Abstract
Finding novel therapeutic agent for the treatment of cerebral ischemia is urgently required. These experiments explored the potential roles of 6-Gingerols (6G) in hypoxia-stimulated rat PC-12 cells. Cell viability, apoptosis and its related proteins were studied by the approaches of MTT assay, flow cytometry assay and Western blot analysis, respectively. In addition, whether 6G achieved its functions in hypoxia-induced injury through miR-103 was illustrated. Moreover, the associated signalling pathways were investigated. Obviously, hypoxia treatment blocked cell viability and enhanced apoptosis while this trend was ameliorated by 6G. Then we observed that hypoxia administration up-regulated miR-103 expression and 6G could further increase miR-103 expression in hypoxia-stimulated PC-12 cells. Inhibition of miR-103 attenuated the neuroprotective effects of 6G on hypoxia-treated PC-12 cells. Moreover, Bcl2/adenovirus EIB 19kD-interacting protein 3 (BNIP3) was a target of miR-103 and BNIP3 upregulation also attenuated the neuroprotective impact of 6G on hypoxia-treated PC-12 cells. Hypoxia activated the p38MAPK and JNK pathways were inactivated by 6G. To sum up, 6G protected hypoxia-stimulated PC-12 cells through miR-103-mediatated down-regulation of BNIP3 by inhibiting p38 MAPK and JNK pathways.
6-Gingerols (6G) is a promising agent for cerebral ischemia therapy.
The neuroprotective effects of 6G are mediated by miR-103 and BNIP3.
Up-regulation of miR-103 exerts neuroprotective effects.
Highlights
Introduction
Cerebral ischemia, which refers to the insufficient blood supply to the brain, includes two types: acute and chronic cerebral ischemia [Citation1,Citation2]. Acute cerebral ischemia is prevalent in seniors and it can increase the incident rate of cerebral infarction [Citation3]. The chronic cerebral ischemia might bring negative effects in cognitive and neurological functions which includes dizziness, impaired memory and sleep disturbance [Citation4]. Cerebral ischemia progression often goes with a hypoxia-induced injury in nerve cells [Citation5]. However, effective therapies against injury induced by cerebral ischemia are still limited. Therefore, getting a better understanding of the underlying mechanism in cerebral ischemia is urgently required.
Nowadays, the use of traditional medicine to treat cerebral ischemia has attracted attention. Ginger is an ancient and traditional medicinal and edible plant. It has been used to treat diseases in China for more than 2000 years. 6-Gingerols (6G), which is a phenolic ingredient purified from ginger, has been reported to exert anti-inflammation, anti-oxidative and anti-apoptotic roles [Citation5–7]. 6G is reported to alleviate myocardial ischemia/reperfusion injury [Citation8]. 6G can also reduce the levels of inflammatory markers, making it a potential strategy for the treatment of atherosclerosis [Citation9]. As for neuronal diseases, Feng indicated that 6G suppressed astrocyte overactivation, through which it contributed to the improvement of cognitive ability [Citation10]. However, whether 6G could be used for cerebral ischemia therapy still remains to be known.
MicroRNAs (miRNAs) play a vital role in regulating their target gene’s expression [Citation11]. Growing evidence has demonstrated the regulating roles of miRNAs in ischemic and its related disorder [Citation12,Citation13]. Among which, miR-103 was observed to relate to ischemic stroke angiogenesis [Citation14]. But it is rarely reported whether miR-103 could participate in traditional Chinese medicines to regulate their function for cerebral ischemia therapy. Furthermore, Bcl2/adenovirus EIB 19kD-interacting protein 3 (BNIP3), a well-known regulator of autophagy and a pro-apoptotic factor was associated with cerebral ischemia in rats [Citation15]. Therefore, we further determined whether BNIP3 co-operated with miR-103 to function in cerebral ischemia cells.
In addition, the previous study has pointed out that p38MAPK and JNK pathways are involved in cerebral ischemia in mice [Citation16]. Therefore, we also detected how these signal pathways are involved in.
