of the EPS, among other factors.
Excessive D-galactose affects normal osmotic pressure in the cell and causes cell swelling and dysfunction, leading to metabolic disorder and aging-related disorders (JJ Cui et al., Citation2010). During the metabolic process, metabolic disorder causes D-galactose to induce excessive free radicals and the initiation of lipid peroxide, damaging the antioxidant defenses of the body (X Cui et al., Citation2006). In recent years, increasing studies have suggested that aging was mainly attributed to the cumulative damage from the generation of reactive oxygen species (Adesulu-Dahunsi et al., Citation2018; Jing et al., Citation2016). In this study, the aging model group showed a significant decrease in the total antioxidant capacity and activities of CAT and GSH-Px in both the serum and liver, a significant decrease in the activity of SOD in the serum, and a significant increase in the level of MDA in both the serum and liver when compared with a normal control. These results indicated a successful aging mice model. The liver index of the high-dose group of mice receiving EPS from Lactobacillus kimchi SR8 was significantly higher than that of the model group, indicating an inhibiting effect on liver atrophy. Additionally, the results obtained from our animal experiment also showed a dose effect of EPS on antioxidant activity. In this study, there was a significant increase in the total antioxidant capacity in the serum at a medium dose (100 mg/kg) and in the liver at a low dose (50 mg/kg). The total antioxidant capacity represents the ability of the non-enzymatic defense to deal with reactive oxygen species (Chen & Kan, Citation2018). When the damage caused by excessive free radicals went beyond repair capacity of body, body aging occurred. Excessive free radicals induce lipid peroxidation in the body to produce MDA, and MDA content may indirectly reflect the content of free radicals in the body. Some antioxidant enzymes, such as CAT, GSH-Px, and SOD are critical for removing excessive free radicals to maintain physiological equilibrium and prevent free radicals damaging the body (Luo et al., Citation2012). These antioxidants play a crucial role in protecting against oxidation and the damage from free radicals (Woo et al., Citation2014). The EPS produced by Lactobacillus kimchi SR8 and ascorbic acid increased the activities of CAT, GSH-Px, and SOD and decreased the levels of MDA in both the serum and liver, compared to the model group. The reduction in the levels of MDA in both the serum and liver suggested that the intake of EPS produced by Lactobacillus kimchi SR8 could oppose the lipid peroxidation in aging mice.
5. Conclusions
The antioxidant activities of CEPS and three EPS fractions (EPS-1, EPS-2, and EPS-3) from Lactobacillus kimchi SR8 were carried out using a variety of in vitro methods and by a D-galactose-induced aging mouse model in vivo. In in vitro antioxidant testing, CEPS, EPS-1, EPS-2, and EPS-3 exhibited potential free radical scavenging activity. Additionally, EPS-1, CEPS, and EPS-3 showed stronger antioxidant activities. In vivo antioxidant assays of the serum and liver indicated that EPS from Lactobacillus kimchi SR8 possessed excellent anti-aging ability. In general, Lactobacillus kimchi SR8-derived EPS may provide evidence for the use of lactic acid bacteria-derived EPS as natural antioxidants to replace synthetically derived supplements.
Disclosure of interest
No conflict of interest was reported by the authors.
Author contributions
The information of the Author Contribution is shown as below: Yulong Zhang: Conceptualization, 25% Investigation, 50% Writing – Original Draft, 50% Writing – Review & Editing, and 50% Formal analysis. Xueying Chen: Conceptualization, 25% Investigation, 50% Writing – Original Draft, 50% Writing – Review & Editing, and 50% Formal analysis. Ping Hu: Conceptualization, Methodology, Supervision, Project administration, and Funding acquisition. Qianwei Liao: 25% Investigation, and 100% Validation. Yong Luo: 25% Investigation. Juan Li: Data Curation. Dandan Feng: Data Curation. Jun Zhang: Data Curation. Zhaoqing Wu: Data Curation. Haoxiang Xu: Data Curation. Finally, all authors agreed to submit the manuscript to the current journal.
