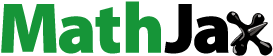
Abstract
A largely defined series of hydrolytic enzymes active during malting and/or mashing, substantially determine the quality, profitability, and efficiency of the brewing process. These enzymes potentially hydrolyze starch, proteins and cell wall non-starch polysaccharides including β-glucan and arabinoxylan. Commercial malts (94) were assayed for the DP enzymes (limit dextrinase, beta/α-amylase), and NSP hydrolyzing enzymes (β-glucanase, xylanase, arabinofuranosidase, β-glucosidase). The levels of enzyme activity were related to conventional measures of malt quality such as extract, fermentability, protein, KI, DP, friability, wort viscosity, FAN, and β-glucan. These parameters were interrelated with less conventional measures of malt quality including coarse extract and fermentability (modified infusion mash 65 °C), lautering efficiency, the Small-scale Wort ‘I’ Filtration Test (SWIFT), and viscosity. Substantial variation was observed between the malt samples for all enzymes assayed. Australian barley, whether malted in Australia (n = 61) or China (n = 24), was observed to be of comparable quality. A limited set of Canadian barley samples (n = 9) were malted in China and produced malts with somewhat higher levels of extract, AAL, and some enzymes. Remarkably, the level of limit dextrinase was observed to be almost double that from previous investigations. Greater levels of steep water aeration were proposed to explain this dramatic increase. The interrelationships between the enzyme activities and malt quality identified, enable potential selection of novel malt quality parameters that are more predictive of a malt’s brewing performance (efficiency and quality) than current measures to provide a malt quality assessment system based on ‘functional’ malt quality.
Introduction
Malt quality parameters and specifications are what brewers use to purchase malt and determine its suitability for their brewing processes. As such, malt quality has a substantial impact on brewing efficiency, profitability, and final beer quality. Surprisingly some of the conventional key indicators used to describe malt quality such as the Congress mash (1815)[Citation1] and Diastatic Power (DP, 1833)[Citation2] are very old, having their origins in the early 19th Century.
Given the level of innovation that has taken place across the brewing and malting industries over the last two centuries, challenging questions have been posed by Prof Barry Axcell (Former Head Brewer, SAB and SAB Miller)[Citation3–5] about their suitability with respect to modern malting and brewing practices. The key and provocative statements posed by Axcell were, “Why is it that malt analysis is still universally used if it gives such limited information?”[Citation3] and, “Malts however, are often purchased with similar analyses that behave completely differently on brewing.”[Citation5] To some extent maltsters and brewers compensate for the failings of conventional malt analysis parameters by an experiential understanding of malt performance empirically gleaned from brewhouse experience.[Citation6] However, these understandings are typically associated with variation within different malts of the same variety. Thus, the modernization of assessments of malt quality that are linked with brewing process-relevant characteristics has long been warranted.
Combined, the processes of malting and mashing essentially result in the hydrolysis of a range of polymeric malt components in the malt.[Citation7] In the brewing/mashing processes, malt yield is primarily controlled by the hydrolysis of starch, proteins, and non-starch polysaccharides (NSP). In essence it is these processes and the products from them that malt analysis seeks to define. Central to the description of malt quality is the method used for small-scale mashing. The Congress mash[Citation8,Citation9] is the mash conventionally used for malt analysis but an increasing number of investigators are now questioning the applicability of the parameters of the Congress mash for determination of malt quality and varietal comparison.[Citation1, Citation10–13] Typically the focus of these investigations has been towards developing an infusion mash at ∼65 °C rather than the traditional ‘programmed’ (45-70 °C) Congress mash. Most importantly for variety evaluation, it has been observed that the Congress mash gives a different ranking to a 65 °C infusion mash.[Citation11,Citation12] Given that the majority of commercial mashing protocols are better represented by the a 65 °C infusion mash, the Congress mash has now perhaps been surpassed.
The generation of yeast fermentable sugars results from the hydrolysis of starch primarily during mashing. The DP enzymes, α-amylase hydrolyzing endo α(1-4) glucosidic links, limit dextrinase (LD) α(1-6) glucosidic branch-links, α-glucosidase exo-α(1-4) glucosidic links (glucose) and beta-amylase exo-α(1-4) glucosidic links (maltose) are the key enzymes for starch hydrolysis.[Citation7] To date, α-glucosidase has been concluded to be of minor importance to the yield of yeast fermentable sugars.[Citation14–16] All of the DP enzymes, except beta-amylase (barley) are mostly or fully synthesized during the malting process, with their expression being promoted by gibberellins.[Citation17]
For conventional malt analysis, the DP parameter has been considered an overall measure of starch degrading activity in the malt.[Citation18] However, an extensive series of investigations by Evans and colleagues has demonstrated that wort fermentability can be predicted, R2 = 0.69-0.91, by multi-linear regression (MLR) algorithms based on the activity balance of α-amylase, LD and beta-amylase, and the inclusion of a measure of protein modification such as KI.[Citation1, Citation11, Citation15,Citation16, Citation19] Of the DP enzymes, only the thermostability of beta-amylase (Sd2H type) has been demonstrated to impact fermentability on small or commercial scales.[Citation1, Citation15, Citation20] The malt modification component can be assessed as either KI or perhaps more directly as osmolyte concentration.[Citation21–24] In either case the malt modification increases the concentration of solutes that protect relatively thermolabile enzymes[Citation25–27] such as LD and beta-amylase. The other area of starch hydrolysis that requires further investigation is the structure and properties of starch, particularly but not only, its gelatinization.[Citation28,Citation29] Overall, the traditional measure for malt starch hydrolysis has been DP, however the predictive value of this measure is typically R2 = 0 − 0.54, which led Evans et al.[Citation30], based on a series of commercial trouble-shooting exercises, to conclude that DP was a poor or even misleading measure of a malt’s starch degrading potential.
NSP hydrolysis contributes mainly towards the efficiency of the brewing process, primarily through those stages where filtration is required, lautering and beer filtration. The primary NSP’s in brewing are β-glucan and arabinoxylan, with β-glucan (70% and 20%, respectively) being the principle NSP of the endosperm, while arabinoxylan is the main NSP of the embryo and aleurone layer (70% and 25% respectively).[Citation31] It follows that endo-(1,3; 1,4)-β-glucanase and to a lesser extent β-glucosidase are the key enzymes for the hydrolysis of β-glucan, while endo-1,4-β-xylanase, arabinofuranosidase, and ferulic acid esterase (β-glucan solubliase) are the primary enzymes for hydrolysis of arabinoxylan.[Citation7] Similar to α-amylase and LD, the NSP cleaving enzymes are mostly or fully accumulated in the malt during the malting process, with their expression being stimulated by gibberellin.[Citation32]
In brewing and malting, the focus has been reducing the amount of wort β-glucan thus wort viscosity. In this the brewing industry has been very successful by using Calcofluor White, which has the very useful property of only complexing with β-glucan with a molecular weight >50 kDa, although peak fluorescence is not fully achieved until the β-glucan reaches 200 kDa.[Citation33–35] It has been shown that the size of wort β-glucan needs to be greater than ∼80-123kDa before it causes problems with beer filtration.[Citation36] It is also presumed a similar relationship between size and impact is associated with arabinoxylan, however the conventional method of arabinoxylan quantification, based on the phloroglucinol method of Douglas,[Citation37] measures total arabinoxylan regardless of size. Various tests have been developed to functionally emulate the impact of NSP’s and other components in the filtration stages of the brewing process. Two relatively efficient, simple, and inexpensive tests that have been developed are the lautering efficiency (LE) test[Citation1] and the Small-Scale Wort ‘I’ Filtration Test (SWIFT) that seek to predict subsequent beer filterability based on the volume of filtrate through a 0.45 µm pore size, nylon membrane.[Citation38]
In this investigation, 94 commercial malt samples from Australia and China were assessed and compared for their malt quality. The focus centered on the measures of brewing efficiency (LE and SWIFT) and yield (extract and fermentability). These malt quality parameters were assessed using a modern 65 °C Modified Infusion Mash and the direct measurement of DP enzymes (α-amylase, LD, and beta-amylase) and NSP hydrolyzing enzymes (β-glucanase, xylanase, arabinofuranosidase, and β-glucosidase).
