Abstract
Humanized tumor mice (HTM) were generated by the co-transplantation of human hematopoietic stem cells and human breast cancer cells overexpressing HER2 into neonatal NOD-scid IL2Rγnull (NSG) mice. These mice are characterized by the development of a human immune system in combination with human breast cancer growth. Due to concurrent transplantation into newborn mice, transfer of MHC-mismatched tumor cells resulted in solid coexistence and immune cell activation (CD4+ T cells, natural killer cells, and myeloid cells), but without evidence for rejection. Histological staining of the spleen of HTM revealed co-localization of human antigen-presenting cells together with human T and B cells allowing MHC-dependent interaction, and thereby the generation of T cell-dependent antibody production. Here, we investigated the capability of these mice to generate human tumor-specific antibodies and correlated immunoglobulin titers with tumor outgrowth. We found detectable IgM and also IgG amounts in the serum of HTM, which apparently controlled tumor development when IgG serum concentrations were above 10 µg/ml. Western blot analyses revealed that the tumor-specific antibodies generated in HTM did not recognize HER2/neu antigens, but different, possibly relevant antigens for breast cancer therapy. In conclusion, HTM offer a novel approach to generate complete human monoclonal antibodies that do not require further genetic manipulation (e. g., humanization) for a potential application in humans. In addition, efficacy and safety of the generated antibodies can be tested in the same mouse model under human-like conditions. This might be of particular interest for cancer subtypes with no currently available antibody therapy.
Introduction
Antibody-based therapies are highly specific and powerful tools for individualized (personalized) treatment of a variety of leukemic and solid malignancies.Citation1,Citation2 For example, antibody therapy is a well established component for the treatment of HER2 receptor positive breast cancer patients using monoclonal humanized trastuzumab (Herceptin®) or pertuzumab (Perjeta®). However, only 20% of invasive breast cancers overexpress HER2 and are therefore eligible for an antigen-specific treatment.Citation3,Citation4 Furthermore, ~50% of the treated patients combat against de-novo or acquired resistance.Citation5 Therefore, new breast cancer-specific antibodies not restricted to HER2 are needed. The generation of a wide range of therapeutic antibodies targeting different tumor antigens will allow cancer treatment regimens to best benefit each individual patient.
Since the invention of hybridoma technology by Köhler and MilsteinCitation6 and the technological advancements of genetic engineering, an enormous variety of therapeutic monoclonal antibodies (mAbs) have been evaluated in human clinical trials. Many of these antibodies are generated in mice and thereafter genetically modified to engineer human-mouse chimeras or “humanized” antibodies. However, the antibodies still retain murine sequences that could cause human anti-mouse antibody responses (HAMA)Citation7,Citation8 or human anti-chimeric antibody responses (HACA).Citation9,Citation10These anti-Ig reactions reduce the half-life of the molecules and can induce fatal side effects.Citation11 In addition, humanization of immunoglobulins often results in reduced antibody affinity, specificity or functionality.Citation12-Citation15
Here, we investigated the potential of the humanized tumor mouse (HTM) modelCitation16,Citation17 to develop novel tumor-specific antibodies. HTM are characterized by the development of a human immune system and the growth of human cancer cells forming solid tumors or tumor cell effusions following co-transplantation of hematopoietic stem cells and breast cancer-derived tumor cells. Simultaneous transplantation of hematopoietic stem cells and tumor cells has been previously described by our groupCitation16 and can be considered as an extension of the generation of NSG-based humanized mice, which have been used for a number of analyses.Citation18,Citation19 The co-transplantation of human hematopoietic stem cells together with cancer cells enables the co-existence of MHC-mismatched cells without inducing rejection.
Nevertheless, the human immune system becomes activated once the presence of tumor cells is detected through immune surveillance by effector cells such as natural killer (NK) and CD4+ T-helper cells. Notably, an increase in human CD4+ T cells activation and maturation is observed in the HTM model.Citation16
Instead of using the common strategies to vaccinate with pre-defined antigens, HTM are able to identify new antigenic targets. Furthermore, the generated antibody secreting human B cells from HTM can be fused with plasmacytoma cell lines to expand the monoclonal immunoglobulins. Antibodies generated and propagated this way do not require genetic modification (with the risk of reduced affinity or altered function) and do not induce strong human anti-Ig responses. Finally, those mAbs with the greatest potential in vitro (apoptosis induction, inhibition of proliferation) can be tested in different tumor-bearing HTM under human-like conditions.
Results
Humanized tumor mice (HTM) combine human tumor outgrowth and the development of a human immune system
Transplantation of human CD34+ hematopoietic stem cells (HSC) together with human breast cancer cell lines (BT474 or SK-BR-3) induced macroscopic BT474 tumor growth in the liver () or tumor cell effusion (SK-BR-3) in the peritoneal cavity, respectively. HER2 and cytokeratin-7 (CK7) staining () revealed the breast cancer cell origin. In addition, tumor dissemination, especially into the lung (BT474 and SK-BR-3) but also in the brain (SK-BR-3), was detectable by histological immunostainings of HER2 (). Furthermore, SK-BR-3 tumor cells also disseminated in various other organs (liver, testis, and peritoneum; data not shown).
Figure 1. Tumor development and metastases in HTM. (A) Tumor growth and vascularization in the liver of BT474 transplanted HTM three months post transplantation. Human breast cancer origin was proven by immunohistochemical staining for HER2 (B) and Cytokeratin-7 (C) in tumors of HTM. SK-BR-3 transplanted HTM showed tumor cell dissemination in lung (D) and brain (E) stained with anti-HER2 antibody. Bars indicating the corresponding size (10, 100 µm) are shown for each specimen.
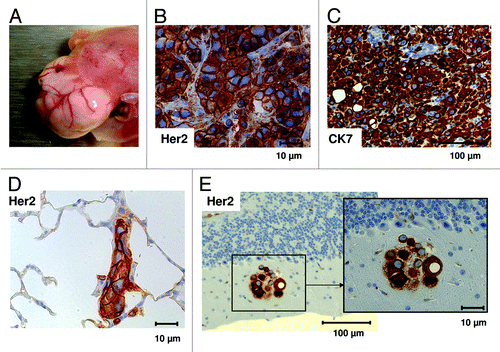
The co-transplantation of HSC into the liver of newborn NSG mice resulted in the generation of a human immune system with human immune cells migrating into different organs (; Fig. S1). All HTM were analyzed for the presence of human hematopoietic cells (CD45+) between 15 and 36 wk post-transplant using flow cytometry () and histological analyses (; Fig. S1). The reconstitution levels and fractions of cell subsets were nearly identical in animals transplanted with SK-BR-3 and BT474, respectively. The average reconstitution level was 51% (+/− 3.7 SEM) in the spleen, 44% (+/− 4.6 SEM) in the bone marrow (bm), and 88% (+/− 3.5 SEM) in the mesenteric lymph nodes. Further characterization of immune cell subsets revealed that the majority of immune cells in the bone marrow and spleen were CD19+ human B cells whereas the lymph nodes were mainly repopulated by CD3+ human T cells. Furthermore nearly all HTM (92.8%; n = 13/14) developed human myeloid cells (CD33+) especially in the bone marrow ().
