Abstract
Objective: Interstitial brachytherapy is becoming an accepted treatment option for lung cancer patients for whom surgery poses a high risk. Robotic surgery has the potential to deliver brachytherapy seeds into tumors while keeping surgeons at a safe distance from the radioactive source. Our aim was to compare the accuracy, number of attempts, and time needed to place seeds next to a target when using a manual technique, video-assisted thoracoscopic surgery (VATS), and the ZEUS robot for minimally invasive surgery (MIS).
Methods: A brachytherapy seed injector was developed and attached to one of the ZEUS robotic arms. Four subjects each inserted inactive dummy brachytherapy seeds into clear agar-gelatin cubes containing a 1.6-mm stainless steel ball target. Two orthogonal radiographs were taken of each agar cube, and the corresponding distances were measured in triplicate using ImageJ processing software. The mean distance between the center of each seed and the corresponding target was calculated using the Pythagorean theorem. Comparisons were made using analysis of variance, t-tests, and Kruskal–Wallis tests, as appropriate.
Results: A total of 384 tests (128 for each technique) were performed. The median accuracies for the manual technique, VATS, and ZEUS were 1.8 mm (range: 0.9–6.7 mm), 2.4 mm (range: 1.0–11.3 mm), and 3.6 mm (range: 1.3–16.7 mm), respectively (p < 0.01). The median numbers of attempts for the manual technique, VATS, and ZEUS were 1 (range: 1–5), 4 (range: 1–14), and 3 (range: 1–20), respectively (p < 0.01). The median times for the manual technique, VATS, and ZEUS were 3.0 s (range: 1–43 s), 86.5 s (range: 6–372 s), and 64.5 s (range: 5–356 s), respectively (p < 0.01).
Conclusions: The manual technique is the most accurate, least traumatic, and fastest method of inserting seeds into tumors. The ZEUS robotic platform was able to place seeds beside a target within a clinically acceptable distance, with an acceptable amount of trauma and time required. It achieved results equal to or better than those obtained with VATS.
Introduction
Lung cancer is the most common cancer in the world, and is also the most common cause of death from cancer Citation[1]. Surgical resection is the treatment of choice, but only one third of patients can undergo a curative resection Citation[2]. For the remaining two thirds, therapeutic options include radiation and chemotherapy, although neither is curative by itself.
Endoluminal high dose rate (HDR) brachytherapy is used routinely in the palliation of lung cancer. The radiation source is usually placed through the tumor with a bronchoscope and then removed. HDR brachytherapy has also been delivered to lung cancers using a percutaneous route Citation[3–5]. Low dose rate (LDR) interstitial brachytherapy involves the permanent implantation of seeds into a tumor. It allows for more precise delivery of radiation and easier adaptation to the tumor shape than is possible with external radiation sources Citation[6], Citation[7]. A higher dose can be delivered to the tumor volume with less damage to the normal lung. Hilaris and Martini reviewed the Memorial Sloan-Kettering Cancer Center experience of over 1000 patients who received intraoperative LDR brachytherapy via an open thoracotomy Citation[6]. The use of encapsulated I125 seeds greatly reduced the radiation outside the treatment volume, and simplified the exposure for medical and nursing staff; however, the intraoperative radiation exposure for the surgeon and the need for a large open thoracotomy with only a modest survival advantage has hindered clinical acceptance of this approach.
There is only limited experience with interstitial brachytherapy using bronchoscopic, fluoroscopic and CT guidanc Citation[2], Citation[8]. There have been technical complications related to inability to penetrate scirrhous tumors, leading to suboptimal dose distribution. The CT-guided percutaneous approach is limited to small tumors in the periphery of the lung, and an optimum radiation dose distribution cannot be achieved due to the bony structures in the chest wall inhibiting precise seed implantation. The risk of injury to proximal mediastinal structures, bleeding, and lung collapse also limit this technique to small tumors adjacent to the chest wall.
Video-assisted thoracoscopic surgery (VATS) employs instruments inserted through small incisions in the patient's chest. The procedure relies on endoscopic video for guidance, and on the surgeon for instrument manipulation. If intraoperative complications should occur, they can be dealt with immediately. The minimally invasive approach reduces postoperative pain and length of hospital stay, and facilitates an earlier return to more normal activity. There are, however, some limitations with this method, such as impaired vision and restricted maneuverability of the instruments. The techniques are difficult to learn and are therefore used less often.