Materials and methods
Commercial malt samples
Combined, 94 commercial malt samples malted in late 2017 and 2018 were sourced from six commercial malthouses in Australia and four malthouses in China. A summary of the broad malting parameters for all of the source malthouses is presented in . Sixty-one malt samples in total were from Australian barley malted in Australia, with 53 malted in the six commercial malthouses (varieties: Bass, Commander, Compass, Flinders, La Trobe, RGT Planet, Schooner, Scope, Spartacus, Westminster) and eight samples were malted in the commercially accredited, 100 kg batch size, Pilot Malting Australia Unimalter (HDP, Cambridge, ON, Canada, varieties: Baudin, Flinders, Latrobe, Planet). Thirty-three malts were produced by four Chinese malthouses using barley from Canada (nine malts, varieties: Copeland and Metcalfe) and Australia (24 malts, varieties: Compass, Fair Average Quality, La Trobe, Scope). These malts were supplied with the determinations for standard commercial Congress parameters (EBC/ASBC)[Citation8,Citation9] including total protein (% dw), Kolbach index (KI %), diastatic power (DP, °WK), friability (%), and free α-amino nitrogen (FAN, mg/L).
Table 1. Summary of the characteristics of the typical malting programs for the malthouses providing malt for this study.a
Milling
All malt samples for enzyme analysis (∼10 g) were milled using a Udy mill (Udy Corporation, Fort Collins) to pass 1 mm screens. Milled samples were stored in tightly capped 15 ml polypropylene tubes to avoid moisture absorption.
Malt enzyme assays (12 x 8 well micro-well format)
DP enzymes
DP enzyme activity (total beta-amylase, α-amylase, and total limit dextrinase) was measured by a small-scale protocol using the Megazyme (Bray, Ireland) Betamyl-3, Ceralpha, and Limit DextriZyme substrates, respectively, with the rapid, 8 × 12 micro-well format protocol, extracting malt and barley with Limit-DextriZyme extraction buffer (0.1 M maleic acid, 25 mM dithiothreitol, 0.02% sodium azide, pH 5.5) as described by Evans.[Citation39] Because of the non-availability of the Betamyl-5 substrate, the protocol was modified to use the Betamyl-3 substrate as described by Evans et al.[Citation19] The relevant extract dilutions for the assays (assay time, 10 min) were:
Ceralpha assay: malt 1/250.
Betamyl-3 assay: malt 1/12.5.
Limit DextriZyme assay: no dilution.
Beta-amylase activity measured with the Betamyl-3 substrate was converted into that recorded by Betamyl-5 substrate, for comparison with previously reported levels of beta-amylase activity, using the equation of Evans et al.,[Citation19]
Betamyl-5 (U/g dwt) = 62.92 × Betamyl-3 (U/g dwt) – 8.8.
For beta-amylase thermostability testing – the sample after 12.5 min heating at 57.5 °C in Limit-DextriZyme extraction buffer (including 25 mM dithiothreitol, and chilled on wet ice for 10 min) was centrifuged and the supernatant diluted 1/3 with the Limit DexriZyme buffer without DTT (see above) to assay for beta-amylase activity. The thermostability of limit dextrinase was not measured as the thermostability of this enzyme was not found to be a useful predictor of fermentability in previous studies.[Citation1, Citation15, Citation19]
β-Glucanase
Malt β-glucanase activity was measured with the Beta-Glucazyme (Megazyme) assay.[Citation19] The extract was obtained by extracting 65 mg of malt with 1 ml of 0.2 M acetate buffer pH 4.5 (20 min with constant mixing). After centrifugation (∼3,900 g for 5 min), a 12 mg Beta-Glucazyme tablet was added to 50 µl of extract and incubated for 15 min at 30 °C without mixing. The reaction was stopped by adding 1.2 ml of 2% Trisma base solution and vigorously mixed. After filtration, the absorbance of the assay was measured at 590 nm, the blank absorbance was subtracted, and the activity calculated according to the Megazyme instructions.
β-Glucosidase
β-Glucosidase activity was measured according to Kanauchi and Bamforth.[Citation40] Milled malt, 65 mg, was mixed with 0.6 ml 0.1 M citrate-phosphate buffer (pH 4.0) for 60 min and then centrifuged ∼3,900 g for 5 min. A 50 µl aliquot of undiluted extract was mixed with 50 µl of 10 mM p-nitrophenyl-β-D-glucopyranoside (Megazyme) at 40 °C. The assay incubation lasted 20 min with the reaction being stopped by mixing 750 µl 1% tri-sodium phosphate (pH ∼11). The absorbance was measured at 405 nm and the blank absorbance was subtracted. The EmM for p-nitrophenol in 1% trisodium phosphate was 18.1.
Endo-xylanase
Malt xylanase activity was measured using the Megazyme XylX6 soluble substrate assay. The extract was obtained by extracting 65 mg of malt with 1 ml of 0.1 M acetate buffer pH 5.5, (30 min with constant mixing). After centrifugation (∼3,900 g for 5 min), 25 µl of XylX6 soluble substrate was added to 125 µl of extract to be incubated for 60 min at 40 °C. The reaction was stopped by adding 225 µl of 2% Trisma base solution (pH 10.0) and vigorously mixed. The absorbance of the assay was measured at 500 nm, the blank absorbance was subtracted, and the activity calculated according to the Megazyme instructions.
Arabinofuranosidase
Malt arabinofuranosidase was measured using the Megazyme p-nitrophenyl-α-L-arabinofuranosidase soluble substrate, essentially as described by Gastl et al.[Citation41] The extract was obtained by extracting 65 mg of malt with 1 ml of 0.1 M acetate buffer pH 5.0 (30 min with constant mixing). After centrifugation (∼3,900 g for 5 min), 100 µl of XylX6 soluble substrate was added to 50 µl of extract and incubated for 60 min at 40 °C with mixing. The reaction was stopped by adding 400 µl of 2% Trisma base solution (pH 10.0) and vigorously mixed. The absorbance was measured at 400 nm and the blank absorbance was subtracted. The activity was calculated. The EmM for p-nitrophenol in 2% Trisma was 18.1.
Small-scale 65 °C modified infusion mash (MIM 65 °C) and analysis
The small-scale Modified Infusion Mash 65 °C (MIM 65 °C), previously named “Final 65 °C mash,” was used for all mashes.[Citation1] Briefly, this mash uses 50 g of malt 0.7 mm disc milled (Buhler Miag, Brunswick, Germany), warmed to 65 °C for 10 min in a Bender and Hobein (Ismaning, Germany) mash bath. The mash was commenced with the addition of 150 ml of 65 °C liquor containing 2.9 mM CaCl2. The mash temperature was maintained at 65 °C for 60 min before the temperature was ramped up to 78 °C (2.5 °C.min−1). The mash was maintained at 78 °C until 74 min, when 150 ml of 78 °C water was added. After a further 10 min at 78 °C, the mash was cooled to 25 °C with the pot containing the mash being made up to 450 g of liquor and malt. The mash was then poured into a 300 mm funnel lined with a 305 mm Machery-Nagle (Düren, Germany) MN 722 filter paper for precisely 25 min into a 500 ml graduated cylinder. At 25 min, the volume of wort separated was recorded (Lautering efficiency) and wort was then allocated for fermentability, extract, viscosity, and SWIFT determination. Fermentability (Apparent Attenuation Limit – AAL) was determined by a 24 h fermentation at 21 °C with constant agitation (150 rpm) with dried Mauribrew Lager 495 yeast (Mauribrew, Minto, NSW, Australia) as described by Evans and Hamet.[Citation42] Wort viscosity and density (extract and AAL calculations) was determined by an DMA 4500 densitometer and an AMV-200 micro-viscometer “rolling ball” (Anton Paar KG). AAL or fermentability was calculated according to Analytica-EBC.[Citation9] The soluble high molecular weight mixed linkage (1,3;1,4)-β-D glucan (β-glucan) fraction in wort was measured by the Congo red method according to Analytica-EBC (method 4.16.3).[Citation9]
SWIFT wort filterability
The small-scale wort filterability test (SWIFT) was conducted according to the method described by Stewart et al.[Citation38] The assembly of syringes, a 13 mm Swinnex filter holder (Millipore, Billerica, MA) into which was placed a 0.45 μm, type 250, 13 mm diameter polyamide membrane filter (Sartorius, Göttingen, Germany) and adaptors was accomplished in accordance with the instructions of Stewart et al.[Citation38] Each syringe assembly was then placed in the SWIFT rack, with the lower syringe plunger drawn to create a vacuum that draws the wort through the membrane into the lower syringe. The SWIFT value is the amount of wort filtered and measured after exactly 3 min.