Our present study investigated whether the exerted potential protective effects of 6G on the hypoxia-stimulated injury of PC-12 cells were through modulation of miR-103.
Materials and methods
Cell lines and treatment
The PC-12 cells were obtained from Cobioer (Nanjing, China). The PC-12 cells were seeded in DMEM medium (Jake bio, Shanghai, China). The medium contains 10% (v/v) fetal bovine serum (FBS, Procell, Wuhai, China), 100 U/ml penicillin and 100 μg/ml streptomycin (Sigma–Aldrich, St. Louis, USA). Cells were cultured at 37 °C in the environment containing 5% CO2. The 3% and 21% O2 concentrations were treated as the hypoxia and normoxia culture conditions, respectively.
6-Gingerol (Xi’an Realin Biotechnology, Xi’an, China) was dissolved in 0.1% ethanol.
MTT assay
The cell viability was detected using MTT colorimetric assay according to standard methods described before. Each experiment was performed three times.
Apoptosis assay
Different groups of cells were washed twice with phosphate buffer saline (PBS). Then 100 μl binding buffer and 10 μl fluorescein isothiocyanate (FITC) tagged Annexin-V were added and incubated in the darkness for 30 min. After that, 5 μl propidine iodide (PI) was administrated. 5 min later, 400 μl binding buffer was added and FACScan (Beckman Coulter, Fullerton, CA, USA) for flow cytometry was used. FlowJo software (FlowJo, Ashland, OR, USA) was used as the result analysis approach.
Western blot
Extracted proteins were quantified using radio immunoprecipitation assay (RIPA) lysis buffer (Solarbio, Beijing, China) as well as BCA™ Protein Assay Kit (Pierce, Appleton, WI, USA), respectively. Proteins were distinguished by 10% SDS-PAGE gel and then transferred to PVDF membranes. Later, primary antibodies were incubated into membranes at 4 °C, overnight. Then, secondary antibodies were added and maintained for 1 h. In the end, Image Lab™ Software (Bio-Rad, Shanghai, China) was used to detect the western blot band and Odyssey 3.0 software was used to quantify the intensity of the bands.
qRT-PCR
RNA extracting was accessed using Trizol reagent (Solarbio, Beijing, China). Firstly, miRNA to cDNA was performed using Taqman MicroRNA Reverse Transcription Kit. Secondly, cDNA was generated in a reverse transcription reaction using Taqman Universal Master Mix II (Thermo Fisher Scientific, Waltham, MA, USA). Thirdly, TaqMan MicroRNA Assay of miR-103 and U6 (Thermo Fisher Scientific, Waltham, MA, USA) were used for determining the miR-103 expression.
Transfection
MiR-103 inhibitor and the negative control (NC) were obtained from Life Technologies Corporation (MD, USA). Then, following Lipofectamine 2000 (Life Technologies, Carlsbad, CA, USA) instructions, miR-103 inhibitor and NC were transfected into cells.
Similarly, pBNIP3 or pcDNA3.1 control vector was transfected into cells. Cells were harvested after transfection for 48 h.
Dual luciferase activity assay
PCR was used to obtain the BNIP3 3’UTR target site and the luciferase reporter constructs with BNIP3 3’UTR and miR-103-binding site. Cell transfection was performed using Lipofectamine 3000 (Life Technologies, Waltham, MA, USA). Dual-luciferase assay system (Promega, WI, USA) was carried out for reporter assay.
Statistical analysis
Data results were expressed as the mean ± standard deviation (SD). GraphPad statistical software was accessed for statistical assay. The p-values were counted using student t-test or ANOVA followed by Tukey’s post-hoc test. P-values < .05 suggests significant difference.
