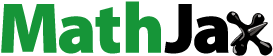
Abstract
Antinutritional factors (ANFs) are the most found chemical in legumes, including beans. This study was done to evaluate the effects of processing techniques on the anti-nutritional factors of common beans. Soaking in water alone, and soaking in sodium bicarbonate (0.05 %) solutions (1:3 in both cases), germination for 24- and 48-hours (at 25 °C), and conventional and pressure cooking, were applied. The dried and ground processed beans were subjected to analysis for phytate and tannin (vanillin-HCl, Burns). The ANFs were significantly affected by each treatment (P < 0.05). Soaking the beans in water and sodium bicarbonate showed a reduction in phytate content of 17.79% and 27.32 %, respectively. The reduction in phytate content by 22.57% for germination of 24 hours and 34.95 % for 48 hours at 25 °C was achieved. The amount of phytate reduced by conventional cooking and pressure cooking was 33.29 % and 35.21 %, respectively. It was also illustrated that the soaking reduced the tannin content by 46.88 % for soaking in water and 54.76 % for soaking in sodium bicarbonate. Reductions in tannin content of 59.44% and 66.69% were obtained by 24-hour and 48-hour germination, respectively, while ordinary and pressure cooking showed tannin reductions of 69.81 % and 77.69 % respectively. Pressure cooking showed the highest reduction in the ANFs and greatly affected the phytate and tannin content, followed by ordinary cooking and germination for 48 hours. It was concluded that pressure cooking shows the highest reduction in phytate and tannin contents.
PUBLIC INTEREST STATEMENT
Micronutrient malnutrition remains a serious public health problem in developing countries. The nutrient bioavailability is a concern when relying on plant-based foods. Common beans are important food and nutrition-security legumes that provide protein, minerals, and vitamins. Processing methods play a significant role in reducing antinutritional factors and enhancing digestion and nutrient bioavailability. Germination activates the phytase enzyme and degrades the phytate naturally found in beans. The cooking and germination results in decreased antinutritional factors and enhanced mineral bio-accessibility in beans. Pressure cooking showed the highest reduction in the ANFs and greatly affected the phytate and tannin content, followed by ordinary cooking and germination for 48 hours. It is concluded that pressure cooking shows the highest reduction in phytate and tannin contents.
1. Introduction
Antinutritional factors (ANFs) are the most commonly found chemical ingredients in legumes, including beans. They mostly form complexes with positively charged minerals that render them inaccessible for absorption in the colon, interfering with digestion and nutrient absorption after consumption. Thus, these chemicals are indirectly contributing to the increase in malnutrition in most developing countries. Micronutrient deficiencies in societies that rely on legumes as staple foods, particularly a bean-based diet, are a problem highly related to the effects of these antinutritional factors. Low consumption of animal products with high levels of dietary iron and zinc that are highly bio-accessible has led to widespread iron and zinc deficiency in low-income countries. The consumption of cereals and legumes, which are high in antinutritional factors, is common in developing regions due to the unaffordability of animal products (Canani et al., Citation2005; Sue Horton & R, Citation2008). Common beans (Phaseolus vulgaris L.), one of the legume crops that offer a sufficient amount of macro- and micronutrients, are grown and consumed in large quantities throughout Africa, including Mozambique and Ethiopia. The most widely used legume that is grown specifically for human consumption is the common bean (Broughton et al., Citation2003; Porch et al., Citation2013). Beans are a mainstay diet and the main iron source for people in Latin America and Eastern Africa.
However, due to inhibitory chemicals, they exhibit limited iron absorption (Petry et al., Citation2015). The dried seeds of common beans, which are lower in methionine and cysteine but higher in tryptophan and lysine and a good source of the minerals iron, copper, and zinc, are a significant source of dietary protein for millions of people throughout the tropics (Jones, Citation1999; Wortmann et al., Citation2006). Besides, common beans are nutritious foods low in fat and high in protein. They are a significant component of many traditional diets, particularly vegetarian diets, which are consumed with different dishes and other food products in different countries (Lovato et al., Citation2018; Suliburska & Krejpcio, Citation2014). Bean consumption is linked to a decline in some cancer types, a reduction in the frequency of degenerative diseases, and a decline in conditions including diabetes, cardiovascular disease, and even neoplasms (Machado et al., Citation2008; Rocha-Guzmán et al., Citation2007).
Endogenous anti-nutritional agents present in beans significantly impact the nutritional value, digestion, and absorption of nutrients. The main anti-nutritional components of beans are phytates and tannins, the latter of which has a propensity to precipitate proteins, and the former can form insoluble complexes with proteins and minerals, reducing the bioavailability of these crucial nutrients in the human diet. Protein digestion and mineral absorption are inhibited by anti-nutritional factors (Mumtaz Hamdani & Ahmed Wani, Citation2017). It has been illustrated that legume utilization is limited due to the presence of these ANFs, and negative consequences are seen after consuming them raw, overshadowing the nutritious benefits (Rahate et al., Citation2021). The quantity of ANFs consumed with food varies depending on how frequently a particular food is consumed. Currently, there is no regulation that limits the recommended safe limit for ANF intake in Ethiopia. For phytic acid, there is no Recommended Dietary Allowance (RDA). With about 250–800 mg of phytate per day, the average Western diet is comparatively low in phytate. People in developing nations who consume mostly cereals and legumes may consume up to 2,000 mg of phytate per day (Frølich, Citation2011).
The role of traditional or improved processing techniques in reducing ANFs and improving the sensory quality of beans has been well-reviewed (Banti & Bajo, Citation2020). Conventional processing methods are important because they are affordable and easily used by rural communities. They can be used as a comparison and as a reference point in conducting scientific experiments. The beans are cooked before their consumption, and most consumers prepare food at the household level. According to Rahate et al. (Citation2021), the advancement in food technologies, including thermal processing, fermentation, and fortification, resulted in a reduction of ANFs in faba beans. Through physicochemical and structural changes to the food matrix, roasting and other heat treatments can increase the digestibility, palatability, and bioavailability of food products (Sruthi et al., Citation2021). It was also reported in previous studies that different processing techniques, including soaking, cooking, germination, fermentation, and enzymatic treatments, can contribute to the bioavailability of nutrients, especially minerals and proteins, improve sensory properties, and reduce anti-nutritional factors in beans (Fabbri & Crosby, Citation2016; Tanasković et al., Citation2021). According to Matella et al. (Citation2005), soaking and germination can increase the utilization of kidney beans. It has also been reported that extrusion cooking, milling, dehulling, and fermentation are mainly used for reducing antinutritional factors in pulses (Kumar et al., Citation2022).
Current research carried out in Mozambique uses the small black common bean variety (A222) released by the Instituto de Investigacao Agraria de Mozambique. Beans are a staple in many southern African recipes, particularly those from Mozambique. Since beans have a good nutrient profile, particularly in terms of protein, it helps to minimize the issue of malnutrition in the area. Notwithstanding the large consumption of beans in the region, there are still problems with the bioavailability of minerals and proteins due to the presence of ANFs, which decrease nutrient digestion, solubility, and absorption. Considering this fact, it is important to employ and assess various processing methods to reduce ANFs in beans in order to improve nutrient bioavailability.
