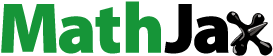
Abstract
Enzymes are powerful versatile biocatalysts, however, industrial application of enzymes is usually hampered by their susceptibility. Bio-inspired Eudragit-α-amylase conjugate (E-AC) was proposed as a biocatalyst for various pharmaceutical and industrial applications. In this study, α -Amylase (E.C. 3.2.1.1) was immobilized by covalent conjugation to Eudragit L-100 under mild conditions. The effect of polymer, carbodiimide and enzyme concentrations on optimization of (E-AC) was investigated. In addition, characterization of the free α -Amylase and E-AC with regard to pH, temperature, kinetic parameters, reusability and operational and storage conditions was carried out. Results showed a shift of the optimum pH of E-AC towards the alkaline side whereas, E-AC exhibited higher thermal stability at all tested temperatures. The kinetic parameters, Km values were 2.87 mg/ml and 3.15 mg/ml and Vmax values were 8.35 mg/ml/min and 8.98 mg/ml/min for free and E-AC, respectively. E-AC retained 85% of the initial activity after five consecutive amylolytic cycles, thus emphasizing its powerful potentials. Operational storage and thermal stability were highly improved as well for E-AC conjugate with an 11.6 stabilization factor in comparison to the free α-amylase. In this study, Eudragit L-100 polymer was successfully used as smart immobilization support to create a reversibly soluble-insoluble enzyme biocatalyst to enforce and extend biotechnological applications of α-amylase in the pharmaceutical industry.
Introduction
α-Amylase (EC 3.2.1.1, 1,4-α-D-Glucan-glucanohydrolase) occupies an important segment (around 25%) of total world enzyme market where it is essential for the conversion of starches into oligosaccharides. Starch is a biodegradable polymer that is extensively used in the food, cosmetic and pharmaceutical industry [Citation1,Citation2]. In fact, the use of amylase enzyme has become a foreseeable successful strategy when a perfect control on reactant and end product are required. A considerable share of the starch processing industry depends on α-amylase for production of maltodextrin, modified starches, or glucose and fructose syrups; and are further extensively used as additives in food and pharmaceutical industry. Advances in biomedical applications have extended the use of starch in nanotechnology for newer biodegradable drug delivery techniques. Hence, sustainable development in the starch processing industry (including gelation, liquefaction or saccharification) primary depends mainly on selective amylolytic activity of α-amylase. α-amylase is also used in therapeutic drug delivery for preparation of digestive aids and is marketed for its anti-inflammatory and anti-eudemons effect. In addition, α-amylase has various applications in biomedical and bioanalytical fields and in a wide range of industries such as food, fermentation, textile, detergent [Citation2,Citation3].
Enzymes are biodegradable biocatalysts that catalyze selective reactions under mild operating conditions. They are cost efficient with the ability to reduce environmental impact unlike conventional chemical processes and they do not produce undesirable waste [Citation4]. However, the industrial applications of enzymes are immensely hindered by high production cost and the inherently sensitive nature of enzymes that compromise their storage shelf life and stability under operational conditions. In addition, enzymes are typically wasted after completion of the catalytic process. Though, regarding their catalytic nature, they still retain their enzymatic activity at the end of the reaction [Citation4,Citation5]. Immobilization is an age-old method of biocatalyst stabilization. Immobilization is a physical or a chemical process in which enzymes are fixed to or confined to a support, creating a heterogeneous immobilized enzyme system that mimics the enzyme natural mode in living cells. Immobilization allows the recovery of enzymes for re-use as biocatalyst which is highly advantageous for industrial applications [Citation4,Citation5]. The choice of the appropriate immobilization support and the efficient immobilization method is fundamental for effective industrial applications of immobilized enzymes [Citation5,Citation6]. In instances where the enzyme has to act on macromolecular substrates (heterogeneous media) it is highly important that the support does not represent a constraint to enzymatic reaction [Citation7].