Figure 2. Human immune cell engraftment in different organs of HTM. (A) Flow cytometric analyses of the expression of human leukocyte common antigen (CD45+) detected the percentage of human leucocytes in spleen, bone marrow (bm) and lymph nodes (ln) of SK-BR-3 and BT474 transplanted HTM. (B) Immune cell populations were further characterized by flow cytometry and percentage of B cells (CD19+), T cells (CD3+), and myeloid cells (CD33+) of spleen, bm, and ln of CD45+ cells are presented in one graph. Numbers of animals are indicated in brackets.
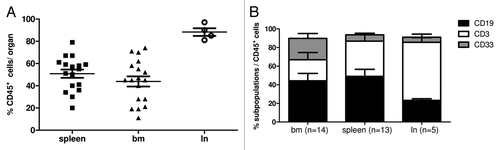
Figure 3. Human immune cell co-localization in the spleen of HTM. Histological staining for CD4 expression in the spleen of BT474 transplanted HTM presented in different magnification (top row). Further staining for macrophages (CD68+), cytotoxic T cells (CD8+), and B cells (CD79A+) of HTM spleens are represented. All scale bars represent 100 µm.
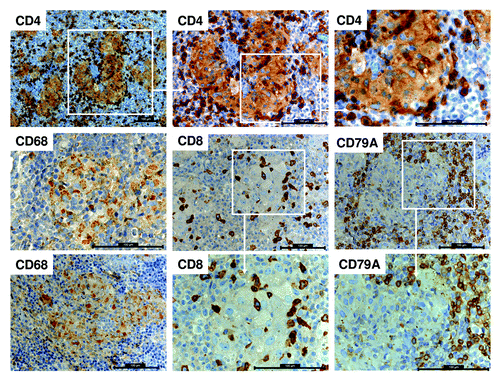
Human immune cell distribution in the spleen of HTM
Human antigen-presenting cells, e.g., macrophages (CD68+), mature in HTM and migrate into the spleen where they form clusters (). Human T (CD4+ and CD8+) and B cells (CD19+) were found to co-localize to areas of macrophage accumulations (). A small number of effector cells were also found within the CD68+ clusters. Interestingly, the co-receptor CD4 is also expressed on CD68+ macrophages similar to the situation in humans (, top row).
Human B cells in HTM appear positive for CD79A ( and ), CD19 (flow cytometric analyses; data not shown) and CD20 (). In HTM, developed B cells were also able to form clusters similar in appearance to B cell follicles (). The maturation of human B cells toward antibody-producing plasma cells in the spleen was proven by histological staining of immunoglobulin kappa constant (IGKC) protein expression ().
Figure 4. Human B cell maturation in the spleen of HTM. Histological sections of the spleen of BT474 transplanted HTM (A) show B cell cluster formation (CD79A+) and a positive staining of the CD20 antigen. (B) The staining of Immunoglobulin kappa constant (IGKC) protein indicates the presence of antibody producing plasma cells. Bars indicating the corresponding sizes (20, 100, 1000 µm) are shown for each specimen.
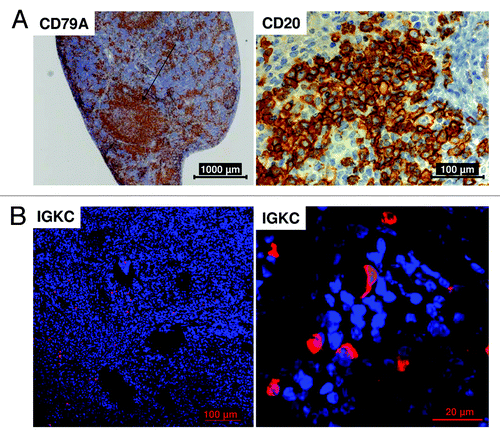
Immunoglobulin production in HTM
Fifteen to 36 wk post co-transplantation serum of HTM was analyzed by ELISA. Independent of SK-BR-3 or BT474 co-transplantation, HTM had detectable levels of IgM (32+/− 3.9 µg/ml (SEM); ) and IgG (31.9 +/− 22 µg/ml (SEM); ). Highest levels of IgG concentration were found after 16 wk in SK-BR-3 and after 21 wk in BT474 transplanted HTM. Antibody production in SK-BR-3 transplanted mice was obviously not capable of controlling tumor growth and thereby ascites formation occurred. However, high IgM and IgG titers apparently prevent tumor outgrowth in BT474 based HTM (circles in ).
Figure 5. HTM are able to generate human IgM and IgG antibodies. Total IgM (A) and IgG (B) concentration in sera of HTM (BT474 and SK-BR-3) were analyzed by ELISA. Tumor antigen-specific antibody generation in HTM was analyzed on tumor-lysate coated ELISA plates and analyzed in SK-BR-3 transplanted HTM (C) and BT474 transplanted HTM (F). Values are given as arbitrary unit [AI] of optical densities (OD). BT474 transplanted HTM were further subdivided into animals with and without tumor development and the corresponding IgM (D) and IgG (E) concentration for each mouse is displayed. The percentage of mice which did not have detectable tumor growth at the end of the experiments in BT474 transplanted HTM was calculated for all mice dependent on the IgM (G; above or below 20 µg/ml) or IgG concentration (H; above or below 10 µg/ml). (I) Kaplan–Meier survival curves are shown for BT474 transplanted HTM.
