Figures & data
Figure 2. A nickel-catalyzed carbozincation approach to enterolignan synthesis (THF: tetrahydrofuran; DMSO: dimethyl sulfoxide; DMF: dimethylformamide; DCM: dichloromethane; NMO: N-methylmorpholine N-oxide; PCC: pyridinium chlorochromate; LDA: lithium diisopropylamide; HMPA: hexamethylphosphoramide; rt: room temperature; er: enantiomeric ratio.
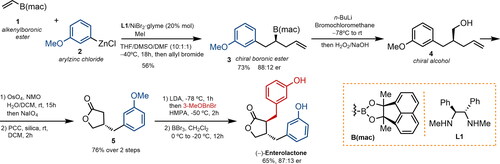
Figure 3. Asymmetric synthesis of both enantiomers of enterolactone via selective epimerization at the α- or β-position. NMO: N-methylmorpholine N-oxide; DBU: 1,8-diazabicyclo(5.4.0)undec-7-ene; DCE: 1,2-dichloroethane; MPM: methoxybenzyl, PPTS: pyridinium p-toluenesulfonate, ee: enantiomeric excess)
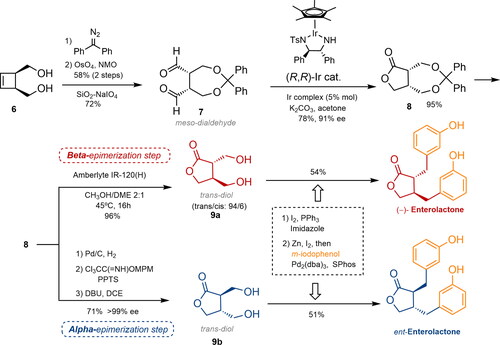
Figure 4. Yetra’s synthetic approach to enterolignans via enantioselective alpha-alkylation of carbonylic compounds with pyridinium salts. DMA, TMSCl: trimethylsilyl chloride; LiHMDS: lithium bis(trimethylsilyl)amide; THF: tetrahydrofuran; DCM: dichloromethane; dr: diastereomeric ratio; ee: enantiomeric excess.
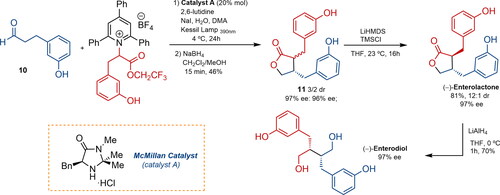
Figure 5. Enterolignan absorption and metabolism. Dietary lignans are metabolized by the gut microbiota to enterolignans (END and ENL), which can passively diffuse across the enterocyte membrane. However, a portion of these molecules undergo phase II metabolism by UDP-glucuronosyltransferases (UGT) and sulfotransferases (ST) in the enterocyte, resulting in the formation of polar and soluble conjugated metabolites (glucuronide and sulfate). These metabolites require active transport to cross the basolateral intestinal membrane for systemic distribution via the portal vein. In the hepatocyte, enterolignans can undergo phase II metabolism by UGT and ST enzymes prior to active bile transport, resulting in enterohepatic recirculation. Once in the systemic circulation, enterolignans can reach various tissues and promote biological responses. Finally, enterolignans can be eliminated in the feces (predominantly) or urine (De Silva and Alcorn Citation2019).
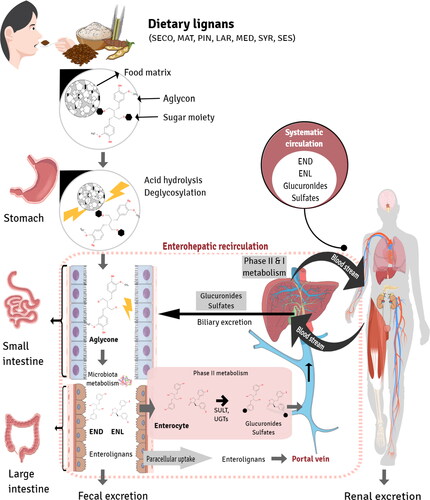
Figure 7. Flow chart depicting the different extraction and analytical methods used to study enterolignans. SPE: solid phase extraction; LLE: liquid-liquid extraction; GC: gas chromatography; MS: mass spectrometry; LC: liquid chromatography; CAD: colourimetric array detector; DAD: diode array detector.
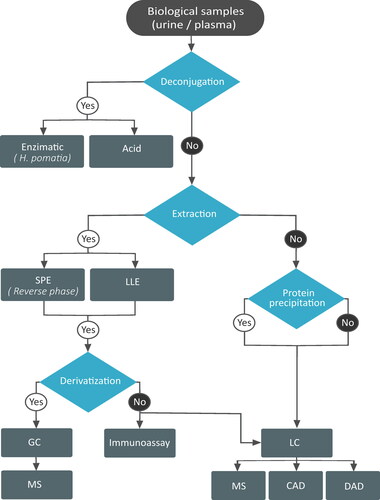