Results
Hypoxia induces apoptosis and autophagy of PC-12 cells
Firstly, the effects of hypoxia on PC-12 cells were detected. We observed that hypoxia suppressed cell viability while increased cell apoptosis compared with control group significantly (; **p < .01; ***p < .001). Moreover, hypoxia treatment increased the expression of apoptosis-related proteins cleaved-caspase 3 and cleaved-caspase 9 (; ***p < .001). For autophagy-related proteins, hypoxia decreased the p62 expression while increasing light chain 3 (LC3)-II/LC3-I ratio (); **p < .01; ***p < .001). The above results pointed out that hypoxia caused cell injury as evidenced by inducing cell apoptosis and autophagy.
Figure 1. Hypoxia induces apoptosis and autophagy of PC-12 cells. (A) Cell viability was studied by MTT assay. (B) Flow cytometry analysis was performed for cell apoptosis detection. (C,D) Relative expression of apoptotic proteins was detected by Western blot. (E,F) The accumulated levels of p62 and LC3-II/LC3-I were detected by Western blot. Data are shown as mean ± SD. **p < .01; ***p < .001.
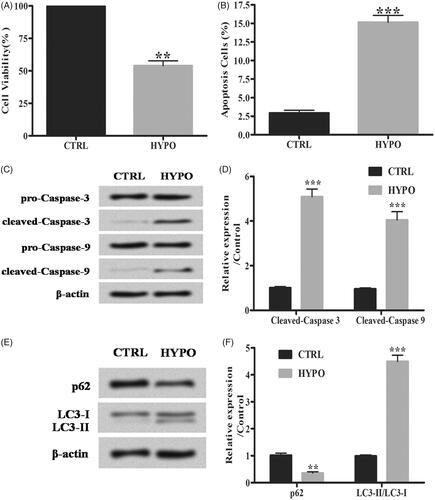
6-Gingerols (6G) alleviates hypoxia induced apoptosis and autophagy in PC-12 cells
The effects of 6G on hypoxia-induced injury in PC-12 cells were analyzed. 6G did not influence cell viability significantly with the concentration lower than 5 μM. However, it decreased cell viability at the concentration of 5 μM (; *p < .05). Then, 6G with the concentration of 5 μM was chosen to treat PC-12 cells under hypoxia. Our data showed that 6G up-regulated cell viability while down-regulating apoptosis in hypoxia (HYPO) + 6G group compared with HYPO group (; *p < .05; **p < .01; ***p < .001). Furthermore, 6G increased cleaved-caspase 3, cleaved-caspase 9 and p62 expression while decreasing LC3-II/LC3-I ratio compared with HYPO group (; *p < .05; **p < .01; ***p < .001). Above results suggested that 6G alleviated hypoxia-induced apoptosis and autophagy in PC-12 cells.
Figure 2. 6-Gingerols (6G) alleviates hypoxia induced apoptosis and autophagy in PC-12 cells. (A,B) Cell viability was detected by MTT assay. (C) Flow cytometry analysis was performed for cell apoptosis detection. (D,E) Relative expression of apoptotic factors was detected by Western blot. (F,G) The accumulated levels of p62 and LC3-II/LC3-I were detected by Western blot. Data are shown as mean ± SD. *p < .05; **p < .01; ***p < .001.
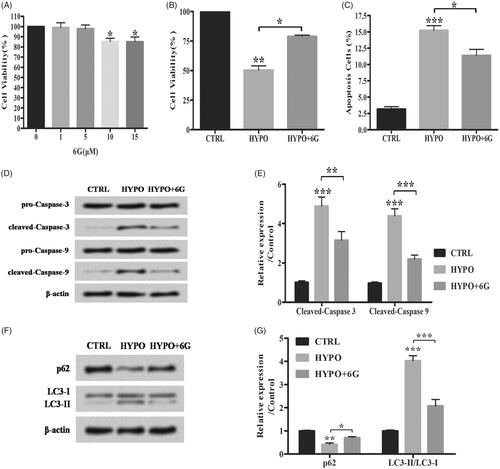
6-Gingerols (6G) increases expression of miR-103 in hypoxia-stimulated PC-12 cells
Results demonstrated that hypoxia increased miR-103 expression in PC-12 cells and 6G treatment could further increase miR-103 expression in PC-12 cells by hypoxia (). These results indicated that 6G could increase miR-103 expression in PC-12 cells.