![Figure 5. HTM are able to generate human IgM and IgG antibodies. Total IgM (A) and IgG (B) concentration in sera of HTM (BT474 and SK-BR-3) were analyzed by ELISA. Tumor antigen-specific antibody generation in HTM was analyzed on tumor-lysate coated ELISA plates and analyzed in SK-BR-3 transplanted HTM (C) and BT474 transplanted HTM (F). Values are given as arbitrary unit [AI] of optical densities (OD). BT474 transplanted HTM were further subdivided into animals with and without tumor development and the corresponding IgM (D) and IgG (E) concentration for each mouse is displayed. The percentage of mice which did not have detectable tumor growth at the end of the experiments in BT474 transplanted HTM was calculated for all mice dependent on the IgM (G; above or below 20 µg/ml) or IgG concentration (H; above or below 10 µg/ml). (I) Kaplan–Meier survival curves are shown for BT474 transplanted HTM.](/cms/asset/fa138f1e-8d26-4309-9fa8-884d22a023a2/kmab_a_10929111_f0005.gif)
To characterize tumor-specific antibody production, HTM serum was tested on ELISA plates alternatively coated with SK-BR-3 or BT474 lysates. In 3/10 SK-BR-3 () and 5/13 BT474 () transplanted mice, tumor antigen-specific antibody titers were measurable. Both BT474-transplanted mice with the highest titers had detectable levels of IgG (average of 3.5 or 3.8 µg/ml). There was, however, no correlation between three SK-BR-3 HTM with the highest titers to their corresponding total IgG concentration (0 µg/ml, 0 µg/ml, 253 µg/ml IgG, respectively).
Most SK-BR-3 transplanted HTM developed the first signs of ascites at the age of 3 mo. The experiments were stopped and sera were analyzed when ascites was clearly visible and before the effusion caused severe suffering of the mice. Only 3/10 mice exhibited notable signs of malaise, which was not correlated with their Ig levels. Only one of 13 BT474-transplanted mice developed signs of sickness, whereas all other animals remained healthy even when massive tumors became apparent at the end of the experiments (). Tumor-free survival in BT474 HTM tended to improve when serum IgM (>20 µg/ml; ) and IgG (>10 µg/ml; ) levels were increased. Due to the early time point of analysis (without signs of sickness) no significant differences in overall survival of BT474 () and SK-BR-3 transplanted HTM (data not shown) were observed.
Different antigens were recognized by the antibodies generated in HTM
To characterize binding specificity of the HTM antibodies, sera were also analyzed using western blot technology. Protein lysates derived from cell lines used for xenograft transplantation (BT474, SK-BR-3) were probed.
In 14 of 23 BT474-transplanted mice and 8 of 11 SK-BR-3-transplanted mice, specific antibodies capable of binding to denatured blotted cellular proteins were detected. Notably, the strongest signals were not detectable on the ~185 kDa HER2/neu antigen that is recognized by the anti-HER2 antibody.
Instead, a number of different antigens were detected, especially in BT474 transplanted HTM (), whereas a reduced pattern was observed in SK-BR-3 mice (). To further differentiate tumor-specific and unspecific binding, sera of HTM were also tested on non-tumorigenic mammary epithelial cell lines (HME, MCF-10A) and a human, normal fibroblast cell line (N1) derived from the adult skin of a healthy donor (Fig. S2). All three tested sera of BT474 HTM (B2, B4, BX) detected antigens on BT474 lysates that were not expressed in any of the three control cell lines (different antigens in the size between 100–150 kDa and one ~60 kDa antigen). Tumor cell line specific binding that did not appear on control cell lines are marked with arrows (; Fig. S2). The SK-BR-3 transplanted HTM S5 showed binding to different antigens that were also expressed on the control cell lines (Fig. S2).
Figure 6. HTM generated antibodies that recognize a variety of antigens. BT474 and SK-BR-3 lysates were separated on a SDS-PAGE, transferred to a PVDF membrane and stained with serum of BT474 transplanted (B1–7) and SK-BR-3 transplanted HTM (S1–7), respectively. A polyclonal anti-HER2 antibody was used as a positive control (HER2). Non-humanized tumor mice (TM) served as negative controls (NC). The annotation “St.” refers to the lane of control proteins with standardized size. Tumor-specific antibodies were visualized by anti-Ig detection antibody (A and B) or anti-IgG detection antibody (C). BT474 HTM without detectable tumor are marked with red circles (B2, B3). Tumor cell line specific binding that did not appear on control cell lines are marked with arrows (A and C). (D) Quantification of bands was performed using the Image Quant software package. Each band contributes to the total value by individual thickness and intensity. (E) Western blot units are shown for SK-BR-3 and BT474 HTM divided into animals with or without tumor outgrowth.
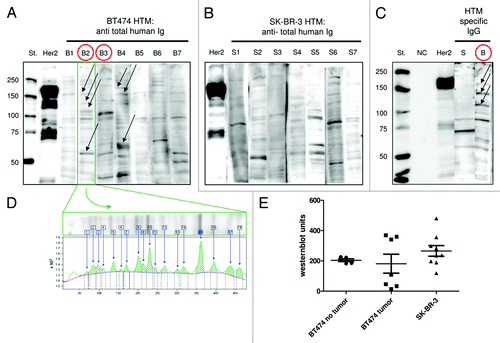
Prevention of tumor outgrowth was observed in two of the animals (circles in ). Tumor-antigen specific IgG antibodies were traceable in four of 13 BT474 and one of 11 SK-BR-3 mice, which are exemplarily shown in . Western blot units for each individual animal were calculated (). The analysis revealed that BT474 HTM without tumor development had similar units (; average 204 +/− 9 SEM) whereas HTM with tumor outgrowth built two clusters: one with (non-protective) high western blot units (356 +/− 8 SEM) and one with nearly no detectable tumor specific antibodies (50 +/− 20 SEM). SK-BR-3 HTM generated overall higher antibody titers (; Western blot units 265 +/− 35 SEM) that did not control ascites formation. In all experiments, tumor mice without human immune system (TM) served as controls and never displayed any signals in ELISA or western blots analyses.
Discussion
A major advantage of therapeutic antibodies in cancer treatment is their target cell specificity, which, in contrast to conventional systemic therapeutic intervention (e.g., chemotherapy, radiation), minimizes severe side effects. MAbs are able to impair tumor growth in different ways: cell proliferation is inhibited by blocking mitogenic signaling, and apoptosis is directly induced or indirectly triggered by antibody-dependent cell-mediated cytotoxicity or complement-dependent lysis related mechanisms. Therefore, the generation of a broad range of new antibodies with anti-tumorigenic capacity will extend and enhance treatment options for cancer patients.
