Figures & data
Figure 1. A typical graph of DMTA frequency sweep of freeze-dried broccoli powder at 0.25% compression
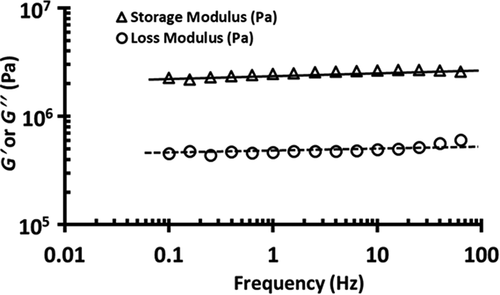
Figure 2. Storage and loss modulus of freeze-dried broccoli powder as a function of DMTA temperature ramp (frequency: 0.1 Hz, temperature: −30°C to 150°C and heating rate: 5°C/min)
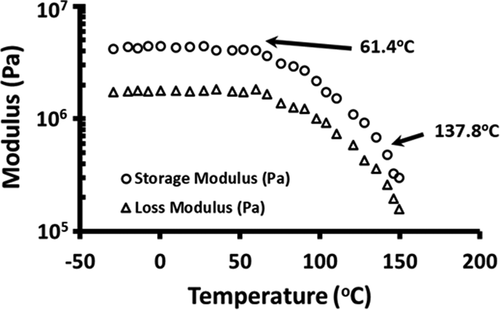
Table 1. Rate constant for vitamin C degradation as a function of temperature and moisture content
Figure 3. Typical graphs showing (a) The change in vitamin C concentration over time in Freeze-dried sample stored at −80°C, (b) Plot of ln (C/Co) versus time for freeze-dried sample stored at −80°C, (c) The change in vitamin C concentration over time in fresh sample stored at 60°C, and (d): Plot of ln (C/Co) versus time for fresh sample
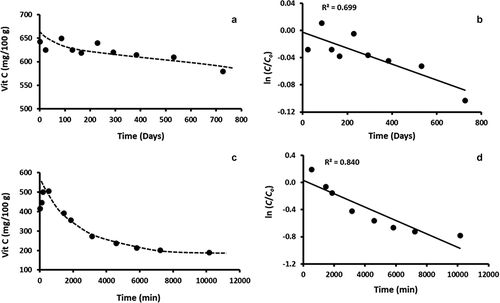
Table 2. The activation energy of the three temperature regions
Figure 4. (a) Plot of log k as a function of temperature for the fresh sample, (b) Arrhenius plot for the fresh sample, (c) Plot of log k as a function of temperature for the freeze-dried sample, (d) Arrhenius plot for the freeze-dried sample
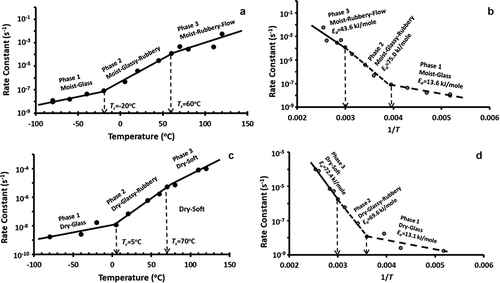
Figure 5. State diagram of broccoli (abc: freezing curve, egf glass transition line, irh: BET-monolayer, kj: solids melting-decomposition line, u: Tm′, experimental ultimate maximal-freeze-concentration melting temperature, v: Tg′′′, experimental ultimate maximal-freeze-concentration glass transition temperature, b: Xs′, conceptual maximal-freeze-concentration solids, d: Tg′, conceptual maximal-freeze-concentration glass transition temperature, m: Xs′′′, conceptual maximal-freeze-concentration solids, g: Tg′′, hypothetical maximal-freeze-concentration glass transition temperature, and 1–13: indicates the micro-regions 1–13)
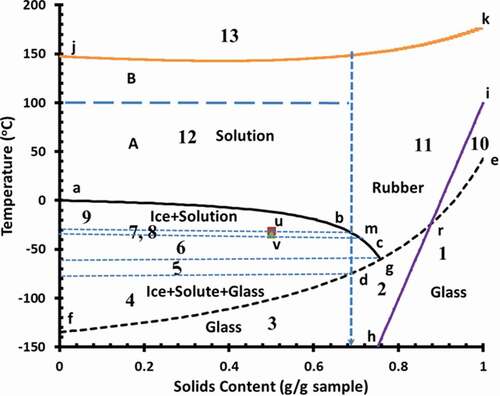
Figure 7. (k/kr)p predicted from EquationEquations 3(3)
(3) and Equation4
(4)
(4) as a function of (k/kr)e and best prediction line
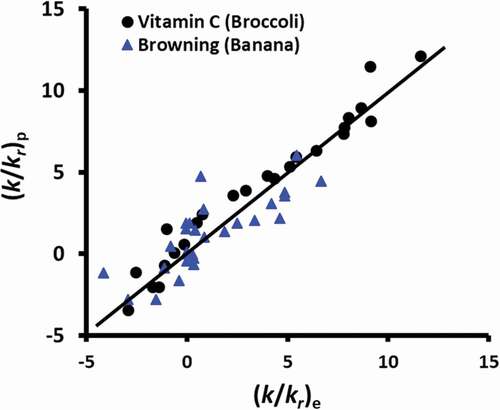