Furthermore, the ANF profile in the released beans was also not studied. Therefore, the current research is carried out to evaluate how processing techniques affect the ANF contents of common beans. This study achieved reduced phytate and tannin content in beans as a function of different processing techniques. It has also been reported by several researchers that processing methods affect food matrix and reduce antinutritional factors (Abioye et al., Citation2018; Hauwa Hajjagana et al., Citation2014; Minuye et al., Citation2021; Pal et al., Citation2017). This could help reduce protein-deficient malnutrition. The result of this study is a crucial input for bean-consuming developing countries, especially in the eastern and southern parts of Africa. The investigated processing techniques can easily be practiced in rural communities, except for pressure cooking. Beans are widely consumed with different dishes in Mozambique, and the bean processing technology which reduces ANFs and enhances nutrient bioavailability should be disseminated.
2. Material and methods
2.1. Study area
The laboratory experimental work was performed at three faculties at Eduardo Mondlane University, including the chemical engineering (food technology) laboratory, the microbiology laboratory, and the department of chemistry (central laboratory) in the faculties of Engineering, Veterinary medicine, and Sciences, respectively. The site is in Mozambique, in Maputo. The area is located on the Southeast coast of Africa at 18.6677 ° S and 35.5296 ° E.
3. Materials
3.1. Chemicals and reagents
The laboratory analysis was conducted using biological and analytical-grade reagents. The reagents used were catechin hydrate (C1251-SIGMA ALDRICH, USA), Phytic acid (MKCB0619V-Sigma Aldrich, St. Louis, MO 63,103, USA), Vanillin (V5000-MINEMA, South Africa), ferric chloride hexahydrate (F1520-MINEMA, South Africa), Hydrochloric acid (AL2443-Alpha Chemika, India), Methanol (BIOCHEM chemopharma, ZA Cosne Sur Loire 58,200, France), and Sodium Bicarbonate.
3.2. Sources, collection, and preparation of research materials
The mature, undamaged, and defect-free black small common bean samples (bean variety A222) were collected from the Institute of Agricultural Research of Mozambique (IIAM). The bean samples were cultivated in the 2020 cropping season and harvested in December 2020. About 5 kg of the collected bean samples were sorted for sands, husks, soils, and any foreign materials and kept at room temperature prior to applying soaking, cooking, and germination treatments. Raw beans (the control sample) were milled using a laboratory mill (ZM200-Retsch Rheinische Straße 3,642,781 Haan, Germany) and stored at ambient temperature prior to further analysis.
3.3. Treatment’s setup
The collected beans were subjected to three distinct processing techniques in two stages. The processing methods studied in the experiment were soaking (water and NaHCO3), cooking previously soaked beans in water (ordinary and pressure cooking), and germination (24 and 48 hours). Sodium bicarbonate solution degrades antinutritional factors through hydrolysis. The alkaline nature of sodium bicarbonates creates a conducive environment that promote the hydrolysis of phytate. The processing techniques were purposefully selected based on their simplicity and affordability by considering rural society so that they can be applied easily. Based on the results of earlier investigations, the levels for each treatment were chosen. For instance, the maximum germination time of 48 hours was adopted because, as the germination time increased, the nutritional content of the beans would be entirely utilized to nourish the growing embryo.
4. Methods
4.1. Physico-chemical parameter analysis
4.1.1. Determination of moisture content
The empty petri dishes were placed in an oven set at 105 degrees Celsius for one hour, dried there for 30 minutes, and then weighed. Fresh samples weighing about 2.00 g were placed in the petri dishes that had been dried and weighed earlier. The plates and their contents were put in the drying oven, where they dried for an hour at 105 degrees Celsius. The petri dishes and their contents were then removed from the oven after drying, cooled in desiccators, and reweighed. When a sample is dried to a constant weight at a temperature close to the boiling point of water, the amount of water present is assumed to be equal to the weight loss (AOAC 925.10).
4.2. Determination of ash content
The content of ash in the samples was determined by burning known weights of the samples at 550°C in a muffle furnace until a white ash was produced. The leftover inorganic material was cooled and weighed after the organic stuff was burned off. In a muffle furnace, heating was done in stages: first to extract the water, then to properly char the product, and finally to ash at 550 °C. The porcelain ash dishes were heated to 550 °C in a muffle furnace for 30 minutes. The dishes were taken out and allowed to cool in desiccators for around 30 minutes at room temperature. Each dish was then weighed to the nearest gram. The amount of flour added to each dish was about 4 g. The plates were set on a hot plate in a fume hood, and the temperature was gradually raised until the samples were totally charred and the smoking stopped. The dishes were heated to 550 °C in a muffle furnace for 18 hours, then taken out and allowed to cool for 1 hour in desiccators. The ash appeared to be tidy and white. Each dish, including the ash, was reweighed once it had reached room temperature. The difference was used to compute the total ash weight, which was then reported as a percentage of the fresh sample.
4.2.1. Hundred seed weight
The pure, free of defect, 100 dry beans seeds were randomly taken and weighed with sensitive balance. Triplicate readings of one hundred seed weights per one hundred grams of seeds were taken, and the average value of the readings was recorded (Balasubramanian et al., Citation1999).
4.2.2. Non-soakers beans
400 bean seeds were taken at random and immersed in water (1:3 beans to water) for 16 hours (overnight). The bean was blotted to remove any remaining water after the soaking water was discarded. The non-soakers were hand-selected, counted, and the percentage value calculated.
4.3. Processing of Beans
The raw bean samples were sorted, cleaned, and ground using a miller (ZM200-Retsch Rheinische Straße 3,642,781 Haan, Germany) to use as a control sample for anti-nutritional, mineral analysis, and in vitro digestion. The remaining bean samples were processed using three processing methods: soaking, germination, and cooking. Soaking, germination, and cooking were performed following the methods of Emire and Rakshit (Citation2007), (Kaur and Kapoor (Citation1990), and Naozuka and Oliveira (Citation2012) for pressure cooking. The pH of water and sodium bicarbonate (NaHCO3) solution before and after processing was measured by a PH meter during the conduct of an experiment (Figure ).
4.4. Soaking
The cleaned common bean seed, free of broken seeds and other foreign materials, was steeped for 12 hours at room temperature in distilled water and a 0.05 % NaHCO3 solution at a ratio of 1:3. The pH of the water and sodium bicarbonate solutions were 7.2 and 8.84, respectively. The soaked seeds were rinsed with distilled water after being washed, and they were then dried in a 60 °C oven. For further investigation, the dried samples were powdered and preserved in vacuum plastic. The cleaned common bean seed, free of broken seeds and other foreign materials, was steeped for 12 hours at room temperature in distilled water and a 0.05 percent NaHCO3 solution at a ratio of 1:3 (Figure ).