Statistics
Statistical evaluations of reproducibility, regression, and MLR were undertaken using JMP version 9.4 m5 statistical software from the SAS Institute (Cary, NC, U.S.A.).
Results and discussion
Comparison of barley grown in Australia or Canada, and commercially malted in Australia or China
A novel comparison of malt quality for barley, sourced primarily from Australia, which was malted in six Australian and four Chinese commercial malthouses were analyzed in this study. The consensus malting protocols typically used in Australia and those applied in the four Chinese malthouses are outlined in . The malting programs were essentially determined by the malthouse design, malthouse temperature control potential with respect to external temperatures, variety, and the customer’s modification requirements. The primary difference between the malting programs was the steeping time and the duration of the wet and dry periods.
Combined, 94 commercial malt samples were analyzed for their malt and mash derived quality parameters (). Sixty-one malt samples were Australian barley malted in Australia, with 53 malted in six commercial malthouses (11 varieties) and eight samples malted in the commercially comparable Pilot Malting Australia Unimalter (four varieties). Thirty-three malts were produced by four Chinese malthouses (two Canadian and four Australian varieties). As expected, the level of Canadian malt protein was on average 6.5-12.7% higher (Australia-China, ). Comparing the amount of DP enzymes, it was noted that on average, and as expected, the China malted Canadian were higher for α-amylase by 23%, limit dextrinase (LD) by 23.7-16.3%, and beta-amylase by 36-15% but beta-amylase thermostability was higher by 240-60% in malted Australian barley. Overall, the fermentability (AAL) of the Canadian malts would be expected to be somewhat higher,[Citation1, Citation15,Citation16, Citation19] although the higher Sd2H beta-amylase thermostability of some of the Australian malts[Citation1, Citation20] would reduce the AAL difference somewhat.
Table 2. Summary of key malt quality parameters. A. Malt protein, key starch and cell wall degrading enzymes, B. DP and mash dependant malt quality parameters.
For the first time, a large number of commercial malts were assessed for the levels of a relatively comprehensive set of cell wall degrading enzymes (). Substantial variation was observed across variety, barley growing location, and malting location. Apart from arabinofuranosidase (5.0-0.0%), higher levels of the β-glucosidase (20.5-17.0%), xylanase (58-48%) and β-glucanase (40.1-50.1%) were observed for the China malted Canadian varieties, than the Australian varieties (Australian barley). Interestingly and perhaps importantly, β-glucosidase can also cleave glycosides present in barley[Citation43,Citation44] to release flavor active aglycones. At this time, it is not known what the influence of high or low levels will have on potential malt flavor or quality. Overall, such results perhaps suggest that the wort filterability and lautering efficiency of the malt made from Canadian grown barley should be higher than from Australian grown barley.
Mashing is a complex brewing process with many interacting parameters converging to determine efficiency and yield outcomes.[Citation7] The 94 malts were mashed using a small-scale, Modified Infusion Mash at 65 °C (MIM 65 °C).[Citation1, Citation11] In comparison to the standard fine grind (0.2 mm) Congress mash, the coarse grind (0.7 mm) MIM 65 °C yields on average 1.1% lower extract, although the more favorable mashing in temperature of 65 °C yields 6-8% higher AAL.[Citation1, Citation16] The higher fermentability of the MIM 65 °C mash, compared to the Congress mash, has been attributed to the increased solute/osmolyte concentration protecting the thermolabile DP enzymes, and the 65 °C mash temperature being the optimum for DP enzyme activity and starch gelation required for efficient hydrolysis.[Citation1, Citation15] In addition, two novel assessments of malt filterability performance were included. A simple volume based lautering efficiency test[Citation1, Citation11] and a measure of wort/beer filterability, the Small-Scale ‘I’ Wort Filtration Test (SWIFT) that was based on the volume of filtrate through a 0.45 µm pore size, nylon membrane.[Citation38]
With respect to malt quality, actual functional quality adheres very much to the old adage, “the proof is in the pudding,” (). shows that the level of Diastatic Power (DP) was on average 18.4-4.6% (Chinese-Australian) higher in the Chinese malted Canadian barley. These differences were somewhat lower than for the average comparison of the DP enzymes outlined above (). The average KI for the Chinese-Canadian malts were higher (7.6-5.6%) although the comparison was on average variable with FAN (16.0 to −1.7%). In terms of wort pH, the Chinese-Canadian malt derived worts were slightly lower, but they completely overlapped. Extract is perhaps the most important malt quality parameter for brewers. The Chinese-Canadian malts were 1.2 and 1.0% higher for the coarse grind MIM 65 °C mash and a similar differential was maintained for the fine grind Congress mash. However, the Chinese-Canadian extract average was inflated by the inclusion of three malts from Chinese Malthouse D by 0.5%. When these three malts were isolated, the Chinese-Canadian extract average was reduced to 79.8% (79.2-80.2, n = 6). It has not been determined if the high extracts of China-D malthouse was due to the malting protocol () or judicious malt selection. As anticipated from the higher levels of DP enzymes (), the AAL of the Chinese-Canadian malts were 0.5 to 1.5% higher.
Assessments of the efficiency of brewing filtration were shown to be very similar between the malt origins (). Wort β-glucan, lautering efficiency, wort viscosity performances indicated high quality malt, which was very similar between malt sources. Similar to what was reported by Cornaggia et al.,[Citation11] all malts tested for lautering efficiency were ‘good’ or ‘very good,’ with no poor malts observed. The SWIFT results were also similar to the Cornaggia et al.[Citation11] study, with a relatively wide distribution between good and poorly performing malt worts, 4.6-10.4 ml.3 min−1. The Chinese-Canadian malts had a slightly higher SWIFT average, 8.3 ml.3 min−1 but its range fitted with that for Australian-Chinese malts at 4.6-10.4 ml.3 min−1.
Prediction of filtration efficiency
The cessation of the production of the Hollingworth and Vose Creped postlip filter papers (HV), originally used for the lautering efficiency (LE) test,[Citation1] required a change to the Machery-Nagle MN 722 filter papers (MN), which had almost identical porosity characteristics. The LE of subset of 31 malts were compared with each filter source. The average for the HV was 326 ml.25 min−1 (range 288-342 ml.25 min−1), which was slightly faster than the MN filter paper averaging 312 ml.25 min−1 (range 278-332 ml.25 min−1). shows that the two filters were highly correlated (R2 = 0.87). Thus, the replacement of the HV – no longer available – with MN filter paper was a reasonable approximation of the original for the LE test, noting that original filter papers lautered slightly faster. In conclusion, the HV, and MN filters essentially provide the same LE results.
Figure 1. Box-plot distributions over almost a decade for A. lautering efficiency, and B. SWIFT.
Malt Sources: Aust = Australia, Intl. = International malt samples from Europe and Nth America, Ch = Chinese malt from malting primarily Australian and Canadian barley, MIM = MIM 65 °C and EBC = Congress mash.
Origin of data: iii. Evans et al.,[Citation1] iiia. Evans,[Citation45] vi. Evans et al.,[Citation16] vii. Cooper et al.,[Citation81] viii. Current study, ix. Cornaggia et al.[Citation11]
![Figure 1. Box-plot distributions over almost a decade for A. lautering efficiency, and B. SWIFT. Malt Sources: Aust = Australia, Intl. = International malt samples from Europe and Nth America, Ch = Chinese malt from malting primarily Australian and Canadian barley, MIM = MIM 65 °C and EBC = Congress mash. Origin of data: iii. Evans et al.,[Citation1] iiia. Evans,[Citation45] vi. Evans et al.,[Citation16] vii. Cooper et al.,[Citation81] viii. Current study, ix. Cornaggia et al.[Citation11]](/cms/asset/4f3b652e-a07f-40b4-9d3c-5f3f24f52c8c/ujbc_a_1979891_f0001_b.jpg)
The historical box plot for LE shows a definite trajectory of improvement from the first trial on 2005-2008 year malts,[Citation1] to the consistently good LE performance in this study () and Cornaggia et al.[Citation11] The basis of this improvement would appear to be largely varietal. Old and superseded Australian varieties such as Schooner, Stirling, and sometimes Gairdner were prone to producing poor LE that could be detected by this test.[Citation1, Citation45] The poor LE was mostly alleviated by the addition of exogenous β-glucanase and cellulase into the mash.[Citation1] Beyond some characteristics of β-glucan and arabinoxylan being key components of LE, further investigation to fully understand the dynamics of lautering is still required.[Citation46]
A relatively small number of studies have been conducted comparing the SWIFT characteristics of a range of malts between 2008-10 and this study in 2017-18 (). The SWIFT assay assesses wort filterability to provide a prediction on potential beer filterability.[Citation38] Although there has been considerable improvement in LE during the past decade or so (), the wide variation for SWIFT performance has shown little improvement over this time. In this study, the correlation between LE and SWIFT was r = 0.584 (), which was similar to the relationship reported by Evans et al.,[Citation16] r = 0.52.