Several techniques have been reported for immobilization of α-amylases including covalent binding, ionic and hydrophobic interaction, entrapment and aggregation. The use of covalent conjugation on proper support has initiated the biocatalysis of enzymes at nanoscale (nano-enzyme reactors) where the immobilized enzyme structure becomes more rigid and hence more stable. Therefore, multipoint covalent conjugation is considered as one of the most promising techniques for enzyme immobilization [Citation7,Citation8]. α-amylase represent a promising candidate for bioconjugation where highly reactive lysine residues are found on amylase surfaces and are rarely involved in enzyme activity [Citation9]. These lysine groups can be conjugated by reaction with aldehyde, methyl, or carboxyl groups.
Smart polymers (sometimes referred to as intelligent polymers) react to chemical or physical changes in their local environment such as changes in pH, temperature or ionic strength in an expected manner. Smart polymers industry expected to reach US $3.5 billion in 2022 with current and growing industrial applications in the medical, smart textile industry, electronic, aerospace, oil and gas, and automotive industries. Biotechnological applications of smart polymers include tissue engineering; nanocarriers for drug delivery; in medical devices for minimally invasive surgery, in diagnosis, and smart polymers for bioseparation [Citation10].
Eudragit polymers are commercially available enteric methacrylate polymers widely used in the pharmaceutical industry. Their solubility can be controlled by pH modification and therefore are promising candidates as support for designing reversibly soluble-insoluble polymer-enzyme conjugates biocatalysts. Whereby, they are soluble in aqueous solutions with pH values over 5.5 and precipitates below this pH [Citation8,Citation10]. Several authors have efficiently used carbodiimide to covalently couple different enzymes with Eudragit polymers. Their reported results indicated the beneficial significance of Eudragit polymer as a support for the immobilized enzymes such as cellulase [Citation11]; protease [Citation12] L-asparaginase [Citation13]; trypsin [Citation9]; lipase [Citation14]; caseinase [Citation15].
Developing a stable, robust and insoluble biocatalyst with efficient recovery and reuse is one of the major challenges facing industrial biocatalysis. The present study aims to investigate a new smart, mild and reproducible approach for immobilization of α-amylase through covalent bio-conjugation to Eudragit L-100 for stabilization and efficient reuse. To our knowledge, this approach has not been reported before. Optimization of the immobilization process and characterization of the immobilized enzyme with respect to optimum pH, temperature, kinetic parameters, operational and storage stabilities have been studied. This study addresses the need for a reproducible simple tool for α-amylase enzyme immobilization for cost-efficient industrial applications.
Materials and methods
α-Amylase (EC 3.2.1.1, 1,4-α-D-Glucan-glucanohydrolase) (≥1500 units/mg protein A6380), 3,5-dinitrosalicylic acid (DNS), soluble starch potato, Bovine serum albumin (BSA), Carbodiimide hydrochloride, ethanolamine and methacrylic acid-methylmethacrylate anionic copolymer, Eudragit® L-100 was used as a reversible soluble/insoluble carrier for enzyme immobilization were all purchased from Sigma Aldrich Chemical Co., St. Louis, MO, USA. All other chemicals used were of analytical grade.
Amylolytic enzyme assay
The activities of the free and immobilized α-amylase enzyme were assayed according to the Bernfeld method [Citation16] Assay method was based on the absorbance values readings using UV-Visible spectrophotometer (U-1800, Shimadzu, Japan) at 540 nm, at room temperature. The assay of free and immobilized α-amylase included in one ml: 0.1 ml of 1% (w/v) soluble starch as enzyme substrate, 100 mM sodium phosphate buffer (pH 7.2) and 0.1 ml of enzyme sample. The assay mixture was allowed an incubation period for 15 min in a water bath at 37 °C. The reaction was then stopped by the addition of 1 ml of DNS reagent, where it remained in a boiling water bath for 10 min. The reaction mixture was allowed to cool to room temperature and the absorbance of the digested products of the enzymatic reaction (reduced sugars) was measured spectrophotometrically at wavelength 540 nm (A blank was prepared in the same manner without the enzyme). A calibration curve was established with maltose, 0.3–4 (µmol/mL) in 1 ml of deionized water. One activity unit of α-amylase (U) is defined as the amount of the enzyme that liberates 1.0 µmol of reducing sugar/min with maltose as a standard, under standard assay conditions. Residual enzyme activity was calculated as the percentage of activity of immobilized enzyme divided by the activity of the free enzyme. All assay experiments were carried out in triplicate. To eliminate the possible interference of the polymer during protein determination, standard solutions were prepared ((BSA) and BSA immobilized on Eudragit) and the absorbance was determined at 280 nm. The protein absorbance values indicated no significant changes in the presence of the polymer.
