Abstract
The plasticization/antiplasticization effect of low molecular weight diluents on the thermal and mechanical properties of bread toast was studied. Those phenomena can influence the quality of food products and therefore it was established a relationship between the loss of crispness and the material state (glass, glass transition, and rubber). Water plasticization showed that the crispy texture was lost during the glassy state. Bread toast was in the glassy state during the hardening effect caused by antiplasticization. The latter phenomenon was observed only for properties analyzed under large deformation, which supported the explanation of “hole-filling” mechanism by diluent molecules (water) during antiplasticization. Since temperature modulated differential scanning calorimeter (TMDSC) revealed a fat melting event at ambient temperature, it is possible that fat molecules may contribute to the antiplasticization of some physical properties.
Introduction
Consumer appreciation of brittle cellular foods, such as bread toast, is highly dependent on textural properties such as crispness. These products have to be stored in dry conditions at room temperature to maintain their quality since an increase in temperature or water content results in loss of the crispy texture and produces a soggy and rubber material. This transition in texture characteristics is associated with the glass transition of the product since an increase in molecular motions changes the material from a glassy (crispy) to a rubber (deformable) state.Citation1 The complex composition of bread toast involving biopolymers, low-molecular weight components, and water also affects the texture.Citation2
The role of water has usually been viewed as that of a plasticizer, such that the mechanical properties related to the hardness decrease as moisture sorption occurs. For many materials, the behavior of stress at fracture or Young's modulus as a function of water activity has a sigmoidal shape and can be described by a model based on the Fermi equation. The loss of crispness as a result of moisture sorption can also be observed by attenuation on the jaggedness in the stress–strain curve.Citation3
In cereal-based materials, the stress at fracture increases up to a certain level over the low- to intermediate-moisture range.Citation4 The maximum level attained by this property may be attributed to “mechanical antiplasticization” by water, which often occurs in the glassy state.Citation2 Moreover, certain mechanical properties may be antiplasticized on humidification from the dry state while others, determined using the same test method, may be immediately plasticized.Citation5
Depression of the glass transition temperature (T g) by water can be described by a number of theoretical approaches, such as free volume or thermodynamic theories. Thermodynamic models, such as those of Gordon and Taylor (G–T),Citation6 Kwei,Citation7 and Couchman–Karasz,Citation8 have been used to predict the T g of a binary mixture and to describe the plasticization effect of water on biopolymers. Recently, temperature modulated differential scanning calorimetry (TMDSC), an enhancement over DSC, has provided new approach for thermal characterization of the glass transition. In TMDSC, a cyclic temperature modulation is superimposed on a linear or isothermal heating program, resulting in modulated heat flow.Citation9 The deconvolution of total heat flow in reverse and non-reverse signals allows the separation of the glass transition from other enthalpic relaxation events, all of which are condensed in only one curve in DSC.
The purpose of this work was to examine the plasticizer or antiplasticizer effects of water and other low-molecular weight diluents on the mechanical and thermal properties (glass transition temperature) of bread toast. Temperature modulated differential scanning calorimetry (TMDSC) technique was used to separate weak thermal events in a complex system, in an attempt to determine the role of each component on the molecular mobility of the system. The correlation between the mechanical and thermal data was examined in order to assess in which state (glass, glass transition, or rubber) the loss of crispness occurred.
Materials and Methods
Materials
Commercial bread toast (25×25×8.5 mm) was purchased at a local market. The average composition is shown in Table . The samples were removed from the package and equilibrated at 25°C over saturated solutions of P2O5, NaOH, LiCl, MgCl2, K2CO3, Mg(NO3)2, NaBr, NaNO2, NaCl, and KCl, until they reached moisture equilibrium. These samples were used to determine the sorption isotherm as well as mechanical and thermal properties.
Table 1 Average composition of commercial bread toast
Moisture Sorption Isotherms
The sorption isotherms were determined by the static gravimetric methodCitation10 at 25°C±1°C. Triplicate samples (1.2±0.15 g) were weighted in small plastic receptacles and placed on acrylic tripods in jars containing saturated salt solution. The samples were equilibrated for 4 weeks to reach a constant weight (±0.001 g/g dry solids). The weights of moisture equilibrated samples, and after drying in vacuum oven at 70°C for 20 h, were determined to a precision of 0.0001 g.