6-Gingerols (6G) alleviates hypoxia-induced injury by up-regulating miR-103 in PC-12 cells
To further elucidate the role of miR-103 in 6G treated PC-12 cells, the miR-103 inhibitor was transfected to suppress miR-103 expression (; **p < .01). MiR-103 inhibitor down-regulated the increased cell viability while up-regulating the decreased apoptosis induced by 6G under hypoxia condition (; *p < .05; **p < .01; ***p < .001). Besides that, miR-103 inhibitor increased cleaved-caspase 3, cleaved-caspase 9 expression and LC3-II/LC3-I ratio while decreasing the p62 expression compared with HYPO + 6G + NC inhibitor group (; *p < .05; **p < .01; ***p < .001). Above results indicated that 6G alleviated hypoxia-induced injury by up-regulating miR-103 in PC-12 cells.
Figure 4. 6-Gingerols (6G) alleviates hypoxia induced injury by up-regulating miR-103 in PC-12 cells. (A) Relative expression of miR-103 was detected by qRT-PCR. (B) Cell viability was examined by MTT assay. (C) Flow cytometry analysis was performed for cell apoptosis detection. (D,E) Relative expression of apoptosis-related proteins was detected by Western blot. (F,G) Relative expression of p62 and LC3/LC3-I ratio were detected by Western blot. Data are reported as mean ± SD. *p < .05; **p < .01; ***p < .001.
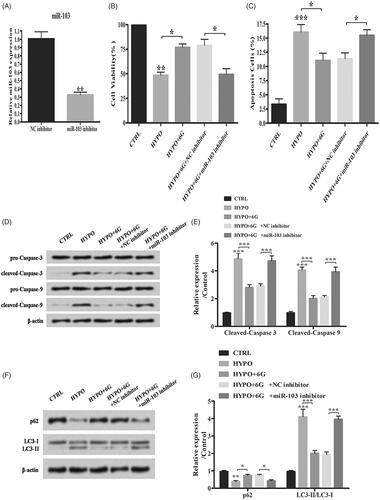
Bcl2/adenovirus EIB 19kD-interacting protein 3 (BNIP3) is a target of miR-103
Here, we observed that the hypoxia induced up-regulation of BNIP3 while 6G treatment had the opposite effect. MiR-103 inhibitor could increase the BNIP3 level compared with HYPO + 6G + NC inhibitor group (). Then, a luciferase reporter assay was performed to verify the regulation relationship between miR-103 and BNIP3. We can see from that miR-103 mimic significantly inhibited luciferase activity of BNIP3-wt. However, miR-103 mimic did not inhibit the luciferase activity of the reporter vector containing BNIP3-mut (, *p < .05). In summary, these data revealed that BNIP3 was a target of miR-103 in PC-12 cells.
Overexpression of BNIP3 induces apoptosis and autophagy of PC-12 cells
The role of BNIP3 in 6G treated PC-12 cells was investigated. Transfection was conducted for BNIP3 overexpression (; ***p < .001). Overexpression of BNIP3 counteracted 6G’s protective effect against hypoxia through increasing apoptosis (, ***p < .001). Overexpression of BNIP3 also increased cleaved-caspase 3, cleaved-caspase 9 expression and LC3-II/LC3-I ratio while decreasing p62 expression compared with HYPO + 6G + pcDNA3.1 group (; *p < .05; **p < .01; ***p < .001). These data revealed that overexpression of BNIP3 counteracted 6G’s protective effect against hypoxia through inducing apoptosis and autophagy in PC-12 cells.