Immobilization onto Eudragit L-100
α-amylase was covalently immobilized on Eudragit L-100 polymer using carbodiimide as cross-linking agent [Citation17,Citation18]. For the optimization of the immobilization process and to attain maximum enzyme-conjugate activity, the polymer concentration, carbodiimide concentration, enzyme percent and immobilization time were studied. Briefly, various concentrations of Eudragit L-100 solution (0.5– 3% (w/v)) were prepared in phosphate buffer pH 7.2. To guarantee complete dissolution, the NaOH solution was used to raise the pH to 11.3. Afterwards, pH was reduced to 7.2 using HCl solution and carbodiimide coupling agent (0.25% (w/v) solution) was added to the prepared Eudragit L-100 solution with continuous stirring for 20 min, followed by, adding 0.05% (v/v) of ethanolamine solution (0.45 g/ml) with stirring for 1 h at room temperature. Subsequently, different concentrations of α-amylase (10– 40 µg/ml) solutions were added at room temperature (25 °C) and kept under stirring for varying immobilization time. The mixture solution pH was lowered to 4.5 with 2 M acetic acid to precipitate Eudragit-amylase conjugate (E-AC). The precipitate was separated by centrifugation (15,000 × g, 15 min) and washed three times using 0.01 M acetate buffer (pH 4.5) for 10 min until the supernatant exhibit no enzyme activity. Repeated washing was additionally performed using 0.02 M phosphate buffer (pH 7.2) and re-precipitated with an acetic acid solution (pH 4.5). Finally, E-AC was redissolved in 100 ml 0.3 M Tris–HCl buffer of pH 7.4 for further investigation [Citation17,Citation18].
Immobilization yield %
The immobilization yield was calculated as the enzyme yield which was immobilized by covalent conjugation onto Eudragit polymer and calculated by the following equation.
(1)
(1)
Where X is the initial activity of free α-amylase added, and Y is the activity of α-amylase in the supernatant. Both X and Y amylolytic activities were assayed under standard assay conditions.
Characterization of the immobilized α-amylase conjugate (E-AC)
pH profile
Rate of hydrolysis of the starch solution was evaluated at various pH values to obtain the effect of pH on α-amylase activity on the enzymatic activity of free α-amylase or E-AC. Enzyme activity was determined under standard assay conditions. In the case of amylase, 0.5 ml of starch solution was taken as substrate and reaction volume was adjusted with 0.5 ml buffer of different pH (3.0–10.0). in order to acquire different pH conditions the following buffers were used, 0.05 M Glycine–HCl (3.0–4.0), acetate (4.0–5.0), succinate (6.0–6.5), potassium phosphate (6.5–8.0), Tris–HCl (7.5–9.0).
Temperature profile
In order to evaluate the effect of immobilization on α-amylase stability, the activity of free and immobilized α-amylase was determined at various temperatures from 20–80 °C in 20 mM Tris, pH 7.2. The residual enzyme activity was determined under standard assay conditions.
Kinetic parameters
The kinetic parameters of α-amylase activation Vmax, and Km for free α-amylase or E-Ac were calculated using starch as substrate standard activity assay. The amylolytic activity assay was performed using different substrate concentrations from 2 to 15 mM. The kinetic parameters Km and Vmax were determined by plotting Lineweaver–Burk plots using the graph pad prism program (version 5.0, GraphPad, San Diego, CA). The catalytic efficiency (Vmax/Km) for both free α-amylase and E-Ac was also calculated.