Table 3. Correlation matrix (linear regression) of malt parameters potentially linking lautering and wort/beer filtration efficiency (six RGT Planet samples were excluded, and one Scope sample was excluded as shown and described for ).
Other correlations noted in with LE were wort viscosity (r = −0.255) and xylanase (r = 0.355). However, an attempt to develop a multi-linear regression (MLR) prediction for LE was not successful beyond the SWIFT x LE relationship. For the most part, this was due to the lack of variation with samples that have poor LE. Taken together, these observations confirm the initial conclusions of Evans et al.[Citation16] that different, though perhaps similar malt factors impact LE compared to wort/beer filtration (SWIFT).
It is widely known in the international malting community that the promising variety RGT Planet can produce worts that are somewhat hazy during small-scale Congress mash testing.[Citation8] The observance of this wort haze does not as yet appear to impact the efficiency of commercial brewing. However, wort haze does have the potential to adversely impact the wort barrier filtration as performed in the SWIFT test.[Citation16] Unfortunately, a haze meter was not available for these mashing trials. A comparison of wort viscosity and SWIFT () did show that the RGT Planet samples segregated to the lower left of the scatterplot indicating a potential bias in the filtration efficiency dataset. As such, the RGT Planet samples and a sample with an anomalously high viscosity and low SWIFT (Scope) were excluded from the filtration efficiency analyses.
Figure 2. Box-plot distributions over almost two decades of the levels of DP enzymes in commercial malt samples: A. beta-amylase, B. α-amylase, and C. limit dextrinase. Horizontal dashed lines indicate range within which most data means were historically observed.
Malt Sources: Aust = Australia, WA-A = Western Australian malthouse noted to have consistently high malt limit dextrinase (Evans et al [Citation53]), Intl. = international malt samples from Europe and Nth America, PMA = Pilot Malting Australia (100 kg batch), China = Chinese malt from malting primarily Australian and Canadian barley. * denotes WA-A or PMA malt samples that have consistently high limit dextrinase levels.
Origin of data: i. Evans et al.,[Citation15] ii. Evans et al.,[Citation53] iii. Evans et al.,[Citation1] iv. Evans et al.,[Citation53] v. Evans and Finn 2008, unpublished, vi. Evans et al.,[Citation16] vii. Cooper et al.,[Citation81] viii. Current study.
![Figure 2. Box-plot distributions over almost two decades of the levels of DP enzymes in commercial malt samples: A. beta-amylase, B. α-amylase, and C. limit dextrinase. Horizontal dashed lines indicate range within which most data means were historically observed. Malt Sources: Aust = Australia, WA-A = Western Australian malthouse noted to have consistently high malt limit dextrinase (Evans et al [Citation53]), Intl. = international malt samples from Europe and Nth America, PMA = Pilot Malting Australia (100 kg batch), China = Chinese malt from malting primarily Australian and Canadian barley. * denotes WA-A or PMA malt samples that have consistently high limit dextrinase levels. Origin of data: i. Evans et al.,[Citation15] ii. Evans et al.,[Citation53] iii. Evans et al.,[Citation1] iv. Evans et al.,[Citation53] v. Evans and Finn 2008, unpublished, vi. Evans et al.,[Citation16] vii. Cooper et al.,[Citation81] viii. Current study.](/cms/asset/fe7a49c6-b3f3-486f-bef1-a3fba714da55/ujbc_a_1979891_f0002_c.jpg)
A correlation matrix of the relationship between SWIFT and potential malt quality predictors was produced (). shows that SWIFT was significantly correlated with wort viscosity (r = −0.591), β-glucan (r = −0.320) xylanase (r = 0.410), and friability (r = 0.227). These regression relationships were used to guide the development of a step-wise MLR for SWIFT. shows that in the MLR to predict SWIFT, wort viscosity was the most valuable predictor followed closely by malt xylanase, then KI, malt protein, and wort β-glucan (adjusted R2 = 0.51). The MLR equation developed is shown in Equation 1:
Table 4. Summary of step-wise multiple linear regression analysis for SWIFT.
Cornaggia et al.[Citation11] similarly observed that wort viscosity, xylanase, and KI could predict SWIFT with an R2 = 0.68, while an early SWIFT MLR attempt by Evans et al.[Citation16] used wort viscosity, wort haze, total beta-amylase, and extract with an R2 = 0.55. In the absence of an efficient test for high molecular weight arabinoxylan, xylanase appears to offer a proxy for this key filtration characteristic. Previous studies have also highlighted the importance of arabinoxylan. [Citation41, Citation46–50] However, depending on the malts surveyed, the MLR developed in this or previous studies[Citation11, Citation16] variously explain 49-68% of the variation in SWIFT using viscosity, xylanase, and other factors. It is therefore clear that some other factor/s that are yet to be measured are missing from the predictors. Of course, arabinoxylans in beer have also been linked to mouthfeel.[Citation51] Perhaps Krebs et al.[Citation52] have a useful future perspective in this regard with their focus on wort polymeric substances that extend beyond β-glucan and arabinoxylan.
Historical assessment of malt DP enzyme levels
A historical perspective on a large number of commercial malts measured by essentially the same DP enzyme method over a time period spanning approximately 15 years was revealing (). For beta-amylase and α-amylase () the majority of samples and box plot means fell in the range 500-800 U.g−1 and 150-220 U.g−1, respectively. Some sample sets fell outside those ranges; however, no discernible pattern/s were evident. Traditionally for LD, the majority of samples and box plot means fell in the range 250-410 U.kg−1, with a typical average of approximately 320 U.kg−1 (). It has been previously identified that one Australian malt house, WA-A, produces malts that have substantially higher levels of LD.[Citation53,Citation54] It was subsequently observed that malts produced in the 100 kg Pilot Malting Australia Unimalter had conspicuously higher levels of LD >>400 U.kg−1, both in Evans and Fox[Citation55] and . Most striking was that all malt samples assessed in this study (), Australian malted, malthouse WA-A, Chinese malted Australian-Canadian, and PMA had similar box plot means well in excess of 550 U.kg−1, with an overall average of 580 U.kg−1 for the 2017-2018 sample set.
An intriguing question from these observations would be how these changes in DP enzyme proportions might impact wort fermentable and unfermentable sugar proportions. For instance, such changes in the sugar spectrum, particularly the proportion of glucose and fructose to maltose, have been shown to influence yeast metabolism and the subsequent ester content and flavor of the beer.[Citation56] Similarly, the levels of dextrins and limit dextrins would be expected to influence mouthfeel.[Citation57–60]
Previously Evans and Fox[Citation55] proposed that the high LD in the PMA malts was due to the design and operation of the Unimalter, which required regular rousing aeration during wet steep and resulted in higher oxygen levels in the steep water. Yousef and Evans[Citation54] also suggested that the design of the steeps for WA-A (malthouse 2) was the driver for higher LD levels being produced by WA-A. These proposals were supported by McCafferty et al.,[Citation61] who observed that aerobic germination conditions increased malt LD levels. The question remains, what change in commercial malting practice has resulted in this LD outcome? Axcell et al.[Citation62,Citation63] suggested that steeping conditions, CO2 build-up during steeping, was a key contributor to the malt mediated, premature yeast flocculation (PYF) problem. On a visit to a number of malthouses in China (November 2018), it was noted that wet steeps were often being vigorously roused (Evans, Per. Comm.). Given that the WA-A (double steep) and PMA malts have consistently produced LD levels above all other malt houses, it was considered that varietal improvement was unlikely to explain the high LD levels. Assuming that steep aeration was the main driver for high malt LD levels, an explanation would be the broad adoption of increased wet steep aeration to avoid the potential for PYF. This hypothesis requires further investigation.