4.5. Germination
The common beans were weighed (120 g) and placed in a plastic bowl. They were then covered and allowed to soak in distilled water (1:5 w/v beans to water) for 12 hours at room temperature. The seeds were then placed in an incubator set at 25 °C for 24 and 48 hours to allow them to germinate, covered in aluminum foil and muslin. During the germination stage, distilled water was sprinkled twice daily on the seeds. The germinated beans were dried and powdered, then stored in vacuum plastic bags for later examination after being pulverized in a sample mill and dried in a hot air oven at 60 °C (Figure ).
4.6. Cooking
The previously soaked beans were cooked in a typical pan with a seed-to-water ratio of 1:6 at 100 °C for 1.47 hours (regular cooking) and 100 °C for 38 minutes (pressure cooker) after soaking in tap water for 12 hours. The cooked samples were manually mashed with a pestle and mortar, then dried in a hot air oven with the temperature set at 60 °C, milled into a fine powder, and then kept for later analysis in vacuum plastic bags.
4.7. Antinutritional contents
4.7.1. Phytate
The phytate content of the bean was determined by the method of Latta and Eskin (Citation1980)and later refined by Vantraub and Lapteva (Citation1988). A sample of bean flour weighing about 0.1 g was centrifuged at 3000 rpm for 30 minutes after being extracted with 10 ml of 2.4% HCl (v/v) in a mechanical shaker for 1 hour at room temperature. After centrifugation, the clear supernatant was collected and utilized to measure the amount of phytate. Three milliliters of the supernatant (sample solution) were combined with two milliliters of wading reagent, which was created by combining a 0.03 percent solution of FeCl3.6H2O with a 0.3 % solution of 1N HCl (v/v) in deionized water. The mixture was then centrifuged (HERAEUS MEGAFUGE 8 Centrifuge 721,113,070,119, China) at 3000 rpm for ten minutes. Sample solutions and blank absorbances were read by a UV-VIS spectrophotometer (Agilent Technologies No. G6860A, Malaysia) at 500 nm.
4.7.2. Preparation of phytic acid standard
The phytic acid working standard solutions comprising 5–40 mg/ml phytic acid in water were made from stock solutions to produce the calibration curve. Three milliliters of the standards and three milliliters of deionized water (used as a zero level) were pipetted into 15-mL centrifuge tubes. After that, 1 ml of the wading reagent was added to each tube, and the solution was blended for 5 seconds with a vortex mixer. The absorbance of the solutions was then measured with a UV-VIS spectrophotometer at 500 nm (water was used as a blank) after centrifuging the contents of the tube for 10 minutes. The difference between the absorbance of the control (3 mL of water plus 1 mL of wading reagent) and that of the samples was used to quantify the phytate concentration. The measurement of phytate was done using the phytic acid standard curve, and the results were given as phytic acids in mg/g dry weight. The relationship between absorbance and concentration was plotted as a standard curve, and the slope and intercept were calculated. Equation 1 presents the following relation, which was used to determine the amount of phytate in beans:
4.7.3. Determination of condensed tannin content
The method of Burns (Citation1971), as modified by Maxson and Rooney (1972), was used to determine the tannin content, with catechin serving as the tannin standard. Approximately 2.0 g of bean samples were weighed into a test tube, and they were extracted for 24 hours at room temperature with a mechanical shaker using 10 ml of 1 % HCl in methanol. The solution was then centrifuged for 5 minutes at 1000 rpm using a HERAEUS MEGAFUGE 8 Centrifuge (721113070119, China). The reaction was then completed by combining 1 ml of the supernatant with 5 ml of vanillin-HCl reagent, which had been previously made by mixing an equal volume of 8% concentrated HCl in methanol with 4 % vanillin in methanol. After zeroing the spectrophotometer with water, the absorbances of the sample solutions and the standard solution were measured at 500 nm.
4.7.4. Preparation of catechin standard
30 mg of catechin, standard for condensed tannin, was dissolved in 25 ml of 1 % methanol in HCl and used as a mother stock solution. The stock solutions were used to create a series of standard solutions with concentrations of 0, 0.2, 0.4, 0.6, 0.8, and 1.0 mg/mL catechin. The calibration curve was created by plotting absorbance versus concentration, and the slope and intercept were used to calculate the condensed tannin according to the following relation: On a dry weight basis, the results of the tannin content were expressed as equivalents of catechin per 100 g of flour.T
4.8. Design of an experiment and data analysis
The studies were carried out in triplicate, and Statistix 10.0 software was used for data analysis. The data collected in triplicate was subjected to analysis of variance (ANOVA), with the least significant difference (LSD) test being used to separate the means.
5. Results and discussions
5.1. Moisture & ash content of beans
The moisture and ash content of processed beans along with a control sample are presented in Table . The statistical analysis revealed that the moisture and ash contents are significantly different among treatments.
Table 1. Moisture and ash content of processed beans
Table presents the moisture, ash, and dry matter content of processed beans. The statistical analysis illustrated that the processing techniques affected the moisture, total minerals, and dry matter of beans. Moisture content was a bit higher after soaking in water and sodium bicarbonate as compared to the control. Germination for 12- and 48-hour soaking followed by ordinary cooking was found to have almost similar moisture contents. However, the highest moisture content was obtained by pressure cooking after soaking. This can be because the seed absorbed water before cooking. Because soaking allows the water to distribute in the protein fraction and starch granules, which help soften the texture of beans, this might be because of the normal functional characteristics of beans.
Ash, or the total mineral contents of beans, is a very important parameter, as are the processing techniques applied to beans. The total mineral content of beans was decreased due to the processing methods applied as compared to the control sample, with germination for 24-hours resulting in the lowest 5.29 % and soaking in sodium bicarbonate resulting in the highest 6.32 % total mineral content following the control sample. Since almost all of the processing methods applied included the steeping of the beans in water except the soaking in sodium bicarbonate treatment, the loss of ash content due to the leaching of mineral elements occurred. This result agrees with the previous study, where the ash content of Baobab seeds decreased from 8.93% (raw) to 5.84% and 8.93% to 6.12% by soaking in water and sprouting, respectively (Saulawa et al., Citation2014). The previous study reported that the total mineral content of beans was in the range of 3.90%–5.70% (Dueñas et al., Citation2016).
Similar findings on the impact of processing methods on field bean ash content showed reductions from 3.0 % to 2.66 %, 3.0 % to 2.83 %, and 3.0 % to 2.05 % after 18 hours of soaking, 40 hours of germination, and cooking of presoaked beans, respectively (Myrene, Citation2013). The dry matter content of the beans was also examined, and it was found that it exhibited an inverse relationship with the moisture content.
5.2. Processing techniques’ effects on the anti-nutritional contents of beans
In this experiment, the phytate and tannin levels of common beans were examined. The effects of soaking, germination, and cooking are summarized and shown in Table .
