Abstract
Seven new O-methylated theaflavins (TFs) were synthesized by using O-methyltransferase from an edible mushroom. Using TFs and O-methylated TFs, metabolic stability in pooled human liver S9 fractions and inhibitory effect on H2O2-induced oxidative damage in human HepG2 cells were investigated. In O-methylation of theaflavin 3′-O-gallate (TF3′G), metabolic stability was potentiated by an increase in the number of introduced methyl groups. O-methylation of TF3,3′G did not affect metabolic stability, which was likely because of a remaining 3-O-galloyl group. The inhibitory effect on oxidative damage was assessed by measuring the viability of H2O2-damaged HepG2 cells treated with TFs and O-methylated TFs. TF3,3′G and O-methylated TFs increased cell viabilities significantly compared with DMSO, which was the compound vehicle (p < 0.05), and improved to approximately 100%. Only TF3′G did not significantly increase cell viability. It was suggested that the inhibitory effect on H2O2-induced oxidative damage was potentiated by O-methylation or O-galloylation of TFs.
Graphical Abstract
Seven new O-methylated theaflavins (3–9) were synthesized. Metabolic stability and inhibitory effect of O-methylated theaflavins on H2O2-induced oxidative damage in human HepG2 cells were investigated.
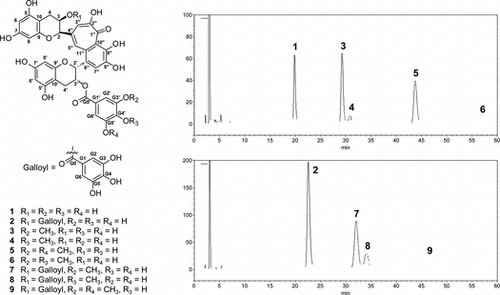
Introduction
Black tea is a very popular beverage in many parts of the world. Functional activities of theaflavins (TFs), a type of black tea polyphenols, have been reported in various studies, such as antioxidant activity in 2,2-diphenyl-1-picrylhydrazyl (DPPH) radical and H2O2-induced oxidative damage system,Citation1) the inhibitory effect of intracellular reactive oxygen species,Citation2) and DNA damage by oxidative stress,Citation3) etc. The ability of food antioxidants, such as TFs, to reduce oxidative damage has been investigated. It has been reported that black tea contains O-methylated TFs, such as theaflavin 3-O-(3-O-methyl)gallate (3MeTF3G) and theaflavin 3-O-(3-O-methyl)gallate,3′-O-gallate, which are formed by oxidative coupling of epicatechin (3-O-gallate) (EC or ECG) and epigallocatechin 3-O-(3-O-methyl)gallate (EGCG3′′Me).Citation4) Our recent study revealed that O-methylated TFs inhibit the intracellular accumulation of triglycerides from terminally differentiated human visceral adipocytes.Citation5) Ishiyama et al. have reported that the O-methylation of TFs decreases peroxyl and DPPH radical scavenging activity.Citation6) It is suggested that the number of hydroxyl groups is directly involved with the radical scavenging activity. However, when an inhibitory effect on oxidative damage in cells is considered, O-methylation of TFs is expected to be effective because it has been reported that O-methylated polyphenols have high metabolic stability in pooled human liver S9 fractions and absorbability in Caco-2 cells.Citation7) Herein, we describe the synthesis of O-methylated TFs and investigations of metabolic stability and the inhibitory effect on oxidative damage in H2O2-damaged human hepatoblastoma G2 (HepG2) cells.