Prediction of fermentability
A correlation matrix was developed to identify the malt factors that were potentially predictive of fermentability or AAL (). Consistent with previous studies, the levels of the DP enzymes (α-amylase, LD, beta-amylase and its thermostability) and malt measures associated with modification (KI, friability, xylanase) were significantly correlated with AAL.[Citation1,Citation11,Citation15,Citation16,Citation19] Given the increased levels of LD consistently observed across the 94 malts studied, step-wise MLR showed that LD was the most valuable predictor of AAL, followed by friability, wort pH, and beta-amylase thermostability (). Overall, Equation 2 predicts 69% of the variation. Interestingly, the beta-amylase thermostability term in the MLR equation was negative, when past work[Citation1, Citation11, Citation15,Citation16, Citation19] and the explanation for its influence on AAL would suggest it be positive. This result could result from collinearity with major factors in the MLR or that the average and beta-amylase activity for Sd1 varieties was 800 U.g−1, while that for Sd2H varieties was 692 U.g−1(Data not shown). This could potentially confound the beta-amylase thermostability impact. Equation 2:
Table 5. Correlation matrix (linear regression) of malt parameters potentially linked to fermentability (AAL) and DP.
Table 6. Summary of step-wise multiple linear regression analysis for fermentability (AAL).
Various other malt measures have been proposed as predictors of fermentability/AAL. These include osmolyte concentration[Citation21–24, Citation64] suggesting that osmolyte concentration on its own might be a simple and overall measure of potential wort fermentability. In general, osmolyte concentration appears analogous to measures of malt modification such as KI and friability. It would, however, be useful to investigate the comparative value of osmolyte concentration compared to KI and friability for fermentability prediction more comprehensively than Hu et al.[Citation64]
Further potential key malt characteristics receiving increased research attention are starch gelatinization and structural properties that impact fermentability.[Citation28,Citation29] A number of interesting studies have been recently published that indicate important relationships between starch properties and DP enzymes that would be potentially useful for predicting fermentability.[Citation65–68] For routine, commercial laboratory malt analyses, it would appear that the Rapid Visco Analyser (RVA) currently has the most potential to assess starch properties efficiently and cost effectively.[Citation69,Citation70]
The AAL prediction algorithm generated appears to concur with the conclusions by MacGregor et al.[Citation71] that “commercial malts contain excess levels of beta-amylase below the levels of limit dextrinase.” Certainly an excess of beta-amylase has typically been observed for North American varieties.[Citation72] Similarly, the levels of α-amylase and beta-amylase, although significantly correlated with AAL (), were confounded by collinearity with LD in this study. However, when past investigations and fermentability outcomes were considered, the almost doubled levels of LD observed (∼320 to 580 U.kg−1dw) in the commercial malts in this study () only resulted in a small increase in AAL of 1-2% (). A study investigating malt blending, observed synergisms between appropriately matched malt for AAL performance.[Citation45] The current study taken together with the outcomes from the malt blending study[Citation45] and the original conclusions of Evans et al.[Citation15] show that it is the balance of the DP enzymes, the “osmolytes’ in the mash, and the properties of the starch substrate, rather than one specific property or enzyme, that determines the fermentability performance.
Table 7. Extraction of data for LD activity and commercial malt fermentability (AAL) from MIM 65 °C produced wort from current and previous investigations.
It is now abundantly clear that the traditional malt DP parameter is an unreliable and sometimes misleading predictor of fermentability in commercial brewing.[Citation30] In routine commercial practice, maltsters and brewers typically rely on DP, sometimes with the addition of α-amylase activity, to provide an indication of potential fermentability or AAL. In previous investigations, the consistently high levels of correlation between DP and beta-amylase activity (r = 0.60-0.99, )[Citation1,Citation15,Citation18,Citation73–76] have shown that DP was largely a measure of beta-amylase activity. In this study of 94 commercial malts, DP was not significantly correlated with AAL (r = −0.044, ), while a historical perspective indicates a coefficient of differentiation (R2) between not significant and R2= 0.54, whereas the combined DP enzyme/modification MLR ranges between R2 = 0.71 to 0.91 (). Brewers and maltsters are able to ameliorate the deficiencies of the DP malt parameter by largely comparing its level on a within-malt variety basis, however barley breeders with 100-1000s of different genetics stocks do not have this luxury. In particular, LD should be measured given its importance in this and other studies () and that it is rarely measured by breeders, maltsters, or brewers. This asks the question, are the days of malt DP analysis over?
Table 8. Historical summary of step-wise linear regression equations for fermentability (AAL) for commercial malts.
Our research group has produced a number of MLR equations to predict fermentability since Evans et al.[Citation15] for the prediction of fermentability (). This heritage of study of fermentability prediction essentially fulfils the tenets outlined by Ioannidis[Citation77] for avoiding false research conclusions. For predominantly Australian malts, the predictive factors have mainly focused on the DP enzymes (α-amylase, LD, beta-amylase, and its thermostability) and measures of modification (KI, friability). For the first two studies, this algorithm was very robust.[Citation15,Citation16] The focus was on identifying the predictive characters rather than producing a universal equation. Evans et al.[Citation15] concluded that it was the balance of these DP enzymes along with measures of modification. This point was highlighted by Evans[Citation45] who demonstrated that blending malts of different quality could result in synergisms between the malts for fermentability as deficiencies within one or both malts were ameliorated. The current study shows that an apparent change in the malting protocol could drastically increase malt LD levels (). The increase in prominence of LD appears to have reduced the predictive contribution of α-amylase and beta-amylase. It was thus not surprising that Herta-Zurita et al.[Citation78] were unable to satisfactorily use the prediction algorithm from Evans et al.[Citation15] for North American malts and varieties grown and malted in North America. It seems clear that MLR algorithms will in the future need to take advantage of machine learning, which is rapidly developing and available with increasing commercial access for routine malt quality analysis.[Citation79,Citation80]
Summary
In this study, 94 commercial malts were assessed for starch degrading enzymes, cell wall degrading enzymes, and quality parameters derived from a small scale MIM 65 °C mash (). The malts were produced from Australian barley that was malted in six Australian malthouses (11 varieties, n = 61) and four Chinese malthouses (four varieties, n = 24). Nine malts were made from Canadian barley (two varieties) in the Chinese malthouses. Despite some differences in the malting protocols (particularly steeping) between Australia and China (), the quality of the malt in terms of the levels of DP enzymes, cell wall degrading enzymes, and mash-derived malt quality parameters were broadly similar.
For the first time, substantial variation in the levels of a comprehensive selection of cell wall enzymes were measured across a large number of commercial malt samples (). On average, Chinese malted Canadian barley had higher levels of β-glucosidase, xylanase, and β-glucanase, compared to Australian barley malted in Australia or China. The level of arabinofuranosidase was consistent across the samples. Interestingly, despite the origin differences for the levels of cell wall degrading enzymes, all malt origins on average, had similar measures for LE and SWIFT. Historically, it was observed that over the past decade LE improved (), so that all samples had LE that was either good (250-299 ml.25 min−1) or very good (300< ml.25 min−1). It was concluded this was the result of varietal improvement. Conversely, little change could be observed in the variation for SWIFT (). In agreement with Cornaggia et al.,[Citation11] wort viscosity and malt xylanase levels were the most predictive of malt sample SWIFT performance (, equation 1). This suggests that breeding programs and maltsters, in lieu of a direct measure of wort arabinoxylan and its size, should consider selection for and measurement of xylanase when developing new varieties and producing malts that make beer that would be more efficiently filtered.
Substantial levels of variation were observed between the malt samples and the origins for DP enzymes (). The Chinese made Canadian malts were observed to be higher in α-amylase, LD, and beta-amylase, but beta-amylase thermostability was higher in malted Australian barley. From a historical perspective, the average level of LD was 320 U.kg−1 compared to 580 U.kg−1 for all the malts in this study, Australian and Chinese malted (). It was proposed that the increased LD, which had been previously observed specifically in the Australian malthouse WA-A and in malt produced by the PMA Unimalter, was most likely the result of increased aeration during steeping.
