Abstract
1G, a novel derivative of curcumin, exhibits promising anticancer activities in various cancer cell lines. To support its further pharmacological studies, a liquid chromatography–tandem mass spectrometry method was developed and validated in accordance with FDA's Guidance. After extraction by protein precipitation, analytes were separated by a 4.5 min gradient elution (water/0.1% formic acid and methanol) on a reverse-phase C18 column at 40 °C. The multiple reaction monitoring mode was used for quantification on a triple quadrupole mass spectrometer with positive ionization. The assay was linear over the concentration range of 5–1000 ng/mL with a correlation coefficient (r) greater than 0.99. Values of intra- and inter-day precision and accuracy were satisfactory, i.e. <10.1% for precision and within ± 14.5% for accuracy. No obvious matrix effect was observed. Recovery of the analyte was higher than 95.3%. 1G was stable during the whole analytic process. The validated method was successfully applied to the pharmacokinetic study of 1G after intravenous and intraperitoneal administration in rats. Favorable pharmacokinetic profiles were demonstrated, including good abdominal absorption (F = 62.58%), moderate clearance and high extravascular distribution. Results indicated that as a novel antitumor agent, 1G exhibited acceptable pharmacokinetic properties for further in vivo pharmacologic evaluation.
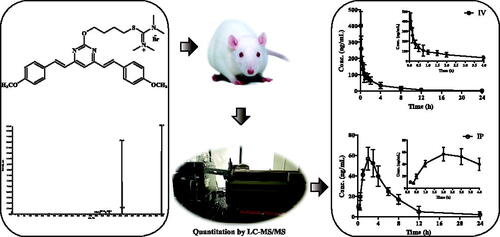
Disclosure statement
No potential conflict of interest was reported by the authors.