Figures & data
Figure 1. (A) Schematic representation of the Wallerian degeneration [Citation9]. (B) Schematic representation of end-to-end surgical reconnection of the injured nerve ends [Citation10]. (C) Schematic representation of the autologous nerve grafting [Citation11].
![Figure 1. (A) Schematic representation of the Wallerian degeneration [Citation9]. (B) Schematic representation of end-to-end surgical reconnection of the injured nerve ends [Citation10]. (C) Schematic representation of the autologous nerve grafting [Citation11].](/cms/asset/c1255b28-44d0-42e7-979d-7b0e64926551/ianb_a_1639723_f0001_c.jpg)
Figure 2. Nerve guidance channels improve axonal growth without the formation of mismatches. (A) Schematic representation of inhibitory elements for axonal regeneration after peripheral nerve injury and the possible formation of mismatches. (B) Presence of a rigid tubular structure provides mechanical support and promotes axonal regeneration [Citation47]. (C,D) SEM images of a nerve guidance channel (NGC) fabricated by RGD-conjugated polyurea and polycaprolactone. (E) Sciatic nerve isolation. (F) Sutured autograft and (G) sutured conduit fabricated by RGD-conjugated polyurea and polycaprolactone [Citation38]. (H) Representative digital image of an NGC fabricated by chitosan-gelatin-polypyrrole polymers. (I) Sciatic nerve isolation and (J) sutured conduit fabricated by chitosan-gelatin-polypyrrole polymers [Citation48]. In the next sections, the physiochemical properties of synthetic polymeric biomaterials are discussed and possible solution for tailoring these polymers for neural tissue engineering is reviewed. In the next section, we reviewed the physiochemical properties of synthetic polymeric biomaterials that make them suitable candidates for nerve tissue engineering purposes.
![Figure 2. Nerve guidance channels improve axonal growth without the formation of mismatches. (A) Schematic representation of inhibitory elements for axonal regeneration after peripheral nerve injury and the possible formation of mismatches. (B) Presence of a rigid tubular structure provides mechanical support and promotes axonal regeneration [Citation47]. (C,D) SEM images of a nerve guidance channel (NGC) fabricated by RGD-conjugated polyurea and polycaprolactone. (E) Sciatic nerve isolation. (F) Sutured autograft and (G) sutured conduit fabricated by RGD-conjugated polyurea and polycaprolactone [Citation38]. (H) Representative digital image of an NGC fabricated by chitosan-gelatin-polypyrrole polymers. (I) Sciatic nerve isolation and (J) sutured conduit fabricated by chitosan-gelatin-polypyrrole polymers [Citation48]. In the next sections, the physiochemical properties of synthetic polymeric biomaterials are discussed and possible solution for tailoring these polymers for neural tissue engineering is reviewed. In the next section, we reviewed the physiochemical properties of synthetic polymeric biomaterials that make them suitable candidates for nerve tissue engineering purposes.](/cms/asset/926f4f14-87d9-4b8d-bb63-bcbd43dbcbc2/ianb_a_1639723_f0002_c.jpg)
Figure 3. The effects of PLLA on porosity of scaffold. (A) 2% wt/v, (B) 5% wt/v and (C) 9% wt/v [Citation101].
![Figure 3. The effects of PLLA on porosity of scaffold. (A) 2% wt/v, (B) 5% wt/v and (C) 9% wt/v [Citation101].](/cms/asset/62b53a69-cb07-4e79-8fd6-0f9bc46a4ab8/ianb_a_1639723_f0003_b.jpg)
Figure 5. Schematic representation of Poly-L-lysine coated PLGA/MWCNTs scaffold design and differentiation of PC12 cells into it with electrical stimulation [Citation108].
![Figure 5. Schematic representation of Poly-L-lysine coated PLGA/MWCNTs scaffold design and differentiation of PC12 cells into it with electrical stimulation [Citation108].](/cms/asset/ade416e3-ee67-467f-8a6c-bc2dff9e15fc/ianb_a_1639723_f0005_c.jpg)
Figure 6. (A,B) FE-SEM micrographs of the AuNPs-doped PCL/chitosan scaffolds exhibited the presence of AuNPs on the substrate without any destruction of microstructure. (C) The evaluation of Schwann cell proliferation on the substrate by MTT assay after 1 and 5 days. (D,E) FE-SEM micrograph of Schwann cell morphology on PCL/chitosan and the AuNPs-doped PCL/chitosan [Citation114].
