Abstract
A new type of carbonic anhydrase inhibitors was identified via differential scanning fluorimetry (DSF) screening. The compounds displayed interesting inhibition profile against human carbonic anhydrase isoforms I, II, IX and XII with an obvious selectivity displayed by one compound toward carbonic anhydrase (CA) IX, an established anti-cancer target. A hypothetical mechanism of inhibitory action by the Strecker-type α-aminonitriles has been proposed.
Introduction
Differential scanning fluorimetry (DSF) or thermal shift assay (TSA) is an established method to directly determine if a small molecule has affinity to a protein targetCitation1. It is based on the ability of a small molecule binding to the protein to stabilize or destabilize the tertiary structure of the latter and thus cause a positive or a negative shift in its melting temperature (ΔTm), respectively. With the aim of establishing a robust, high-throughput DSF screening program, we selected bovine carbonic anhydrase (bCA) as the protein target and performed screening of an unbiased, chemically diverse set of 8000 compounds selected from the Enamine screening collection comprising over 2 000 000 compoundsCitation2. This led, unexpectedly, to the discovery of three compounds that produced noticeable thermal shift of bCA Tm, while not belonging to any of the known classes of CA inhibitors. In this “Preliminary Communication”, we report the details of these findings and further characterization of the new CA inhibitory chemotype.
Materials and methods
Chemical compounds
The chemical compounds for screening were obtained directly from the Enamine screening collectionCitation2. The three compounds identified as bCA binders (1Citation3, 2Citation4 and 3Citation5) are known compounds (see Supplementary material for their 1H NMR spectra). Their identity and purity was additionally confirmed by 1H NMR spectroscopy prior to biochemical testing in CA inhibition assay.
Differential scanning fluorimetry (thermal shift) assay
Thermal shift assay was carried out using ViiA™ 7 Real-Time PCR System equipped with 384-well block (Applied Biosystems, Waltham, MA). The TSA procedure was adopted from the literatureCitation6–8 and was modified in order to allow measuring the bCA melting temperature on interaction with various compounds, including the known CA inhibitor azetazolamide (AZ)Citation9, which was used in this study as a reference bCA binder at 10 and 20 μM concentration.
For 8000-compound screening, the test reactions were set up in the following buffer: 10 mM NaH2PO4/Na2HPO4, pH 7.0, 10 mM NaCl. The total volume of the reaction mixture per well was 10 μL. Carbonic anhydrase (Sigma Aldrich Cat# C3934, St. Louis, MO) in 300 μg/mL concentration was pre-mixed with environment-sensitive SYPRO orange dye (Invitrogen, Cat# S6650, Waltham, MA) at final concentration in the reaction of 10×, with regard to the stock concentration stated by the vendor. The mixtures were pre-incubated for 1 h at 4 °C with 20 μM concentrations of the compounds (and 1% final concentration of DMSO), placed into MicroAmp® Optical 384-Well Reaction Plate (Thermo Fisher Scientific, Cat# 4309849, Waltham, MA). The reaction mixture was kept at room temperature for 15 min to ensure full protein-compound interactions. The temperature was raised at 1.6 °C/s rate to 40 °C without signal reading. Starting from 40 °C up to 85 °C the heating rate was set to 0.05 °C/s with constant fluorescence reading, using 470/623 nm filter set. The raw data of dye fluorescence intensity change upon melting of the protein were obtained from the instrument ViiA 7 RUO software (Thermo Fisher Scientific, Waltham, MA). Further data processing and visualization was performed by custom-made Microsoft Excel scripts (Redmond, WA). The peak of the first derivative for the fluorescence curve was used to define melting temperature Tm. Tm for DMSO control wells, having only protein, dye and 1% DMSO was used as a To to determine melting temperature shifts (ΔTm). All measurements were made in quadruplicates. For the control experiments KCN (Enamine) and vitamin B12 (Pharmaceutical firm Darnitsa, Kiev, Ukraine) were used. KCN was added at the compound addition step to DMSO control wells at the final concentrations in the reaction 1.25–40 μM to test the amino nitriles model of action. Vitamin B12 was added along with compounds at the final concentration equaling half that of the compounds 1 and 2.