Recycling and reusability
The reuse capability of the immobilized α-amylase was measured by subjecting it to five consecutive amylolytic cycles. One milliliter of 1% (w/v) starch in 20 mM phosphate buffer (pH = 7.2) was added to the immobilized enzyme and incubated for 5 min under constant shaking for each cycle. At the end of each cycle, E-AC was recovered by lowering the pH to 4.5, centrifugation, alternative washing of the precipitated polymer with acetate buffer pH 4.5 and phosphate buffer pH 7.2 and re-dissolution in Tris–HCl buffer, pH 7.4 and then a fresh substrate solution was added to start a new cycle. The supernatant was assayed after each cycle for residual activity under standard assay conditions.
Determination of operational and thermal stability
For operational stability, the free α-amylase or E-Ac was tested at a different temperature under stress conditions. Enzyme activity was determined by incubating the enzyme samples for 120 min at various temperatures at 5, 25, 37 and 60 °C stirring at 120 rpm of. Enzyme activity was determined at regular time intervals of 30 min. The residual enzyme activity was measured at 37 °C under standard assay conditions. The stabilization factor (SF) was calculated as the ratio of the half-life of the immobilized enzyme in relation to that of free enzyme.
Evaluation of storage stability
The activity of free α-amylase (in 50 mM potassium phosphate buffer, pH 7.2) or E-AC stored at 5 °C and at 25 °C in tightly closed Eppendorf® tubes for 60 d was examined. Samples were tested after 15, 30 and 60 d post storage under standard assay conditions. The enzyme activity at zero time was taken as 100% activity and the percentage of residual catalytic activity was calculated for either free α-amylase or E-AC.
Statistical analysis
Experimental data were expressed as the mean ± SD. A statistical representation of data was performed using GraphPad Prism software (version 5.0, GraphPad, San Diego, CA).
Results and discussion
Optimization of immobilization conditions
Immobilizing α-amylase enzyme through covalent conjugation to the soluble matrix can overcome some of the operational limitations faced during industrial application making the process simply controlled and cost-efficient.
Effect of Eudragit L-100 polymer concentration
Immobilization support plays a major role in the performance of the bioconjugate system. α-amylase was immobilized onto Eudragit L-100 and the effect of polymer concentration was tested at varying amounts from 0.5 to 3% (w/v) and activation with 0.25% (w/v) carbodiimide for 20 min. The Eudragit concentration had a pronounced effect on the enzyme-conjugate activity whereas; it increased with the increase in polymer concentration. Optimum concentration was achieved at 2% (w/v) polymer concentration with enzyme immobilization yield of 85%. Higher polymer concentration resulted in reduced specific amylolytic activity and immobilization yield (. The decrease in specific amylolytic activity can be attributed to steric hindrance of the immobilized protein molecules at higher polymer concentration. This crowdedness effect was previously observed by several authors, where this reduction in enzyme activity was attributed to intermolecular binding at higher Eudragit concentrations or due to enzyme denaturation [Citation17–19].
Effect of carbodiimide coupling agent
2% (w/v) Eudragit polymer solution was prepared and 20 µg/ml α-amylase was immobilized in the presence and absence of carbodiimide as a coupling agent. Non-covalent immobilization was dominant in the absence of carbodiimide, this resulted in low immobilization yield (27%) and lower enzyme-conjugate activity. On the other hand, upon the conjugation in the presence of carbodiimide at 0.25% (w/v) the immobilization yield improved up to 85%. Similar results were reported before for noncovalent conjugation [Citation18].
