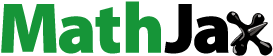
ABSTRACT
In Ethiopia, sesame is number one oilseed crop in terms of export value, but with low value addition practice. This research aimed to determine effect of varieties and pre-oil extraction treatments on yield and quality of the oil. Results showed that yield varied from 47.1 to 57.5% with iodine value (IV) from 106 to 113 g/100 g, free fatty acid (FFA) 0.47 to 1.36%, and peroxide value (PV) of 0.66 to 7.04 meq O2/kg. Palmitic, oleic and linoleic acid were the major fatty acids in the oil and characterized by high unsaturated (80–85%) and less saturated (15–20%) fatty acids. All pre-oil extraction treatments increased the oil content, with minor effects on color, FFA, and PV as compared to the oil from the raw seed. However, roasting enabled to extract better oil yield with low PV and less constitutes of FFA.
1. Introduction
Africa (59.4%) and Asia (37.5%) produce the majority of the world’s sesame seeds, accounting for about 97% of the total production (FAOSTAT, Citation2020). Sesame is one of the major oilseed crops in Ethiopia representing more than 30% of the total oilseed production (Central StatisticsAgency [CSA], Citation2018) and it is one of the top oil crops to generate foreign currency, next to coffee. The crop has multifunctional uses including paste (tahini), halaweh or halva, salads, cooking oil, and bakery (Abu-Jdayil et al., Citation2002; Elleuch et al., Citation2007). As an ingredient, it is mainly used for many processed food products due to its good flavor and attractive color. It is used as a condiment oil along with different fragrances, for salad, soup, forcemeat, and refreshments (Ji et al., Citation2019) as well as medicinal and cosmetic values (Anilakumar et al., Citation2010; Pathak et al., Citation2014). In Ethiopia sesame is traditionally used as an ingredient for stew preparation by mixing the roasted seed flour with water, salt, onion, and chili pepper, which is eaten with baked products. Sesame whole seed is also used as an ingredient in bakeries.
The chemical composition and nutritive values of sesame oil have been reported in different previous studies from elsewhere (Borchani et al., Citation2010; Gharby et al., Citation2017; Wan et al., Citation2015). Sesame oil contains appreciable amounts of lipids/oil (45–60%), protein (18–25%), fiber (3.5–7%), ash (3–5), carbohydrate (13.5%), and high levels of many important micronutrients. Its oil is rich in mono and polyunsaturated fatty acid constituting ~85%, with beneficial human health effects.
Different processing methods such as roasting, cooking, and dehulling are among the commonly employed practices in the traditional processing of sesame seeds to improve the oil extractability and organoleptic characteristics of the oil (Elleuch et al., Citation2007; Ji et al., Citation2019; Tenyang et al., Citation2017). However, most of the studies generally focus on roasting treatment with limited information on cooking and dehulling. The physicochemical properties of sesame oil may not only be affected by prior seed processing treatment but also could vary among different sesame seed varieties. There is limited information about the combined effect of variety and seed processing treatments on the quality characteristics of sesame oil of Ethiopian varieties. Therefore, this study aimed at investigating the impact of different seed processing treatments on the physicochemical properties of Ethiopian sesame oil extracted from five different varieties.
2. Materials and methods
2.1. Sample collection area and description of varieties
Three sesame varieties (Abasena, Humera-1, and Setit-1) and two local cultivars (Gojam Azene and Wollega type) were collected from the major sesame producing areas (). Varieties of Humera-1 and Setit-1 were obtained from the Humera Agricultural Research Center of Tigray Regional State. Abasena and Gojam azene were collected from Gondar Agricultural Research Center of Amhara Regional State. Wollega (local cultivar) which is grown and well known by local farmers was collected from farmers in east Wollega Zones of Oromia Regional State. All varieties harvested in the same production season are handled and stored in the same condition before pre-oil extraction processing treatments. The detailed description of the three sesame varieties and two cultivars used in the experiment is mentioned in .
Table 1. Description of sesame varieties used in the experiment.
2.2. Experimental design
A factorial (5 × 4) design with three replications was employed to determine the effects of variety (5 levels: Abasena, Gojam Azene, Humera-1, Setit-1, and Wollega) and pre-oil extraction treatments (4 levels: roasting, cooking, dehulling, and raw/untreated (control)).
2.3. Seed processing treatments
Before processing, the seeds were manually cleaned. Cleaned seed samples were subjected to roasting, cooking, and dehulling treatments as shown in .
2.3.1. Roasting
About 250 g of sesame seed samples were spread uniformly on aluminum plate sheets and roasted in a convective air oven (Thermo scientific, UT 6760, Germany) at 160 ± 2ºC for 25 min based upon the optimized conditions of previous research works (Hwang, Citation2005; Ji et al., Citation2019; Tenyang et al., Citation2017). The roasted samples were then cooled to ambient temperature before the commencement of oil extraction.