Surgical robots have been shown to vastly improve the efficiency of minimally invasive surgery (MIS) by providing superior 3D magnification, superior dexterity with 7 degrees of freedom, and improved precision of dissection through implementation of tremor filtration and motion scaling Citation[9–11]. Further, the robots provide the surgeon with improved ergonomics and enable a shorter learning curve. The ZEUS surgical system (manufactured by Computer Motion, Inc., Goleta, CA) was the world's first telesurgical robotic platform. It is an ergonomically optimal environment and interfaces with a networked operating room and surgical devices. Surgical robots not only facilitate the surgical removal of cancer using thoracoscopic and laparoscopic techniques, but also enable image-guided therapy for more precise, consistent and safe implantation of brachytherapy seeds with minimal patient morbidity Citation[15]. Robotic surgery has the potential to deliver brachytherapy seeds into tumors while keeping surgeons at a safe distance from the radioactive source.
The recent increase in VATS has renewed interest in the role of adjuvant brachytherapy for lung cancer Citation[12–17]. Following resection of small lung tumors (T1N0), intraoperative I125 seeds have been implanted along the resection margins with Vicryl® meshCitation[12], Citation[13], Citation[17]. Pisch and colleagues have recently reported on the da Vinci robot's ability to suture brachytherapy seeds into a porcine lung Citation[15]. VATS wedge resections were performed in the upper and lower lobes, and dummy I125 seeds embedded in absorbable sutures were sewn into the resection margin with the aid of the da Vinci robotic system without complications. The robotic technology allowed direct placement of radioactive seeds into the resection margin by endoscopic surgery. This renewed enthusiasm for lung brachytherapy has resulted in a randomized phase III study of sublobar resection versus sublobar resection plus brachytherapy in high-risk patients with non-small cell lung cancer, sponsored by the American College of Surgeons Oncology Group Citation[18].
Our overall goal is to develop the technique of depositing individual brachytherapy seeds into lung cancers using a minimally invasive robot-assisted brachytherapy seed delivery system. The objective of the current study was to compare the accuracy, number of attempts, and time required to place seeds next to a target when using a manual technique, VATS, and the ZEUS MIS robot.
Materials and methods
A custom-designed VATS box was used to simulate the chest cavity (). Clear agar-gelatin cubes (4.5 cm × 4.5 cm × 4.5 cm) were used to simulate the lung tissue Citation[19]. They were made from gellant gum powder (Sigma–Genosys Canada, Oakville, ON) dissolved in warm water, poured into moulds, and allowed to cool. Each cube contained a 1.6-mm stainless steel ball (measured size: 1.582 mm) to serve as the target.
Figure 1. The set-up for the experiments with the ZEUS system using the injector to insert seeds into agar cubes within the VATS box. The surgeon could only see the agar cube on the monitor. [Color version available online.]
![Figure 1. The set-up for the experiments with the ZEUS system using the injector to insert seeds into agar cubes within the VATS box. The surgeon could only see the agar cube on the monitor. [Color version available online.]](/cms/asset/10f7a2d2-1fb9-4616-9582-3a62a4b8a004/icsu_a_262546_f0001_b.gif)
Inactive “dummy” brachytherapy seeds (length: 5 mm; diameter: 0.777 to 0.800 mm) were loaded in an 18-gauge brachytherapy needle (Mentor, Minneapolis, MN). Four test subjects–two thoracic surgeons and two non-surgeons–each inserted seeds into the clear agar cubes, trying to hit the visible steel ball target. The thoracic surgeons had some previous experience with VATS and the ZEUS system, but all participants received training and were allowed to practice until they felt comfortable with the tasks. Three different techniques were used: manual, VATS and ZEUS.
The manual technique involved direct freehand manual insertion of the needle into the agar cube, relying only on visualization to maintain the trajectory to the target. The VATS box was not used and the needles could be inserted into the agar cubes using any trajectory. This method simulated an open thoracotomy approach, which allows a direct view of the target. With the VATS technique, the agar cubes were placed inside the VATS box and the brachytherapy seeds were implanted by manually inserting the brachytherapy needle through a thoracoscopic port into the agar cube using 2D thoracoscopic visual guidance. The cubes and targets could only be visualized via the monitor (Stryker Vision, Hamilton, Ontario). This method simulated a VATS procedure.