To evaluate the potential of tumor cell co-transplanted humanized mice to generate novel cancer specific human antibodies, we analyzed serum from BT474 and SK-BR-3 transplanted HTM. These mice developed a human immune system together with human tumor growth and revealed immune cell stimulation as evident by increased NK and CD4+ helper cell activation.Citation16 Detailed characterization of HTM performed in this study revealed the production of tumor-specific IgM and IgG antibodies with different antigen specificity. Antibody generation in HTM apparently controlled tumor outgrowth when IgG concentration in serum was found to be above 10 µg/ml. In addition, the frequency of myeloid cells (CD33+) was higher in HTM compared with humanized mice. Notably, antigen-presenting cells (APCs), i.e., macrophages (CD68+), were enriched in the spleen of HTM forming clusters. These human macrophages also express CD4, which is believed to be involved in macrophage differentiation and increased ability to induce T cell activation.Citation22 The improved development and maturation of myeloid cells might be predominantly triggered by granulocyte (macrophage) colony-stimulating factor (G(M)-CSF), a phenomenon that has been observed to enhance myeloid cell populations in humanized mice. The injection of G-CSF,Citation23 a bolus-administration of GM-CSF and IL-4,Citation24 or the administration of human GM-CSF (in combination with stem cell factor (SCF), IL-3 or IL-4) to transgenic mice all caused an increase of myeloid cell populations.Citation25-Citation28 GM-CSF is not cross-reactive between mouse and human,Citation29 but can be produced by cells such as activated T cells.Citation30 Thus, activated human T-helper cells in HTM might trigger macrophages enrichment by the release of GM-CSF. Moreover, a release of stimulating cytokines might additionally be triggered by the co-transplanted human breast cancer cells.Citation31-Citation35
The induction of human APCs, its co-localization with human T and B effector cells, the activation of Th cells and the presence of plasma cells in the spleen of HTM implies an interaction of these cell types similar to the natural occurrence in humans. Therefore, tumor-specific antibody production, as well as Ig class switch, could occur in HTM. Cytokines, which are needed for enhanced myeloid formation (GM-CSF, IL-4), also enhance B cell maturation and Ig class switch.Citation24 Additional stimulation with IL-15/IL-15R are promising approaches to further trigger antibody production and Ig class switch toward antigen-specific IgG in HTM.Citation36 In fact, immune (e.g., NK) cell activation by IL-15/IL-15R in HTM has been described before, but this was done without further identification and characterization of antibody production.Citation16 Another option to promote Ig generation and IgG maturation in humanized mice or HTM might be the substitution of human IL-12, which has been shown to stimulate the production of anti-HER2 specific antibodies in humanized BALB/c-Rag2−/−Il2rg−/−.Citation37
Overall, the study we present here suggests that HTM have a marked capacity to produce human antibodies, even though the ability of human B cells to produce antigen-specific antibodies in humanized mice has been controversially discussed previously. On one hand, impaired B cell maturation in these mice,Citation38 as well as a limited production of human IgM and a defective isotype class switch toward IgG, has been reported.Citation39-Citation44 On the other hand, others describe an eminent human-like antibody repertoire (V, D, J genes)Citation45,Citation46 and the production of neutralizing antibodies against Dengue virusCitation47 or Borrelia hermsii in humanized mice.Citation38
IgM concentrations between 119–600 µg/ml and IgG amounts between 1–257 µg/ml in immunized humanized mice have been reported elsewhere.Citation48 Based on the experimental protocol we used in this study, HTM generated similar Ig concentration without any further immune stimulation (0–121 µg/ml IgM or 0–305 µg/ml IgG). Notably, the co-transplantation with HER2-positive breast cancer cells produced detectable levels of tumor-specific, but not HER2-related antibodies; nevertheless, some of the animals were able to control tumor outgrowth. Conceivably, co-transplantation of primary tumor cells into HTM might result in the generation of patient specific antibodies with anti-tumorigenic capacity.
Western blot technology enabled the visualization of the antigenic binding capacity of HTM antibodies. Some antigens were specifically expressed by the tumor cell lines and not by the control cells (HME, MCF-10A, N1), and therefore the antibodies that bind them can be considered tumor specific. Nevertheless, the antibodies that bind antigens expressed on normal tissue should be further tested to avoid the exclusion of possible therapeutically relevant antibodies. For example, trastuzumab recognizes a common antigen (HER2) expressed in normal (breast) cells, but is potentially overexpressed in breast cancer cells. Further evaluation of the generated antibodies (e.g., detection of native proteins using methods such as flow cytometry), functional studies (e.g., in vitro apoptosis assay, proliferation inhibition assay), and finally the characterization of the antigen (and epitope) will be necessary to select the most promising antibodies, which can then be tested in vivo using HTM.
In summary, isolation of IgG producing plasma cells derived from HTM and their propagation by the hybridoma techniqueCitation6 might be a promising approach to generate human mAbs with therapeutic, anti-tumorigenic capacity. This could be achieved without further IgG genetic engineering (humanization / chimerization). In parallel to this study, we fused splenic B cells from tetanus-toxoid immunized humanized mice with a human plasmocytoma cell line (Karpas 707).Citation49 Using this approach, we have cleared the first hurdle toward isolating a monoclonal tetanus-toxoid specific human IgM antibody with therapeutic capacity (unpublished data). In conclusion, HTM allow (1) the maturation of novel tumor-specific antibodies, (2) analysis of anti-tumorigenic functionality during the process of generation, and (3) testing of ex vivo generated mAbs under human-like conditions using the same mouse model.
Materials and Methods
Mice
Mice (NOD-scid IL2Rγnull; (NSG) used for the experiments were obtained from The Jackson Laboratory (005557) and bred and kept in a specified pathogen-free facility at the University of Regensburg. For the generation of humanized tumor mice (HTM), the animals were irradiated (1 Gy) and 3 h later transplanted with either 3x106 BT474 or SK-BR-3 tumor cells together with 3x105 human CD34+ cells isolated from umbilical cord blood* (CB) using immunomagnetic beads (Miltenyi Biotech 130–046–702) according to the manufacturer’s protocol. To generate control tumor mice (TM), either 3x106 BT474 or SK-BR-3 cells were transplanted. In all experiments, cells were co-transplanted into the liver of newborn mice. Animals were sacrificed and analyzed between 15 and 36 wk post transplantation.
All animal work was approved by the local veterinary authorities from the district government based on the European guidelines and national regulations of the German animal protection act (permission no. 54–2532.1–13/09 and 54–2532.1–27/11). *Cord blood samples were taken with approval from the Ethics Committee of the University of Regensburg (permission no. 11–101–0287). All patients included in the experiments provided written informed consent. All experiments were performed in accordance with relevant institutional and national guidelines, regulations and approvals.