Table 2. Effect of different processing techniques on the reduction of phytate and tannin content of beans
5.3. Soaking, germination, and cooking effects on phytate
Table displays the ANF results along with the percentage reduction brought on by the processing techniques. The statistical analysis revealed that the phytate contents of all the processing techniques are substantially different from the control sample (P ≤ 0.05). Unprocessed beans had a phytate concentration of 52.25 mg per gram, while overnight soaking in water decreased that to 42.95 mg per gram, and overnight soaking plus pressure cooking reduced that to 33.8 mg per gram (Table ).
However, the previous study illustrated that the amount of phytate in common bean varieties is in the range of 10.4–29.30 mg.g.1 (black), 5.0–27.00 mg.g.1 (great northern bean), 6.1–23.80 mg.g.1 (pinto bean), 8.1–20.70 mg.g.1 (red beans), 12.0–26.30 mg.g.1 (red kidney), and 5.5–18.0 mg.g.1 (white) (Reddy & Sathe, Citation2002). The phytate content of Ethiopian common bean varieties ranges from 9.50 mg/g to 25.0 mg/g (Minuye et al., Citation2021). The present result with regard to the phytate content of unprocessed beans is not in agreement with the previous studies. This could be due to the differences in varietal and environmental conditions as well as geographical regions. The highest and lowest reductions in phytate contents were obtained for the pressure-cooked and water-soaked beans, with reductions of 35.21 % and 17.79 %, respectively.
The soaking in water and salt solutions (NaHCO3) affected the contents of phytate and showed a reasonable reduction of 17.79 % and 27.32 %, respectively. This is in agreement with the previous result for kidney beans, which showed a reduction of phytate content by 18 percent after soaking in water (Emire & Rakshit, Citation2007). It has been reported by Hotz and Gibson (Citation2007) that the phytic acid hydrolysis during steeping varies based on variety, pH conditions, temperature, soaking time, and other conditions. In a previous study, it was reported that a pH of 7.5 is optimum for phytate degradation in pea (Fredrikson et al., Citation2001).
To ®nd the maximum phytate degradation, the effects of temperature and pH during pea ®our incubation were investigated. The most ef®cient phytate degradation in pea ¯our incubation was achieved at pH 7.5 and 45°C.
The implementation of a simple household soaking procedure resulted in a 50 % reduction in the phytate content of unrefined maize flour (Hotz & Gibson, Citation2001). According to (Akeem et al., Citation2019), the leaching of water-soluble antinutritional elements like sodium, potassium, or magnesium phytate is enhanced by steeping in water. According to Ertaş and Türker (Citation2014). after soaking chickpeas for 2 to 12 hours, the phytic acid level decreased by 47.45 percent to 55.71 percent. During steeping, endogenous or exogenous phytase enzymes could be enhanced. It enhances reduction of ANFs through leaching and hydrolysis. Germination also greatly affected the phytate contents, with reductions of up to 33.949 % and 22.574 % for beans germinated for two days and one day, respectively. Germination allows the dry seeds to gain water, which activates the phytase activity and, in turn, enhances the degradation of phytate. The activity of natural enzymes in the beans is activated when water is taken up by the beans. Germination treatment is among the processing methods that increase the activity of native enzymes in legumes (either synthesizing or activating phytase) (Sandberg, Citation2002). Phytates are primarily contained in the cotyledons and embryo axes of common beans in nature (Blair et al., Citation2012). Accordingly, the application of germination is very important to release natural enzymes so that they can act up on the phytate salt to release phosphate and minerals and make them bioavailable to support emerging seedlings. A variety of metabolic processes, including the creation of hydrolytic enzymes that result in the hydrolysis of reserve food into a simple, accessible form for embryo ingestion, are activated during germination.
According to Hauwa Hajjagana et al. (Citation2014), it was found that wheat’s phytate and phytic acid concentrations were significantly reduced by 65 % as a result of germination. Similar to this, a recent study found that finger millet’s antinutritional components (phytate and tannin) were reduced by 50 % following germination for 0–96 hours (Abioye et al., Citation2018). The application of 24-hour germination of lentil, cowpea, chickpea, and green gram resulted in a phytate content reduction of about 20 % (Ghavidel & Prakash, Citation2007). Another investigation revealed that the level of phytic acid in green chickpea, as a result of germination for 96 hours, was reduced by 88.06 % (Singh et al., Citation2014). The study conducted in Egypt reported that germination significantly reduced the phytate level (24.9 2% to 35.27 %) in sorghum varieties (Afify et al., Citation2011). In this study, the germination period of 24 hours was not able to show a high reduction in phytate because a lot did not happen to degrade phytate content. The period of 24 hours was observed to be the commencement time for the actual and complete germination to occur. The germination time was not enough to allow the bean matrix to get soft and degrade the phytate in the embryo.
The degradation of the food matrix by heat processing could result in the liberation of phytate from beans. Soaking in combination with cooking showed a reduction of up to 35.21 % (soaking in water + pressure) and 33.29 % (soaking in water +ordinary cooking) in phytate content. It was reported that InsP6 was decreased by 7 % in ordinarily cooked black beans after discarding soaking water (Feitosa et al., Citation2018). This is not in agreement with the current result of this study. According to Emire and Rakshit (Citation2007), with ordinary cooking of kidney beans after steeping in water, a reduction of 65 % of the phytate content was achieved. In this study, the highest reduction in phytate concentration was achieved by pressure-cooking presoaked beans in water. This could be due to the degradation of phytate by the extreme steam that circulates at high pressure in the pressure cooker, which can easily soften the bean matrix. Cooking reportedly softens the food matrix and aids in the release of antinutritional elements (Viadel et al., Citation2006). A similar result has also been reported, as the cooking of presoaked faba beans reduced the phytic content from 501 g to 287 g, which is a 57 % reduction (Myrene, Citation2013). In the intestinal environment, phytate produces soluble and insoluble compounds with minerals, which adds to the difficulty of beans’ nutrient absorption (Gibson et al., Citation2010). It is widely present in legumes and cereal-staple foods for most Africans and inhibit the absorption of iron and zinc (Afify et al., Citation2011; Gibson et al., Citation2010). Autoclaving is also a crucial method for reducing the phytic acid content of legumes. Application of autoclaving for 45 minutes resulted in a 44 percent reduction in phytic acid in tribal pulse. According to Diouf et al. (Citation2019), fermentation resulted in 50% of the antinutritional factors. The extrusion at 140 °C reduces ANFs by up to 55.8 percent. Due to their near proximity to proteins, phytates make it more difficult to separate them during a straightforward processing step like milling in legumes (Sinha et al., Citation2017). Because it is a negatively charged ions, it typically forms complexes with positively charged metal ions including zinc, iron, magnesium, and calcium, and decreases their bioavailability by causing slower absorption rates.