For the ZEUS technique, a seed injector for implanting radioactive seeds was developed, and attached to one of the arms of the ZEUS surgical robotic system (Computer Motion, Inc., Goleta, CA) ( and ) Citation[20], Citation[21]. The current weight of this injector is 970 g. The main body of the device, the needle support, and the plunger support are all fully sterilizable, while the motor, encoder and cables can be covered with sterile drapes. The speed of insertion is controllable and varied between 1.7 and 3.5 mm/s. The maximum achievable speed is 33.3 mm/s. The agar cubes were placed inside the VATS box, and the seeds were implanted telesurgically with the ZEUS system. The operator sat at the ZEUS console at a “safe” distance from the seeds, and manipulated the arm to insert the needle into the agar cube. Once the ideal position was found, the brachytherapy seeds were deposited into the cube using the injector.
Measurements
The accuracy of each technique was determined from two orthogonal radiographs taken of each agar cube () using ImageJ software. ImageJ is a public domain Java image processing program inspired by NIH Image from the National Institutes of Health Citation[22]. In each of the orthogonal images, the distance from the center of the seed to the center of the target was measured, then projected onto the two coordinate axes visible in the image plane of the radiograph (taking into account the angle of the cube with respect to the image). From the two images it was then possible to determine the projections of the distances onto the three coordinate axes of the cube, using the Pythagorean theorem to calculate the final distance. All measurements were made in triplicate. The number of attempts at reaching the target was recorded as the number of times the needle penetrated the agar cubes prior to seed release. The time taken to insert the needle was also recorded. The assessors were blinded to the methods used and the measurements by the other assessors.
Sample size
We hypothesized that the ZEUS robot would achieve results similar to those with the VATS technique. The manual technique was assumed to be the ideal method and was anticipated to be superior to the others. Expert clinical opinion from radiation oncologists and physicists estimated that an accuracy (Δ error) of 2 mm would be biologically and clinically acceptable. We assumed that a difference of less than 2 mm would establish equivalency between VATS and the ZEUS robot. Our pilot work estimated the standard error (σ) with ZEUS to be 4.5 mm. Assuming a type I error rate (α) of 0.05 and type II error rate (β) of 0.9, the minimum estimated number of injections required was 106 for each method to demonstrate equivalency between ZEUS and VATS Citation[23].
Statistical analysis
The Statistical Package for Social Sciences (SPSS) software, version 13.0 for Windows (SPSS, Inc., Chicago, IL), was used for statistical analysis. A two-way, random effects analysis of variance (ANOVA) was performed to compare the accuracies and times for the three methods. Unpaired t-tests were used to compare the accuracies and times for VATS and ZEUS, while the numbers of attempts for the three methods were compared using the Kruskal–Wallis test.
Results
The distributions of results were not normal. The mean and the median values are both reported, and the median is more representative of the results because the distributions were skewed.
Accuracy
The median accuracies (distance from the target) for the manual, VATS, and ZEUS techniques were 1.8 mm (range: 0.9–6.7 mm), 2.4 mm (range: 1.0–11.3 mm) and 3.6 mm (range: 1.3–16.7 mm), respectively ( and ). The manual technique was found to be the most accurate (giving the smallest error) when implanting seeds beside the target in agar, while the ZEUS technique was the least accurate. However, the difference between the ZEUS method and the VATS method was only 1.2 mm, which was less than our a priori estimate of 2 mm. The differences were all statistically significant (p < 0.001).
Figure 4. The median accuracy of each method is displayed as the distance from the center of the injected seed to the center of the target. The accuracy of the ZEUS technique was inferior to that of the VATS technique by 1.2 mm.
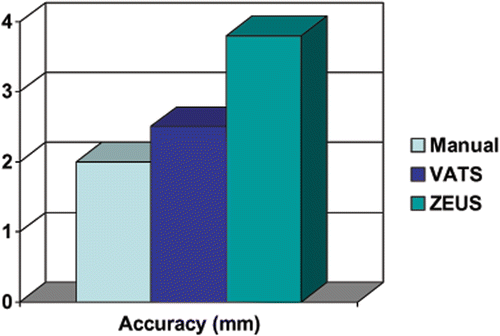
Table I. The accuracy of each method is displayed as the measured distance from the center of the injected seed to the center of the target.