2.3.2. Cooking
Raw sesame seed samples (250 g) were cooked in boiling water (1:10 w/v sample: water ratio) for 30 min (Makinde & Akinoso, Citation2013) with some modifications. Boiled samples were then drained and dried in hot air convective oven at 45°C for 12 h and cooled to room temperature before oil extraction.
2.3.3. Dehulling
Whole sesame seed samples were soaked in water (1:5 w/v) for 4 h at room temperature to rupture the seed coat (Makinde & Akinoso, Citation2013) with some modification. The ruptured seed coat was dehulled manually and then the seeds were dried in a hot air convective oven at 45°C for 12 h. Finally, the samples were cooled to ambient temperature before extraction.
2.4. Oil extraction and purification
Pre-treated and dried sesame seeds were subjected to a screw press machine (IBG Monfort, CA59 G Oetoec, Germany) as shown in to extract the oil. The extracted oil samples were kept at 4°C for 48 h to remove settled and suspended particles. Next, the upper layer oil free from suspended particles was stored inside a refrigerator set at 4°C until it was used for laboratory analysis. The meal was milled using a coffee grinder machine (Sayona, SZJ-830, Deluxe coffee and spice grinder, China) and the powder was packed in polypropylene bags and stored inside a refrigerator at 4°C until the analysis.
2.5. Determination of the physicochemical properties of oil
2.5.1. Oil content
Oil content of sesame seeds was determined using Soxhlet apparatus using hexane as extraction solvent, following AOAC (Citation1990) method 963.15.
2.5.2. Refractive index
The refractive index of oil samples was determined at 20°C by using a digital refractometer (Model: Kruss – Optronic, Germany) following the Official Method of AOCS method cc 7–25 (AOCS, Citation2017).
2.5.3. Color
Color of sesame oil was determined using Lovibond Tintometer (Lovibond PFX 995/5 V10.8 Tintometer, UK) following the official method of AOCS method cc 13e-92 (AOCS, Citation2017). The height of the column tube was 133.35 mm (5.25 inches). The oil sample was filled into the tube to the desired level. The tube containing the test portion was placed in the colorimeter and the color reading was taken ten times per sample to verify the consistency of the result. The reading displays of the results were in terms of RYBN (red, yellow, blue, and neutral). The value for red and yellow may range from 0 to 70, for blue 0 to 40, and for neutral 0 to 3.9.
2.5.4. Free fatty acid
The free fatty acid (FFA) content of the sesame oil sample was determined as per the AOCS methods of Ca 5a-40 (AOCS, Citation2017). The oil sample was taken out from the refrigerator and kept at room temperature until clear from turbidity. Oil samples (3 g) were weighed into a 250 mL conical flask. Then, 50 mL neutral ethanol and 1 mL phenolphthalein indicator were added to the oil sample and finally titrated against 0.1N NaOH solution until the color changed to a faint pink color. FFA values in sesame oil were calculated based on oleic acid (factor = 28.2) and expressed as a percentage of oleic acid as indicated in EquationEquation (1)(1)
(1) .
2.5.5. Peroxide value
Peroxide value (PV) was determined using the recommended procedures of the AOCS method cd 8b-90 (AOCS, Citation2017). About 5 g of oil sample was weighed in a 250 mL conical flask. Next, 25 mL of 3:2 acetic acid/chloroform solution was added to the sample and swirled until the oil was dissolved. Then, 1 mL of a freshly prepared saturated potassium iodide (KI) solution was added to the solution and the solution was allowed to stand for 1 min in a dark place with occasional shaking. Then, 30 mL of distilled water and 2–3 drops of 1% starch solution was added to the sample solution. Finally, the solution was titrated with 0.01 M of sodium thiosulfate (Na2S2NO3). The peroxide value (PV) of the samples was calculated by using Eq. 2 and expressed as meq O2/kg sample, which is also equal to mmol active O2/2 kg sample.
where S is the volume (ml) of sodium thiosulfate required to titrate the sample, B is the volume (ml) of sodium thiosulfate required for the blank, N is the calculated normality of the standardized sodium thiosulfate solution, and W is the weight of the sample (g).
2.5.6. Iodine value
The Iodine value (IV) of the oil samples was determined using the official methods of AOCS method cd 1c-85 (AOCS, Citation2017).