The level of reduction in phytic acid contents due to the impacts of processing methods in this study was relatively low as compared to other previous studies. This difference could be due to the genetic characteristics of beans because they are different in their water absorption capacity, which also affects and facilitates the degree of phytate degradation. It would also be as a result of the difference in the complex interaction of phytate with nutrients in different beans. According to previous studies, environmental elements like growth circumstances, farming methods, and geography, as well as genetic variances, affect the amount of phytate that common beans contain (Helbig et al., Citation2003; Martinez Meyer et al., Citation2013; Pedrosa et al., Citation2015). It is widely accepted in science that the activation of natural phytase during bean processing is the primary factor in the decrease in phytate. Azeke et al. (Citation2011) found that the level of phytase activity grew and reached its maximum value after seven (16-fold), six (5-fold), five (7-fold), seven (3-fold), and eight (6-fold) days for rice, maize, millet, sorghum, and wheat, respectively, during germination.
5.4. Soaking, germination, and cooking effects on tannin content
Table presents the effects of processing techniques on the tannin content of beans. The processing methods significantly affected the tannin content of beans (p ≤ 0.02). The tannin content was reduced by 47.87, 54.75, 59.43, 66.69, 69.81 and 78.15 % for water-soaked, sodium bicarbonate-soaked, 24-germinated, 48hrs-germinated, ordinary-cooked and pressure-cooked beans samples respectively, as compared to untreated (control) sample. The previous study showed that the tannin level of raw beans was found to be 0.62 to 12.02 mg.g-1, and the reduction in the tannin content from 17–85% was reported for various varieties of beans as a result of cooking (Corzo-Ríos et al., Citation2020). The soaking in water and NaHCO3 solution showed a significant reduction in tannin content to 46.88% and 54.76%, respectively. This result is by far higher than the results reported by Emire and Rakshit (Citation2007) for kidney beans, which were 25.0% and 27.0%, respectively. This could be due to the genetic variations in bean varieties, soil types, and personal errors made by laboratory personnel during analysis.
The germination of beans also affected the tannin content of beans and resulted in a significant reduction depending on the treatment applied (Table ). The reduction in tannin content of beans by 59.43 % and 66.69 % was achieved for germination times of 24 hours and 48 hours, respectively. Similarly, the previous study reported that the tannin content of the germinated wheat was reduced by (64 %) (Hauwa Hajjagana et al., Citation2014). According to Ghavidel and Prakash (Citation2007), 30 percent reductions in the tannin content of legumes were reported after 24-hours germination period. It was also reported by Singh et al. (Citation2014) that the maximum reduction of 93.59 percent in tannin content of green chickpea was achieved by application of germination for 4 days. Germination and fermentation have been reported to be more effective methods to reduce heat-stable ANFs like phytate and polyphenols than thermal processing and soaking. Fermentation is a prominent method for neutralizing the ANFs due to its favorable effects and capacity to improve the bio-accessibility and bioavailability of nutrients from various crops (Jeyakumar & Lawrence, Citation2022).
Cooking treatment has shown a significant reduction in the tannin level of beans. Accordingly, the content of tannin was reduced to 69.81 % for ordinary cooked beans, whereas it was reduced to 78.1 % for pressure cooking. Pressure cooking showed the highest reduction in tannin content as compared to the control and other treatments. Similar findings made by Pal et al. (Citation2017) demonstrated that cooking five different lentil varieties reduced the tannin concentration by 68.5–84.63 percent.
The previous study reported that other processing unit operations, like dehulling, were used to reduce the anti-nutritional content of legumes. For instance, it was shown that after dehulling mung bean seeds, the activity of antinutritional factors such as trypsin inhibitor, hemagglutinin activity, tannins, and phytic acid was reduced by 7.59, 32.6, 33.3, and 20.7 %, respectively (Mubarak, Citation2005). The use of proper processing methods to lower the tannin level in beans is crucial to improving their nutritional value, particularly their protein content, as tannins in beans render protein inaccessible and diminish its digestion in both humans and animals. Dehulling and decortication are postharvest practices that have been observed to help reduce ANF levels in beans and grains (Korant et al., Citation2023; Singh et al., Citation2022).
The digestion process is impacted by the binding of tannins to minerals or proteins, which reduces nutritional availability and absorption. The hydroxyl groups of tannins and the carbonyl groups of proteins establish a sequence of hydrogen bonds, as a result of which the complex (tannin-protein) is created. Complexes produced by tannins’ binding to dietary proteins and digestive enzymes are difficult to digest. The binding of tannins to minerals or proteins impacts the digestion process, which reduces nutrition’s availability and absorption. The hydroxyl groups of tannins and the carbonyl groups of proteins establish a sequence of hydrogen bonds, as a result of which the complex (tannin-protein) is created. Complexes produced by tannins’ binding to dietary proteins and digestive enzymes are difficult to digest (Nikmaram et al., Citation2017; Raes et al., Citation2014). Proteases, which break down cellular proteins and enzymes to provide free amino acids that nourish seedlings, reduce ANFs throughout the germination process.
6. Conclusions
The common bean is a staple pulse in Africa, especially in the southern and eastern parts of the continent. Being a good source of proteins and minerals (Fe, Ca, Zn, and copper), beans are consumed alone or mixed with other foods. This study reveals that the phytate and tannin content of common beans were significantly affected by soaking, germination, and cooking. The phytate and tannin content of beans were reduced by the investigated processing techniques. Though all of the processing methods resulted in reductions in ANFs of beans, the highest reductions of 35.21 % and 77.69 % in phytate and tannin were achieved by the application of pressure cooking, respectively. Pressure cooking was the best processing technique, which resulted in a reduction of antinutritional factors (phytate and tannin) as compared to other processing methods. It is therefore, recommended to be used in societies where it is available and affordable. Traditional cooking, widely used in rural areas of bean-producing countries, also resulted in a reasonable reduction of ANFs following pressure cooking. The beans are widely consumed alone or mixed with other dishes in southern and eastern African countries, including Mozambique and Ethiopia. Nutrient bioavailability is still a problem and is attempted to be solved by applying the ANFs reduction strategy in this study. Considering this reality, the appropriate processing techniques, especially soaking in water followed by ordinary cooking, to help degrade the antinutritional factors and, in turn, enhance the bio-accessibility of essential minerals, should be applied. The Agricultural Research Centers and academic institutions should also train the rural communities that are relying on legumes, and beans in particular, as protein and essential mineral sources, and closely follow them to practice appropriate and easily applicable processing techniques. They should mainly provide training on legume processing for better nutritional value in terms of bioavailability and improved palatability.
About authors.docx
Download MS Word (12.8 KB)Public interest statement.docx
Download MS Word (12.3 KB)Acknowledgment
I would also like to thank the staff of the University of Eduardo Mondlane, Eng. Asimina Esmail Sulemane, Dr. Adolfo Firmino Condo, and Eng. Virginia Ivone Gongole, for their support during my research. I am also grateful to the European Union (EU) for financial support and the Intra-Africa Academic Mobility Scheme (MOUNAF) project coordinators for their sincere support.