The GABCitation11 and BETCitation12 models [Eqs. (Equation1) and (Equation2)] were used to describe the dry basis (d.b.) moisture content (X) as a function of water activity (a w):
The criterion used to evaluate the goodness-of-fit of each equation was the mean relative percentage deviation (P) [Eq. (Equation3)], defined as:
Thermal Analysis
The glass transition temperatures were measured in TMDSC (DSC 2920 Modulated DSC, TA Instruments, New Castle, USA) coupled to a refrigerating cooling system (RCS). The DSC cells were purged with dried helium (26 mL/mm) and nitrogen (150 mL/min) was used as a cooling gas line. The DSC cell was calibrated for baseline without pans. Heat flow and temperature were calibrated by measuring the melting point of indium (156.6°C) and water (0°C) at the same scanning rate as in the experiments. The heat capacity calibration was done using sapphire (aluminium oxide).
The samples were analyzed over a temperature range from −50°C to 250°C, at a rate of 2.5°C/min and modulation temperature amplitude of ±0.5°C over a period of 40 s. The modulation parameters were chosen based on the consideration that there should be at least five cycles through any transitionCitation14 and that the signal of the modulated heat flow should not be distorted. The TMDSC scans were run on samples weighing 6–12 mg and sealed in hermetic DSC aluminium pans (from TA Instruments). All experiments were done using pairs of aluminium pans matched for weight to within ±0.1 mg. An empty aluminium pan was used as a reference.
The glass transition temperature was determined from the midpoint of the heat capacity change. To predict the water plasticization, the T g data were modeled using empirical models of Gordon–TaylorCitation6 and KweiCitation7 [Eqs. (Equation4) and (Equation5)].
Mechanical Properties
Mechanical properties were determined by uniaxial compression tests using a TA-XTIIi texture analyzer (Stable Microsystems Ltd., UK). Ten replicates of each sample were obtained and a mean value was calculated. The measurements were done at 25°C±1°C with a 3.5 cm diameter acrylic plate and a crosshead speed of 0.8 mm/s. The samples were compressed to produce up to 80% deformation.
Hencky stress (σ H ) and strain (ε H ) at rupture [Eqs. (Equation6) and (Equation7)] were calculated from the force and height data. Young's modulus (E) was determined from the slope of the linear region of the stress–strain plot.Citation15
The behavior of Young's modulus as a result of moisture sorption was characterized mathematically by Eq. (Equation8), based on the mathematical form of the Fermi distribution function.Citation3
The water activities of the samples used in the compression test (Table ) were determined in an Aqualab CX-2T (Decagon, USA) hygrometer, since the bread toast moisture could change as it was removed from the jars containing the saturated salt solution.
Results and Discussion
Sorption Isotherm
Knowledge of moisture sorption behavior is very important in dried cereal-based foods because of their crispness. Figure shows that the sorption isotherm of toast at 25°C gave the characteristic sigmoidal curve typical of many foods.Citation16 These data were fitted using the BET and GAB equations, which are based on a multilayer adsorption concept and have, respectively, two and three parameters with physical meaning. The parameters calculated for the GAB and BET equations are shown in Table , along with the goodness-of-fit of both models.
Table 2 GAB (X m, C, K) and BET (X m, C) parameters fitted to the isotherm of moisture sorption of toast
Despite the theoretical limitations of the BET adsorption analysis (a w<0.56), its monolayer parameter is very important for dried products and can be correlated to the initial loss of crispness.Citation17 The monolayer values calculated from the GAB equation were higher than those calculated from the BET equation, in agreement with similar findings in many food systems.Citation18 The additional constant present in the GAB model is a factor that corrects the properties of multilayer molecules with respect to the bulk liquid. This parameter is always lower than 1 and a high value, as observed for bread toast, indicates a structured state of the sorbate in the layer beyond the monolayer. The high value of k produced a pronounced upswing in the profile in the higher water activity range.Citation18 Since the GAB parameters were more representative than the corresponding BET values,Citation18 the GAB model was used in the remainder of this study.
Thermal Analysis
A typical TMDSC thermogram during the glass transition is presented in Fig. (A), showing the reversing and modulated heat flow. Reversing and non-reversing heat flow signals are related to changes in the amplitude of the modulation and in the underlying heat flow, respectively. The step in reversing heat flow indicates the glass transition while the enthalpic relaxation associated with the glass transition is seen in the total heat flow signal. The amplitude of the modulated heat flow signal showed a slight increase during the transition region since the heat capacity increased during this event.