Effect of enzyme concentration
To optimize conjugation parameters it was essential to find out the optimum required enzyme concentration. Eudragit polymer solution (2% w/v) was activated with 0.25% (w/v) carbodiimide for 20 min and varying concentration of amylase from 10 to 40 µg/ml was used for coupling of E-AC. shows the effect of enzyme concentration on immobilization yield. Maximum yield was attained at 20 µg/ml (equivalent to 30 U). where increasing the enzyme concentration further caused no increase in determined conjugate activity. The reduced amylolytic activity at higher enzyme concentrations is a result of polymer oversaturation which leads to clustering and eventually intermolecular steric hindrance that would restrain the diffusion of the substrate and product. Similar observations were reported by several authors where a reduction in enzyme immobilization yield occurs beyond the optimum concentration including protease [Citation17], esperase [Citation20], cellulose [Citation21] and catalase [Citation22]. Thus, the 20 µg/ml α-amylase was chosen as the optimum concentration for further immobilization studies.
Effect of immobilization on pH profile of α-amylase
The effect of pH on amylolytic activity of α-amylase free enzyme and Eudragit-amylase conjugate was determined at various pH values ranging from pH 3.0 to pH 10.0 (. Maximum amylolytic activity for the free enzyme was observed at pH 6. The optimal pH of α- amylase was shifted to pH 7.0 upon immobilization. In addition, as shown in , E-AC exhibited higher amylolytic activity than the free enzyme at all measured pH values above pH 7. It is expected from the anionic nature of Eudragit L-100 polymer that the solubility increases above pH 5.5 which resulted in a shift of the optimum pH of the enzyme conjugate to the alkaline side. Similar results indicating a shift in optimum pH towards the alkaline side were reported before for different enzymes upon using Eudragit polymers such as protease [Citation17], cellucast/Eudragit conjugate [Citation18], chemotrypsin [Citation23] and cellulase [Citation24,Citation25].
Effect of immobilization on temperature profile of α-amylase
To examine the effect of temperature on the free and immobilized α-amylase stability, amylolytic activity was assayed at varying temperatures from 20–80 °C (. Both free and immobilized α-amylase exhibited amylolytic activity over a wide range of temperature however, optimum temperature was observed at 50 and 60 °C for free and immobilized enzyme, respectively. A significant shift in the optimum temperature of α-amylase upon immobilization to a higher temperature was recorded. For free α-amylase a sharp decrease in activity was recorded above 60 °C. on the other hand, the immobilized α-amylase retained 61% residual activity at 70 °C (. Remarkably, E-AC exhibited higher activity than the free enzyme at all measured temperatures and hence higher thermal stability. Improvement of α-amylase enzyme thermal stability upon immobilization has been reported before [Citation26]. The change in thermal stability upon immobilization is brought about by formation of multipoint covalent bonds via amino groups with the conjugation polymer. This strong rigidification limits the enzyme’s freedom to undergo conformational changes and hence lose its activity. The increase in optimum temperature of enzymes upon immobilization has been previously reported by several authors [Citation8,Citation27–29]. Loss of enzyme activity at higher temperatures is very common for enzymes where, as the temperature rises, thermal inactivation of proteins structure occur accompanied by loss of conformational structure and/or hydrolysis of the peptide chain and aggregation [Citation27,Citation28].
Determination of kinetic parameters
Data about the possible interaction between the enzyme and its immobilization support can often be figured out by comparing between the kinetic parameters of the free and the immobilized enzyme. The Michaelis–Menten parameters (Km and Vmax) for free and immobilized α-amylase were determined by Lineweaver–Burk plot using starch as substrate. presents a comparative data of the kinetic parameters and catalytic efficiencies for free and immobilized α-amylase.
Table 1. Kinetic parameters (Km, Vmax) and amylolytic efficiency of free and bio-conjugated α-amylase enzyme (E-AC).