Materials and methods
Chemicals, plant materials, instruments
All solvents (high or HPLC grade) were purchased from Wako Pure Chemical Industries, Ltd. (Osaka, Japan). Theaflavin 3′-O-gallate (TF3′G) and theaflavin 3,3′-di-O-gallate (TF3,3′G) were purchased from Funakoshi Co. (Tokyo, Japan). Commercially prepared black tea leaves and an edible mushroom (Flammulina velutipes) were obtained from a supermarket in Japan. Time-of-flight mass spectrometry (TOFMS) was performed on a QSTAR Elite (AB SCIEX, Tokyo, Japan) equipped with an electrospray ionization source. TOFMS analysis was collected by Analyst QS 2.0 software. The TurboIonSpray source was set to 450 °C and the scanning range was m/z 100–1000. The optimized instrument settings were as follows: ion spray voltage, −4500 V; auxiliary gas, 50 psi; nebulizer gas, 50 psi; nitrogen curtain gas, 30 psi; declustering potential, −30 V; focusing potential, −250 V; declustering potential 2, −15 V; accumulation time, 1 s. Nuclear magnetic resonance (NMR) spectra were recorded using a Bruker AV600 instrument (Bruker Biospin GmbH, Rheinstetten, Germany). NMR acquisition was collected by TopSpin2 software. 1H NMR (600 MHz), 13C NMR (150 MHz), heteronuclear single quantum coherence (HSQC), and heteronuclear multiple bond correlation (HMBC) spectra were recorded at ambient temperature, and the chemical shifts are given in δ (ppm) relative to the solvent signal (acetone-d6, δH 2.05; δC 29.8). A Sep-Pak C18 35 cc Vac Cartridge (Waters Corp., Milford, MA, USA) and an Inertsil ODS-3 (4.6 mm i.d. or 20 mm i.d. × 250 mm, GL Sciences Inc., Tokyo, Japan) were used for column chromatography. The purification of compounds was carried out with a Hitachi HPLC system (Tokyo, Japan), an L-7150 pump, an L-7420 UV–vis detector, and a D-2500 chromato-integrator. The analysis of compounds was carried out with a Hitachi LaChrom Elite system, an L-2130 pump, an L-2450 diode-array detector, an L-2350 column oven, and an L-2200 autosampler.
Preparation of theaflavins
TF3′G and TF3,3′G (1 and 2) were prepared from black tea leaves by modifying a previous method.Citation4) About 200 g of black tea leaves were extracted three times with 1500 mL of 50% EtOH containing 2% L(+)-ascorbic acid (ASA) by sonicating for 20 min. The extracts were centrifuged (3000 rpm, 1673 × g, 4 °C, 5 min) and 50% EtOH was evaporated. The extracts were applied to the Sep-Pak C18 35 cc Vac Cartridge. The column was rinsed with 80 mL of 15% EtOH and TFs were eluted with 80 mL of 40% EtOH. After evaporation of the eluate, the residue was dissolved with 40% EtOH and applied to the Inertsil ODS-3 column (20 mm i.d. × 250 mm) for fractionation. The mobile phase was acetonitrile–EtOAc–0.05% H3PO4 (21:3:76). The flow rate was 12 mL/min and the detection was 280 nm. The required peaks of 1 and 2 were collected and evaporated. They were purified in the Sep-Pak C18 by rinsing with water and eluting with ethanol to remove H3PO4. After lyophilization, 1 (0.57 g) and 2 (0.73 g) were obtained.
Synthesis and purification of O-methylated theaflavins
For the synthesis of O-methylated TFs, the O-methyltransferase was prepared from an edible mushroom (F. velutipes) according to the method described in our recent paper.Citation5) The stalk of an edible mushroom was cut, and the surfaces were disinfected with 0.5% hypochlorous acid for 15 min. The internal stalk was cut off and washed with sterilized water. After a small piece of the stalk was cultured in potato dextrose agar medium (Becton Dickinson, Sparks, MD, USA) at 25 °C, the mycelium was isolated. The mycelium was cultured in a liquid culture medium (0.02% glucose, 0.01% peptone, 0.002% yeast extract, 0.002% KH2PO4, and 0.001% MgSO4) at 28 °C with shaking. The broth was filtered through gauze, and the cultured mycelium was homogenized with a buffer (pH 7.5) containing 20 mM Tris–HCl, 1 mM dithiothreitol, 1 mM EDTA, and 10% glycerol for extraction. After the homogenate was centrifuged (10,000 rpm, 18,592 × g, 4 °C, 10 min), a crude enzyme solution was obtained. O-methylated TFs were synthesized by the enzymatic reaction in the presence of TFs, the crude enzyme, and S-adenosyl-L-methionine (SAM) as a methyl donor. The reaction mixture (1000 mL) contained 20 mM phosphate buffer (pH 6.5), 2.5 mM MgCl2, 0.05 mM TFs, 0.5 mM SAM, 0.04% ASA, and 3.3% of crude enzyme solution. The mixture was incubated at 37 °C for 8 h and then the reaction was stopped with the addition of 24 mL of 1 N HCl. The enzymatic reaction solution including O-methylated TFs was extracted three times with EtOAc. Removal of EtOAc by evaporation gave the mixture solid of O-methylated TFs. The mixture solid was dissolved in 40% EtOH and applied to the Inertsil ODS-3 column (20 mm i.d. × 250 mm). After purification, similar to the procedure for purifying 1 and 2, O-methylated TFs were obtained.