Figure 2. (A) Temperature modulated differential scanning calorimetry thermogram showing the glass transition and enthalpic relaxation of bread toast. (B) Lissajous figure obtained during heating of bread toast from 0°C to 20°C at 2.5°C/min with a 40 s period and amplitude of 0.5°C.
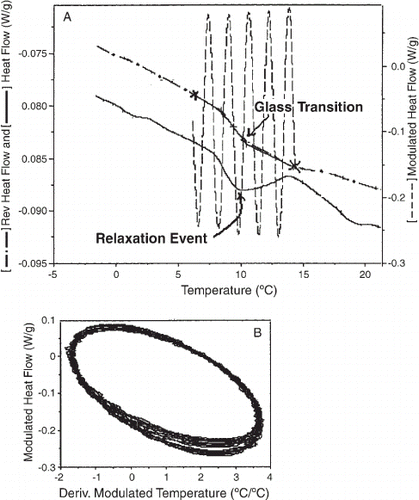
Two major conditions are required in a TMDSC scan to obtain a result free of artefacts. First, the glass transition region should have at least five modulation cycles,Citation14 and second, a linear response is needed for data treatment. In Fig. (A), five modulation cycles were obtained during the glass transition, as stated. The linearity of the response is shown in the Lissajous figure [Fig. (B)], in which the modulated heat flow is plotted against modulated temperature. Each cycle of the ellipse was not superimposed on the previous cycle, indicating that a transition was taking place. However, this small loss of linearity does not affect the mathematical treatment.
The typical behavior of the water plasticizing effect on amorphous food materials was observed as the T g values decreased with increasing moisture content (Fig. ). Glass transition temperature values ranged from 154°C for bread toast at 0% moisture to −14.5°C for samples with 18.3% moisture (wet basis). The glass transition temperature for many high-molecular-weight food polymers, such as polysaccharides,Citation19 Citation20 proteins,Citation21 or mixtures of both,Citation22 have been studied. The T g of bread toast with 0% moisture was similar to that reported for dry gluten (160–175°C)Citation19 Citation21 but quite different from that of dry, gelatinized starch (237°C).Citation23 The glass transition range was very broad because of the complexity and heterogeneity of bread toast and it was difficult to assign the non-reversing relaxation event to an individual ingredient (starch or gluten). No transition temperature was observed in samples with 2.6–6.9% moisture (wet basis) because of the superposition of other events, such as a water–polysaccharide interaction in the range of 40–70°C, as reported by Appelqvist et al.,Citation24 and a fat-melting peak near 32°C. Since the bread toast was manufactured with hydrogenated vegetable fat, the melting event should occur at a temperature higher than 20°C.
Figure 3. Midpoint glass transition temperature of bread toast equilibrated at different water activities.
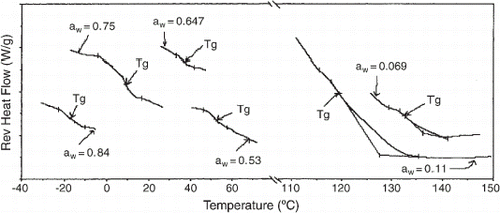
Slade et al.Citation25 reported a pair of small fat-melting endotherms in cookies and crackers with an onset temperature of 35°C. In a calorimetric scan, the melting of solid fat is frequently followed by a recrystallization phenomenon, which makes it difficult to determine the onset temperature of a single event.Citation26 However, the use of TMDSC technique allowed us to observe this superposed event, which was not evident in other DSC analyses of glassy bread.Citation27
To predict the water plasticization of bread toast, the glass transition temperatures as a function of solid content were fitted to the Gordon–Taylor and Kwei equations (Fig. ). The latter gave the best fit to the experimental data (Table ), especially at high water content (>12% moisture, wet basis). The parameter k of the Gordon–Taylor equation is a constant proportional to the plasticizing effect. The k value found was the same order of magnitude as those previously determined for wheat starch, k=5.2–5.6,Citation19 and gluten, k=5.1.Citation21 The Kwei equation is also an empirical model that takes into consideration secondary bonds, which usually occur in water–polymer systems with increasing water content. The first term (k), which is equivalent to the Gordon–Taylor parameter, is derived from free volume theory and described the plasticizing effect of water at low water content better than the Gordon–Taylor model. The second term (qw s w w) describes the low plasticizing effect of water on the matrix at high water contentsCitation28 and can be interpreted as the contribution of hydrogen bonds.Citation7 Thus, the high q value obtained for bread toast (q=195.3) strengthens the idea of a water–polysaccharide event between 40–70°C.