Additional information
Funding
References
- Adesulu-Dahunsi, A. T., Sanni, A. I., & Jeyaram, K. (2018). Production, characterization and in vitro antioxidant activities of exopolysaccharide from Weissella cibaria GA44. LWT-Food Science and Technology, 87, 432–442. https://doi.org/https://doi.org/10.1016/j.lwt.2017.09.013
- Ahmed, Z., Wang, Y. P., Anjum, N., Ahmadm, A., & Khan, S. T. (2013). Characterization of exopolysaccharide produced by Lactobacillus kefiranofaciens ZW3 isolated from Tibet kefir-part II. Food Hydrocolloid, 30(1), 343–350. https://doi.org/https://doi.org/10.1016/j.foodhyd.2012.06.009
- Balzaretti, S., Taverniti, V., Guglielmetti, S., Fiore, W., Minuzzo, M., Ngo, H. N., Ngere, J. B., Sadiq, S., Humphreys, P. N., & Laws, A. P. (2017). A novel rhamnose-rich hetero-exopolysaccharide isolated from Lactobacillus paracasei DG activates THP-1 human monocytic cells. Applied and Environmental Microbiology, 83(3), 1–15. https://doi.org/https://doi.org/10.1128/AEM.02702-16
- Bradford, M. M. (1976). A rapid and sensitive method for the quantitation of microgram quantities of protein utilizing the principle of protein-dye binding. Analytical Biochemistry, 72(1–2), 248–254. https://doi.org/https://doi.org/10.1016/0003-2697(76)90527-3
- Cheirsilp, B., Suksawang, S., Yeesang, J., & Boonsawang, P. (2018). Co-production of functional exopolysaccharides and lactic acid by Lactobacillus kefiranofaciens originated from fermented milk, kefir. Journal of Food Science and Technology, 55(1), 331–340. https://doi.org/https://doi.org/10.1007/s13197-017-2943-7
- Chen, G., & Kan, J. (2018). Ultrasound-assisted extraction, characterization, and antioxidant activity in vitro and in vivo of polysaccharides from Chestnut rose (Rosa roxburghii tratt) fruit. Journal of Food Science and Technology, 55(3), 1083–1092. https://doi.org/https://doi.org/10.1007/s13197-017-3023-8
- Cui, J. J., Yuan, J. F., & Zhang, Z. Q. (2010). Anti-oxidation activity of the crude polysaccharides isolated from Polygonum cillinerve (Nakai) Ohwi in immunosuppressed mice. Journal of Ethnopharmacology, 132(2), 512–517. https://doi.org/https://doi.org/10.1016/j.jep.2010.08.052
- Cui, X., Zuo, P., Zhang, Q., Li, X., Hu, Y., Long, J., & Liu, J. (2006). Chronic systemic D-galactose exposure induces memory loss, neurodegeneration and oxidative damage in mice: Protective effects of R-alpha-lipoic acid. Journal of Neuroscience Research, 84(3), 647–654. https://doi.org/https://doi.org/10.1002/jnr.20899
- Domingos-Lopes, M. F. P., Nagy, A., Stanton, C., Ross, P. R., Gelencser, E., & Silva, C. C. G. (2017). Immunomodulatory activity of exopolysaccharide producing Leuconostoc citreum strain isolated from Pico cheese. Journal of Functional Foods, 33, 235–243. https://doi.org/https://doi.org/10.1016/j.jff.2017.03.054
- Du, R., Qiao, X., Zhao, F., Song, Q., Zhou, Q., Wang, Y., Pan, L., Ye, H., & Zhou, Z. (2018). Purification, characterization and antioxidant activity of dextran produced by Leuconostoc pseudomesenteroides from homemade wine. Carbohydrate Polymers, 198, 529–536. https://doi.org/https://doi.org/10.1016/j.carbpol.2018.06.116
- Duboc, P., & Mollet, B. (2001). Applications of exopolysaccharides in dairy industry. International Dairy Journal, 11(9), 759–768. https://doi.org/https://doi.org/10.1016/S0958-6946(01)00119-4