Carbonic anhydrase inhibition assay
An Applied Photophysics stopped-flow instrument (Leatherhead, UK) was used for assaying the CA catalyzed CO2 hydration activityCitation10. Phenol red (at a concentration of 0.2 mM) was used as an indicator, working at the absorbance maximum of 557 nm, with 20 mM Hepes (pH 7.5) as a buffer, and 20 mM Na2SO4 (for maintaining constant ionic strength), following the initial rates of the CA-catalyzed CO2 hydration reaction for a period of 10–100 s. The CO2 concentrations ranged from 1.7 to 17 mM for the determination of the kinetic parameters and inhibition constants. For each inhibitor at least six traces of the initial 5–10% of the reaction have been used for determining the initial velocity. The non-catalyzed rates were determined in the same manner and subtracted from the total observed rates. Stock solutions of inhibitor (0.1 mM) were prepared in distilled-deionized water and dilutions up to 0.01 nM were done thereafter with the assay buffer. Inhibitor and enzyme solutions were combined and pre-incubated for 15 min at room temperature prior to running the assay, in order to allow for the formation of the ezyme–inhibitor complex. The inhibition constants were obtained by non-linear least-squares methods using PRISM 3 (GraphPad Software, La Jolla, CA) and the Cheng–Prusoff equation, as reported earlierCitation11, and represent the mean from at least three different determinations. All CA isoforms were recombinant ones obtained in-house as reported earlierCitation12–16.
Results and discussion
Relying on the robust protocol for DSFCitation7, we established the screening platform using AZ which produced strong (>5 °C) thermal shift of bCA Tm at 10 and 20 μM concentrations (). Several thermal shifters identified in the screening of the 8000-compounds set comprising mostly primary sulfonamides. We were pleased to see our assay to reliably identify these compounds akin to reference AZ. However, this was of lesser interest as this chemical class are well-studied CA inhibitors where the SO2NH2 moiety acts as a zinc-binding group (ZBG)Citation17. What caught our attention, however, were the three Strecker-type α-aminonitriles 1–3 () also identified as thermal shift hits. Following the screening, these compounds were scrutinized at different concentrations in comparison with AZ and were found indeed to produce dose-dependently increasing thermal shift of bCA Tm which was highest (1.5–2 °C) at 40 μM and noticeably lower than that produced by 10 μM concentration of acetazolamide ().
Figure 1. Melting thermal shift of bovine CA produced by acetazolamide (AZ), a known CA inhibitor (shown in fluorescence/T, °C (left) or derivative fluorescence/T, °C (right) coordinates).
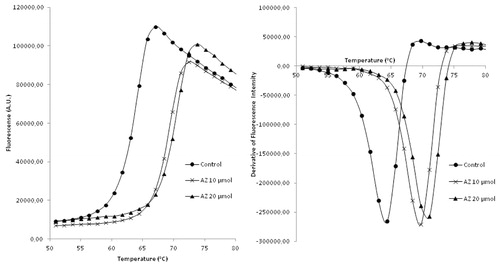
Figure 2. Structures of three Strecker-type α-aminonitriles (1–3) identified as CA Tm shifters in this study.
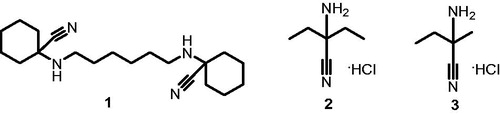
Figure 3. Melting thermal shift of bovine CA produced by compounds 1 (a), 2 (b), 3 (c) in comparison with that of acetazolamide.
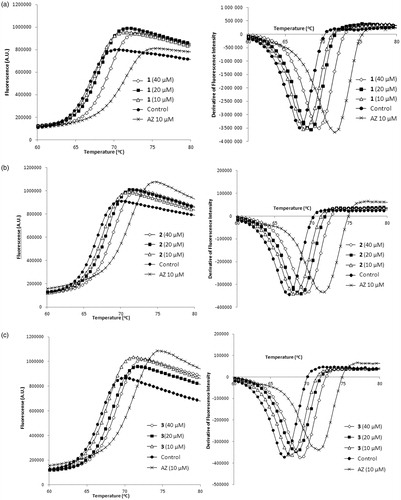
Strecker-type α-aminonitriles such as 1–3 have not been reported in the literature although they could be considered as related to N-cyanomethylsulfonamides reported as hCA (h = human) inhibitors by Winnum et alCitation18. We were therefore interested to see if the preliminary indication of affinity to bCA established for compound 1–3 using DSF would translate into inhibition of enzymatic activity of various isoforms of hCA. To our delight, all of these compounds exhibited submicromolar to micromolar inhibitory activity against hCA I, II, IX and XII (). Particularly noticeable is the selectivity displayed by compound 3 toward hCA IX, which is a membrane-bound isoform of the enzyme and an established anti-cancer targetCitation19.
Table 1. Inhibition profile of compounds 1–3 against hCA I, II, IX and XII (h = human).