Figure 4. State diagram from T g analysis of bread toast as determined by TMDSC at heating rate of 2.5°C/min, 40 s of period and 0.5°C of amplitude.
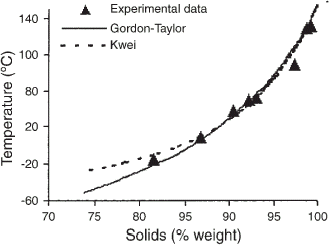
Table 3 Fitted parameters obtained from the Gordon–Taylor (k) and Kwei (k, q) equations
Table 4 Strain at fracture (ε H ) and Young's modulus (E) as a function of water activity (a w)
Mechanical Behavior
Experimental stress–strain curves for bread toast at different water activities are shown in Fig. . At 18.6% relative humidity (RH), the curve was very jagged and an increase in water activity attenuated the jaggedness, as seen for samples with 55.1% and 81.1% RH. This is characteristic of a plasticization effect that occurs because of an increase in moisture.Citation3 However, the total loss of crispness probably occurred at water activities higher than 0.551 (7.3% moisture content, wet basis), as observed for glassy corn cakes by Li et al.,Citation17 since the curve obtained in these conditions was still jagged. Thus, bread toast at 55.1% RH was probably still in the glassy state (see later).
Figure shows the behavior of the mechanical properties as a function of water activity. The standard deviations for brittle materials data were high because of the difficulty in determining the mechanical properties from high jagged stress–strain curves. The Young's modulus [Fig. (A)] evaluated at different water activities agreed with the plasticization behavior, as previously observed for starch-based food.Citation29 Thus, the Young's modulus data exhibit a sigmoidal behavior and fitted well to a model based on the Fermi equation (R 2=0.8764). The critical water activity obtained by the Young's modulus data was 0.637 (9.3% moisture, wet basis). The BET monolayer moisture content was smaller (3.7%, wet basis) than that observed for the critical water activity, thus confirming that this parameter was not related to the moisture that caused the loss of crispness.Citation17 Statistical analysis of the Young's modulus as a function of a w (Table ) showed three groups that were significantly different from each other. These three groups represent the three stages of the sigmoidal curve (glassy, glass transition, and rubber states).
Figure 6. Behavior of the mechanical properties as a function of water activity. (A) Young's modulus, (B) stress at fracture.
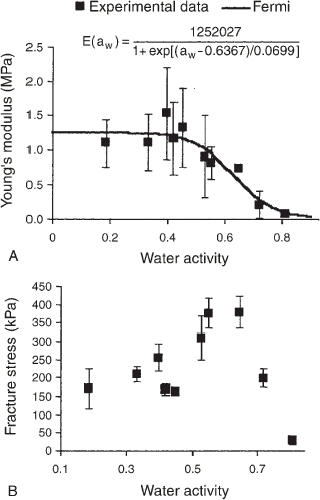
The stress [Fig. (B)] and strain at fracture (Table ) showed an antiplasticization phenomenon over a short range of water activity values (0.529–0.648), which corresponded to a moisture range of 6.9–9.6%. This behavior has been reported as “moisture toughening” or mechanical antiplasticization by water and is characteristic of cereal-based products. However, as the material completes its plasticization (beyond 9.6%), the fracture stress decreases and the softening effect of water becomes more important. This stiffening effect was also observedCitation1 in cereal-based foods but at a slightly higher moisture range of 9–11%.