Metabolic stability
The metabolic stability of TFs was investigated in a pooled human liver S9 fraction (pH 7.5) to estimate metabolism, i.e. glucuronidation, sulfation, and oxidation, in the liver.Citation7) The 50 μL reaction mixture contained pooled human S9 fractions (50 μg of protein), 1 mM uridine 5′-diphosphoglucuronic acid, 0.1 mM 3′-phosphoadenosine-5′-phosphosulfate, 1 mM NADPH, 50 mM Tris–HCl buffer (pH 7.5), 10 mM MgCl2, 0.063% bovine serum albumin, 8 mM dithiothreitol, and 5 μM TFs. After incubation at 37 °C for 0–60 min, the reaction was stopped with the addition of 45 μL of ice-cold methanol and 5 μL of 1% ASA. The reaction solution was centrifuged at 14,000 × g for 3 min. The supernatants were applied to the Inertsil ODS-3 column (4.6 mm i.d. × 250 mm) for HPLC analysis. The mobile phase was same as for the purification procedure. The flow rate was 1.0 mL/min and the column temperature was 50 °C. The residual ratios of TFs were analyzed. The values were expressed as means ± standard error of the mean (SEM) for triplicate experiments. Comparisons between groups at the same incubation time were performed using the Tukey test, and p < 0.05 was considered to be significant.
Viability of H2O2-damaged human HepG2 cells
The inhibitory effect of TFs on oxidative damage was investigated by measuring the viability of H2O2-damaged HepG2 cells. Cell viability assay was carried out by modifying a previous method.Citation8) HepG2 cells (40,000 cells/well, DS Pharma Biomedical Co., Ltd., Osaka, Japan) cultured in Dulbecco’s modified Eagle medium (DMEM, Wako Pure Chemical Industries, Ltd., Osaka, Japan) containing 10% heat-inactivated fetal bovine serum (SAFC, Brooklyn, Australia), 100 unit/mL penicillin (Life Technologies Japan, Tokyo, Japan), and 100 μg/mL streptomycin (Life Technologies Japan, Tokyo, Japan) were divided into 96-well plates and incubated with 5% CO2 at 37 °C for 24 h. During incubation, cells were treated with 0.2 μM TFs. The control cells were treated with DMSO, which was a vehicle of TFs. The culture supernatants were removed and oxidative damage was caused by addition of 150 μL of DMEM containing 12 mM H2O2 instead of TFs. The control cells were treated with DMEM that did not contain H2O2. After 2 h incubation, the medium was removed and 200 μL of DMEM containing 10% (v/v) alamarBlue (Life Technologies Japan, Tokyo, Japan) was added and incubated for 3 h. Cell viability was determined by the fluorescence with excitation at 544 nm and emission at 590 nm. The values were evaluated as a percentage of the control cells and expressed as means ± SEM (n = 6). Comparisons between groups were performed using the Tukey test, and p < 0.05 was considered to be significant.