Cell lines
BT474 or SK-BR-3 breast cancer cells were used for cotransplantation. The BT474 cell line was isolated by Lasfargues and Coutinho from a solid, invasive ductal carcinoma of the breast (ATCC #HTB-20). The SK-BR-3 cell line, first described by Trempe and Old, was derived from a pleural effusion (ATCC #HTB-30). Authenticity of both cell lines was approved by the German Collection of Microorganisms and Cell Cultures. Non-tumorigenic mammary epithelial cell lines MCF-10A (ATCC #CRL-10317) and HME (ATCC #CRL-4010) were kindly provided by Prof. Christoph Klein (Dpt. of Experimental Medicine and Therapy Research, University of Regensburg). Additionally, a human normal fibroblast cell line (N1) derived from the adult skin of a healthy donor was used for control purposes.Citation50
Mononuclear cell isolation and serum preparation
Mononuclear cells (MNC) were isolated from different mouse tissues as previously described.Citation16,Citation20 Briefly, MNC from bone marrow were isolated from femurs by rinsing with 10 ml of PBS + 2 mM EDTA using a 27G needle syringe (BD Bioscience, 302200). Spleen and mesenteric lymph nodes were passed through a 40 µm cell strainer (BD Bioscience, 352340) and rinsed with 20 ml PBS + 2 mM EDTA. The resulting MNC suspensions were centrifuged at 300x g at 4 °C for 10 min and analyzed by flow cytometry.
For serum collection, peripheral blood was taken retrobulbarly from anesthetized mice, clotted for 30 min at 4 °C and centrifuged at 1200x g for 10 min at 4 °C. The resulting serum was stored at –80 °C.
Flow cytometric analysis
Reconstitution with human immune cells was determined by flow cytometry analysis using an LSR-II flow cytometer and Diva software package (BD Biosciences). To reduce non-specific binding, cells were incubated with mouse serum (1%) on ice for 20 min before staining. Samples were stained using the following mAbs: anti-CD3-FITC (clone HIT3a, BD Biosciences 555332), anti-CD19-PE (clone HIB19, BD Biosciences 555413), anti-CD33-PerCP-eFluor® 710 (clone P67.6; eBioscience 9046–0337–120) and anti-CD45-APC (clone HI30, BD 555485). Appropriate mouse antibodies of respective isotypes were used as controls for all stainings.
Immunohistochemistry
Immunohistochemistry was performed as previously described.Citation16 Briefly, tissue specimens (spleen, liver, brain, lymph nodes, lung) were fixed with 4% formalin, embedded in paraffin and stained automatically by a Ventana Nexes autostainer (Ventana) using the streptavidin-biotin-horseradish peroxidase complex method and 3,3′-diaminobenzidine as chromogen. The autostainer was programmed based on the instructions given by the iView DAB detection kit (Ventana, 760–091). The following antibodies were used: anti-CD3 (clone SP7, Thermo Fisher Scientific Inc., RM-9107-S), anti-CD79a, (clone JCB117, Dako, M 7018), anti-CK7 (clone OV-TL-12/30, Dako, M7018), anti-CD45/LCA (clone 2B11-PD7/26, Dako, M 0701), anti-CD68 (clone P6M1, Dako, M 0876), anti-HER2 rabbit polyclonal (Dako, A0485), and anti-CD20 (clone L26, Dako, M 0755). The following antibodies were used from Ventana (Roche): anti-CD4 (clone SP35, Ventana, 790–4423), anti-CD8 (clone SP57, Ventana, 790–4460).
All antibodies are designated as human specific (no cross-reaction to mice antigens) by the manufacturers. Nevertheless, we tested and verified their specificity using tumor mice without human immune cells and humanized mice without co-transplanted tumor cells. In general, the same positive controls as used in routine diagnostic were used in this study.
Immunofluorescence of IGKC
Immunohistochemical localization of IGKC producing cells was performed as described previously.Citation21 Tissue sections were deparaffinized by washing in Rotihistol (Carl-Roth, 6640) followed by hydration in a descending ethanol gradient (100%, 95%, 90%,70%, 50%, and 30% ethanol for 5 min each). Tissue sections were then boiled twice in a microwave oven, 7 min each, in 0.01 M citrate buffer (Carl-Roth, 3580; pH 6.0). After washing 3 times in 1xPBS, the tissue sections were incubated for 2 h with 3% BSA, 0.1% Tween20. Subsequently, the tissue sections were incubated overnight at 4 °C with rabbit anti-kappa light chain antibody (Abcam, ab76837, 1:100 dilution) to visualize IGKC. The incubation period was followed by three subsequent washing steps in 1x PBS at room temperature for 10 min each. The tissue sections were then incubated with secondary antibodies labeled with fluorescent dyes: IGKC was visualized using Cy5-labeled donkey anti-rabbit (Dianova, 711–175–152; 1:200 dilution). Both primary and secondary antibodies were diluted in 0.3% BSA, 0.1% Tween20 in 1x PBS. Then, tissue sections were washed in 1xPBS and nuclei were visualized using 4',6-diamidino-2-phenylindole (DAPI; Life Technologies, D1306). The slides were mounted using Entellan (Merck, 107961) and were stored in the dark at room temperature until further analysis. Image analysis was performed using a confocal microscope (Olympus FV-1000). All series included appropriate positive and negative controls.
Western blot
BT474 or SK-BR-3 tumor cell lines (in vitro cultures) were lysed in 1x cell lysis buffer containing Triton X-100 (SIGMA T8787) and 20 µg protein/lane were separated on a 7.5% SDS-PAGE under reducing conditions (mercaptoethanol), and blotted onto PVDF membranes. Subsequently, membranes were blocked in 5% low-fat milk and incubated in 1:6 diluted HTM serum overnight. For control, serum from non-humanized tumor mice (TM) was used. For positive control, one lane was incubated with anti-HER2 (mouse) antibody [clone 3B5, Calbiochem (Merck), OP15–100UG]. For the detection the following antibodies were used: rabbit anti-human immunoglobulin HRP (SIGMA, A8794), rabbit anti-human IgG (BD Bioscience, 555788), anti-mouse Ig (Cell signaling, 7076S), and anti-biotin HRP [BIORAD, 161–0747)]. The Precision Plus Protein Western C Standard marker (BIORAD, 161–0376) was used to determine the molecular weights of the separated proteins. The blots were then visualized using the enhanced chemiluminescent western blotting detection system (GE Healthcare, ECL RPN2209) and analyzed by ImageQuant LAS 4000mini imager. Western blot bands of each sample were quantified using ImageQuant software (Ver. 7.0, GE Healthcare) by which intensity of IgG reactions is expressed in relative and arbitrary units. Serum of non-humanized TM was used as control.