The Km value slightly increased upon immobilization (from 2.87 to 3.15 mg/ml) while Vmax values marginally increased upon immobilization (from 8.35 to 8.98 mg/ml/min). An increase in Km upon immobilization indicate that the immobilized enzyme exhibit an apparent lower affinity for its substrate than the free one. The increase in Vmax indicates that the rate of amylolytic reaction has slightly increased upon immobilization. The Increase in Km value upon immobilization has been reported before for protease, catalase and α-amylase upon using various soluble or insoluble supports including smart polymers [Citation17,Citation22,Citation28,Citation29]. Change in substrate affinity could occur upon immobilization due to steric hindrance of the active site by the support which hinders its access to the substrate; especially for large substrates such as starch [Citation17,Citation21,Citation27]. Kinetic parameters study results implies that the conjugation conditions employed did not have a negative effect on the enzyme specific activity.
Reusability of the conjugted α-amylase enzyme
Economical industrial application of enzymes demands reusability of the immobilized enzyme. In this work, immobilized α-amylase (E-AC) was tested for 5 repeated amylolytic cycles (. Results showed that E-AC exhibited high reusability against repeated decomposition of starch without significant loss in activity; regardless of the significant lowering of the pH that is required for recovery of the enzyme after each cycle. The E-AC conjugate retained 85% residual activity after 5 consecutive amylolytic cycles (. Immobilization causes a steric restriction that induces stabilization whereby it restrains the formation of an inactive form of α-amylase. Similar results were reported by other authors for α-amylase [Citation30], β-galactosidase [Citation31] and lipase [Citation14] enzymes where improved recovery and reuse was recorded upon using the polymeric matrix for immobilization.
Determination of operational and thermal stability
Thermal stability of enzymes is a demand for successful industrial application of these biocatalysts in various fields. Hence, the effect of immobilization of α-amylase on its thermal stability was studied. presents the operational and thermal stability of the free and immobilized α-amylase at 5, 25, 37 and 60 °C. It is interesting to find that there was a significant improvement in the thermal stability of E-AC conjugate in comparison to the free amylase with a considerable stabilization factor between a low of 6.8 and a high of 11.6.
Table 2. Half-life times (t1/2) for the free and immobilized (E-CA) α-amylase at different temperatures.
α-Amylase showed good stability at 5 °C with t1/2 of 100 d and exhibited a 6.8 fold increase in t1/2 after immobilization. At 25 °C free α-amylase exhibited less stability with t1/2 of 6 d however, E-AC showed better stability with 7.5 stabilization factor. The lifetime for the free and immobilized α-amylase was examined at 37 °C, the E-AC conjugate had higher stability with a stabilization factor of 9.3. Immobilization of α-amylase by conjugation onto Eudragit polymer generously improved its thermal stability at 60 °C in comparison with free enzyme where it retained 97% of its initial activity with t1/2 of 8 h. Results showed that α-amylase achieved better thermal and operational stability upon covalent conjugation with Eudragit polymer. It has been previously reported that enzyme immobilization induces an increase in enzyme rigidity, which is commonly revealed through improved stability towards thermal denaturation at higher temperatures [Citation27]. Improvement of thermal stability has been reported before for protease [Citation17], catalase [Citation22] and α-amylase [Citation28,Citation32]. These findings showed improved α-amylase operational and thermal stability which would widen and enhance the possible applications of α-amylase in processes that demand elevated temperature.
Shelf life stability
The success in developing an efficient immobilized enzyme system relies on the chosen enzyme support which is generally considered as the most crucial component. The stability of free and immobilized (E-AC) α-amylase was examined at 5 and 25 °C (. At 5 and 25 °C, the E-AC retained the initial specific activity for 15 d. As represented in , free α-amylase retained 50 and 37% of its initial activity after 60 d of storage at 5 and 25 °C, respectively, whereas, the immobilized enzyme retained 90 and 69% residual activity after 2 months storage at 5 and 25 °C, respectively. Improvement of storage stability of different enzymes upon immobilization has been observed before by several authors [Citation33–35]. In conclusion, stability results demonstrated the advantage of covalent conjugation of α-amylase onto Eudragit L-100 polymer whereas conjugation resulted in improved shelf life stability.