Figure 6. 6-Gingerols (6G) inhibits p38 MAPK and JNK signalling pathways through down-regulation of BNIP3. (A,B) Relative expression of BNIP3 was detected by Western blot. (C) Flow cytometry analysis was performed for cell apoptosis detection. (D,E) Relative expression of apoptosis-related factors was detected by Western blot. (F,G) Relative expression of p62 and LC3/LC3-I ratio were detected by Western blot. Data are reported as mean ± SD. *p < .05; **p < .01; ***p < .001.
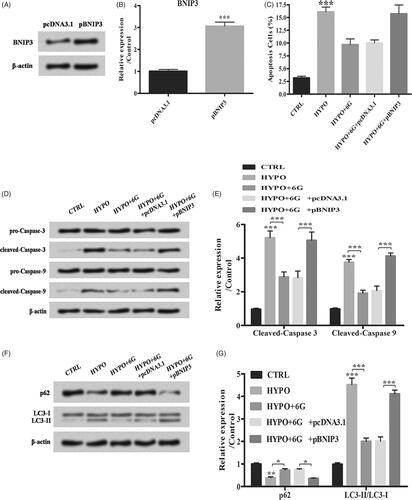
6-Gingerols (6G) inhibits p38 mitogen-activated protein kinase (MAPK) and c-Jun NH 2-terminal kinase (JNK) signalling pathways through down-regulation of BNIP3
Results from Western blot showed that hypoxia increased p/t-p38MAPK and p/t-JNK ratios while 6G treatment alleviated its effects (; p < 0.001). Moreover, BNIP3 overexpression increased the p/t-p38MAPK and p/t-JNK ratios compared with HYPO + 6 G + pcDNA3.1 group (; **p < .01; ***p < .001). Our results elucidated that 6G inhibited p38MAPK and JNK signalling pathways through down-regulation of BNIP3 in PC-12 cells.
Figure 7. 6-Gingerols (6G) inhibits p38 MAPK and JNK signalling pathways through down-regulation of BNIP3. (A,B) Relative expression of t-p38MAPK and p-p38MAPK was measured by Western blot. (C,D) Relative expression of t-JNK and p-JNK was detected by Western blot. Data are reported as mean ± SD. **p < .01; ***p < .001.
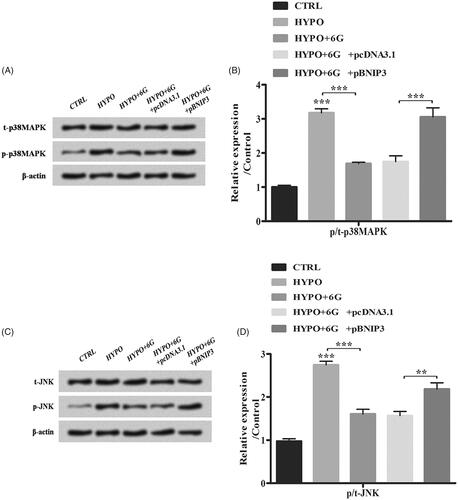
Discussion
Cerebral ischemia, as a type of disorder in the central nervous system, is often caused by ischemia and hypoxia [Citation1]. Nowadays, effective therapies are applied to ameliorate ischemic injury but they still remain far from satisfaction. The current study established in vitro cell model in PC-12 cells and demonstrated that 6G counteracted hypoxia-induced injury through suppressing apoptosis and autophagy. MiR-103 was demonstrated to be up-regulated in hypoxia-stimulated cells and knockdown of miR-103 counteracted the protective effects of 6G by targeting BNIP3. This is the first study which built a network between cytoprotective functions of 6G and the functions of miR-103/BNIP3 in hypoxia-induced injury. From our limited knowledge, this is the first time that the roles of 6G were approved in hypoxia-treated PC-12 cells through regulation of miR-103/BNIP3.