Number of attempts
The median numbers of attempts for the manual, VATS, and ZEUS techniques were 1 (range: 1–5), 4 (range: 1–14) and 3 (range: 1–20), respectively ( and ). The manual technique was the least traumatic approach (i.e., requiring the fewest needle punctures) to implanting the seeds in the agar, while the VATS was the most traumatic. The results were significantly different from one another (p < 0.001).
Figure 5. The median number of attempts required for the needle to reach the target for each method. The ZEUS technique was superior to the VATS technique, requiring one less attempt on average.
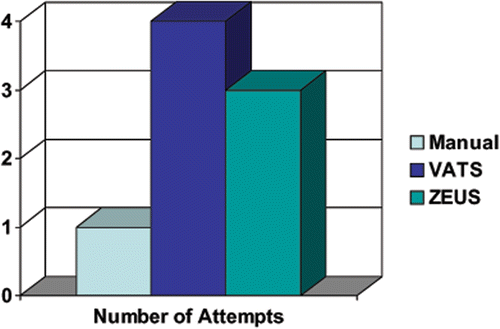
Table II. Number of attempts to reach the target for each method.
Time
The median times required to insert the needles for the manual, VATS, and ZEUS techniques were 3.0 s (range: 1–43 s), 86.5 s (range: 6–372 s) and 64.5 s (range: 5–356 s), respectively ( and ). The manual technique was the fastest at positioning the needle in the agar, while the VATS technique was the slowest. The results were significantly different from one another (p < 0.001).
Figure 6. The median time required to insert the needle to the desired location for each method. The ZEUS technique was faster than the VATS technique by 22 s.
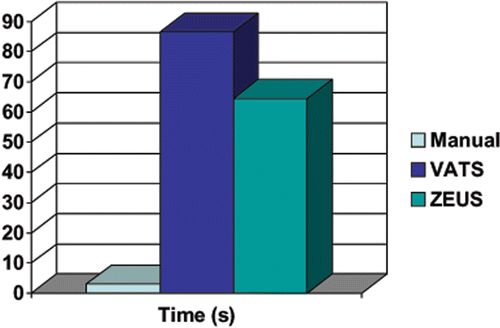
Table III. Time required to insert the needle and deploy the seed to the desired location for each method.
Discussion
Many researchers have been working on ways to improve robotic brachytherapy procedures Citation[24–31] (also see http://www3.imperial.ac.uk/mechatronicsinmedicine/projects/roboticsforurology/seedbasedprostatebrachytherapy/roboticsconcepts). However, none of these devices is currently available commercially due to their complexity and lack of regulatory approval for clinical use. Additionally, they are designed only for percutaneous needle insertion, and most have been designed specifically to treat tumors located in the prostate. In order to implant brachytherapy seeds into lung tumors using the ZEUS robot, we developed a device that can be attached to any of the arms of the ZEUS robotic system Citation[21]. The surgeon uses the robotic manipulator and real-time thoracoscopic images of the agar cube and target to position the tip of the needle. The device is then used to deploy the dummy seeds as close as possible to the target (). When compared to percutaneous needle penetration, this method is novel in that it allows the position and orientation of the needle to be adjusted inside the patient's body prior to penetrating the target tissue, thereby allowing better access to organs by avoiding obscured access due to the rib cage. In addition, direct visualization of vital structures through the use of an endoscope allows the procedure to be carried out in a safer manner and minimizes seed misplacement caused by tissue shift. The remote activation of the needle retraction relative to the plunger avoids exposure of the clinician to the radiation source. The ZEUS system used in conjunction with the injector device performed well, and was able to deploy seeds consistently.