Conclusion
The ultimate goal of a successful enzyme immobilization approach is to achieve high stability without compromising specific activity with the possibility for recovery and reuse. In this study, α-amylase was successfully covalently immobilized onto smart stimuli-responsive Eudragit L-100 polymer to prepare a soluble-reversible insoluble biocatalyst. Results demonstrated the efficiency of the chosen immobilization process in improving the thermal, storage and operational stability while preserving enzyme specific activity.
Meanwhile, the stimuli-responsive polymer provided a smart simple method for recovery and reuse of α- amylase. It is worth noting that the polymeric Eudragit matrix used for α- amylase immobilization is water soluble, commercially available, non-toxic (enteric polymer), recoverable from solution by lowering pH.
These results are of significant importance for the industrial and biotechnological application of α- amylase considering the fact that the major obstacle is usually the loss of enzymatic activity and catalytic efficiency. In conclusion, it is suggested that α- amylase, in the presented immobilized form (E-AC), is a promising candidate for application in the pharmaceutical industry. Deep investigations with an emphasis on starch processing shall be further conducted.
Disclosure statement
No potential conflict of interest was reported by the authors.
References
- Rodrigues A, Emeje M. Recent applications of starch derivatives in nanodrug delivery. Carbohyd Polym. 2012;87:987–994.
- Mohamed S, Al-Harbi M, Almulaiky Y, et al. Immobilization of Trichoderma harzianum α-amylase on PPyAgNp/Fe3O4-nanocomposite: chemical and physical properties. Artif Cells Nanomed Biotechn. 2018;46:201–206.
- Abdel-Mageed H, Fouad S, Teaima M, et al. Optimization of nano spray drying parameters for production of α-amylase nanopowder for biotheraputic applications using factorial design. Drying Technol. 2019.
- Homaei AA, Sariri R, Vianello F, et al. Enzyme immobilization: an update. J Chem Biol. 2013;6:185–205.
- Cooney MJ. Kinetic measurements for enzyme immobilization. Meth Mol Biol. 2017;1504:215–232.
- Knežević Ž, Grbavčić RB, Stefanović I, et al. Covalent immobilization of enzymes on Eupergit® supports: effect of the immobilization protocol. In: Minteer S, editor. Enzyme stabilization and immobilization. Methods in molecular biology, Vol 1504. New York (NY): Humana Press; 2017.
- Li X, Yu Z, Bian Z, et al. Physiochemical characterization of α-amylase as cross linked enzyme aggregates. Catalysts 2018;8:299.
- Kumar A, Gupta MN. Immobilization of trypsin on an enteric polymer Eudragit S-100 for the biocatalysis of macromolecular substrate. J Mol Catal B: Enzym. 1998;5:289–294.
- Silva C, Sousa F, Gübitz G, et al. Chemical modifications on proteins using glutaraldehyde. Food Technol Biotechnol. 2004. p. 51–56.
- Majid H, Hamdy MAS, editors. Industrial applications for intelligent polymers and coatings. Cham, Switzerland: Springer International Publishing; 2016. p. 973–978.
- Yu Y, Yuan J, Wang Q, et al. Noncovalent immobilization of cellulases using the reversibly soluble polymers for biopolishing of cotton fabric. Biotechnol Appl Biochem. 2015;62:494–501.
- Smith E, Schroeder M, Guebitz G, et al. Covalent bonding of protease to different sized enteric polymers and their potential use in wool processing. Enz Microb Technol. 2010;47:105–111.
- Qiao J, Jiang J, Liu L, et al. Enzyme reactor based on reversible pH-controlled catalytic polymer porous membrane. ACS Appl Mater Interf. 2019;11:15133–15141.
- Knežević Z, Milosavić N, Bezbradica D. Immobilization of lipase from Candida rugosa on Eupergit® C supports by covalent attachment. Biochem Eng J. 2006;30:269–278.
- Ahmed S, Abdel Wahab W, Abdel-Hameed S. Comparative study in kinetics and thermodynamic characteristics of immobilized caseinase on novel support from basalt by physical adsorption and covalent binding. Biocatal Agric Biotechnol. 2019;18:101028.