- El-Deeb, N. M., Yassin, A. M., Al-Madboly, L. A., & El-Hawiet, A. (2018). A novel purified Lactobacillus acidophilus 20079 exopolysaccharide, LA-EPS-20079, molecularly regulates both apoptotic and NF-kappa B inflammatory pathways in human colon cancer. Microbial Cell Factories, 17(1), 1–15. https://doi.org/https://doi.org/10.1186/s12934-018-0877-z
- Gentes, M. C., Turgeon, S. L., & St-Gelais, D. (2016). Impact of starch and exopolysaccharide-producing lactic acid bacteria on the properties of set and stirred yoghurts. International Dairy Journal, 55, 79–86. https://doi.org/https://doi.org/10.1016/j.idairyj.2015.12.006
- Ghalem, R. B. (2017). Antioxidant and antimicrobial activities of exopolysaccharides from yoghurt starter. Advances in Biochemistry, 5(5), 97–101. https://doi.org/https://doi.org/10.11648/j.ab.20170505.13
- Han, X., Yang, Z., Jing, X., Yu, P., Zhang, Y., Yi, H., & Zhang, L. (2016). Improvement of the texture of yogurt by use of exopolysaccharide producing lactic acid bacteria. BioMed Research International, 2016, 1–6. https://doi.org/https://doi.org/10.1155/2016/7945675
- Hong, S. P., Shin, S. C., Kang, W. K., Chin, Y. W., Turner, T. L., Choi, H. W., song, K. M., & Kim, H. J. (2017). Complete genome sequence of Leuconostoc garlicum KCCM 43211 producing exopolysaccharide. Journal of Biotechnology, 246, 40–44. https://doi.org/https://doi.org/10.1016/j.jbiotec.2017.02.018
- Imran, M. Y. M., Reehana, N., Jayaraj, K. A., Ahamed, A. A. P., Dhanasekaran, D., Thajuddin, N., Alharbi, N. S., & Muralitharan, G. (2016). Statistical optimization of exopolysaccharide production by Lactobacillus plantarum NTMI05 and NTMI20. International Journal of Biological Macromolecules, 93, 731–745. https://doi.org/https://doi.org/10.1016/j.ijbiomac.2016.09.007
- Jeong, D., Kim, D. H., Kang, I. B., Kim, H., Song, K. Y., Kim, H. S., & Seo, K. H. (2017). Characterization and antibacterial activity of a novel exopolysaccharide produced by Lactobacillus kefiranofaciens DN1 isolated from kefir. Food Control, 78, 436–442. https://doi.org/https://doi.org/10.1016/j.foodcont.2017.02.033
- Jing, Y., Gao, Y., Wang, W., Cheng, Y., Lu, P., Ma, C., & Zhang, Y. (2016). Optimization of the extraction of polysaccharides from tobacco waste and their biological activities. International Journal of Biological Macromolecules, 91, 188–197. https://doi.org/https://doi.org/10.1016/j.ijbiomac.2016.05.069
- Kim, K., Lee, G., Thanh, H. D., Kim, J. H., Konkit, M., Yoon, S., Park, M., Yang, S., Park, E., & Kim, W. (2018). Exopolysaccharide from Lactobacillus plantarum LRCC5310 offers protection against rotavirus-induced diarrhea and regulates inflammatory response. Journal of Dairy Science, 101(7), 5702–5712. https://doi.org/https://doi.org/10.3168/jds.2017-14151
- Kukihi, F. E., & Afiati, F. (2016). Exopolysaccharide (EPS) activity test of lactic acid bacteria (LAB) as immunomodulatory. Jurnal Ilmu Ternak Dan Veteriner, 21(3), 182–189. https://doi.org/https://doi.org/10.14334/jitv.v21i3.1414
- Li, W., Ji, J., Chen, X., Jiang, M., Rui, X., & Dong, M. (2014). Structural elucidation and antioxidant activities of exopolysaccharides from Lactobacillus helveticus MB2-1. Carbohydrate Polymers, 102, 351–359. https://doi.org/https://doi.org/10.1016/j.carbpol.2013.11.053