2.5.7. Fatty acid composition
The fatty acid (FA) profile of the sesame oil sample was determined by using an official AOCS method ce 1a-13 (AOCS, Citation2017). Methyl esterification was performed by adding sesame oil (2 mL) into the solution of methanol (100 mL) and sulfuric acid (10 mL). The mixture was then heated for 2 h using a heating mantle attached with a condenser and transferred into a separating funnel, with the addition of 100 mL n-hexane. After shaking for a minute, the solution was allowed to stand until a clear layer was formed. Then, the upper layer (hexane fraction) residual n-hexane was removed using a rotary evaporator (BUCHI Rotavapor R-200, UK). Then, a sample (1 µL) of its methyl esters was injected into gas chromatography (GC, Agilent 7890B, USA) equipped with FID detector to analyze the FA compositions. GC was operated with injection and detection temperature of 250°C and the oven temperature was 180°C. The carrier gas was hydrogen with column head pressure of 170 kpa (25 psi), flow rate 1. 0 mL/min, linear velocity 40 cm/sec, and split ratio 100:1. The obtained results were compared to standard fatty acid methyl esters based upon their retention time and expressed as the relative percentage of present FA in the sample.
2.6. Statistical analysis
All measurements were conducted in triplicate and analyses of the data were performed using SAS (SAS Version 9.4) computer software program. Mean comparison for significant (p < .05) results was performed using Tukey’s means comparison test. Relevant graphs were made using Microsoft excel.
3. Results and discussion
3.1. Oil content
The oil contents of sesame seed ranged from 47.1 to 57.5% with significant variation among varieties and pre-oil extraction treatments (). The results showed that in general, processing treatments increased the yield of oil as compared to control (raw or unprocessed) samples. The highest value of oil yield was from the dehulled samples for all varieties followed by roasted samples as compared to the rest of the processing treatments. This observation is in agreement with a previous study in which dehulling of the sesame seed was reported to increase the oil yield (Makinde & Akinoso, Citation2013). This might be associated with the removal of the outer coat of the seed during dehulling, which increases the extraction efficiency of the oil. Furthermore, the hull might be responsible for absorption of the oil leading to a lower volume of the extracted oils. The seed processing treatments of roasting and cooking were also reported to increase the oil content (Mariod et al., Citation2012; Tenyang et al., Citation2017) as compared to the raw seeds. The water loss during roasting could contribute to better extraction efficiency as indicated in Tenyang et al. (Citation2017). Protein denaturation of the oil body due to heat could also contribute to the increasing release of the oil during extraction. Therefore, as compared to raw sesame, higher percentage of oil yield was achieved for all varieties after pre-oil extraction treatments, particularly from dehulled and roasted samples.
Table 2. Mean values of oil content, RI, color, and IV of oils obtained from pre-oil extraction processing treatments of five sesame varieties.
3.2. Color
The color of oil samples extracted from different sesame varieties treated differently before oil extraction are presented in red and yellow units (). The mean color values for red units ranged from 2.07 to 6.20 in all varieties and pre-oil extraction processing treatments. There was no significant variation of oil color observed as affected by variety in terms of the red unit (). However, pre-oil extraction treatment showed significant differences (p < .05) in terms of the red unit (dehulling>roasting>cooking>raw) but with no difference (p > .05) for yellow. Similarly, dehulling, roasting, and cooking were found to increase the color intensity in sesame oil (Elleuch et al., Citation2007). The increase of color intensity of oil might be due to the accumulation of products from nonvolatile decomposition such as oxidized triacylglycerols and FFA during processing (Chandran et al., Citation2017).
Different reports (Agila & Barringer, Citation2012; Şimşek et al., Citation2015) also confirmed that the application of heating increases the intensity of red color units. The color formation in sesame oil during roasting could be attributed to the non-enzymatic browning and phospholipids degradation (Borchani et al., Citation2010; Elleuch et al., Citation2007). Różańska et al. (Citation2019) also investigated the increase in color pigments in crude oil during roasting due to the increase in efficiency of extraction of chlorophyll and β-carotene due to the rupturing of cell membranes. However, the color intensity of yellow units for all samples is at the maximum level of Lovibond yellow (70.00) with no significant difference (p > .05) (). This could be due to the natural deep yellow color of sesame oil.
In general, the color value is an important measurement to predict the quality and acceptability of edible oil (Muzzio et al., Citation2014; Tan et al., Citation2004). The lower value for the red color unit indicates better oil quality with fewer impurities. Oil samples extracted from the raw, cooked, and roasted sesame seed were better than compared to the oil extracted from dehulled seed in terms of color quality. Humera-1 and Setit-1 were better among varieties of sesame in terms of color.
3.3. Refractive index
The refractive index (RI) ranged from 1.4698 to 1.4701 (). Some of the values in this study are relatively lower than RI of sesame oil from Sudan, Morocco, and Yemen (El Khier et al., Citation2008; Gharby et al., Citation2017; Mohammed et al., Citation2018), but higher than the varieties from Egypt (Hassan, Citation2012; Latif & Anwar, Citation2011). The values measured in this study are higher than the Ethiopian Standard Agency (ESA) which is 1.465–1.469 (Ethiopian Standard Agency [ESA], Citation2014). An increase in RI from the normal range is an indication of deterioration of the oil due to an increase in the amount of conjugated fatty acids (Godswill et al., Citation2018). RI of oils is related to the free fatty acids, the average degree of unsaturation, and length of the hydrocarbon chain, and status of oxidation of oils. However, pre-oil extraction treatments showed a less or no significant effect on RI value as compared to raw seed (). Consistent with this result, Elleuch et al. (Citation2007) revealed that there is no significant effect due to processing treatments of dehulling and roasting on RI of sesame oil.