ELISA
For detection of antigen-specific antibodies 96-well plates were coated with tumor cell extracts. Protein extracts were prepared by incubating 107 scraped SK-BR-3 or BT474 cells, in 20 mM TRIS-HCl, pH 7.5, 150 mM NaCl, 1 mM EDTA, 1% Triton containing 1x protease inhibitor cocktail complete (Roche Diagnostics, 05892953001), diluted in 50 mM Na-carbonate buffer, pH 9.6, to 10 µg/ml and immobilized onto 96-well plates (Medisorp, Nunc, 467320) at 4 °C overnight. Plates were washed three times with PBS-T (phosphate-buffered saline, 154 mM NaCl, 0.05% Tween 20) and blocked with 1x Blocking Reagent (Roche Diagnostics, 11112589001) for 1 h at room temperature. Mice sera were diluted 1:50 in PBS-T, loaded in duplicate (100 µl/well) onto the wells, serially diluted (1:50 to 1:3,200) and incubated overnight at 4 °C. After washing three times the bound IgG or IgM antibodies were detected with AffiniPure F(ab)2 fragment goat anti-human IgG, Fcγ-fragment specific HRP (Jackson ImmunoResearch, 109–036–098) and AffiniPure F(ab)2 fragment goat anti-human IgM, Fcµ-fragment specific-HRP (Jackson ImmunoResearch, 109–036–129), respectively, and using soluble tetramethylbenzidine (TMB-E; DUNN Labortechnik) as substrate solution.
Total serum levels of human IgG and IgM were quantified using an in-house Sandwich-ELISA as follows. AffiniPure goat anti-human IgG (H+L) (Jackson ImmunoResearch Europe, 109–005–088) was used as capture reagent for both IgM and IgG quantification. ChromePure human IgG(Jackson ImmunoResearch, 009–000–003), whole molecule, and ChromePure Human IgM (myeloma) (Jackson ImmunoResearch, 009–000–012), whole molecule, were serially diluted in a range from 50 ng/ml to 98 pg/ml in washing buffer PBS-T (phosphate buffered saline, 300 mM NaCl, 0.05% Tween 20) to establish a calibration curve. Detection was performed using AffiniPureF(ab)2 fragment goat anti-human IgG, Fcγ fragment specific HRP (Jackson ImmunoResearch, 109–036–098) and AffiniPureF(ab)2 fragment goat anti-human IgM (Jackson ImmunoResearch, 109–036–129), Fcµ fragment specific-HRP using TMB-E (Dunn Labortechnik) as substrate solution. For blocking after coating 0.5% casein in PBS-T was used. Each sample was tested in duplicate.
Abbreviations: | ||
HTM | = | humanized tumor mice |
TM | = | tumor mice |
HER2 | = | human epidermal growth factor receptor 2 |
NK | = | natural killer |
Ig | = | immunoglobulin |
Th cells | = | T helper cells |
CB | = | cord blood |
CK7 | = | cytokeratin-7 |
HSC | = | hematopoietic stem cells |
IGKC | = | immunoglobulin kappa constant |
bm | = | bone marrow |
ln | = | lymph nodes |
Additional material
Download Zip (2.1 MB)Disclosure of Potential Conflicts of Interest
No potential conflicts of interest were disclosed.
Funding Acknowledgment
This work was supported by the Deutsche Forschungsgemeinschaft (grant number: WE 3606/2–1) and the Fraunhofer Gesellschaft.
Acknowledgments
We thank Prof. L. Shultz for providing access to NSG mice and Prof. O. Kölbl (Dpt. of Radiotherapy, Regensburg) for giving access to the linear accelerators. We are especially grateful to Dr. Nancie Archin (University of North Carolina at Chapel Hill School of Medicine, USA) for proof-reading the manuscript and M. Siebörger and Ulrike Scholz for excellent assistance.
References
- Harris M. Monoclonal antibodies as therapeutic agents for cancer. Lancet Oncol 2004; 5:292 - 302; http://dx.doi.org/10.1016/S1470-2045(04)01467-6; PMID: 15120666
- Dalle S, Thieblemont C, Thomas L, Dumontet C. Monoclonal antibodies in clinical oncology. Anticancer Agents Med Chem 2008; 8:523 - 32; http://dx.doi.org/10.2174/187152008784533071; PMID: 18537534
- Hudis CA. Trastuzumab--mechanism of action and use in clinical practice. N Engl J Med 2007; 357:39 - 51; http://dx.doi.org/10.1056/NEJMra043186; PMID: 17611206
- Slamon DJ, Clark GM, Wong SG, Levin WJ, Ullrich A, McGuire WL. Human breast cancer: correlation of relapse and survival with amplification of the HER-2/neu oncogene. Science 1987; 235:177 - 82; http://dx.doi.org/10.1126/science.3798106; PMID: 3798106
- Lan KH, Lu CH, Yu D. Mechanisms of trastuzumab resistance and their clinical implications. Ann N Y Acad Sci 2005; 1059:70 - 5; http://dx.doi.org/10.1196/annals.1339.026; PMID: 16382045
- Köhler G, Milstein C. Continuous cultures of fused cells secreting antibody of predefined specificity. Nature 1975; 256:495 - 7; http://dx.doi.org/10.1038/256495a0; PMID: 1172191
- Sgro C. Side-effects of a monoclonal antibody, muromonab CD3/orthoclone OKT3: bibliographic review. Toxicology 1995; 105:23 - 9; http://dx.doi.org/10.1016/0300-483X(95)03123-W; PMID: 8638282
- Khazaeli MB, Conry RM, LoBuglio AF. Human immune response to monoclonal antibodies. J Immunother Emphasis Tumor Immunol 1994; 15:42 - 52; http://dx.doi.org/10.1097/00002371-199401000-00006; PMID: 8110730
- Hwang WY, Foote J. Immunogenicity of engineered antibodies. Methods 2005; 36:3 - 10; http://dx.doi.org/10.1016/j.ymeth.2005.01.001; PMID: 15848070
- Brüggemann M, Winter G, Waldmann H, Neuberger MS. The immunogenicity of chimeric antibodies. J Exp Med 1989; 170:2153 - 7; http://dx.doi.org/10.1084/jem.170.6.2153; PMID: 2584938