- Bernfeld PA. Amylase α and β. Meth Enzymol. 1955;1:149–151.
- Silva M, Zhang Q, Shen J, et al. Immobilization of proteases with a water soluble-insoluble reversible polymer for treatment of wool. Enz Microb Technol. 2006;39:634–640.
- Dourado F, Bastos M, Mota M, et al. Studies on the properties of Celluclast/Eudragit L-100 conjugate F. J Biotechnol. 2002;99:121–131.
- Madhu A, Chakraborty JN. Recovery and reuse of immobilized α-amylase during desizing of cotton fabric. Textile and Apparel. 2018;22:271–290.
- Smith E, Zhang Q, Shen J, et al. Modification of esperase by covalent bonding to Eudragit polymers L 100 and S 100 for wool fiber surface treatment. Biocat Biotrans. 2008;26:391–398.
- Zhou J. Immobilization of cellulase on a reversibly soluble-insoluble support: properties and application. J Agric Food Chem. 2010;58:6741–6746.
- Abdel-Mageed H, Fahmy AS. Shaker DS, et al. Development of novel delivery system for nanoencapsulation of catalase: formulation, characterization, and in vivo evaluation using oxidative skin injury model. Artif Cells Nanomed Biotechnol. 2018;24:362–371.
- Sharma S, Kaur P, Jain A, et al. A smart bioconjugate of chymotrypsin. Biomacromolecules 2003;4:330–336.
- Yu Y, Yuan J, Wang Q. Covalent immobilization of cellulases onto a water-soluble–insoluble reversible polymer. Appl Biochem Biotechnol. 2012;166:1433.
- Yu Y, Yuan J, Wang Q, et al. Cellulase immobilization onto the reversibly soluble methacrylate copolymer for denim washing. Carbohyd Polym. 2013;95:675–680.
- Chang M-Y, Juang R-S. Activities, stabilities, and reaction kinetics of three free and chitosan–clay composite immobilized enzymes. Enz Microb Technol. 2005;36:75–82.
- Rodrigues RC, Ortiz C, Berenguer-Murcia Á, et al. Modifying enzyme activity and selectivity by immobilization. Chem Soc Rev. 2013;42:6290–6307.
- Homaei A, Saberi D. Immobilization of α-amylase on gold nanorods: an ideal system forstarch processing. Process Biochem. 2015;50:1394–1399.
- Tavano OL, Fernandez-Lafuente R, Goulart AJ, et al. Optimization of the immobilization of sweet potato amylase using glutaraldehyde-agarose support characterization of the immobilized enzyme. Process Biochem. 2013;48:1054–1058.
- Jaiswal N, Prakash O. Immobilization of soybean α-amylase on gelatin and its application as a detergent additive. Asian J Biochem. 2011;6:337–346.
- Lee J, Park I, Cho J. Immobilization of the Antarctic Bacillus sp. LX-1 β-Galactosidase on Eudragit L-100 for the production of a functional feed additive. Asian Australas J Anim Sci. 2013;26:552–557.
- Talekar S, Chavare S. Optimization of immobilization of α-amylase in alginate gel and its comparative biochemical studies with free α-amylase. Rec Res Sci Technol. 2012;4:1–5.
- Mohamed S, Abdel-Mageed H, Tayel S, et al. Characterization of Mucor racemosus lipase with potential application for the treatment of cellulite. Process Biochem. 2011;46:642–648.
- Arasaratnam V, Galaev IY, Mattiasson B. Reversibly soluble biocatalyst: optimization of trypsin coupling to Eudragit S-100 and biocatalyst activity in soluble and precipitated forms. Enzyme Microb Technol. 2000;27:254–263.
- Godoy CA. New strategy for the immobilization of lipases on glyoxyl–agarose supports: production of robust biocatalysts for natural oil transformation. IJMS. 2017;18:2130.