Disclosure statement
No potential conflict of interest was reported by the author(s).
Data availability statement
We confirm that all the data concerning this manuscript are available.
Supplementary material
Supplemental data for this article can be accessed online at https://doi.org/10.1080/23311932.2023.2289713
Additional information
Funding
Notes on contributors
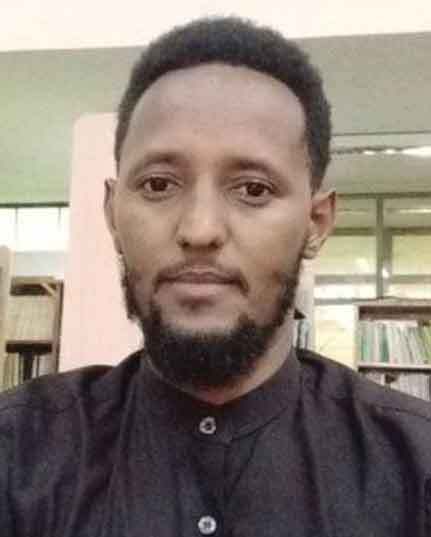
Wabi Bajo Nagessa
Wabi Bajo Nagessa is a researcher in food science and nutrition at the Ethiopian Institute of Agricultural Research, based at the Melkassa Research Center. He is a holder of a Master’s degree in Food Technology from the University of Eduardo Mondlane, Mozambique. He focuses on food processing techniques that result in nutrient retention, reduced antinutritional factors, and improved bioavailability of micro-and-macronutrients. His future research areas include food processing, functional and therapeutic food product development mainly for infants, pregnant women, and lactating women, food digestion, and public health nutrition.
Borges Chambal
Borges Chambal is a full-time professor at the University of Eduardo Mondlane and coordinator of the Ph.D. in Food Science and Technology at the department of chemical engineering. Prof. Dr. Custodia Macuamule is a professor and instructor at the University of Eduardo Mondlane, Faculty of Veterinary Medicine.
References
- Abioye, V. F., Ogunlakin, G. O., & Taiwo, G. (2018). Effect of germination on anti-oxidant activity, total phenols, flavonoids, and anti-nutritional content of finger Millet Flour. Journal of Food Processing & Technology, 09(2), 1–15. https://doi.org/10.4172/2157-7110.1000719
- Afify, A. E. M. M. R., El-Beltagi, H. S., El-Salam, S. M. A., & Omran, A. A. (2011). Bioavailability of iron, zinc, phytate, and phytase activity during soaking and germination of white sorghum varieties. PLoS ONE, 6(10), 1–7. https://doi.org/10.1371/journal.pone.0025512
- Akeem, S. A., Kolawole, F. L., Joseph, J. K., Monday, R., Kayode, O., & Akintayo, O. A. (2019). Traditional Food Processing Techniques and Micronutrients. Annals Food Science and Technology, 20(1), 30–41.
- Azeke, M. A., Egielewa, S. J., Eigbogbo, M. U., & Ihimire, I. G. (2011). Effect of germination on the phytase activity, phytate and total phosphorus contents of rice (Oryza sativa), maize (Zea mays), millet (panicum miliaceum), sorghum (sorghum bicolor) and wheat (Triticum aestivum). Journal of Food Science and Technology, 48(6), 724–729. https://doi.org/10.1007/s13197-010-0186-y
- Balasubramanian, P., Slinkard, A. R., & Tyler, A. V. (1999). Genotype and environment effect on canning quality of kabuli chickpea. Canadian Journal of Plant Science, 82(2), 267–272. https://doi.org/10.4141/P01-082
- Banti, M., & Bajo, W. (2020). Review on nutritional Importance and anti-nutritional factors of legumes. International Journal of Nutrition and Food Sciences, 9(6), 138–149. https://doi.org/10.11648/j.ijnfs.20200906.11
- Blair, M. W., Herrera, A. L., Sandoval, T. A., Caldas, G. V., Filleppi, M., & Sparvoli, F. (2012). Inheritance of seed phytate and phosphorus levels in common bean (Phaseolus vulgaris L.) and association with newly-mapped candidate genes. Molecular Breeding, 30(3), 1265–1277. https://doi.org/10.1007/s11032-012-9713-z
- Broughton, W. J., Hern, G., Blair, M., Beebe, S., Gepts, P., & Vanderleyden, J. (2003). Beans (Phaseolus spp .) – model food legumes. Plant and Soil, 252(1), 55–128. https://doi.org/10.1023/A:1024146710611
- Burns, R. E. (1971). Method for estimation of tannin in grain sorghum 1. Agronomy Journal, 63(3), 511–512. https://doi.org/10.2134/agronj1971.00021962006300030050x
- Canani, R. B., Cirillo, P., Buccigrossi, V., Ruotolo, S., Passariello, A., De Luca, P., Porcaro, F., De Marco, G., & Guarino, A. (2005). Zinc inhibits cholera toxin-induced, but not Escherichia coli heat-stable enterotoxin-induced, ion secretion in human enterocytes. Journal of Infectious Diseases, 191(7), 1072–1077. https://doi.org/10.1086/428504
- Corzo-Ríos, L. J., Sánchez-Chino, X. M., Cardador-Martínez, A., Martínez-Herrera, J., & Jiménez-Martínez, C. (2020). Effect of cooking on nutritional and non-nutritional compounds in two species of Phaseolus (P. vulgaris and P. coccineus) cultivated in Mexico. International Journal of Gastronomy and Food Science, 20(October), 100206. https://doi.org/10.1016/j.ijgfs.2020.100206
- Diouf, A., Sarr, F., Sene, B., Ndiaye, C., Fall, S. M., & Cyrille Ayesso, N. (2019). Pathways for reducing anti-nutritional factors: Prospects for Vigna unguiculata. Journal of Nutritional Health & Food Science, 7(2), 1–10. https://doi.org/10.15226/jnhfs.2019.001157
- Dueñas, M., Sarmento, T., Aguilera, Y., Benitez, V., Mollá, E., Esteban, R. M., & Martín-Cabrejas, M. A. (2016). Impact of cooking and germination on phenolic composition and dietary fibre fractions in dark beans (Phaseolus vulgaris L.) and lentils (lens culinaris L.). LWT - Food Science and Technology, 66, 72–78. https://doi.org/10.1016/j.lwt.2015.10.025
- Emire, S. A., & Rakshit, S. K. (2007, December). Effect of processing on antinutrients and in vitro digestibility of kidney bean (Phaseolus vulgaris L .) varieties grown in East Africa. https://doi.org/10.1016/j.foodchem.2006.08.005.