3.4. Iodine value
The mean iodine value (IV) of different varieties pre-treated under different conditions ranged between 106 and 113 g I2/100 g () and falls within the range of standards (104–120 g I2/100 g) set by the Ethiopian Standard Agency (ESA, Citation2014) and codex joints of FAO/WHO for sesame oil (Gunstone, Citation2011). Seed processing treatments and varieties showed a significant difference (p < .05) in IV of oil. Among the seed processing treatments roasting showed relatively higher values as compared to dehulling treatments for all seed varieties. Also, roasting resulted in a relatively higher IV as compared to cooking. Increased IV due to high-temperature roasting was similarly reported by Tenyang et al. (Citation2017). Comparing IV of oils of studied varieties, WO variety exhibited the highest value followed by GA>SE>AB>HU (). In general, the IV is related to the total number of double bonds (C=C) or degree of unsaturation of oils, and the higher the value the better oil quality (Gharby et al., Citation2017; Kyriakidis & Katsiloulis, Citation2000).
3.5. Free fatty acids
Significant (p < .05) variation in free fatty acids (FFA) of oil samples was observed as affected by variety and pre-oil extraction processing treatments. shows the effect of pre-oil extraction processing treatment and variety on FFA values of sesame oils. The values ranged from 0.31 to 1.36% in all oil samples, which is in agreement with sesame seed oil from Morocco (Gharby et al., Citation2017), Sudan (El Khier et al., Citation2008), Cameroon (Tenyang et al., Citation2017), and Congo (Nzikou et al., Citation2009).
Figure 4. Changes in free fatty acid (FFA) value of oil from five sesame varieties due to roasting, cooking and dehulling as compared to the raw (unprocessed).
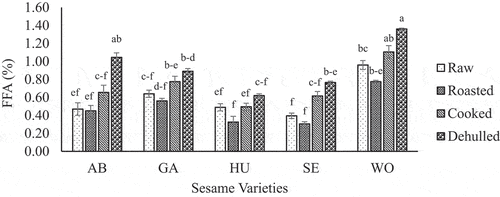
The FFA composition of varieties is the highest from the oil of Wollega (WO) variety, followed by Gojam Azene (GA)>Setit1 (SE)>Abasena (AB)>Humera1 (HU). This implies that sesame oil from HU or AB variety would be more stable against oxidative degradation as compared to WO, GA, and SE. The values measured in this study are still below 4% recommended for healthy sesame oil intended for human consumption by Codex Alimentarius (FAO/WHO, Citation2009). The result values are also below the maximum limit of the Ethiopian standard set for sesame oil specification which is 2% (ESA, Citation2014).
The highest FFA value of oil is from dehulled (0.62–1.36%) seeds followed by cooked (0.50–1.11%) and raw sample (0.47–0.96%). The higher value from the dehulling process might be associated with exposure of the seed oil to high moisture that enhances the oil hydrolysis. Since higher FFA is an indicator of the extent of oil hydrolysis leading to deterioration (Abdulkarim et al., Citation2007; Mahesar et al., Citation2014). On the other hand, oils from roasted seeds result in the lowest (0.31–0.78%) values. It might be that roasting as a dry heat treatment could reduce moisture in the seed to make the oil less prone to hydrolysis. Heat treatment also might contribute to inactivate lipase enzymes to minimize their role in the degradation of fatty acids. In a similar work, Ji et al. (Citation2019) reported that the FFA or acid value of sesame oil decreased with a roasting temperature of 160°C and 180°C. However, a contradicting result indicated that an increased in FFA content with an increase in roasting temperature (Ji et al., Citation2019; Tenyang et al., Citation2017). Cooking or boiling of seeds before extraction of oil might also contribute to inactivate lipase enzymes as compared to no heat treatment for a dehulled sample. Therefore, the roasting of seeds offers a better advantage in terms of reduction of FFA from sesame oil even if the FFA composition of oils from all pre-oil extraction processing treatments is an acceptable level.