Considering the structural novelty of CA inhibitors 1–3, understanding the mechanism of their inhibitory action is of high importance. Cyanide is a metal poison and has been shown to inhibit carbonic anhydrase by coordinating to the prosthetic zinc ion (and replacing the zinc-coordinated water which forms a hydrogen bond with Thr199 in the native structure)Citation20. Therefore, first of all, we needed to rule out a possibility that the observed DSF thermal shifts and the biochemical inhibition were due to an adventitious cyanide that could be present in minute amounts in the samples of products of the Strecker reaction – either from the reaction itself or due to the reversibility of the reactionCitation21. To this end, we measured the melting temperatures of bCA in presence of increasing concentrations of potassium cyanide. It was established that at concentrations as high as 40 μM, the changes in the melting temperature were negligible (). Therefore, the 1.5–2 °C shift of bCA Tm produced by the same concentrations of compounds 1–3 was most likely due to the compounds’ specific affinity to the enzyme. Furthermore, for compounds 1 and 2 taken in 40 μM concentrations, the thermal shift values were negligibly influenced by the addition of vitamin B12 which is a known scavenger of cyanide anions ()Citation22.
Figure 4. Melting thermal shifts of bovine CA produced by various concentrations of KCN in comparison with that produced by AZ.
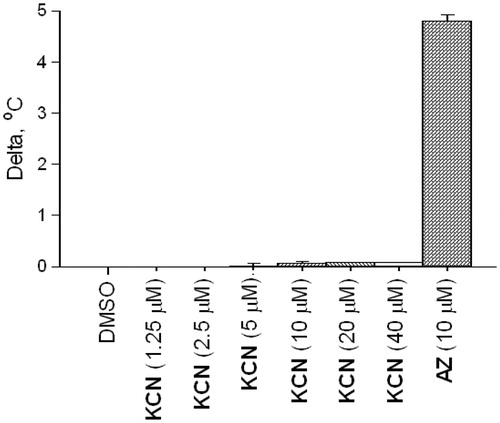
Figure 5. Melting thermal shifts of bovine CA produced by compounds 1 and 2 (40 μM) with or without addition of vitamin B12.
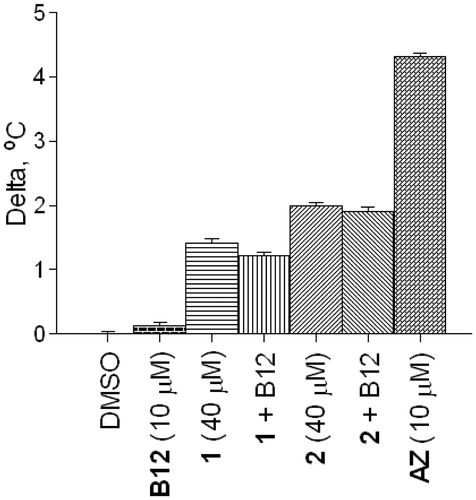
In our view, compounds 1–3 may still exhibit their inhibitory action toward CA acting as cyanide anion donors. They clearly have an affinity toward carbonic anhydrases, as was established by DSF experiments. This affinity itself may not be sufficient to cause the observed levels of biochemical inhibition () but it most likely ensure higher effective concentration of the inhibitor in the proximity of the active site, where the release of the cyanide can be triggered by the interaction with the zinc ion. Similarly to cyanide introduced externally, the cyanide thus generated in situ would replace the water from the tetracoordinate CA native stateCitation20. The inhibitory action thus exerted could be further potentiated by the possible interaction of the imine (or iminium) portion of the Strecker-type inhibitor with the nearby Thr199, which was hydrogen bound through said water in the native state (Scheme 1). Should this be the case, inhibitors 1–3 could be regarded as suicide inhibitors similar to N-cyanosulfonamides reported by Supuran et alCitation23.
Conclusion
Using DSF (thermal shift assay) screening platform, we identified a novel type of ligands (1–3) for bovine carbonic anhydrase. We further confirmed these compounds’ exhibiting interesting inhibition profile on human CA I, II, IX and XII isoforms with one compounds (3) displaying an obvious selectivity toward CA IX, which is an established anti-cancer target. We demonstrated that the thermal shift observed had not been due to adventitious cyanide that could be present in Strecker-type products. We proposed a hypothetical mechanism of action for these compounds, according to which they might indeed act as in situ cyanide donors activated by the zinc ion and even suicide inhibitors due to the electrophilicity of the imine portion of the Strecker compound thus released. Further studies are underway which aim to survey a larger body of Strecker-type α-aminonitriles and verify the mechanistic hypothesis put forward in this “Preliminary Communication”.
Supplementary material available online
IENZ_1156676_SupplementaryMaterial.pdf
Download PDF (3 MB)Acknowledgement
This research was supported by the Russian Scientific Fund (project grant 14–50-00069).
Declaration of interest
The authors declare no conflict of interest. The authors are solely responsible for the content and results presented in this paper.