- Liao, Q., Hu, P., Zhang, Y., Xie, H., Zhu, Q., & Wang, X. (2017). Optimization of fermentation and extraction process of exopolysaccharides of lactic acid bacteria SR2-2 isolated from sour meat of the Dong Minority. China Brewing, 36(5), 118–122. http://www.cnki.com.cn/Article/CJFDTOTAL-ZNGZ201705028.htm
- Liu, L. B., Sun, D., Gu, C. T., Zhao, F., Xu, J., & Zhou, Y. L. (2013). Screening of lactic acid bacteria strains with high production-exopolysaccharide and their application in Cottage Cheese. Science and Technology of Food Industry, 34(11), 132–136. http://www.en.cnki.com.cn/Article_en/CJFDTotal-SPKJ201311024.htm
- Lu, H. Q., Huo, W. M., Huang, L., Gu, X. X., Li, C., Li, J. C., & Tian, H. T. (2017). Screening and application in Chinese sauerkraut fermentation of lactic acid bacteria of probiotic function. Journal of Food Science and Technology, (7), 2–7. http://en.cnki.com.cn/Article_en/CJFDTotal-SSPJ201707002.htm
- Luo, A., Luo, A., Huang, J., & Fan, Y. (2012). Purification, characterization and antioxidant activities in vitro and in vivo of the polysaccharides from Boletus edulis Bull. Molecules, 17(7), 8079–8090. https://doi.org/https://doi.org/10.3390/molecules17078079
- Lynch, K. M., Coffey, A., & Arendt, E. K. (2018). Exopolysaccharide producing lactic acid bacteria: Their techno-functional role and potential application in gluten-free bread products. Food Research International, 110, 52–61. https://doi.org/https://doi.org/10.1016/j.foodres.2017.03.012
- Matsuzaki, C., Takagaki, C., Tomabechi, Y., Forsberg, L. S., Heiss, C., Azadi, P., Matsumoto, K., Katoh, T., Hosomi, K., & Kunisawa, J. (2017). Structural characterization of the immunostimulatory exopolysaccharide produced by Leuconostoc mesenteroides strain NTM048. Carbohydrate Research, 448, 95–102. https://doi.org/https://doi.org/10.1016/j.carres.2017.06.004
- Mende, S., Rohm, H., & Jaros, D. (2016). Influence of exopolysaccharides on the structure, texture, stability and sensory properties of yoghurt and related products. International Dairy Journal, 52, 57–71. https://doi.org/https://doi.org/10.1016/j.idairyj.2015.08.002
- Moradi, M., Guimarāes, J., & Sahin, S. (2020). Current application of exopolysaccharides from lactic acid bacteria in the development of food active edible packaging. Current Opinion in Food Science, 40, 33–39. https://doi.org/https://doi.org/10.1016/j.cofs.2020.06.001
- Nadkarni, M. A., Chen, Z. L., Wilkins, M. R., & Hunter, N. (2014). Comparative genome analysis of Lactobacillus rhamnosus clinical isolates from initial stages of dental pulp infection: Identification of a new exopolysaccharide cluster. PLoS ONE, 9(3), 1–14. https://doi.org/https://doi.org/10.1371/journal.pone.0090643
- Ng, I. S., & Xue, C. (2017). Enhanced exopolysaccharide production and biological activity of Lactobacillus rhamnosus ZY with calcium and hydrogen peroxide. Process Biochemistry, 52, 295–304. https://doi.org/https://doi.org/10.1016/j.procbio.2016.10.006
- Pachekrepapol, U., Lucey, J. A., Gong, Y., Naran, R., & Azadi, P. (2017). Characterization of the chemical structures and physical properties of exopolysaccharides produced by various Streptococcus thermophilus strains. Journal of Dairy Science, 100(5), 3424–3435. https://doi.org/https://doi.org/10.3168/jds.2016-12125