3.6. Peroxide value
depicts the effect of pre-oil extraction treatments on peroxide value (PV) of sesame seed oils of different varieties treated under different pre-oil extraction treatments. The values varied from 0.7 to 5.9 meq O2/kg, the lowest value belongs to the control (raw) sesame seed oil, while the highest belongs to cooked seed oil. This result is in agreement with the findings reported on sesame seed oil in previous studies (Gharby et al., Citation2017; Ogbonna & Ukaan, Citation2013; Tenyang et al., Citation2017). Even if the PV of oil samples from cooked sesame is the highest, the value is still lower than the standard set by Ethiopian Standard Agency and FAO/WHO, which is 10 meq O2/kg for edible oil (ESA, Citation2014; FAO/WHO, Citation2009).
Figure 5. Changes in peroxide value of oil from five sesame varieties due to roasting, cooking and dehulling as compared to the raw (unprocessed).
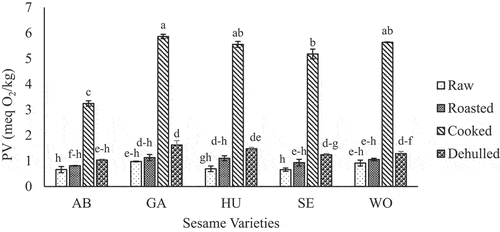
PV is an indicator of lipid oxidation due to processing or storage duration (Gotoh & Wada, Citation2006). A higher value due to seed processing treatment of sesame oil was also reported in different works (Abou-Gharbia et al., Citation1997; Kinge et al., Citation2019; Mariod et al., Citation2012). It might be due to the high accumulation or formation of hydroperoxides during cooking as compared to other pre-oil extraction processing treatments (Nyam et al., Citation2013). Hydroperoxides are primary oxidation products and unstable during heating, then transformed rapidly to several secondary oxidation products such as aldehydes, ketones, and esters (Loukou et al., Citation2019) during processing. On the contrary, a decrease in PV of sesame seed oil after boiling/cooking treatment has been reported in a previous study (Tenyang et al., Citation2017). The dissimilarity might be attributed to the variation in processing methods employed by the authors.
Dehulling and roasting treatments had also increased the PV as compared to the raw seeds (). In agreement with the results in this study, oil from dehulled sesame seed were found to have higher PV than oils from coated seeds (Abou-Gharbia et al., Citation1997). This indicates that the oil obtained from the raw intact sesame seed was more stable than oil obtained from dehulled seeds, which might be associated with an antioxidant effect of the seed coat in various in vitro systems (Chang et al., Citation2002; Jeon et al., Citation2016).
An increase in PV with sesame roasting was also reported (Ji et al., Citation2019; Tenyang et al., Citation2017). The increase during roasting might be the result of thermal oxidation of unsaturated fatty acids. However, Ji et al. (Citation2019) showed a decrease in peroxide value when the roasting temperature is above 180°C.
3.7. Fatty acids composition
Fatty acid (FA) composition of sesame oil with varying amounts of saturated fatty acids (SFA), monounsaturated fatty acids (MUFA), and polyunsaturated fatty acids (PUFA) from different seed varieties are shown in . The commonly identified FA compositions in the oil samples were Palmitic acid (C16:0), Palmitoleic acid (C16:1), Stearic acid (C18:0), Oleic acid (C18:1), Linoleic acid (C18:2), Linolenic acid (C18:3), Eicosanoic acid (C20:0), Eicosenoic acid (C20:1) and Behenic acid (C22:0). Palmitic acid (8.7–11.1%) was the highest SFA found in oil samples followed by Stearic acid (5.7–7.5%). Oleic (36.7–41.6%) and Linoleic acid (41.5–44.8%) were the two abundant MUFA and PUFA in oil samples, respectively. As expected, the oils from different varieties and pre-oil extraction processing treatments were shown to contain high unsaturated fatty acids (UFA) from 80 to 85% and low SFA (15–19%) ().
Table 3. Mean values of fatty acid composition (%) of oils obtained from pre-oil extraction processing treatments of three sesame varieties.
Table 4. Mean values of fatty acid composition (%) of oils obtained from pre-oil extraction processing treatments of two sesame varieties.
FA composition values reported on sesame oil by earlier studies (El Khier et al., Citation2008; Gharby et al., Citation2017; Hassan, Citation2012; Ji et al., Citation2019; Mohammed et al., Citation2018; Tenyang et al., Citation2017) are consistent with the results obtained in our study. However, the observed few variations might be due to the difference in kind of genotype, oil processing methods, pre-harvest, and postharvest management practices as reported in previous works (Gharby et al., Citation2017; Hassan, Citation2012; Kadkhodaie et al., Citation2014). The oil from varieties of HU and SE consisted relatively more of SFA as compared to the rest of the varieties, whereas oil samples from WO showed the highest percentage of UFA () .