The strain at fracture (Table ) did not show the increase expected with the gain in water. Rather, the strain at fracture of bread toast did not vary significantly up to a water activity of 0.529. Beyond this point, there was an increase up to 64.8% RH, with a subsequent decrease in the magnitude of this property, similar to that obtained for stress at fracture. This observation agrees with those for cereal-based foodCitation30 and tapioca starch film.Citation29
Correlation Between Moisture Content, Texture, and Thermal Analysis
Commercial bread toast (3.6% moisture content, wet basis) had a T g of 88.9°C, as determined by the Kwei equation, indicating that at ambient temperature toast is commercialized in the glassy state. The transition from the glassy to rubber state at 25°C corresponds to a water content of 11.1%, which was higher than the moisture necessary for complete loss of the crispy texture (9.3% moisture, wet basis). Therefore, the loss of crispness occurred while bread toast was in the glassy state. This could be related to sub-T g relaxation or may be associated with motions immediately preceding the onset of α-relaxation.Citation31
The antiplasticization effect shown by stress at fracture in the moisture range from 6.9–9.6% (wet basis), has been explained in various ways. Vrentas et al.Citation32 associated this effect with a hindering of polymer chain motions. Fontanet et al.Citation33 suggested that the hardening could result from a short-range reorganization allowed by greater local molecular mobility. Le Meste et al.Citation34 proposed that water could enhance the intermolecular, low-energy interactions between biopolymers, thereby improving the sample resistance to fracture. Harris and PelegCitation35 explained this unexpected stiffening effect of water by postulating a change in the fracture mechanism at an intermediate water content. In this hydration range, the compression would deform rather than disintegrate the matrix, which is partially plasticized at this point and could thus offer more resistance to fracture.Citation35 Seow et al.Citation2 hypothesized that antiplasticization by water could be responsible for the hardening effect. In addition to this explanation, Chang et al.Citation29 proposed a “hole-filling” mechanism involving the filling of “holes” created under high stress/deformation with diluent (water) molecules, thereby effectively reducing the free volume of the system. The latter hypothesis is particularly important because an antiplasticization effect is generally observed in properties obtained by large deformation tests, whereas a plasticization effect occurs on small deformations and thermal properties.
In the present study a fat-melting event with an onset temperature of 32°C was observed. However, the true melting temperature probably occurred before 32°C, since the melting and crystallization of fats usually occur concomitantly. In view of the latter and the fact that the physical state of low-moisture foods may be affected by the phase transition of lipids,Citation26 the fat component of bread toast may contribute to the antiplasticization behavior. Baltsavias et al.Citation36 observed that the mechanical properties of cellular material, such as biscuits, are governed mainly by the air volume fraction and fat volume fraction. The fat continuity also affects these properties, even when the non-fat phase is in the glassy state. In another study, Baltsavias et al.Citation37 showed that low-fat biscuits behaved as fat dispersed systems and that fat globules may be deformed and coalesce to produce a continuous fat phase. Thus, an increase in moisture content and the water plasticization phenomenon should reduce the viscosity of the matrix of brittle cellular material (bread toast), thereby allowing fat globules to coalesce under compression. Thus, fat and water would then fill “holes” created under high stress/deformationCitation29 and lead to the antiplasticization phenomena.
Conclusions
The plasticization or antiplasticization effect of small molecules was dependent on the physical properties analyzed. Even when the same test method was used, the Young's modulus was plasticized while the stress and strain at fracture were antiplasticized. The fact that antiplasticization was only observed on properties evaluated under large deformation supports the hypothesis for the hardening effect in which water would fill “holes” created under high stress/deformation. In this study, TMDSC revealed a fat melting event at ambient temperature, which suggested that fat molecules could contribute to the antiplasticization effect of water. At high levels of hydration the large deformation properties were fully plasticized. The plasticization effect of water at intermediate moisture content only affected the mechanical properties under small deformation and the glass transition temperature. The determination of the critical water content for the glass transition and the loss of crispness at room temperature showed that texture stability was lost during the glassy state. These findings indicate that more than one technique may be needed to understand the role of small molecules as plasticizers and antiplasticizers. The data also suggest that the texture quality of products such as that analyzed here may be better controlled by mechanical tests than by the glass transition temperature.
Acknowledgments
This work was supported by Fundação de Amparo à Pesquisa do Estado de Sa˜o Paulo—Brazil (FAPESP, grant no. 99/12917-7). RLC is supported by the Conselho Nacional de Desenvolvimento Científico e Tecnológico (CNPq, Brazil) and ALMB is supported by Coordenação de Aperfeiçoamento de Pessoal de Nível Superior (CAPES, Brazil) and FAPESP (grant no. 00/00790-1).
References
- Roudaut , G. , Dacremont , C. and Le Meste , M. 1998a . Influence of water on the crispness of cereal-based foods: acoustic, mechanical, and sensory studies . J. Texture Stud. , 29 ( 2 ) : 199 – 213 .