- Ertaş, N., & Türker, S. (2014). Bulgur processes increase nutrition value: Possible role in in-vitro protein digestability, phytic acid, trypsin inhibitor activity and mineral bioavailability. Journal of Food Science and Technology, 51(7), 1401–1405. https://doi.org/10.1007/s13197-012-0638-7
- Fabbri, A. D. T., & Crosby, G. A. (2016). A review of the impact of preparation and cooking on the nutritional quality of vegetables and legumes. International Journal of Gastronomy and Food Science, 3, 2–11. https://doi.org/10.1016/j.ijgfs.2015.11.001
- Feitosa, S., Greiner, R., Meinhardt, A. K., Müller, A., Almeida, D. T., & Posten, C. (2018). Effect of traditional household processes on iron, zinc and copper bioaccessibility in black bean (Phaseolus vulgaris L.). Foods, 7(8), 123. Www.Mdpi.Com/Journal/Foods. https://doi.org/10.3390/foods7080123
- Fredrikson, M., Alminger, M. L., Carlsson, N. G., & Sandberg, A. S. (2001). Phytate content and phytate degradation by endogenous phytase in pea (Pisum sativum). Journal of the Science of Food and Agriculture, 81(12), 1139–1144. https://doi.org/10.1002/jsfa.918
- Frølich, W. (2011). Phytate – a natural component in plant food. National Food Institute, Technical University of Denmark.
- Ghavidel, R. A., & Prakash, J. (2007). The impact of germination and dehulling on nutrients, antinutrients, in vitro iron and calcium bioavailability and in vitro starch and protein digestibility of some legume seeds. LWT - Food Science and Technology, 40(7), 1292–1299. https://doi.org/10.1016/j.lwt.2006.08.002
- Gibson, R. S., Bailey, K. B., Gibbs, M., & Ferguson, E. L. (2010). A review of phytate, iron, zinc, and calcium concentrations in plant-based complementary foods used in low-income countries and implications for bioavailability. Food and Nutrition Bulletin, 31(2), S134–S146. https://doi.org/10.1177/15648265100312s206
- Hauwa Hajjagana, L., Sheriff, M., & Arab Alhaji, M. (2014). Evaluation of the chemical composition, anti-nutrients and mineral element level of a composite meal from pearl millet, wheat, cowpea and groundnut. Sky Journal of Food Science, 3(8), 34–40. http://www.skyjournals.org/SJFS
- Helbig, E., De Oliveira, A. C., Queiroz, K. D. S., & Reis, S. M. P. M. (2003). Effect of soaking prior to cooking on the levels of phytate and tannin of the common bean (Phaseolus vulgaris, L.) and the protein value. Journal of Nutritional Science and Vitaminology, 49(2), 81–86. https://doi.org/10.3177/jnsv.49.81
- Hotz, C., & Gibson, R. S. (2001). Assessment of home-based processing methods to reduce the phytate content and phytate/zinc molar ratio of white maize (Zea mays). Journal of Agricultural and Food Chemistry, 49(2), 692–698. https://doi.org/10.1021/jf000462w
- Hotz, C., & Gibson, R. S. (2007). Traditional food-processing and preparation practices to enhance the bioavailability of micronutrients in plant-based diets. The Journal of Nutrition, 137(4), 1097–1100. https://doi.org/10.1093/jn/137.4.1097
- Jeyakumar, E., & Lawrence, R. (2022). Microbial fermentation for reduction of antinutritional factors. Current Developments in Biotechnology and Bioengineering Elsevier, 239–260. https://doi.org/10.1016/B978-0-12-823506-5.00012-6
- Jones, A. L. (1999). Phaseolus bean: Post-harvest operations. In Post-harvest compendium. Food and Agriculture Organization of the United Nations. Www.Fao.Org/3/a-Av015e.Pdf
- Kaur, D., & Kapoor, A. C. (1990). Starch and protein digestibility of rice bean (Vigna umbellata): Effects of domestic processing and cooking methods. Food Chemistry, 38(4), 263–272. https://doi.org/10.1016/0308-8146(90)90183-5
- Korant, N. P., Ramani, H. R., Patel, P. S., Bk, R., & Sankat, K. B. (2023). Review on nutritional and anti-nutritional factor of raw, cooked and sprouted cowpea. Asian Journal of Dairy & Food Research, 12(4), 585–589. https://doi.org/10.18805/ajdfr.DR-2092
- Kumar, Y., Basu, S., Goswami, D., Devi, M., Shivhare, U. S., & Vishwakarma, R. K. (2022). Anti-nutritional compounds in pulses: Implications and alleviation methods. Legume Science, 4(2), 1–13. https://doi.org/10.1002/leg3.111
- Latta, M., & Eskin, M. (1980). A simple and rapid colorimetric method for phytate determination. Journal of Agricultural and Food Chemistry, 28(6), 1313–1315. https://doi.org/10.1021/jf60232a049
- Lovato, F., Kowaleski, J., Da Silva, S. Z., & Heldt, L. F. S. (2018). Composição centesimal e conteúdo mineral de diferentes cultivares de feijão biorfortificado (Phaseolus vulgaris L.). Brazilian Journal of Food Technology, 21. https://doi.org/10.1590/1981-6723.6817
- Machado, C. M., Ferruzzi, M. G., & Nielsen, S. S. (2008). Impact of the hard-to-cook phenomenon on phenolic antioxidants in dry beans (Phaseolus vulgaris). Journal of Agricultural and Food Chemistry, 56(9), 3102–3110. https://doi.org/10.1021/jf072861y
- Martinez Meyer, M. R., Rojas, A., Santanen, A., & Stoddard, F. L. (2013). Content of zinc, iron and their absorption inhibitors in Nicaraguan common beans (Phaseolus vulgaris L.). Food Chemistry, 136(1), 87–93. https://doi.org/10.1016/j.foodchem.2012.07.105
- Matella, N. J., Dolan, K. D., Stoeckle, A. W., Bennink, M. R., Lee, Y. S., & Uebersax, M. A. (2005). Use of hydration, germination, and α-galactosidase treatments to reduce oligosaccharides in dry beans. Journal of Food Science, 70(3), C203–C207. https://doi.org/10.1111/j.1365-2621.2005.tb07126.x
- Minuye, M., Bajo, W., & Tejada Moral, M. (2021). Common beans variability on physical, canning quality, nutritional, mineral, and phytate contents. Cogent Food and Agriculture, 7(1). https://doi.org/10.1080/23311932.2021.1914376
- Mubarak, A. E. (2005). Nutritional composition and antinutritional factors of mung bean seeds (Phaseolus aureus) as affected by some home traditional processes. Food Chemistry, 89(4), 489–495. https://doi.org/10.1016/j.foodchem.2004.01.007
- Mumtaz Hamdani, A., & Ahmed Wani, I. (2017). Guar and Locust bean gum: Composition, total phenolic content, antioxidant and antinutritional characterisation. Bioactive Carbohydrates & Dietary Fibre, 11, 53–59. https://doi.org/10.1016/j.bcdf.2017.07.004
- Myrene, R. D. (2013). Effect of traditional processing methods on nutritional quality of. Advances in Bioresearch, 4(September), 29–33.