Oils from roasted samples for AB and GA varieties exhibited a relatively better (>3% increase) on UFA composition as compared to oil from dehulled sample, but with less difference to the raw sample. According to Ji et al. (Citation2019), no observable difference was found between roasted and unroasted sesame seed oil. However, more or less the same effect was observed for oils from raw and cooked seeds. For other varieties (HU, SE, and WO) oil from the raw seed exhibited a relatively higher percentage of UFA as compared to oils from other pre-oil extraction processing treatments. A significant change in FA composition (MUFA and PUFA) of sesame oils due to roasting is also indicated in Tenyang et al. (Citation2017).
It was further noted that a slight increment for all SFA was observed due to cooking treatment except in C16:0. The dehulling treatment made a variation on total SFA and UFA, however, with little changes. It also shows that dehulling processes did not cause a significant modification in the major fatty acid of sesame oil (Elleuch et al., Citation2007). However, the dehulling treatment decreased palmitic acid (C16:0), but increased Stearic acid (C18:0), Eicosanoic acid (C20:0), and Behenic acid (C22:0) as compared to oil from the raw seed (). Abou-Gharbia et al. (Citation1997) also indicated a few significant changes in the fatty acid composition of oil reported due to the dehulling treatment of sesame seeds.
FA composition is an essential indicator of the nutritional value and health benefits of oils. The compositions might vary with various types and pre-oil extraction processing conditions. However, as such, no significant variation was observed among oil samples from the studied varieties except relatively higher values of UFA from the WO variety. Values of UFA of oils for raw and roasted samples are more or less the same with a minor difference but relatively lower percentage values were recorded for oil samples from dehulled seeds except for SE variety. The lower percentage value of UFA from the dehulled sample might be associated with exposure of seed oil to ambient oxygen after removal of the hull, which results in oxidative degradation of UFA. The ratio of SFA/UFA is more or less consistent when pre-oil extraction processing treatments are considered but relatively varies among varieties, with relatively higher values for HU and SE varieties ().
4. Conclusion
This study has shown that the influence of variety and processing methods led to significant variation in sesame seeds oil content, color, FFA, PV and IV. The oil content had increased by processing treatments (dehulling > roasting > cooking) compared to the raw seeds. However, dehulled seed oils contained comparatively larger amounts of FFA acids and PV. On the other hand, roasted sesame seed oil has less FFA. Among the cultivar, WO gave higher oil yield followed by HU>GA>SE>AB. Fatty acid composition of sesame seeds oil was characterized by high UFA and low SFA contents with no significant variation on both UFA and SFA due to processing treatments. Overall, the findings from this study showed that the volume of oil extracted from sesame seeds can be increased by applying processing treatments. Oil from roasted seeds could be more stable than other pre-oil extraction processing treatments because of its lower free fatty acid level and peroxide value.
Acknowledgments
The authors are grateful to staffs of the H.E.J Research Institute of Chemistry and Dr Panjwani Center for Molecular Medicines and Drug Research, University of Karachi, Karachi, Pakistan.
Disclosure statement
No potential conflict of interest was reported by the author(s).
Additional information
Funding
References
- Abdulkarim, S., Long, K., Lai, O. M., Muhammad, S., & Ghazali, H. (2007). Frying quality and stability of high-oleic Moringa oleifera seed oil in comparison with other vegetable oils. Food Chemistry, 105(4), 1382–1389. https://doi.org/10.1016/j.foodchem.2007.05.013
- Abou-Gharbia, H., Shahidi, F., Adel, A., Shehata, Y., & Youssef, M. (1997). Effects of processing on oxidative stability of sesame oil extracted from intact and dehulled seeds. Journal of the American Oil Chemists’ Society, 74(3), 215–221. https://doi.org/10.1007/s11746-997-0126-9
- Abu-Jdayil, B., Al-Malah, K., & Asoud, H. (2002). Rheological characterization of milled sesame (tehineh). Food Hydrocolloids, 16(1), 55–61. https://doi.org/10.1016/S0268-005X(01)00040-6
- Agila, A., & Barringer, S. (2012). Effect of roasting conditions on color and volatile profile including HMF level in sweet almonds (Prunus dulcis). Journal of Food Science, 77(4), C461–468. https://doi.org/10.1111/j.1750-3841.2012.02629.x
- Anilakumar, K. R., Pal, A., Khanum, F., & Bawa, A. S. (2010). Nutritional, medicinal and industrial uses of sesame (Sesamum indicum L.) seeds-an overview. Agriculturae Conspectus Scientificus, 75(4), 159–168.
- AOAC. (1990). Official method of analysis of the association of official analytical chemist. AOAC Press.
- AOCS. (2017). Official methods and recommended practices of the American oil chemist’s society. AOCS Press.
- Borchani, C., Besbes, S., Blecker, C., & Attia, H. (2010). Chemical characteristics and oxidative stability of sesame seed, sesame paste, and olive oils. Journal of Agricultural Science and Technology, 12(5), 585–596.