The accuracy when trying to reach the target with the tip of the needle (error rate) was determined by measuring the distance from the centers of the seeds to the centers of the targets. This distance measurement includes the radii of both the seed and the target. We measured and estimated a theoretically perfect result (i.e., the closest distance when the seed actually touches the target) to be 1.18 mm. We elected not to correct for this and have reported the raw data as is. The manual and VATS techniques were able to achieve this perfect result while the ZEUS system came within 0.18 mm. The manual technique, as expected, was consistently the most accurate method (smallest error). This technique simulated open surgery by allowing unlimited degrees of freedom in needle and phantom manipulation and 3D direct visualization of the target for needle insertion. The accuracy of the VATS technique was slightly superior to that obtained using the ZEUS system, but by only 1.2 mm. This difference is less than our a priori equivalency estimate of 2 mm.
The number of attempts recorded during each of the trials simulated the number of punctures needed to position the brachytherapy needle within the lung tissue according to the dosimetry plan. The manual technique was the least traumatic, requiring fewer needle punctures to implant the seeds in the agar. The ZEUS method was slightly superior to the VATS technique in this respect, requiring one less attempt on average. The ZEUS robot provided more stability and consistency in the movements with reduced tremor compared to the VATS technique.
The ZEUS robot required significantly more time to deploy the seeds than the manual approach, but the robot was faster than the VATS technique by 22 s on average. The minimum time required to hit the target using the ZEUS robot was only 5 s, but occasionally approximately 6 min was required. In practice, these time differences are unlikely to be clinically significant.
There are a few limitations with the ZEUS system. Although the injector device worked well in deploying seeds and establishing a proof of principle, we found it to be too heavy (at 970 g), and the friction within the components was too high. Modifications are underway to reduce the overall weight, size, and internal friction of the instrument. As anticipated, the manual technique was significantly superior to both the ZEUS robot and the VATS techniques. The lack of depth perception, the limited screen resolution, and the location of the screen relative to the instruments all contributed to reduced performance when implementing the ZEUS and VATS techniques. It was challenging to direct the needle toward the target using only 2D visualization. The subjects often guessed the trajectory and the location of the needle with respect to the target, and a trial-and-error approach seemed more important than skill. The more meaningful comparison of the ZEUS robot and the VATS techniques demonstrated that the robot was superior with respect to the time required and the number of attempts needed to deposit the seeds. This is consistent with the mechanical advantages of using a robotic system.
It was surprising that the accuracy achieved when using the ZEUS robot was somewhat less than that obtained with the VATS approach. Possible explanations for this include a longer than anticipated learning curve and a differential enabling effect. Although each of the four users trained on the ZEUS robot to obtain a level of comfort and confidence with the technique, a post hoc analysis demonstrated a continued learning effect, as shown in . Comparing the first and second halves of the experimental series, all subjects showed improvements in completion time and the number of attempts required, but there was no improvement in accuracy. It is possible that with more practice the accuracy would also improve.
Table IV. Effect of training with the ZEUS robot. The latter halves of the experimental series show a reduction in the number of attempts and mean time required. There was no significant improvement in accuracy.
We assumed the experienced thoracic surgeons would acquire the robotic skills at a faster rate than the non-surgeons. However, the ZEUS system is an enabling technology that varies with different users, and this differential enabling effect could allow less-experienced non-surgeons to acquire the skills more quickly. Our analysis supports this, as we found there was no difference between the two surgeons and the two non-surgeons in the number of attempts and time required (). The surgeons were, however, statistically more accurate than the non-surgeons by 1.2 mm (p = 0.048).
Table V. Comparison of surgeons versus non-surgeons using the ZEUS robot. There was no significant difference in the number of attempts and time required with respect to the level of training. However, surgeons were significantly more accurate than non-surgeons.
The experiments presented in this report used a model that allowed the target to be visualized. In clinical surgery, however, the tumor is often below the lung surface and cannot be seen. It is anticipated that image guidance and navigation techniques will improve the performance of the ZEUS robotic system for lung brachytherapy. This was not addressed in these experiments, but will be the subject of future investigations.
It should be noted that Computer Motion, Inc., the manufacturer and initial distributor of the ZEUS system, was acquired by its competitor Intuitive Surgical, Inc., in 2003, and the ZEUS robotic platform is no longer available for clinical use. Therefore, while telesurgical brachytherapy seed insertion has been established in this first-generation surgical robot, future work will require modifications of the injector and instruments for use with other robotic systems.