PC-12 cells are classically used to explore the mechanism of neuronal injury induced by hypoxia [Citation17,Citation18]. In our present study, firstly, the effects of 6G on hypoxia-treated PC-12 cells were measured. In addition, caspases were activated in the process of apoptosis [Citation19]. Autophagy is also a type of cell death which degrades and recycles long-lived or damaged proteins and cytoplasmic organelles by lysosomes [Citation20]. During autophagy, p62 protein is degraded while LC3-I is converted to LC3-II [Citation21]. Here, results showed a hypoxia-induced up-regulation of cleaved-caspase 3, cleaved-caspase 9 and an increase of LC3-II/LC3-I ratio while down-regulating of p62. Meanwhile, it was obviously seen from results that these trends were attenuated by 6G, suggesting that 6G might decrease hypoxia-induced PC-12 cells injuries through repressing apoptosis and autophagy. Similarly, previous studies reported that 6G inhibited apoptosis in response to myocardial ischemia/reperfusion (I/R) injury as a possible mechanism to attenuate I/R-induced injury in the heart [Citation8]. Besides that, the neuroprotective effects of 6G have been reported in scopolamine-induced amnesia in C57BL/6 mice [Citation22]. However, to our knowledge, this is the first study to show that 6G exerted neuroprotective effects by suppressing hypoxia-induced apoptosis and autophagy in PC-12 cells.
In addition, we investigated whether there was a regulation association between 6G and miR-103. Intriguingly, we found out that miR-103 expression was significantly enhanced by hypoxia, and 6G treatment, further, increased miR-103 expression in hypoxia-treated cells. A previous study suggested that several miRNAs are regulated by hypoxia and miR-103 was reported to be up-regulated under hypoxia [Citation23]. As 6G could further increase miR-103 expression in PC-12 cells under hypoxia, we considered that up-regulation of miR-103 could be against hypoxia-induced injury. To verify our hypothesis, miR-103 inhibitor was transfected into PC-12 cells for the knockdown of miR-103. Our results showed that the neuroprotective effects of 6G on hypoxia-stimulated PC-12 cells could be alleviated by miR-103 knockdown, suggesting that up-regulation of miR-103 could be one explanation for the neuroprotective effects of 6G in hypoxia-treated cells.
miRNAs are responsible for the post-translational regulation of mRNA expression through targeting them [Citation24]. BNIP3 belongs to the BH3 subfamily of the Bcl-2 superfamily and is a proapoptotic protein [Citation25]. It has been reported that BNIP3 is increased by the treatment of hypoxia through the activation of hypoxia inducing factor 1 (HIF1) and it is closely related with autophagic and apoptotic cell deaths in response to hypoxia [Citation26–28]. Combined with the roles of 6G on hypoxia-induced apoptosis and autophagy, we inferred that BNIP3 might exert crucial roles in the regulatory mechanism of 6G. Results demonstrated that hypoxia increased BNIP3 expression while 6G had the opposite effect. BNIP3 expression was negatively modulated by miR-103. Furthermore, results from dual luciferase activity assay indicated that BNIP3 was a target of miR-103. BNIP3 upregulation was observed to counteract the neuroprotective effects of 6G on hypoxia-injured PC-12 cells, suggesting that 6G reduced hypoxia-induced PC-12 cells apoptosis and autophagy through up-regulating miR-103 and down-regulating BNIP3.
P38MAPK and JNK signalling pathways are important signal pathways, regulated in the biological activities from the cell membrane to the nucleus [Citation29]. Studies have demonstrated that p38MAPK and JNK pathways can be activated by a variety of stimuli including hypoxia [Citation30]. Hence, inactivating ERK1/2, p38MAPK and JNK or antioxidants might have effects in treating injuries induced by hypoxia [Citation31]. Consistent with previous researches, we also observed that hypoxia activated p38MAPK and JNK pathways while 6G showed the opposite effects. Overexpression of BNIP3 was found to activate the inhibited p38MAPK and JNK pathways in 6G treated group. Interestingly, JNK and BNIP3 were reported to work as an axis “JNK/BNIP3” in hepatocellular carcinoma [Citation32]. Combined with the previous study and our data, we inferred that BNIP3 and p38MAPK and JNK pathways might work in a network in regulating cell biological activities.