- Seow , C.C. , Cheah , P.B. and Chang , Y.P. 1999 . Antiplasticization by water in reduced-moisture food systems . J. Food Sci. , 64 ( 4 ) : 576 – 581 .
- Wollny , M. and Peleg , M. 1994 . A model of moisture-induced plasticization of crunchy snacks based on Fermi's distribution function . J. Sci. Food Agr. , 64 ( 4 ) : 467 – 473 . [CSA]
- Suwonsichon , T. and Peleg , M. 1998 . Instrumental and sensory detection of simultaneous brittleness loss and moisture toughening in three puffed cereals . J. Texture Stud. , 29 ( 3 ) : 255 – 274 .
- Chang , Y.P. , Cheah , P.B. and Seow , C.C. 2000a . Variations in flexural and compressive fracture behavior of a brittle cellular food (dried bread) in response to moisture sorption . J. Texture Stud. , 31 ( 5 ) : 525 – 540 .
- Gordon , M. and Taylor , J.S. 1952 . Ideal copolymers and the second-order transitions of synthetic rubbers. I. Non-crystalline copolymers . J. Appl. Chem. , 2 ( 9 ) : 493 – 500 .
- Kwei , T.K. 1984 . The effect of hydrogen-bonding on the glass-transition temperatures of polymer mixtures . J. Polym. Sci. Part C-Polym. Lett. , 22 ( 6 ) : 307 – 313 .
- Couchman , P.R. and Karasz , F.E. 1978 . A classical thermodynamic discussion of effect of composition on glass-transition temperatures . Macromolecules , 11 ( 1 ) : 117 – 119 .
- Reading , M. , Elliott , D. and Hill , V.L. 1993 . A new approach to the calorimetric investigation of physical and chemical-transitions . J. Therm. Anal. , 40 ( 3 ) : 949 – 955 .
- Spiess , W.E.L. and Wolf , W. 1983 . “ The results of the COST 90 project on water activity ” . In Physical Properties of Foods Edited by: Jowitt , R. , Escher , F. , Hallstrom , F.B. , Meffert , M.F. , Spiess , W.E.L. and Vos , G. 65 – 91 . London : Applied Science Publishers .
- Van den Berg , C. 1985 . “ Development of B.E.T. like models for sorption of water on foods. Theory and relevance ” . In Properties of Water in Foods in Relation to Quality and Stability Edited by: Simatos , D. and Multon , J.L. 119 – 131 . Dordrecht : Martinus Nijhoff Publishers .
- Brunauer , S. , Emmett , P.H. and Teller , E. 1938 . Adsorption of gases in multimolecular layers . J. Am. Chem. Soc. , 60 : 309 – 319 .
- Lomauro , C.J. , Bakshi , A.S. and Labuza , T.P. 1985 . Evaluation of food moisture sorption isotherm equations. I. Fruit, vegetable and meat products. Lebensmittel–Wissenschaft und Technologie . 18 ( 2 ) : 111 – 117 .
- Hill , V.L. , Craig , D.Q.M. and Feely , L.C. 1998 . Characterisation of spray-dried lactose using modulated differential scanning calorimetry . Int. J. Pharm. , 161 ( 1 ) : 95 – 107 . [CROSSREF]
- Steffe , J.R. 1996 . Rheological Methods in Food Process Engineering 418 pp. East Lansing : Freeman Press .
- Iglesias , H.A. and Chirife , J. 1982 . Handbook of Food Isotherms: Water Sorption Parameters for Food and Food Components 347 pp. New York : Academic Press .
- Li , Y. , Kloeppel , K.M. and Hsieh , F. 1998 . Texture of glassy corn cakes as a function of moisture content . J. Food Sci. , 63 ( 5 ) : 869 – 872 .