In conclusion, insertion of brachytherapy needles using a manual technique was found to be the most accurate, least traumatic (requiring fewer insertion attempts), and fastest method of placing seeds into tumors. However, the ZEUS robotic platform was able to place seeds beside a target within a clinically acceptable distance and amount of time. The median number of attempts was acceptable, but needs improvement. Nevertheless, the ZEUS robot has the potential to achieve results equal to or better than those obtained with VATS insertion.
Acknowledgments
This project was supported by the Canada Foundation for Innovation (National Centre for Minimally Invasive Robotic Surgery Project # 3088), Ontario Innovation Trust, and Ontario Research and Development Challenge Fund under Grant # 00-May-0709. The authors would like to thank David Browning and David Harrison for their technical support, Aida Kashigar, and the rest of the staff at Canadian Surgical Technologies & Advanced Robotics for their valuable support.
References
- Parkin DM, Pisani P, Ferlay J. Estimates of the worldwide incidence of 25 major cancers in 1990. Int J Cancer 1999; 80(6)827–841
- Martinez-Monge R, Garran C, Vivas I, Lopez-Picazo JM. Percutaneous CT-guided 103Pd implantation for the medically inoperable patient with T1N0M0 non-small cell lung cancer: A case report. Brachytherapy 2004; 3(3)179–181
- Brach B, Buhler C, Hayman MH, Joyner LRJ, Liprie SF. Percutaneous computed tomography-guided fine needle brachytherapy of pulmonary malignancies. Chest 1994; 106(1)268–274
- Imamura F, Chatani M, Nakayama T, Uda H, Nakamura S, Horai T. Percutaneous brachytherapy for small-sized non-small cell lung cancer. Lung Cancer 1999; 24(3)169–174
- Ricke J, Wust P, Wieners G, Hengst S, Pech M, Lopez HE, Felix R. CT-guided interstitial single-fraction brachytherapy of lung tumors: Phase I results of a novel technique. Chest 2005; 127(6)2237–2242
- Hilaris BS, Martini N. The current state of intraoperative interstitial brachytherapy in lung cancer. Int J Radiat Oncol Biol Phys 1988; 15(6)1347–1354
- Fleischman EH, Kagan AR, Streeter OE, Tyrell J, Wollin M, Leagre CA. Harvey JC. Iodine125 interstitial brachytherapy in the treatment of carcinoma of the lung. J Surg Oncol 1992; 49(1)25–28
- Tomberlin JK, Halperin EC, Kusin P, Leopold K, Bentel G, George S, Powers M, Wolfe W. Endobronchial interstitial Au-198 implantation in the treatment of recurrent bronchogenic carcinoma. J Surg Oncol 1992; 49(4)213–219
- Buckingham RA, Buckingham RO. Robots in operating theatres. BMJ 1995; 311(7018)1479–1482
- Munz Y, Moorthy K, Dosis A, Hernandez JD, Bann S, Bello F, Martin S, Darzi A, Rockall T. The benefits of stereoscopic vision in robotic-assisted performance on bench models. Surg Endosc 2004; 18(4)611–616
- Corcione F, Esposito C, Cuccurullo D, Settembre A, Miranda N, Amato F, Pirozzi F, Caiazzo P. Advantages and limits of robot-assisted laparoscopic surgery: Preliminary experience. Surg Endosc 2005; 19(1)117–119
- d'Amato TA, Galloway M, Szydlowski G, Chen A, Landreneau RJ. Intraoperative brachytherapy following thoracoscopic wedge resection of stage I lung cancer. Chest 1998; 114(4)1112–1115
- Fernando HC, Santos RS, Benfield JR, Grannis FW, Keenan RJ, Luketich JD, Close JM, Landreneau RJ. Lobar and sublobar resection with and without brachytherapy for small stage IA non-small cell lung cancer. J Thoracic Cardiovascular Surg 2005;129(2):261–267.
- Lee W, Daly BD, DiPetrillo TA, Morelli DM, Neuschatz AC, Morr J, Rivard MJ. Limited resection for non-small cell lung cancer: Observed local control with implantation of I-125 brachytherapy seeds. Ann Thoracic Surg 2003; 75(1)237–242
- Pisch J, Belsley SJ, Ashton R, Wang L, Woode R, Connery C. Placement of 125I implants with the da Vinci robotic system after video-assisted thoracoscopic wedge resection: A feasibility study. Int J Radiat Oncol Biol Phys 11-1-2004;60(3):928–932.