The levels of DP enzymes and a measure of malt modification were again demonstrated to be superior to the Congress DP test, the traditional malt parameter for predicting fermentability. A step-wise MLR was developed that showed AAL was predicted by an algorithm of LD, friability, wort pH, and beta-amylase thermostability (, equation 2, adjusted R2 = 0.69). shows that this algorithm was broadly consistent with equations developed previously. Overall, the Chinese malted Canadian malted barley had somewhat higher levels of fermentability (AAL) than the Australian malted barley () but not as much as might have initially been expected based on the differential in DP enzymes. This brings to the fore the original concept of Evans et al.[Citation15] that it was the balance of the DP enzymes that was paramount for predicting fermentability. The importance of this concept was further advanced by malt blending trials that demonstrated synergies in fermentability with appropriately matched malts in which one malt overcame the DP enzyme deficiencies of the malt with lower fermentability.[Citation45]
Of course, the DP enzyme basis for predicting fermentability can be improved and built upon. It is considered that a better understanding of the DP enzyme substrate starch[Citation28,Citation29] and perhaps also mash osmolyte concentration[Citation21–24] could potentially improve the accuracy of fermentability prediction. In this study, the conventional DP was again of no value in predicting fermentability (r = −0.044), which was concordant with Evans et al.[Citation30] that DP was an unreliable and sometimes misleading predictor of potential fermentability. In addition, given the substantial change identified in the proportions of malt LD with respect to α-amylase and beta-amylase, the measurement of fermentable sugar levels (fermentability) and non-fermentable limit dextrins/dextrins could be a malt quality parameter worthy of further research. It also follows that with the very high levels of LD observed in this study that brewers, breeders, and maltsters are recommended to routinely measure at least LD to gain a more accurate indication of a malt’s potential fermentability.
Supplemental Material
Download MS Word (353.8 KB)Acknowledgements
The authors gratefully acknowledge the GRDC (Project Number: 9176702), Coopers Brewery Ltd, Glencore Ltd, for funding this project. We are also grateful to Pilot malting Australia, and the Australian and Chinese maltsters that contributed malts samples that enabled this study. Dan Carey (New Glarus Brewing, WI) is thanked for constructive comments. We also thank the School of Natural Sciences, University of Tasmania, and the School of Agriculture, Food and Wine, University of Adelaide for the facilities to enable the study.
Disclosure statement
No potential conflict of interest was reported by the authors.
Literature cited
- Evans, D. E.; Goldsmith, M.; Dambergs, R.; Nischwitz, R. A Comprehensive Reevaluation of the Small-Scale Congress Mash Protocol Parameters for Determination of Extract and Fermentability. J. Am. Soc. Brew. Chem. 2011, 69, 13–27. DOI: 10.1094/ASBCJ-2011-0111-01.
- Payen, A.; Persoz, J. F. Memoire Sur la Diastase, Les Principaux Produits de Ses Reactions, et Leurs Applications Aux Arts Industriels. Ann. Chim. 1833, 53, 73–92.
- Axcell, B. C. Malt Analysis – Prediction or Predicament. Tech. Quart. Master Brew. Ass. Am. 1998, 35, 28–30.
- Axcell, B. C. Don’t Let Sleeping Dogmas Lie - a Personal Journey in Brewing. Brewer and Distiller Int. 2018, 14, 28–33.
- Axcell, B. C.; Morrall, P.; Tulej, R.; Murray, J. Malt Quality specifications - A Safeguard or Restriction on Quality. Tech. Quart. Master Brew. Ass. Am. 1984, 21, 101–106.
- Back, W.; Narziss, L. Malt Parameters and Beer Quality. Brauwelt Int. 1997, 15, 29–37.
- Evans, D. E. Mashing. Am. Soc. Brew. Chem., and Mast. Brew. Am. Ass., Pilot Knob, Minneapolis, MN, 2021.
- American Society of Brewing Chemists ASBC Methods of Analysis; American Society of Brewing Chemists: Pilot Knob; 2009.
- European Brewery Convention Analytica - EBC, ed. E.A. Committee. Vol. 7th Edition. Verlag Hans Carl: Nurnberg, Germany, 2008.
- ANON. Malt Analysis: Industry-Wide Change in Analysis Approach for Barley Malt 5 EBC. Brauwelt Int. 2020.
- Cornaggia, C.; Evans, D. E.; Draga, A.; Mangan, D.; McCleary, B. V. Prediction of Potential Malt Extract and Beer Filterability from Conventional and Novel Malt Quality Assessment Parameters. J. Inst. Brew. 2019, 125, 294–309. DOI: 10.1002/jib.567.
- Gastl, M.; Knofel, T.; Becker, T. Conversion of Isothermal 65 °C Mash-Measurement of Quality Characteristics. Brauwelt 2014, 154, 274–276.
- Stenholm, K.; Home, S.; Pietila, K.; Jaakkola, N.; Leino, E. Are the Days of Congress Mashing over? Proc. Barley Malt Wort Symp., Inst. Brew.; Central and South African Section: Zimbabwe, 1996; pp. 149–163.
- Bamforth, C. W. Barley and Malt Starch in Brewing: A General Review. Tech. Quart. Master Brew. Ass. Am. 2003, 40, 89–97.
- Evans, D. E.; Collins, H. M.; Eglinton, J. K.; Wilhelmson, A. Assessing the Impact of the Level of Diastatic Power Enzymes and Their Thermostability on the Hydrolysis of Starch during Wort Production to Predict Malt Fermentability. J. Am. Soc. Brew. Chem. 2005, 63, 185–198. DOI: 10.1094/ASBCJ-63-0185.
- Evans, D. E.; Redd, K.; Haraysmow, S. E.; Elvig, N.; Metz, N.; Koutoulis, A. Small Scale Comparison of the Influence of Malt Quality on Malt Brewing with Barley Quality on Barley Brewing Using Ondea Pro. J. Am. Soc. Brew. Chem. 2014, 72, 192–207. DOI: 10.1094/ASBCJ-2014-0630-01.
- Hardie, D. G. Control of Carbohydrate Formation by Gibberellic Acid in Barley Endosperm. Phytochem. 1975, 14, 1719–1722. DOI: 10.1016/0031-9422(75)85281-2.
- Arends, A. M.; Fox, G. P.; Henry, R. J.; Marschke, R. J.; Symons, M. H. Genetic and Environmental Variation in the Diastatic Power of Australian Barley. J. Cereal Sci. 1995, 21, 63–70. DOI: 10.1016/S0733-5210(95)80009-3.
- Evans, D. E.; Dambergs, R.; Ratkowsky, D.; Li, C.; Harasymow, S.; Roumeliotis, S.; Eglinton, J. K. Refining the Prediction of Potential Malt Fermentability by Including an Assessment of Limit Dextrinase Thermostability and Additional Measures of Malt Modification, Using Two Different Methods for Multivariate Model Development. J. Inst. Brew. 2010, 116, 86–97. DOI: 10.1002/j.2050-0416.2010.tb00403.x.
- Eglinton, J. K.; Langridge, P.; Evans, D. E. Thermostability Variation in Alleles of Barley Beta-Amylase. J. Cereal Sci. 1998, 28, 301–309. DOI: 10.1016/S0733-5210(98)90010-8.
- Duke, S. H.; Henson, C. A. Tracking the Progress of Wort Sugar Production during Congress Mashing with North American Barley Cultivars and Comparisons to Wort Osmolyte Concentration and Malt Extract. J. Am. Soc. Brew. Chem. 2011, 69, 200–213. DOI: 10.1094/ASBCJ-2011-0829-01.
- Duke, S. H.; Henson, C. A.; Vinje, M. A. Comparisons of Barley Malt Amylolytic Enzyme Thermostabilities to Work Osmolyte Concentrations, Malt Extract and ASBC Measures of Malt Quality, and Intial Enzyme Activities. J. Am. Soc. Brew. Chem. 2014, 72, 271–284. DOI: 10.1094/ASBCJ-2014-1027-01.
- Henson, C. A.; Duke, S. H. A Comparison of Standard and Nonstandard Measures of Malt Quality. J. Am. Soc. Brew. Chem. 2008, 66, 11–19. DOI: 10.1094/ASBCJ-2007-1210-01.