- Central Statistics Agency. (2018). Federal democratic republic Ethiopia: Agricultural sample survey 2017/2018 (2010 E.C) (Volume I. Report on Area and Production of crops (private peasant holdings, Meher season). Statistical Bulletin No: 586.
- Chandran, J., Nayana, N., Roshini, N., & Nisha, P. (2017). Oxidative stability, thermal stability and acceptability of coconut oil flavored with essential oils from black pepper and ginger. Journal of Food Science and Technology, 54(1), 144–152. https://doi.org/10.1007/s13197-016-2446-y
- Chang, L. -W., Yen, W. -J., Huang, S. C., & Duh, P. -D. (2002). Antioxidant activity of sesame coat. Food Chemistry, 78(3), 347–354. https://doi.org/10.1016/S0308-8146(02)00119-X
- El Khier, M. K. S., Ishag, K. E. A., & Yagoub, A. A. (2008). Chemical composition and oil characteristics of sesame seed cultivars grown in Sudan. Research Journal of Agriculture and Biological Sciences, 4(6), 761–766.
- Elleuch, M., Besbes, S., Roiseux, O., Blecker, C., & Attia, H. (2007). Quality characteristics of sesame seeds and by-products. Food Chemistry, 103(2), 641–650. https://doi.org/10.1016/j.foodchem.2006.09.008
- Ethiopian Standard Agency. (2014) . Sesame seed oil – Specification, CEA 15:2014.
- FAOSTAT. (2020). Food and agriculture organization of the statistical databases. http://www.fao.org/faostat/en/#data/QC
- FAO/WHO. (2009). Report of the 21st session of the codex alimentarius committee on fats and oils.
- Gharby, S., Harhar, H., Bouzoubaa, Z., Asdadi, A., El Yadini, A., & Charrouf, Z. (2017). Chemical characterization and oxidative stability of seeds and oil of sesame grown in Morocco. Journal of the Saudi Society of Agricultural Sciences, 16(2), 105–111. https://doi.org/10.1016/j.jssas.2015.03.004
- Godswill, A. C., Amagwula, I. O., Igwe, V. S., & Gonzaga, A. I. (2018). Effects of repeated deep frying on refractive index and peroxide value of selected vegetable oils.
- Gotoh, N., & Wada, S. (2006). The importance of peroxide value in assessing food quality and food safety. JAOCS, Journal of the American Oil Chemists’ Society, 83(5), 473. https://doi.org/10.1007/s11746-006-1229-4
- Gunstone, F. (2011). Vegetable oils in food technology: Composition, properties and uses. John Wiley & Sons.
- Hassan, M. A. (2012). Studies on Egyptian sesame seeds (Sesamum indicum L.) and its products 1-physicochemical analysis and phenolic acids of roasted Egyptian sesame seeds (Sesamum indicum L.). World Journal of Dairy & Food Sciences, 7(2), 195–201.
- Hwang, L. S. (2005). Sesame oil. In F. Shahidi (Ed.), Bailey’s industrial oil and fat products (6th ed., pp. 537–576). John Wiley & Sons.
- Jeon, J. -S., Park, C. L., Syed, A. S., Kim, Y. -M., Cho, I. J., & Kim, C. Y. (2016). Preparative separation of sesamin and sesamolin from defatted sesame meal via centrifugal partition chromatography with consecutive sample injection. Journal of Chromatography B, 1011, 108–113. https://doi.org/10.1016/j.jchromb.2015.12.062
- Ji, J., Liu, Y., Shi, L., Wang, N., & Wang, X. (2019). Effect of roasting treatment on the chemical composition of sesame oil. Lwt, 101, 191–200. https://doi.org/10.1016/j.lwt.2018.11.008
- Kadkhodaie, A., Razmjoo, J., Zahedi, M., & Pessarakli, M. (2014). Oil content and composition of sesame (Sesamum indicum L.) genotypes as affected by irrigation regimes. Journal of the American Oil Chemists’ Society, 91(10), 1737–1744. https://doi.org/10.1007/s11746-014-2524-0
- Kinge, E. E., Tonfack Djikeng, F., Karuna, M. S. L., Zambou Ngoufack, F., & Womeni, H. M. (2019). Effect of boiling and roasting on the physicochemical properties of Djansang seeds (Ricinodendron heudelotii). Food Science & Nutrition, 7(11), 3425–3434. https://doi.org/10.1002/fsn3.1163
- Kyriakidis, N. B., & Katsiloulis, T. (2000). Calculation of iodine value from measurements of fatty acid methyl esters of some oils: Comparison with the relevant American oil chemists society method. Journal of the American Oil Chemists’ Society, 77(12), 1235–1238. https://doi.org/10.1007/s11746-000-0193-3
- Latif, S., & Anwar, F. (2011). Aqueous enzymatic sesame oil and protein extraction. Food Chemistry, 125(2), 679–684. https://doi.org/10.1016/j.foodchem.2010.09.064
- Loukou, A., Agbo, A., Traore, S., Zoro, B., Lognay, G., & Brou, K. (2019). Effect of cooking and extraction method on oleaginous cucurbit seed oils quality. African Crop Science Journal, 27(1), 77–88. https://doi.org/10.4314/acsj.v27i1.6
- Mahesar, S., Sherazi, S., Khaskheli, A. R., Kandhro, A. A., & Uddin, S. (2014). Analytical approaches for the assessment of free fatty acids in oils and fats. Analytical Methods, 6(14), 4956–4963. https://doi.org/10.1039/C4AY00344F
- Makinde, F., & Akinoso, R. (2013). Nutrient composition and effect of processing treatments on anti nutritional factors of Nigerian sesame (Sesamum indicum Linn) cultivars. International Food Research Journal, 20(5), 2293–2300.