- Naozuka, J., & Oliveira, P. V. (2012). Cooking effects on iron and proteins content of beans (Phaseolus vulgaris L.) by GF AAS and MALDI-TOF MS. Journal of the Brazilian Chemical Society, 23(1), 156–162. https://doi.org/10.1590/S0103-50532012000100022
- Nikmaram, N., Leong, S. Y., Koubaa, M., Zhu, Z., Barba, F. J., Greiner, R., Oey, I., & Roohinejad, S. (2017). Effect of extrusion on the anti-nutritional factors of food products: An overview. Food Control, 79, 62–73. https://doi.org/10.1016/j.foodcont.2017.03.027
- Pal, R. S., Bhartiya, A., Yadav, P., Kant, L., Mishra, K. K., Aditya, J. P., & Pattanayak, A. (2017). Effect of dehulling, germination and cooking on nutrients, anti-nutrients, fatty acid composition and antioxidant properties in lentil (Lens culinaris). Journal of Food Science and Technology, 54(4), 909–920. https://doi.org/10.1007/s13197-016-2351-4
- Pedrosa, M. M., Cuadrado, C., Burbano, C., Muzquiz, M., Cabellos, B., Olmedilla-Alonso, B., & Asensio-Vegas, C. (2015). Effects of industrial canning on the proximate composition, bioactive compounds contents and nutritional profile of two Spanish common dry beans (Phaseolus vulgaris L.). Food Chemistry, 166, 68–75. https://doi.org/10.1016/j.foodchem.2014.05.158
- Petry, N., Boy, E., Wirth, J. P., & Hurrell, R. F. (2015). Review: The potential of the common bean (Phaseolus vulgaris) as a vehicle for iron biofortification. Nutrients, 7(2), 1144–1173. https://doi.org/10.3390/nu7021144
- Porch, T. G., Beaver, J. S., Debouck, D. G., Jackson, S. A., Kelly, J. D., Dempewolf, H., Rico, P., Trust, D., Crop, G., Lansing, E., Sciences, M., Program, G. R., Sciences, A., & Rico, P. (2013). Use of wild relatives and closely related species to adapt common bean to climate change. Agronomy, 3(2), 433–461. https://doi.org/10.3390/agronomy3020433
- Raes, K., Knockaert, D., Struijs, K., & Van Camp, J. (2014). Role of processing on bioaccessibility of minerals: Influence of localization of minerals and anti-nutritional factors in the plant. Trends in Food Science and Technology, 37(1), 32–41. https://doi.org/10.1016/j.tifs.2014.02.002
- Rahate, K. A., Madhumita, M., & Prabhakar, P. K. (2021). Nutritional composition, anti-nutritional factors, pretreatments-cum-processing impact and food formulation potential of faba bean (vicia faba L .): A comprehensive review. LWT, 138(December), 110796. https://doi.org/10.1016/j.lwt.2020.110796
- Reddy, N. R., & Sathe, S. K. (Eds.). (2002). Food phytates. CRC Press.
- Rocha-Guzmán, N. E., González-Laredo, R. F., Ibarra-Pérez, F. J., Nava-Berúmen, C. A., & Gallegos-Infante, J. A. (2007). Effect of pressure cooking on the antioxidant activity of extracts from three common bean (Phaseolus vulgaris L.) cultivars. Food Chemistry, 100(1), 31–35. https://doi.org/10.1016/j.foodchem.2005.09.005
- Sandberg, A.-S. (2002). Bioavailability of minerals in legumes. British Journal of Nutrition, 88(S3), 281–285. https://doi.org/10.1079/bjn/2002718
- Saulawa, L. A., Yaradua, A. I., Shuaibu, L., State, K., & State, K. (2014). Effect of different processing methods on proximate, mineral and anti nutritional factors content of Baobab (adansonia digitata) seeds. Pakistan Journal of Nutrition, 13(6), 314–318. https://doi.org/10.3923/pjn.2014.314.318
- Singh, P. K., Gautam, A. K., Panwar, H., Singh, D. K., Srivastava, N., Bhagyawant, S. S., & Upadhayay, H. (2014). Effects of germination on antioxidant and anti- nutritional factors of commonly used pulses. International Journal of Research in Chemistry and Environment, 4(2), 100–104.
- Singh, S., Singh, A. K., Singh, K., Singh, B., & Author, C. (2022). Millets processing, nutritional quality & fermented product: A review. The Pharma Innovation Journal, 11(5), 304–308. www.thepharmajournal.com
- Sinha, K., Khare, V., Scientist, J., & Bharti, L. (2017). Review on: Antinutritional factors in vegetable crops. The Pharma Innovation Journal, 6(12), 353–358. www.thepharmajournal.com
- Sruthi, N. U., Premjit, Y., Pandiselvam, R., Kothakota, A., & Ramesh, S. V. (2021). An overview of conventional and emerging techniques of roasting: Effect on food bioactive signatures. Food Chemistry, 348(January), 129088. https://doi.org/10.1016/j.foodchem.2021.129088
- Sue Horton, H. A., & R, J. A. (2008). Copenhagen Consensus 2008 Challenge paper: hunger and malnutrition. http://www.copenhagenconsensus.com/The%2010%20challenges/Malnutrition%20and%20Hunger-1.aspx. Global Crises, Global Solutions, 1–648. https://doi.org/10.1017/CBO9780511492624
- Suliburska, J., & Krejpcio, Z. (2014). Evaluation of the content and bioaccessibility of iron, zinc, calcium and magnesium from groats, rice, leguminous grains and nuts. Journal of Food Science and Technology, 51(3), 589–594. https://doi.org/10.1007/s13197-011-0535-5
- Tanasković, S. J., Šekuljica, N., Jovanović, J., Gazikalović, I., Grbavčić, S., Đorđević, N., Sekulić, M. V., Hao, J., Luković, N., & Knežević-Jugović, Z. (2021). Upgrading of valuable food component contents and anti-nutritional factors depletion by solid-state fermentation: A way to valorize wheat bran for nutrition. Journal of Cereal Science, 99(December), 103159. https://doi.org/10.1016/j.jcs.2020.103159
- Vaintraub, I., & Lapteva, N. (1988). Colorimetric determination of phytate in unpurified extracts of seeds and the products of their processing. Analytical Biochemistry, 175, 227–230. https://doi.org/10.1016/0003-2697(88)90382-X
- Viadel, B., Barberá, R., & Farré, R. (2006). Uptake and retention of calcium, iron, and zinc from raw legumes and the effect of cooking on lentils in caco-2 cells. Nutrition Research, 26(11), 591–596. https://doi.org/10.1016/j.nutres.2006.09.016
- Wortmann, C. S., Brink, M., & Belay, G. (2006). Phaseolus vulgaris L.(common bean). In Record from PROTA4U. Brink, M. & Belay, G. PROTA (Plant Resources of Tropical Africa/Ressources Végétales de l’Afrique Tropicale).