Results and discussion
Structures of O-methylated theaflavins
TOFMS and NMR analyses were conducted to investigate the number and sites of introduction of methyl groups incorporated in 1 and 2. Initially, the O-methylation of 1 was examined and compounds 3–6 were obtained (Fig. ). Compounds 3 and 4 were obtained as orange amorphous powders. They were shown to be isomers on the basis of their molecular formulas (C37H30O16) obtained from TOFMS (m/z 729.1456 [M–H]− for 3 and 729.1464 [M–H]− for 4). 1H NMR spectra of 3 and 4 were similar to that of 1, except for the methoxy proton signals. Moreover, a difference in the chemical shifts of H-G2′ and H-G6′, the meta-coupled signals (δ 6.97 and 7.07) for 3, and the two-proton singlet signals (δ 6.93) for 4 indicated that 3 and 4 had asymmetrical and symmetrical methyl galloyl groups, respectively. An asymmetrical methylgalloyl group of 3 and a symmetrical methylgalloyl group of 4 were also suggested by 13C NMR spectra. In the HMBC spectrum of 3, the methoxy proton and H-G2′ (δ 6.97) correlated to C-G3′. Therefore, 3 was established as theaflavin 3′-O-(3-O-methyl)gallate (3MeTF3′G) (Fig. , Tables and ). The 13C NMR spectrum of 4 was not observed because of the small amount of the compound available; however, HSQC experiments showed a chemical shift of methoxy carbon (δ 60.5). The two-proton singlet signals of H-G2′ and H-G6′, and the HMBC correlation of the methoxy proton to a carbon signal at δ 139.9 considered to be C-G4′ indicated that 4 had the 4-O-methylgalloyl group.Citation9) Therefore, 4 was identified as theaflavin 3′-O-(4-O-methyl)gallate (4MeTF3′G) (Fig. , Table ). Compounds 5 and 6 were obtained as orange amorphous powders and were indicated to be isomers on the basis of their molecular formulas (C38H32O16) obtained from TOFMS (m/z 743.1625 [M–H]− for 5 and 743.1642 [M–H]− for 6). 1H NMR spectra of 5 and 6 were similar to that of 1, except for the methoxy proton signals, H-G2′, and H-G6′. Compound 5 was identified as theaflavin 3′-O-(3,5-di-O-methyl)gallate (3,5diMeTF3′G) (Fig. , Tables and ) because of the downfield shift of H-G2′ (H-G6′) and the HMBC correlation of the methoxy proton signal with C-G3′ (C-G5′). Compound 6 was identified as theaflavin 3′-O-(3,4-di-O-methyl)gallate (3,4diMeTF3′G) (Fig. , Tables and ) because of the meta-coupled signals of H-G2′ and H-G6′, and the HMBC correlations from the methoxy proton signals and H-G2′ to C-G3′ and C-G4′.
Fig. 1. Structures of 1–9 and HPLC chromatograms of the enzymatic reaction solution of 1 and 2.
Notes: Peaks are 1, TF3′G; 2, TF3,3′G; 3, 3MeTF3′G; 4, 4MeTF3′G; 5, 3,5diMeTF3′G; 6, 3,4diMeTF3′G; 7, 3MeTF3,3′G; 8, 4MeTF3,3′G; 9, 3,5diMeTF3,3′G.
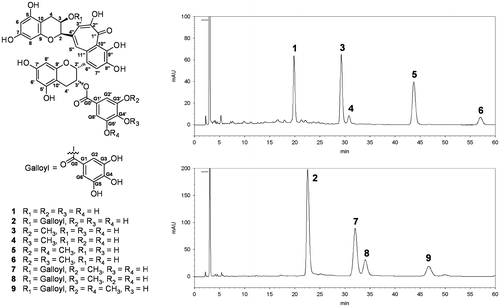
Table 1. 13C NMR spectroscopic data (δC, 150 MHz, CD3OD) for 3, 5–9.
Table 2. 1H NMR spectroscopic data (δH, 600 MHz, CD3OD) for 3–6.
Furthermore, the O-methylation of 2 was examined, and compounds 7–9 were obtained (Fig. ). Compound 7 was obtained as an orange amorphous powder. Its molecular formula was deduced to be C44H34O20 on the basis of the TOFMS (m/z 881.1544 [M–H]−), indicating that it was monomethylated TF3,3′G. 1H NMR spectrum of 7 was similar to that of 2 except for the methoxy proton signal and the meta-coupled signals of H-G2′ and H-G6′. Compound 7 was identified as theaflavin 3-O-gallate,3′-O-(3-O-methyl)gallate (3MeTF3,3′G) (Fig. , Tables and ) because HMBC experiments showed the correlation of the methoxy proton signal and H-G2′ to C-G3′. Compounds 8 and 9 were obtained as orange amorphous powders. TOFMS (m/z 881.1564 [M–H]− for 8 and 895.1720 [M–H]− for 9) confirmed the molecular formulas C44H34O20 and C45H36O20, respectively. 1H NMR spectra of 8 and 9 were also similar to that of 2 except for the chemical shifts of the two-proton singlet signals (H-G2′ and H-G6′) and the presence and integral value of the methoxy proton signal. Compound 8 was identified as theaflavin 3-O-gallate,3′-O-(4-O-methyl)gallate (4MeTF3,3′G) (Fig. , Tables and ) by HMBC correlations of the methoxy proton signal to C-G4′. Compound 9 was identified as theaflavin 3-O-gallate,3′-O-(3,5-di-O-methyl)gallate (3,5diMeTF3,3′G) (Fig. , Tables and ) by HMBC correlations of the methoxy proton signal to C-G3′ (C-G5′).