- Mariod, A. A., Ahmed, S. Y., Abdelwahab, S. I., Cheng, S. F., Eltom, A. M., Yagoub, S. O., & Gouk, S. W. (2012). Effects of roasting and boiling on the chemical composition, amino acids and oil stability of safflower seeds. International Journal of Food Science & Technology, 47(8), 1737–1743. https://doi.org/10.1111/j.1365-2621.2012.03028.x
- Mohammed, F., Abdulwali, N., Guillaume, D., Tenyang, N., Ponka, R., Al-Gadabi, K., Bchitou, R., Abdullah, A. H., & Naji, K. M. (2018). Chemical composition and mineralogical residence of sesame oil from plants grown in different Yemeni environments. Microchemical Journal, 140, 269–277. https://doi.org/10.1016/j.microc.2018.04.011
- Muzzio, C. R., Díaz, R. J., & Dini, N. G. (2014). In-line measurement of sunflower oil color in the Lovibond scale using a low-cost robust device. Journal of Food Engineering, 120, 88–93. https://doi.org/10.1016/j.jfoodeng.2013.07.023
- Nyam, K., Wong, M., Long, K., & Tan, C. (2013). Oxidative stability of sunflower oils supplemented with kenaf seeds extract, roselle seeds extract and roselle extract, respectively under accelerated storage. International Food Research Journal, 20(2), 695–701.
- Nzikou, J., Matos, L., Bouanga-Kalou, G., Ndangui, C., Pambou-Tobi, N., Kimbonguila, A., Silou, T., Linder, M., & Desobry, S. (2009). Chemical composition on the seeds and oil of sesame (Sesamum indicum L.) grown in Congo-Brazzaville. Advance Journal of Food Science and Technology, 1(1), 6–11.
- Ogbonna, P., & Ukaan, S. (2013). Chemical composition and oil quality of seeds of sesame accessions grown in the Nsukka plains of South Eastern Nigeria. African Journal of Agricultural Research, 8(9), 797–803.
- Pathak, N., Rai, A., Kumari, R., & Bhat, K. (2014). Value addition in sesame: A perspective on bioactive components for enhancing utility and profitability. Pharmacognosy Reviews, 8(16), 147. https://doi.org/10.4103/0973-7847.134249
- Różańska, M. B., Kowalczewski, P. Ł., Tomaszewska-Gras, J., Dwiecki, K., & Mildner-Szkudlarz, S. (2019). Seed-roasting process affects oxidative stability of cold-pressed oils. Antioxidants, 8(8), 313. https://doi.org/10.3390/antiox8080313
- Şimşek, E., Özcan, M., Arslan, D., Ünver, A., & Kanbur, G. (2015). Changes in chemical composition of some oils extracted from seeds roasted at different temperatures. Quality Assurance and Safety of Crops & Foods, 7(5), 801–808. https://doi.org/10.3920/QAS2014.0509
- Tan, Y. A., Kuntom, A., Lee, C. K., & Low, K. S. (2004). Comparative evaluation of palm oil color measurement using a prototype palm oil colorimeter. Journal of the American Oil Chemists’ Society, 81(8), 733–736. https://doi.org/10.1007/s11746-004-0970-z
- Tenyang, N., Ponka, R., Tiencheu, B., Djikeng, F. T., Azmeera, T., Karuna, M. S., Prasad, R. B., & Womeni, H. M. (2017). Effects of boiling and roasting on proximate composition, lipid oxidation, fatty acid profile and mineral content of two sesame varieties commercialized and consumed in Far-North Region of Cameroon. Food Chemistry, 221, 1308–1316. https://doi.org/10.1016/j.foodchem.2016.11.025
- Wan, Y., Li, H., Fu, G., Chen, X., Chen, F., & Xie, M. (2015). The relationship of antioxidant components and antioxidant activity of sesame seed oil. Journal of the Science of Food and Agriculture, 95(13), 2571–2578. https://doi.org/10.1002/jsfa.7035