References
- Cummings MD, Farnum MA, Nelen MI. Universal screening methods and applications of ThermoFluor®. J Biomol Screen 2006;11:854–63
- The screening and the building block collection of Enamine Ltd. Available from: https://www.enaminestore.com/catalog [last accessed 20 Jan 2016]
- Mai K, Patil G. A fast N-substituted α-aminonitrile synthesis. Synth Commun 1985;15:157–63
- Zali-Boeini H, Mobin M, Hajibabaei K, Ghani M. Approaches to the construction of substituted 4-amino-1H-pyrrol-2(5H)-ones. J Org Chem 2012;77:5808–12
- Deng S, Liu D. Studies on phosphoroheterocycle chemistry II: a simple and new route to 1,3,2-diazaphospholidine-4-thione 2-sulfide derivatives. Synthesis 2001;2445–9
- Lo MC, Aulabaugh A, Jin G, et al. Evaluation of fluorescence-based thermal shift assays for hit identification in drug discovery. Anal Biochem 2004;332:153–9
- Matulis D, Kranz JK, Salemme FR, Todd MJ. Thermodynamic stability of carbonic anhydrase: measurements of binding affinity and stoichiometry using ThermoFluor. Biochemistry 2005;44:5258–66
- Niesen FH, Berglund H, Vedadi M. The use of differential scanning fluorimetry to detect ligand interactions that promote protein stability. Nat Protoc 2007;2:2212–21
- Alterio V, Di Fiore A, D’Ambrosio K, et al. Multiple binding modes of inhibitors to carbonic anhydrases: how to design specific drugs targeting 15 different isoforms? Chem Rev 2012;112:4421–68
- Khalifah RG, Edsall JT. Carbon dioxide hydration activity of carbonic anhydrase: kinetics of alkylated anhydrases B and C from humans. Proc Natl Acad Sci U S A 1972;69:172–6
- Krasavin M, Korsakov M, Dorogov M, et al. Probing the ‘bipolar’ nature of the carbonic anhydrase active site: aromatic sulfonamides containing 1,3-oxazol-5-yl moiety as picomolar inhibitors of cytosolic CA I and CA II isoforms. Eur J Med Chem 2015;101:334–47
- Maresca A, Temperini C, Vu H, et al. Non-zinc mediated inhibition of carbonic anhydrases: coumarins are a new class of suicide inhibitors. J Am Chem Soc 2009;131:3057–62
- Temperini C, Innocenti A, Scozzafava A, et al. The coumarin-binding site in carbonic anhydrase accommodates structurally diverse inhibitors: the antiepileptic lacosamide as an example and lead molecule for novel classes of carbonic anhydrase inhibitors. J Med Chem 2010;53:850–4
- Alterio V, Hilvo M, Di Fiore A, et al. Crystal structure of the catalytic domain of the tumor-associated human carbonic anhydrase IX. Proc Natl Acad Sci U S A 2009;106:16233–8
- Casey JR, Morgan PE, Vullo D, et al. Carbonic anhydrase inhibitors. Design of selective, membrane-impermeant inhibitors targeting the human tumor-associated isozyme IX. J Med Chem 2004;47:2337–47
- Casini A, Scozzafava A, Mincione F, et al. Carbonic anhydrase inhibitors: water-soluble 4-sulfamoylphenylthioureas as topical intraocular pressure-lowering agents with long-lasting effects. J Med Chem 2000;43:4884–92
- Winum JY, Scozzafava A, Montero JL, Supuran CT. Design of zinc binding functions for carbonic anhydrase inhibitors. Curr Pharm Des 2008;14:615–21
- Winnum JY, Cecchi A, Seridi A, et al. Carbonic anhydrase inhibitors. N-Cyanomethylsulfonamides – a new zinc binding group in the design of inhibitors targeting cytosolic and membrane-anchored isoforms. J Enzyme Inhib Med Chem Med 2006;21:477–81
- McDonald PC, Winum JY, Supuran CT, Dedhar S. Recent developments in targeting carbonic anhydrase IX for cancer therapeutics. Oncotarget 2012;3:84–97
- De Simone G, Supuran CT. (In)organic anions as carbonic anhydrase inhibitors. J Inorg Biochem 2012;111:117–29
- Opatz T. The chemistry of deprotonated α-aminonitriles. Synthesis 2009;1941–59
- Sauer S, Keim M. Hydroxocobalamin: improved public health readiness for cyanide disasters. Improved health for disasters. Ann Emerg Med 2001;37:635–41
- Supuran CT, Scozzafava A, Briganti F. Carbonic anhydrase inhibitors: N-cyanosulfonamides, a new class of high affinity isozyme II and IV inhibitors. J Enzyme Inhib 1999;14:289–306