- Birdas TJ, Koehler RP, Colonias A, Trombetta M, Maley RH, Jr, Landreneau RJ, Keenan RJ. Sublobar resection with brachytherapy versus lobectomy for stage Ib nonsmall cell lung cancer. Ann Thoracic Surg 2006; 81(2)434–438
- Chen A, Galloway M, Landreneau R, d'Amato T, Colonias A, Karlovits S, Quinn A, Santucci T, Kalnicki S, Brown D. Intraoperative 125I brachytherapy for high-risk stage I non-small cell lung carcinoma. Int J Radiat Oncol Biol Phys 1999; 44(5)1057–1063
- American College of Surgeons Oncology Group. Z4032 study: A randomized Phase III study of sublobar resection versus sublobar resection plus brachytherapy in high risk patients with non-small cell lung cancer (NSCLC), 3 cm or smaller. https://www.acosog.org/studies/organ_site/thoracic/index.jsp.
- Hornblower VD, Yu E, Fenster A, Battista JJ, Malthaner RA. 3D thoracoscopic ultrasound volume measurement validation in an ex vivo and in vivo porcine model of lung tumours. Phys Med Biol 2007; 52(1)91–106
- Trejos AL, Patel RV, Malthaner RA. A device for robot-assisted minimally-invasive lung brachytherapy. Proceedings of the 2006 IEEE International Conference on Robotics and Automation, Orlando, FL, May 2006. pp 4187–4192.
- Trejos AL, Aaronson NK, Lin AW, Pytel MP, Patel RV, Malthaner RA. Robot-assisted minimally invasive lung brachytherapy. Int J Med Robotics Comput Assist Surg 2007; 3: 44–55
- Rasband W, National Institute of Mental Health. ImageJ. http://rsb.info.nih.gov/ij/docs/index.html.
- Rosner, B, editor. Fundamentals of Biostatistics. 5th edition. Pacific Grove, CA: Duxbury Press; 2000.
- Hoskins MW. Brachytherapy instrument and methods. US Patent Number 6,752,753.
- Wei Z, Gardi L, Downey DB, Fenster A. Automated localization of implanted seeds in 3D TRUS images used for prostate brachytherapy. Med Phys 2006; 33(7)2404–2417
- Wei Z, Ding M, Downey D, Fenster A. 3D TRUS guided robot assisted prostate brachytherapy. In: Duncan JS, Gerig G, editors. Proceedings of 8th International Conference on Medical Image Computing and Computer-Assisted Intervention (MICCAI 2005), Palm Springs, CA, October 2005. Part II. Lecture Notes in Computer Science 3750. Berlin: Springer; 2005. pp 17–24.
- Wei Z, Wan G, Gardi L, Mills G, Downey D, Fenster A. Robot-assisted 3D-TRUS guided prostate brachytherapy: System integration and validation. Med Phys 2004; 31(3)539–548
- Stoianovici D, Cadeddu JA, Demaree RD, Basile SA, Taylor RH, Whitcomb LL, Sharpe WN Jr, Kavoussi LR. A novel mechanical transmission applied to percutaneous renal access. Proceedings of ASME Dynamic Systems and Control Division 1997;DSC-61:401–406.
- Cleary K, Freedman M, Clifford M, Lindisch D, Onda S, Jiang L. Image-guided robotic delivery system for precise placement of therapeutic agents. J Control Release 2001; 74(1–3)363–368
- Yanof J, Bauer C, Wood B. Tactile feedback and display system for CT-guided, robot-assisted percutaneous procedures. In: Lemke HU, Vannier MW, Inamura K, Farman AG, Doi K, Reiber DHC, editors. Computer Assisted Radiology and Surgery. Proceedings of the 18th International Congress and Exhibition (CARS 2004), Chicago, IL, June 2004. Amsterdam: Elsevier; 2004. pp 521–526.
- Hong J, Dohi T, Hashizume M, Konishi K, Hata N. An ultrasound-driven needle-insertion robot for percutaneous cholecystostomy. Phys Med Biol 2004; 49(3)441–455