In conclusion, our study investigated the role of 6G in hypoxia-exposed PC-12 cells and results showed that 6G exerted neuroprotective effects in hypoxia-stimulated PC-12 cells by regulating miR-103 which targeted BNIP3. BNIP3 overexpression activated the p38MAPK and JNK pathways. This study might provide some novel insight for the study of 6G function, making 6G a promising agent for cerebral ischemia therapy.
Disclosure statement
No potential conflict of interest was reported by the authors.
References
- Zhao ZY, Gao YY, Gao L, et al. Protective effects of bellidifolin in hypoxia-induced in pheochromocytoma cells (PC12) and underlying mechanisms. J Toxicol Env Health Part A. 2017;80:1187–1192.
- Yang Y, Rosenberg GA. Blood-brain barrier breakdown in acute and chronic cerebrovascular disease. Stroke 2011;42:3323–3328.
- Zhou Y, Lu M, Du RH, et al. MicroRNA-7 targets Nod-like receptor protein 3 inflammasome to modulate neuroinflammation in the pathogenesis of Parkinson’s disease. Mol Neurodegen. 2016;11:28.
- Fedin AI. The efficacy of cortexin and memantinol (memantine) in the treatment of cognitive impairment in patients with chronic cerebral ischemia. Z Nevrol Psikhiatr im SS Korsakova. 2018;118:30–36.
- Zheng WX, Cao XL, Wang F, et al. Baicalin inhibiting cerebral ischemia/hypoxia-induced neuronal apoptosis via MRTF-A-mediated transactivity. Euro J Pharmacol. 2015;767:201–210.
- Li Y, Xu B, Xu M, et al. 6-Gingerol protects intestinal barrier from ischemia/reperfusion-induced damage via inhibition of p38 MAPK to NF-kappaB signalling. Pharmacol Res. 2017;119:137–148.
- Choi JG, Kim SY, Jeong M, et al. Pharmacotherapeutic potential of ginger and its compounds in age-related neurological disorders. Pharmacol Ther. 2018;182:56–69.
- Lv X, Xu T, Wu Q. 6-Gingerol activates PI3K/Akt and inhibits apoptosis to attenuate myocardial ischemia/reperfusion injury. Evidence-Based Compl Alter Med. 2018;2018:9024034.
- Wang S, Tian M, Yang R, et al. 6-Gingerol ameliorates behavioral changes and atherosclerotic lesions in ApoE(–/–) mice exposed to chronic mild stress. Cardiovasc Toxicol. 2018;18:420–430.
- Zhang F, Zhang JG, Yang W, et al. 6-Gingerol attenuates LPS-induced neuroinflammation and cognitive impairment partially via suppressing astrocyte overactivation. Biomed Pharmacother. 2018;107:1523–1529.
- Ouyang YB, Stary CM, Yang GY, et al. microRNAs: innovative targets for cerebral ischemia and stroke. Curr Drug Targ. 2013;14:90–101.
- Wan Y, Jin HJ, Zhu YY, et al. MicroRNA-149-5p regulates blood-brain barrier permeability after transient middle cerebral artery occlusion in rats by targeting S1PR2 of pericytes. FASEB J. 2018;32:3133–3148.
- Wang J, Zhang Y, Xu F. Function and mechanism of microRNA-210 in acute cerebral infarction. Exp Ther Med. 2018;15:1263–1268.
- Shi FP, Wang XH, Zhang HX, et al. MiR-103 regulates the angiogenesis of ischemic stroke rats by targeting vascular endothelial growth factor (VEGF). Iran J Basic Med Sci. 2018;21:318–324.
- Althaus J, Bernaudin M, Petit E, et al. Expression of the gene encoding the pro-apoptotic BNIP3 protein and stimulation of hypoxia-inducible factor-1alpha (HIF-1alpha) protein following focal cerebral ischemia in rats. Neurochem Int. 2006;48:687–695.