- Vinje, M. A.; Duke, S. H.; Henson, C. A. Comparison of Factors Involved in Starch Degradation in Barley under Laboratory and Malting Conditions. J. Am. Soc. Brew. Chem. 2015, 73, 195–205. DOI: 10.1094/ASBCJ-2015-0318-01.
- Arakawa, T.; Timasheff, S. N. Stabilization of Protein Structure by Sugars. Biochemistry 1982, 21, 6536–6544. DOI: 10.1021/bi00268a033.
- Back, J. F.; Oakenfull, D.; Smith, M. B. Increased Thermal Stability of Proteins in the Presence of Sugars and Polyols. Biochemistry 1979, 18, 5191–5196. DOI: 10.1021/bi00590a025.
- Frigon, R. P.; Lee, J. C. The Stabilization of Calf-Brain Microtubule Protein by Sucrose. Arch. Biochem. Biophys. 1972, 153, 587–589. DOI: 10.1016/0003-9861(72)90376-1.
- Bamforth, C. W.; Fox, G. P. Critical Aspects of Starch in Brewing. Brewing Sci. 2020, 73, 126–139. DOI: 10.23763/BrSc20-16bamforth.
- Fox, G. P. Starch in Brewing Applications. In Starch in Food, Sjöö, M., Nilsson, L., Eds.; Woodhead Publishing Series in Food Sci. Tech. Nut.: Sawston, Cambridge, 2018; pp 633–659. DOI: 10.1016/B978-0-08-100868-3.00016-0.
- Evans, D. E.; Li, C.; Eglinton, J. K. A Superior Prediction of Malt Attenuation. Eur. Brew. Conv. 31st Congr. Proc. 2007, Venice, presentation #4. CD Fachverlag Hans Carl, Nürenberg.
- Burton, R. A.; Fincher, G. B. Evolution and Development of Cell Walls in Cereal Grains. Front Plant Sci. 2014, 5, 456–471. DOI: 10.3389/fpls.2014.00456.
- Fincher, G. B. Molecular and Cellular Biology Associated with Endosperm Mobilization in Germinating Cereal Grains. Annu. Rev. Plant Physiol. Plant Mol. Biol. 1989, 40, 305–346. DOI: 10.1146/annurev.pp.40.060189.001513.
- Anderson, I. W. The Effect of β-Glucan Molecular Weight on the Sensitivity of Dye Hinding Assay Procedures for β-Glucan Estimation. J. Inst. Brew. 1990, 96, 323. DOI: 10.1002/j.2050-0416.19–90.tb01038.x.326.
- Manzanares, P.; Navarro, A.; Sendra, J. M.; Carbonell, J. V. Selective Determination of β-Glucan of Differing Molecular Size, Using the Calcofluor-Fluourimetric Flow-Injection-Analysis (FIA) Method. J. Inst. Brew. 1991, 97, 101–104. DOI: 10.1002/j.2050-0416.1991.tb01057.x.
- Manzanares, P.; Navarro, A.; Sendra, J. M.; Carbonell, J. V. Determination of the Average Molecular Weight of Barley β-Glucan within the Range 30-100k by the Calcofluor-FIA Method. J. Cereal Sci. 1993, 18, 211–223. DOI: 10.1006/jcrs.1993.1048.
- Stewart, D. C.; Hawthorne, D.; Evans, D. E. Cold Sterile Filtration: A Small Scale Filtration Test and Investigation of Membrane Plugging. J. Inst. Brew. 1998, 104, 321–326. DOI: 10.1002/j.2050-0416.1998.tb01003.x.
- Douglas, S. C. A Rapid Method for the Determination of Pentosans in Wheat Flour. Food Chem. 1981, 7, 139–145. DOI: 10.1016/0308-8146(81)90059-5.
- Stewart, D. C.; Freeman, G.; Evans, D. E. Development and Assessment of a Small-Scale Wort Filtration Test for the Prediction of Beer Filtration Efficiency. J. Inst. Brew. 2000, 106, 361–366. DOI: 10.1002/j.2050-0416.2000.tb00526.x.
- Evans, D. E. A More Cost and Labour Efficient Assay for the Combined Measurement of the Diastatic Power Enzymes, β-Amylase, β-Amylase and Limit Dextrinase. J. Am. Soc. Brew. Chem. 2008, 66, 215–222. DOI: 10.1094/ASBCJ-2008-0909-01.
- Kanauchi, M.; Bamforth, C. W. The Relevance of Different Enzymes for the Hydrolysis of β-Glucans in Malting and Mashing. J. Inst. Brew. 2008, 114, 224–229. DOI: 10.1002/j.2050-0416.2008.tb00332.x.
- Gastl, M.; Kupetz, M.; Becker, T. Determination of Cytolytic Malt Modification – Part I: Influence of Variety Characteristics. J. Am. Soc. Brew. Chem. 2021, 79, 53–65. DOI: 10.1080/03610470.2020.1796156.
- Evans, D. E.; Hamet, M. A. G. The Selection of a Dried Yeast Strain for Use in the Apparent Attenuation Limit Malt Analysis (AAL) Procedure. J. Inst. Brew. 2005, 111, 209–214. DOI: 10.1002/j.2050-0416.2005.tb00668.x.
- Jin, H.; Rogers, P. Novel Recovery of Malt Flavours from Their Glycosidically Bound Precursors. Tech. Quart. Master Brew. Ass. Am. 2000, 37, 79–83.
- Liang, Z.; Zhongxiang Fang, Z.; Pai, A.; Luo, J.; Gan, R.; Gao, Y.; Lu, J.; Zhang, P. Glycosidically Bound Aroma Precursors in Fruits: A Comprehensive Review. Crit. Rev. Food Sci. Nutr. 2020, 60, 1–29. DOI: 10.1080/10408398.2020.1813684.
- Evans, D. E. The Impact of Malt Blending on Extract, Lautering Efficiency and Fermentability. J. Am. Soc. Brew. Chem. 2012, 70, 50–54. DOI: 10.1094/ASBCJ-2011-1208-01.
- Hennemann, M.; Gastl, M.; Becker, T. Inhomogeneity in the Lauter Tun: A Chromatographic View. Eur. Food Res. Technol. 2019, 245, 521–533. DOI: 10.1007/s00217-018-03226-4.
- Kupetz, M.; Gastl, M. Arabioxylan - a Cytolytic Parameter Often Times Disregarded?! Brauwelt Intl. 2020, 38, 10–14.
- Li, Y.; Lu, J.; Gu, G. Control of Arabinoxylan Solubilization and Hydrolysis in Mashing. Food Chem. 2005, 90, 101–108. DOI: 10.1016/j.foodchem.2004.03.031.
- Sadosky, P.; Schwarz, P. B.; Horsley, R. D. Effect of Arabinoxylans, β-Glucans and Dextrins on the Viscosity and Membrane Filterability of a Beer Model System. J. Am. Soc. Brew. Chem. 2002, 60, 153–162. DOI: 10.1094/ASBCJ-60-0153.
- Sun, J.; Lu, J.; Xie, G. Secretome Analysis of Trichoderma reesei CICC41495 for Degradation of Arabinoxylan in Malted Barley. J. Inst. Brew. 2018, 124, 352–358. DOI: 10.1002/jib.505.
- Langenaeken, N. A.; Schutter, D. P.; Courtin, C. M. Arabinoxylan from Non-Malted Cereals Can Act as Mouthfeel Contributor in Beer. Carbohydr. Polym. 2020, 239, 116257. DOI: 10.1016/j.carbpol.2020.116257.
- Krebs, G.; Becker, T.; Gastl, M. Characterization of Polymeric Substance Classes in Cereal-Based Beverages Using Asymmetrical Flow Field-Flow Fractionation with a Multi-Detection System. Anal. Bioanal. Chem. 2017, 409, 5723–5734. DOI: 10.1007/s00216-017-0512-6.
- Evans, D. E.; Li, C.; Eglinton, J. K. Improved Prediction of Malt Fermentability by the Measurement of the Diastatic Power Enzymes, β-Amylase, α-Amylase and Limit Dextrinase. I. Survey of the Levels of Diastatic Power Enzymes in Commercial Malts. J. Am. Soc. Brew. Chem. 2008, 66, 223–232. DOI: 10.1094/ASBCJ-2008-0909-02.