- Rajoka, M. S. R., Jin, M., Zhao, H., Li, Q., Shao, D., Jiang, C., Huang, Q., Yang, H., Shi, J., & Hussain, N. (2018). Functional characterization and biotechnological potential of exopolysaccharide produced by Lactobacillus rhamnosus strains isolated from human breast milk. LWT-Food Science and Technology, 89, 638–647. https://doi.org/https://doi.org/10.1016/j.lwt.2017.11.034
- Salazar, N., Gueimonde, M., de Los Reyes-gavilan, C. G., & Ruas-Madiedo, P. (2016). Exopolysaccharides produced by lactic acid bacteria and bifidobacteria as fermentable substrates by the intestinal microbiota. Critical Reviews in Food Science, 56(9), 1440–1453. https://doi.org/https://doi.org/10.1080/10408398.2013.770728
- Saravanan, C., & Shetty, P. K. H. (2016). Isolation and characterization of exopolysaccharide from Leuconostoc lactis KC117496 isolated from idli batter. International Journal of Biological Macromolecules, 90, 100–106. https://doi.org/https://doi.org/10.1016/j.ijbiomac.2015.02.007
- Sasikumar, K., Vaikkath, D. K., Devendra, L., & Nampoothiri, K. M. (2017). An exopolysaccharide (EPS) from a Lactobacillus plantarum BR2 with potential benefits for making functional foods. Bioresource Technology, 241, 1152–1156. https://doi.org/https://doi.org/10.1016/j.biortech.2017.05.075
- Seesuriyachan, P., Kuntiya, A., Chaiyaso, T., Hanmoungjai, P., Leksawasdi, N., & Techapun, C. (2014). Enhancement and optimization of exopolysaccharide production by Weissella confusa TISTR 1498 in pH controlled submerged fermentation under high salinity stress. Chiang Mai Journal of Science, 41(3), 503–512. http://epg.science.cmu.ac.th/ejournal/
- Seesuriyachan, P., Kuntiya, A., Hanmoungjai, P., & Techapun, C. (2011). Exopolysaccharide production by Lactobacillus confuses TISTR 1498 using coconut water as an alternative carbon source: The effect of peptone, yeast extract and beef extract. Songklanakarin Journal of Science & Technology, 33(4), 379–387. http://rdo.psu.ac.th/sjstweb/journal/33-4/0125-3395-33-4-379-387.pdf
- Shin, J. S., Jung, J. Y., Lee, S. G., Shin, K. S., Rhee, Y. K., Lee, M. K., … Lee, K. T. (2016). Exopolysaccharide fraction from Pediococcus pentosaceus KFT18 induces immunostimulatory activity in macrophages and immunosuppressed mice. Journal of Applied Microbiology, 120(5), 1390–1402. https://doi.org/https://doi.org/10.1111/jam.13099
- Sun, L. L., Gao, W., Zhang, M. M., Li, C., Wang, A. G., Su, Y. L., & Ji, T. F. (2014). Composition and antioxidant activity of the anthocyanins of the fruit of Berberis heteropoda Schrenk. Molecules, 19(11), 19078–19096. https://doi.org/https://doi.org/10.3390/molecules191119078
- Wang, X., Shao, C., Liu, L., Guo, X., Xu, Y., & Lv, X. (2017). Optimization, partial characterization and antioxidant activity of an exopolysaccharide from Lactobacillus plantarum KX041. International Journal of Biological Macromolecules, 103, 1173–1184. https://doi.org/https://doi.org/10.1016/j.ijbiomac.2017.05.118
- Woo, J. Y., Gu, W., Kim, K. A., Jang, S. E., Han, M. J., & Kim, D. H. (2014). Lactobacillus pentosus var. plantarum C29 ameliorates memory impairment and inflammaging in a D-galactose-induced accelerated aging mouse model. Anaerobe, 27, 22–26. https://doi.org/https://doi.org/10.1016/j.anaerobe.2014.03.003