![Figure 6. (A,B) FE-SEM micrographs of the AuNPs-doped PCL/chitosan scaffolds exhibited the presence of AuNPs on the substrate without any destruction of microstructure. (C) The evaluation of Schwann cell proliferation on the substrate by MTT assay after 1 and 5 days. (D,E) FE-SEM micrograph of Schwann cell morphology on PCL/chitosan and the AuNPs-doped PCL/chitosan [Citation114].](/cms/asset/54f928c0-0dc5-4b8e-a4a0-a0f9a38ffe2e/ianb_a_1639723_f0006_b.jpg)
Figure 7. (A) Schematic representation of SPL201 cell on the cross-linked polymer disk. (B) Morphology (a-c × 25;d-f, ×200) of SPL201 cells on the cross-linked disks 7 days after seeding. (a) PCLF, (b) PPF/PCLF (25%), (c) PPF/PCLF (50%), and (d) tissue culture polystyrene (TCPS). (C) MTS absorption of SPL201 cells on the cross-linked disks of PCLF and PPF/PCLF (25%) and (50%) relative to cell-seeded TCPS as positive (+) control and empty TCPS as negative (−) control. (p < 0.05 between PPF/PCLF (50%) and PCLF, PPF/PCLF (25%)).
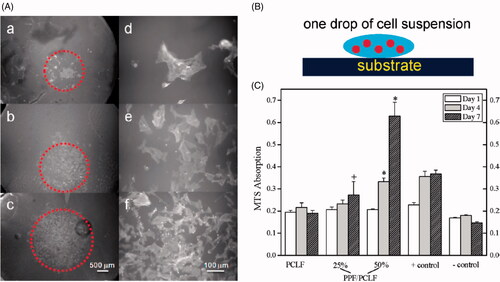
Figure 8. (A) Schematic representation of conduit containing PPF, collagen, and CBD-NT3 for treatment of peripheral nerve injury. (B) The greatest connectivity and integration with a normal spinal cord was found for the PPF + C+NT3 group. (C,D) The highest reduction of the chondroitin sulfate proteoglycans (CSPGs) as an inhibitor of regeneration was observed in the PPF + C+NT3 group. (E,F) The highest reduction of fibrotic scarring (Laminin) was observed in the PPF + C+NT3 group [Citation80].
![Figure 8. (A) Schematic representation of conduit containing PPF, collagen, and CBD-NT3 for treatment of peripheral nerve injury. (B) The greatest connectivity and integration with a normal spinal cord was found for the PPF + C+NT3 group. (C,D) The highest reduction of the chondroitin sulfate proteoglycans (CSPGs) as an inhibitor of regeneration was observed in the PPF + C+NT3 group. (E,F) The highest reduction of fibrotic scarring (Laminin) was observed in the PPF + C+NT3 group [Citation80].](/cms/asset/9d3fc1f8-0417-4781-94de-de5a5a7f938f/ianb_a_1639723_f0008_c.jpg)
Figure 9. (A,B) The fluorescence images of PC12 cells a and C6 cells b on PVA nanofibers, KNPs/PVA nanofibers with different mass ratios of KOS to PVA, and KOS/PVA blend nanofibers after 24 h. (C,D) Quantitative analyses of Cell numbers and length ratio on different nanofibers [Citation127].
![Figure 9. (A,B) The fluorescence images of PC12 cells a and C6 cells b on PVA nanofibers, KNPs/PVA nanofibers with different mass ratios of KOS to PVA, and KOS/PVA blend nanofibers after 24 h. (C,D) Quantitative analyses of Cell numbers and length ratio on different nanofibers [Citation127].](/cms/asset/88b4e3d9-8051-4e53-ad3e-8a11b46b28b3/ianb_a_1639723_f0009_c.jpg)