- Mirick GR, Bradt BM, Denardo SJ, Denardo GL. A review of human anti-globulin antibody (HAGA, HAMA, HACA, HAHA) responses to monoclonal antibodies. Not four letter words. Q J Nucl Med Mol Imaging 2004; 48:251 - 7; PMID: 15640788
- Nakanishi T, Tsumoto K, Yokota A, Kondo H, Kumagai I. Critical contribution of VH-VL interaction to reshaping of an antibody: the case of humanization of anti-lysozyme antibody, HyHEL-10. Protein Sci 2008; 17:261 - 70; http://dx.doi.org/10.1110/ps.073156708; PMID: 18227432
- Nakanishi T, Maru T, Tahara K, Sanada H, Umetsu M, Asano R, Kumagai I. Development of an affinity-matured humanized anti-epidermal growth factor receptor antibody for cancer immunotherapy. Protein Eng Des Sel 2013; 26:113 - 22; http://dx.doi.org/10.1093/protein/gzs088; PMID: 23118340
- Queen C, Schneider WP, Selick HE, Payne PW, Landolfi NF, Duncan JF, Avdalovic NM, Levitt M, Junghans RP, Waldmann TA. A humanized antibody that binds to the interleukin 2 receptor. Proc Natl Acad Sci U S A 1989; 86:10029 - 33; http://dx.doi.org/10.1073/pnas.86.24.10029; PMID: 2513570
- Yoon SO, Lee TS, Kim SJ, Jang MH, Kang YJ, Park JH, Kim KS, Lee HS, Ryu CJ, Gonzales NR, et al. Construction, affinity maturation, and biological characterization of an anti-tumor-associated glycoprotein-72 humanized antibody. J Biol Chem 2006; 281:6985 - 92; http://dx.doi.org/10.1074/jbc.M511165200; PMID: 16407221
- Wege AK, Ernst W, Vollmann-Zwerenz A, Männel DN, Ortmann O, Kroemer A, Brockhoff G. Humanized tumor mice--a new model to study and manipulate the immune response in advanced cancer therapy. Int J Cancer 2011; 129:2194 - 206; http://dx.doi.org/10.1002/ijc.26159; PMID: 21544806
- Wege AK, Schardt K, Schaefer S, Brockhoff G, Jung EM. High resolution ultrasound including elastography and contrast-enhanced ultrasound (CEUS) for early detection and characterization of liver lesions in the humanized tumor mouse model. Clin Hemorheol Microcirc 2012; 52:93 - 106; PMID: 22975935
- Brehm MA, Jouvet N, Greiner DL, Shultz LD. Humanized mice for the study of infectious diseases. Curr Opin Immunol 2013; 25:428 - 35; http://dx.doi.org/10.1016/j.coi.2013.05.012; PMID: 23751490
- Macchiarini F, Manz MG, Palucka AK, Shultz LD. Humanized mice: are we there yet?. J Exp Med 2005; 202:1307 - 11; http://dx.doi.org/10.1084/jem.20051547; PMID: 16301740
- Ernst W, Zimara N, Hanses F, Männel DN, Seelbach-Göbel B, Wege AK. Humanized mice, a new model to study the influence of drug treatment on neonatal sepsis. Infect Immun 2013; 81:1520 - 31; http://dx.doi.org/10.1128/IAI.01235-12; PMID: 23439310
- Schmidt M, Micke P, Gehrmann M, Hengstler JG. Immunoglobulin kappa chain as an immunologic biomarker of prognosis and chemotherapy response in solid tumors. Oncoimmunology 2012; 1:1156 - 8; http://dx.doi.org/10.4161/onci.21653; PMID: 23170262
- Kitchen S, Krutzik S, Levin B, Rezek V, Kitchen C, Zack J. Ligation of CD4 on human blood monocytes triggers macrophage differentiation. J Immunol 2011; 186:158
- Tanaka S, Saito Y, Kunisawa J, Kurashima Y, Wake T, Suzuki N, Shultz LD, Kiyono H, Ishikawa F. Development of mature and functional human myeloid subsets in hematopoietic stem cell-engrafted NOD/SCID/IL2rγKO mice. J Immunol 2012; 188:6145 - 55; http://dx.doi.org/10.4049/jimmunol.1103660; PMID: 22611244
- Chen Q, He F, Kwang J, Chan JK, Chen J. GM-CSF and IL-4 stimulate antibody responses in humanized mice by promoting T, B, and dendritic cell maturation. J Immunol 2012; 189:5223 - 9; http://dx.doi.org/10.4049/jimmunol.1201789; PMID: 23089398
- Chen Q, Khoury M, Chen J. Expression of human cytokines dramatically improves reconstitution of specific human-blood lineage cells in humanized mice. Proc Natl Acad Sci U S A 2009; 106:21783 - 8; http://dx.doi.org/10.1073/pnas.0912274106; PMID: 19966223
- Billerbeck E, Barry WT, Mu K, Dorner M, Rice CM, Ploss A. Development of human CD4+FoxP3+ regulatory T cells in human stem cell factor-, granulocyte-macrophage colony-stimulating factor-, and interleukin-3-expressing NOD-SCID IL2Rγ(null) humanized mice. Blood 2011; 117:3076 - 86; http://dx.doi.org/10.1182/blood-2010-08-301507; PMID: 21252091
- Nicolini FE, Cashman JD, Hogge DE, Humphries RK, Eaves CJ. NOD/SCID mice engineered to express human IL-3, GM-CSF and Steel factor constitutively mobilize engrafted human progenitors and compromise human stem cell regeneration. Leukemia 2004; 18:341 - 7; http://dx.doi.org/10.1038/sj.leu.2403222; PMID: 14628073
- Willinger T, Rongvaux A, Takizawa H, Yancopoulos GD, Valenzuela DM, Murphy AJ, Auerbach W, Eynon EE, Stevens S, Manz MG, et al. Human IL-3/GM-CSF knock-in mice support human alveolar macrophage development and human immune responses in the lung. Proc Natl Acad Sci U S A 2011; 108:2390 - 5; http://dx.doi.org/10.1073/pnas.1019682108; PMID: 21262803
- Manz MG. Human-hemato-lymphoid-system mice: opportunities and challenges. Immunity 2007; 26:537 - 41; http://dx.doi.org/10.1016/j.immuni.2007.05.001; PMID: 17521579
- Shi Y, Liu CH, Roberts AI, Das J, Xu G, Ren G, Zhang Y, Zhang L, Yuan ZR, Tan HS, et al. Granulocyte-macrophage colony-stimulating factor (GM-CSF) and T-cell responses: what we do and don’t know. Cell Res 2006; 16:126 - 33; http://dx.doi.org/10.1038/sj.cr.7310017; PMID: 16474424