- Yousif, A. M.; Evans, D. E. Changes in Malt Quality during Production in Two Commercial Maltings. J. Inst. Brew. 2020, 126, 233–252. DOI: 10.1002/jib.609.
- Evans, D. E.; Fox, G. P. The Comparison of DP Enzyme Release and Persistence with the Production of Yeast Fermentable Sugars during Modified IoB 65 °C and Congress Programmed Mashes. J. Am. Soc. Brew. Chem. 2017, 75, 302–311. DOI: 10.1094/ASBCJ-2017-4707-01.
- Verstrepen, K. J.; Derdelinckx, G.; Dufour, J.-P.; Winderickx, J.; Thevelein, J. M.; Pretorius, I. S.; Delvaux, F. R. Flavor-Active Esters: Adding Fruitiness to Beer. J. Biosci. Bioeng. 2003, 96, 110–118. DOI: 10.1016/S1389-1723(03)90112-5.
- Krebs, G.; Müller, M.; Becker, T.; Gastl, M. Characterization of the Macromolecular and Sensory Profile of Non-Alcoholic Beers Produced With Various Methods. Food Res. Int. 2019, 116, 508–517. DOI: 10.1016/j.foodres.2018.08.067.
- Langstaff, S. A.; Lewis, M. J. The Mouthfeel of Beer - a Review. J. Inst. Brew. 1993, 99, 31–37. DOI: 10.1002/j.2050-0416.1993.tb01143.x.
- Ragot, F.; Guinard, J. X.; Shoemaker, C. F.; Lewis, M. J. The Contribution of Dextrins to Beer Sensory Properties. Part 1 Mouthfeel. J. Inst. Brew. 1989, 95, 427–430. DOI: 10.1002/j.2050-0416.1989.tb04650.x.
- Rubsam, H.; Gastl, M.; Becker, T. Influence of the Range of Molecular Weight Distribution of Beer Components on the Intensity of Palate Fullness. Eur. Food Res. Technol. 2013, 236, 65–75. DOI: 10.1007/s00217-012-1861-1.
- McCafferty, C. A.; Perch-Nielsen, N.; Bryce, J. H. Effects of Aerobic and Anaerobic Germination on the Debranching Enzyme, Limit Dextrinase, in Barley Malt. J. Am. Soc. Brew. Chem. 2000, 58, 47–50. DOI: 10.1094/ASBCJ-58-0047.
- Axcell, B. C.; Tulej, R.; Mulder, C. J. The Influence of the Malting Process on Malt Fermentability Performance. In Proc. Inst. Brew. (Australia & New Zealand) 19th Conv. Hobart, 1986, 63–69.
- Axcell, B. C.; Van Nierop, S.; Vundla, W. Malt Induced Premature Flocculation. Tech. Quart. Master Brew. Ass. Am. 2000, 37, 501–507.
- Hu, S.; Fan, W.; Dong, J.; Yin, H.; Yu, J.; Liu, J.; Huang, S.; Huang, S.; Zhang, C. Validation and Application of Osmolyte Concentration as an Indicator to Evaluate Fermentability of Wort and Malt. J. Inst. Brew. 2017, 123, 488–496. DOI: 10.1002/jib.454.
- Fox, G. P.; Staunton, M.; Agnew, E.; D’Arcy, B. Effect of Varying Starch Properties and Mashing Conditions on Wort Sugar Profiles. J. Inst. Brew. 2019, 125, 412–421. DOI: 10.1002/jib.585.
- Hu, S.; Yu, J.; Dong, J.; Evans, D. E.; Liu, J.; Huang, S.; Huang, S.; Fan, W.; Yin, H.; Li, M. Relationship between Levels of Diastatic Power Enzymes and Wort Sugar Production from Different Barley Cultivars during the Commercial Mashing Process of Brewing. Starch 2014, 66, 615–623. DOI: 10.1002/star.201300152.
- Langenaeken, N. A.; De Schepper, C. F.; De Schutter, D. P.; Courtin, C. M. Different Gelatinization Characteristics of Small and Large Barley Starch Granules Impact Their Enzymatic Hydrolysis and Sugar Production during Mashing. Food Chem. 2019, 295, 138–146. DOI: 10.1016/j.foodchem.2019.05.045.
- Langenaeken, N. A.; de Schepper, C. F.; de Schutter, D. P.; Courtin, C. M. Carbohydrate Content and Structure during Malting and Brewing: A Mass Balance Study. J. Inst. Brew. 2020, 126, 253–262. DOI: 10.1002/jib.619.
- Balet, S.; Guelpa, A.; Fox, G. P.; Manley, M. Rapid Visco Analyser (RVA) as a Tool for Measuring Starch-Related Physiochemical Properties in Cereals: A Review. Food Anal. Methods 2019, 12, 2344–2360. DOI: 10.1007/s12161-019-01581-w.
- Fox, G. P.; Visser, J.; Skov, T.; Meijering, I.; Manley, M. Effect of Different Analysis Conditions of a Rapid Visco Analyser Malt Viscograms in Relation to Malt of Varying Fermentability. J. Inst. Brew. 2014, 120, 183–192. DOI: 10.1002/jib.137.
- MacGregor, A. W.; Bazin, S. L.; Macri, L. J.; Babb, J. C. Modelling the Contribution of Alpha-Amylase, Beta-Amylase and Limit Dextrinase to Starch Degradation during Mashing. J. Cereal Sci. 1999, 29, 161–169. DOI: 10.1006/jcrs.1998.0233.
- Edney, M. J.; Eglinton, J. K.; Collins, H. M.; Barr, A. R.; Legg, W. G.; Rossnagel, B. G. Effects of the High Heat Stable Beta-Amylase Allele on Fermentability of Malts with Low vs High Diastatic Power. Eur. Brew. Conv. 31st Cong. Proc 2007, Venice, paper #50 CD Fachverlag Hans Carl, Nürenberg.
- Erdal, K.; Jensen, M. O.; Kristensen, M.; Krough, J. J.; Riis, P.; Vaag, P. Total β-Amylase in Barley Used as a Screening Criteria for Combined Amylolytic Activity in Malt. Eur. Brew. Conv. Cong. Proc. Oslo; IRL Press: Oxford, 1993; pp 147–154.
- Gibson, T. S.; Solah, V.; Glennie Holmes, M. R.; Taylor, H. R. Diastatic Power in Malted Barley: contributions of Malt Parameters to Its Development and the Potential of Barley Grain β-Amylase to Predict Malt Diastatic Power. J. Inst. Brew. 1995, 101, 277–280. DOI: 10.1002/j.2050-0416.1995.tb00867.x.
- Lin, Y. K.; Yu, Z. L. Correction Analysis of Beta-Amylase Activity in Mature Barley Seeds and Malt Diastatic Power. Hereditas - Beijing 1990, 12, 12–14.
- Santos, M. M. M.; Riis, P. Optimized McCleary Method for Measurement of Total β-Amylase in Barley and Its Applicability. J. Inst. Brew. 1996, 102, 271–275. DOI: 10.1002/j.2050-0416.1996.tb00912.x.
- Ioannidis, J. P. A. Why Most Published Research Findings Are False. PLoS Med. 2005, 2, e124. DOI: 10.1371/journal.pmed.0020124.
- Huerta-Zurita, R.; Barr, J.; Horsley, R. D.; Schwarz, P. B. Predicting Malt Fermentability in Malting Barley Breeding Lines. J. Am. Soc. Brew. Chem. 2020, 78, 50–62. DOI: 10.1080/03610470.2019.1670037.
- Ghahramani, Z. Probabilistic Machine Learning and Artificial Intelligence. Nature (London) 2015, 521, 452–459. DOI: 10.1038/nature14541.
- Jordan, M. I.; Mitchell, T. M. Machine Learning: Trends, Perspectives, and Prospects. Science 2015, 349, 255–260. DOI: 10.1126/science.aaa8415.
- Cooper, C.; Evans, D. E.; Yousif, A.; Metz, N.; Koutoulis, A. Comparison of the Impact on Performance of Small-Scale Mashing with Different Proportions of Unmalted Barley, Ondea Pro®, Malt and Rice. J. Inst. Brew. 2016, 122, 218–227. DOI: 10.1002/jib.325.