- Zhen Y, Ding C, Sun J, et al. Activation of the calcium-sensing receptor promotes apoptosis by modulating the JNK/p38 MAPK pathway in focal cerebral ischemia-reperfusion in mice. Am J Translat Res. 2016;8:911–921.
- Wang Q, Li L, Li CY, et al. SIRT3 protects cells from hypoxia via PGC-1alpha- and MnSOD-dependent pathways. Neuroscience 2015;286:109–121.
- Hartwig K, Fackler V, Jaksch-Bogensperger H, et al. Cerebrolysin protects PC12 cells from CoCl2-induced hypoxia employing GSK3beta signaling. Int J Develop Neurosci. 2014;38:52–58.
- Chio CC, Wei L, Chen TG, et al. Neuron-derived orphan receptor 1 transduces survival signals in neuronal cells in response to hypoxia-induced apoptotic insults. J Neurosurg. 2016;124:1654–1664.
- Song S, Tan J, Miao Y, et al. Intermittent-hypoxia-induced autophagy activation through the ER-stress-related PERK/eIF2alpha/ATF4 pathway is a protective response to pancreatic beta-cell apoptosis. Cell Physiol Biochem. 2018;51:2955–2971.
- Tanida I, Ueno T, Kominami E. LC3 conjugation system in mammalian autophagy. Int J Biochem Cell Biol. 2004;36:2503–2518.
- Kim CY, Seo Y, Lee C, et al. Neuroprotective effect and molecular mechanism of [6]-Gingerol against scopolamine-induced amnesia in C57BL/6 mice. Evidence-Based Compl Alter Med. 2018;2018:8941564.
- Chen Z, Lai TC, Jan YH, et al. Hypoxia-responsive miRNAs target argonaute 1 to promote angiogenesis. J Clin Invest. 2013;123:1057–1067.
- Dykstra-Aiello C, Jickling GC, Ander BP, et al. Altered expression of long noncoding RNAs in blood after ischemic stroke and proximity to putative stroke risk loci. Stroke 2016;47:2896–2903.
- Zhao X, Liu L, Li R, et al. Hypoxia-inducible factor 1-alpha (HIF-1alpha) induces apoptosis of human uterosacral ligament fibroblasts through the death receptor and mitochondrial pathways. Med Sci Monit. 2018;24:8722–8733.
- Singh A, Azad M, Shymko MD, et al. The BH3 only Bcl-2 family member BNIP3 regulates cellular proliferation. PLoS One. 2018;13:e0204792.
- Vande Velde C, Cizeau J, Dubik D, et al. BNIP3 and genetic control of necrosis-like cell death through the mitochondrial permeability transition pore. Mol Cell Biol. 2000;20:5454–5468.
- Kanzawa T, Zhang L, Xiao L, et al. Arsenic trioxide induces autophagic cell death in malignant glioma cells by upregulation of mitochondrial cell death protein BNIP3. Oncogene 2005;24:980–991.
- Broom OJ, Widjaya B, Troelsen J, et al. Mitogen activated protein kinases: a role in inflammatory bowel disease? Clin Exper Immunol. 2009;158:272–280.
- Suganuma T, Workman JL. MAP kinases and histone modification. J Mol Cell Biol. 2012;4:348–350.
- Lan A, Liao X, Mo L, et al. Hydrogen sulfide protects against chemical hypoxia-induced injury by inhibiting ROS-activated ERK1/2 and p38MAPK signaling pathways in PC12 cells. PLoS One. 2011;6:e25921.
- Shi C, Cai Y, Li Y, et al. Yap promotes hepatocellular carcinoma metastasis and mobilization via governing cofilin/F-actin/lamellipodium axis by regulation of JNK/Bnip3/SERCA/CaMKII pathways. Redox Biol. 2018;14:59–71.