- Timmermann , E.O. , Chirife , J. and Iglesias , H.A. 2001 . Water sorption isotherms of foods and foodstuffs: BET or GAB parameters? . J. Food Eng. , 48 ( 1 ) : 19 – 31 . [CROSSREF]
- Kalichevsky , M.T. , Jaroszkiewicz , E.M. , Ablett , S. , Blanshard , J.M.V. and Lillford , P.J. 1992 . The glass-transition of amylopectin measured by DSC, DMTA and NMR . Carbohyd. Polym. , 18 ( 2 ) : 77 – 88 . [CROSSREF]
- Lourdin , F. , Coignard , L. , Bizot , H. and Colonna , P. 1997 . Influence of equilibrium relative humidity and plasticizer concentration on the water content and glass transition of starch materials . Polymer , 38 ( 21 ) : 5401 – 5406 . [CROSSREF]
- Micard , V. and Guilbert , S. 2000 . Thermal behaviour of native and hydrophobized wheat gluten, gliadin and glutenin-rich fractions by modulated DSC. Int . J. Biol. Macromol. , 27 ( 3 ) : 229 – 236 . [CROSSREF]
- Kalichevsky , M.T. and Blanshard , J.M.V. 1992 . A study of the effect of water on the glass-transition of 1/1 mixtures of amylopectin, casein and gluten using DSC and DMTA . Carbohyd. Polym. , 19 ( 4 ) : 271 – 278 . [CSA] [CROSSREF]
- Zeleznak , K.J. and Hoseney , R.C. 1987 . The glass transition in starch . Cereal Chem. , 64 ( 2 ) : 121 – 124 .
- Appelqvist , I.A.M. , Cooke , D. , Gidley , M.J. and Lane , S.J. 1993 . Thermal properties of polysaccharides at low moisture. 1. An endothermic melting process and water-carbohydrate interactions . Carbohyd. Polym. , 20 ( 4 ) : 291 – 299 . [CSA] [CROSSREF]
- Slade , L. , Levine , H. , Wang , M. and Ievolella , J. 1996 . DSC analysis of starch thermal properties related to functionality in low-moisture baked goods . J. Therm. Anal. , 47 ( 5 ) : 1299 – 1314 .
- Roos , Y.H. 1995 . “ Food components and polymers ” . In Phase Transitions in Foods 109 – 156 . San Diego : Academic Press .
- Roudaut , G. , Van Dusschoten , D. , Van As , H. , Hennubga , M.A. and Le Meste , M. 1998b . Mobility of lipids in low moisture bread as studied by NMR . J. Cereal Sci. , 28 : 147 – 155 . [CROSSREF]
- Cuq , B. and Vernière , C.I. 2001 . Characterisation of glass transition of durum wheat semolina using modulated differential scanning calorimetry . J. Cereal Sci. , 33 ( 2 ) : 213 – 221 . [CROSSREF]
- Chang , Y.P. , Cheah , P.B. and Seow , C.C. 2000b . Plasticizating-antiplasticizating effects of water on physical properties of tapioca starch films in the glassy state . J. Food Sci. , 65 ( 3 ) : 445 – 451 .
- Attenburrow , G.E. and Davis , A.P. 1993 . “ The mechanical properties of cereal-based foods in and around the glassy state ” . In The Glassy State in Foods Edited by: Blanshard , J.M.V. and Lillford , P.J. 317 – 331 . Loughborough : Nottingham University Press .
- Champion , D. , Le Meste , M. and Simatos , D. 2000 . Towards an improved understanding of glass transition and relaxations in foods: molecular mobility in the glass transition range . Trends Food Sci. Technol. , 11 ( 2 ) : 41 – 55 . [CROSSREF]
- Vrentas , J.S. , Duda , J.L. and Ling , H.C. 1988 . Antiplasticization and volumetric behavior in glassy-polymers . Macromolecules , 21 ( 5 ) : 1470 – 1475 . [CSA]
- Fontanet , I. , Davidou , S. , Dacremont , C. and Le Meste , M. 1997 . Effect of water on the mechanical behaviour of extruded flat bread . J. Cereal Sci. , 25 : 303 – 311 . [CROSSREF]
- Le Meste , M. , Roudaut , G. and Davidou , S. 1996 . Thermomechanical properties of glassy cereal foods . J. Therm. Anal. , 47 ( 5 ) : 1361 – 1376 .
- Harris , M. and Peleg , M. 1996 . Patterns of textural changes in brittle cellular cereal foods caused by moisture sorption . Cereal Chem. , 73 ( 2 ) : 225 – 231 .
- Baltsavias , A. , Jurgens , A. and Van Vliet , T. 1999a . Fracture properties of short-dough biscuits: effect of composition . J. Cereal Sci. , 29 ( 3 ) : 235 – 244 . [CROSSREF]
- Baltsavias , A. , Jurgens , A. and Van Vliet , T. 1999b . Properties of short-dough biscuits in relation to structure . J. Cereal Sci. , 29 : 245 – 255 . [CROSSREF]