- Xu, R., Shang, N., & Li, P. (2011). In vitro and in vivo antioxidant activity of exopolysaccharide fractions from Bifidobacterium animalis RH. Anaerobe, 17(5), 226–231. https://doi.org/https://doi.org/10.1016/j.anaerobe.2011.07.010
- Xu, Z., Guo, Q., Zhang, H., Wu, Y., Hang, X., & Ai, L. (2018). Exopolysaccharide produced by Streptococcus thermophiles S-3: Molecule, partial structural and rheological properties. Carbohydrate Polymers, 194, 132–138. https://doi.org/https://doi.org/10.1016/j.carbpol.2018.04.014
- Yan, S., Zhao, G., Liu, X., Zhao, J., Zhang, H., & Chen, W. (2017). Production of exopolysaccharide by Bifidobacterium longum isolated from elderly and infant feces and analysis of priming glycosyltransferase genes. RSC Advances, 7(50), 31736–31744. https://doi.org/https://doi.org/10.1039/C7RA03925E
- Yang, Y., Feng, F., Zhou, Q., Zhao, F., Du, R., Zhou, Z., & Han, Y. (2018). Isolation, purification and characterization of exopolysaccharide produced by Leuconostoc pseudomesenteroides YF32 from soybean paste. International Journal of Biological Macromolecules, 114, 529–535. https://doi.org/https://doi.org/10.1016/j.ijbiomac.2018.03.162
- Zannini, E. 2015. Functional application of lactic acid bacteria exopolysaccharide in complex food systems. Ph.D. Thesis, University of College Cork.
- Zannini, E., Waters, D. M., Coffey, A., & Arendt, E. K. (2016). Production, properties, and industrial food application of lactic acid bacteria-derived exopolysaccharides. Applied Microbiology and Biotechnology, 100(3), 1121–1135. https://doi.org/https://doi.org/10.1007/s00253-015-7172-2
- Zhan, J., Chen, Y., Huang, L., Che, P., & Hu, P. (2013). Isolation and identification of lactic acid bacteria from sour meat and sour fish of minor nationalities in Guizhou Province. Meat Research, 27(7), 40–43. http://www.docin.com/p-1490413763.html
- Zhang, J., Gao, Z., Li, S., Gao, S., Ren, Z., Jing, H., & Jia, L. (2016). Purification, characterization, antioxidation, and antiaging properties of exopolysaccharides and endopolysaccharides of the royal sun medicinal mushroom, Agaricus brasiliensis (Agaricomycetes). International Journal of Medicinal Mushrooms, 18(12), 1071–1081. https://doi.org/https://doi.org/10.1615/IntJMedMushrooms.v18.i12.20
- Zhang, X., Liu, H., Cao, C., & Ji, L. (2012). Purification and structural characterization of exopolysaccharide produced by Streptococcus thermophilus CH9. Food Science, 33(19), 107–112. http://en.cnki.com.cn/Article_en/CJFDTotal-SPKX201219024.htm
- Zhang, Y., Hu, P., Fan, M., & Liao, Q. (2017). Study on effect elements of exopolysaccharide production of Lactobacillus Kimchi SR8 and DPPH radical scavenging activity. Journal of Food and Nutrition Research, 5(12), 928–934. http://pubs.sciepub.com/jfnr/5/12/8/jfnr-5-12-8.pdf
- Zhang, Y., Hu, P., Xie, Y., & Wang, X. (2020). Co-fermentation with Lactobacillus curvatus LAB26 and Pediococcus pentosaceus SWU73571 for improving quality and safety of sour meat. Meat Science, 170, 108240. https://doi.org/https://doi.org/10.1016/j.meatsci.2020.108240
- Zhu, Y., Wang, C., Jia, S., Wang, B., Zhou, K., Chen, S., Yang, Y., & Liu, S. (2018). Purification, characterization and antioxidant activity of the exopolysaccharide from Weissella cibaria SJ14 isolated from Sichuan paocai. International Journal of Biological Macromolecules, 115, 820–828. https://doi.org/https://doi.org/10.1016/j.ijbiomac.2018.04.067