- Eichbaum C, Meyer AS, Wang N, Bischofs E, Steinborn A, Bruckner T, Brodt P, Sohn C, Eichbaum MH. Breast cancer cell-derived cytokines, macrophages and cell adhesion: implications for metastasis. Anticancer Res 2011; 31:3219 - 27; PMID: 21965729
- Asano S, Urabe A, Okabe T, Sato N, Kondo Y. Demonstration of granulopoietic factor(s) in the plasma of nude mice transplanted with a human lung cancer and in the tumor tissue. Blood 1977; 49:845 - 52; PMID: 300638
- Suda T, Miura Y, Mizoguchi H, Kubota K, Takaku F. A case of lung cancer associated with granulocytosis and production of colony-stimulating activity by the tumour. Br J Cancer 1980; 41:980 - 4; http://dx.doi.org/10.1038/bjc.1980.177; PMID: 6968569
- Shijubo N, Inoue Y, Hirasawa M, Igarashi T, Mori M, Matsuura A, Uede T, Suzuki A. Granulocyte colony-stimulating factor-producing large cell undifferentiated carcinoma of the lung. Intern Med 1992; 31:277 - 80; http://dx.doi.org/10.2169/internalmedicine.31.277; PMID: 1376180
- Chavey C, Bibeau F, Gourgou-Bourgade S, Burlinchon S, Boissière F, Laune D, Roques S, Lazennec G. Oestrogen receptor negative breast cancers exhibit high cytokine content. Breast Cancer Res 2007; 9:R15; http://dx.doi.org/10.1186/bcr1648; PMID: 17261184
- Huntington ND, Alves NL, Legrand N, Lim A, Strick-Marchand H, Mention JJ, Plet A, Weijer K, Jacques Y, Becker PD, et al. IL-15 transpresentation promotes both human T-cell reconstitution and T-cell-dependent antibody responses in vivo. Proc Natl Acad Sci U S A 2011; 108:6217 - 22; http://dx.doi.org/10.1073/pnas.1019167108; PMID: 21444793
- De Giovanni C, Nicoletti G, Landuzzi L, Romani F, Croci S, Palladini A, Murgo A, Antognoli A, Ianzano ML, Stivani V, et al. Human responses against HER-2-positive cancer cells in human immune system-engrafted mice. Br J Cancer 2012; 107:1302 - 9; http://dx.doi.org/10.1038/bjc.2012.394; PMID: 22929887
- Vuyyuru R, Patton J, Manser T. Human immune system mice: current potential and limitations for translational research on human antibody responses. Immunol Res 2011; 51:257 - 66; http://dx.doi.org/10.1007/s12026-011-8243-9; PMID: 22038527
- Traggiai E, Chicha L, Mazzucchelli L, Bronz L, Piffaretti JC, Lanzavecchia A, Manz MG. Development of a human adaptive immune system in cord blood cell-transplanted mice. Science 2004; 304:104 - 7; http://dx.doi.org/10.1126/science.1093933; PMID: 15064419
- Ito R, Shiina M, Saito Y, Tokuda Y, Kametani Y, Habu S. Antigen-specific antibody production of human B cells in NOG mice reconstituted with the human immune system. Curr Top Microbiol Immunol 2008; 324:95 - 107; http://dx.doi.org/10.1007/978-3-540-75647-7_6; PMID: 18481455
- Ishikawa F, Yasukawa M, Lyons B, Yoshida S, Miyamoto T, Yoshimoto G, Watanabe T, Akashi K, Shultz LD, Harada M. Development of functional human blood and immune systems in NOD/SCID/IL2 receptor gamma chain(null) mice. Blood 2005; 106:1565 - 73; http://dx.doi.org/10.1182/blood-2005-02-0516; PMID: 15920010
- Choi B, Chun E, Kim M, Kim ST, Yoon K, Lee KY, Kim SJ. Human B cell development and antibody production in humanized NOD/SCID/IL-2Rγ(null) (NSG) mice conditioned by busulfan. J Clin Immunol 2011; 31:253 - 64; http://dx.doi.org/10.1007/s10875-010-9478-2; PMID: 20981478
- Rajesh D, Zhou Y, Jankowska-Gan E, Roenneburg DA, Dart ML, Torrealba J, Burlingham WJ. Th1 and Th17 immunocompetence in humanized NOD/SCID/IL2rgammanull mice. Hum Immunol 2010; 71:551 - 9; http://dx.doi.org/10.1016/j.humimm.2010.02.019; PMID: 20298731
- Scheeren FA, Nagasawa M, Weijer K, Cupedo T, Kirberg J, Legrand N, Spits H. T cell-independent development and induction of somatic hypermutation in human IgM+ IgD+ CD27+ B cells. J Exp Med 2008; 205:2033 - 42; http://dx.doi.org/10.1084/jem.20070447; PMID: 18695003
- Becker PD, Legrand N, van Geelen CM, Noerder M, Huntington ND, Lim A, Yasuda E, Diehl SA, Scheeren FA, Ott M, et al. Generation of human antigen-specific monoclonal IgM antibodies using vaccinated “human immune system” mice. PLoS One 2010; 5:5; http://dx.doi.org/10.1371/journal.pone.0013137; PMID: 20957227
- Ippolito GC, Hoi KH, Reddy ST, Carroll SM, Ge X, Rogosch T, Zemlin M, Shultz LD, Ellington AD, Vandenberg CL, et al. Antibody repertoires in humanized NOD-scid-IL2Rγ(null) mice and human B cells reveals human-like diversification and tolerance checkpoints in the mouse. PLoS One 2012; 7:e35497; http://dx.doi.org/10.1371/journal.pone.0035497; PMID: 22558161
- Kuruvilla JG, Troyer RM, Devi S, Akkina R. Dengue virus infection and immune response in humanized RAG2(-/-)gamma(c)(-/-) (RAG-hu) mice. Virology 2007; 369:143 - 52; http://dx.doi.org/10.1016/j.virol.2007.06.005; PMID: 17707071
- Seung E, Tager AM. Humoral immunity in humanized mice: a work in progress. J Infect Dis 2013; 208:Suppl 2 S155 - 9; http://dx.doi.org/10.1093/infdis/jit448; PMID: 24151323
- Karpas A, Dremucheva A, Czepulkowski BH. A human myeloma cell line suitable for the generation of human monoclonal antibodies. Proc Natl Acad Sci U S A 2001; 98:1799 - 804; http://dx.doi.org/10.1073/pnas.98.4.1799; PMID: 11172031
- Schuster U, Büttner R, Hofstädter F, Knüchel R. A heterologous in vitro coculture system to study interaction between human bladder cancer cells and fibroblasts. J Urol 1994; 151:1707 - 11; PMID: 8189601