Table 3. 1H NMR spectroscopic data (δC, 600 MHz, CD3OD) for 7–9.
In this study, a new monomethylated TF3,3′G, theaflavin 3-O-gallate,3′-O-(3-O-methyl)gallate (7) rather than a known theaflavin 3-O-(3-O-methyl)gallate,3′-O-gallateCitation4) was synthesized by the O-methylation of 2. It is suggested that the O-methyltransferase used in this study methylated 3′-O-gallate rather than 3-O-gallate. Compounds 3, 4, 7, and 8 are new compounds possibly contained in black tea because epigallocatechin, EGCG, epicatechin 3-O-(3-O-methyl)gallate, and epicatechin 3-O-(4-O-methyl)gallate,Citation10) which were their precursors, have been identified in Camellia sinensis. Compounds 3, 4, 7, and 8 may be identified in black tea by an analytical technique, such as liquid chromatography–tandem mass spectrometry; however, they were not identified by HPLC analysis. On the other hand, it is very unlikely that 5, 6, and 9 are contained in black tea because epicatechin 3-O-(3,5-di-O-methyl)gallateCitation11) and epicatechin 3-O-(3,4-di-O-methyl)gallate,Citation12) which were their precursors, have not been identified in C. sinensis.
Metabolic stability of O-methylated theaflavins
Previous studies have shown that O-methylated polyphenols have much higher metabolic stability than their corresponding unmethylated polyphenols.Citation7) Therefore, the metabolic stability of TFs and O-methylated TFs was examined, except for 4MeTF3′G, 3,4diMeTF3′G, and 4MeTF3,3′G because of their small amounts (Fig. ). After incubation for 15 min, 3,5diMeTF3′G was significantly more stable than TF3′G (p < 0.05). The difference of residual ratios of the other TFs was not assessed. After incubation for 30 min, 3MeTF3′G and 3,5diMeTF3′G were significantly more stable than TF3′G (p < 0.05). After incubation for 60 min, the order of stability in the series of O-methylation of TF3′G was 3,5diMeTF3′G (67.3%) > 3MeTF3′G (56.3%) > TF3′G (42.0%) (p < 0.05). It was suggested that the O-methylation of the galloyl group improved the metabolic stability of TF3′G because of inhibiting the oxidation by the cytochrome system as well as dissolved oxygen and the conjugation by sulfatase and glucuronidase.Citation7) On the other hand, O-methylation of TF3,3′G did not consistently affect metabolic stability over a period of 60 min, which was likely because of a remaining 3-O-galloyl group. In the previous study, it was reported that the oral bioavailability of theaflavins was very low.Citation13) O-Methylation of theaflavins may increase their oral bioavailability because of the improved metabolic stability.
Fig. 2. Metabolic stabilities of 1–3, 5, 7, and 9.
Notes: The values were calculated as the residual ratios of compounds, and expressed as means ± SEM for triplicate experiments. Comparisons between groups at the same incubation time were performed. Different symbols above bars indicate significant difference (p < 0.05).
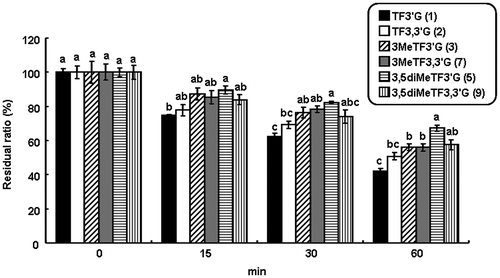
Inhibitory effect of O-methylated TFs on oxidative damage in H2O2-damaged HepG2 cells
It has been suggested that the number of hydroxyl groups is important in DPPH radical scavenging activity by comparison with EGCG, ECG, and EGCG3′′Me,Citation14) or TFs and O-methylated TFs.Citation6) In the case of DPPH radical scavenging assay, direct reaction of the DPPH radical and antioxidants was observed. However, in the case of inhibitory effect on oxidative damage in cells, the involvement of several factors, such as metabolic stability and absorption of the antioxidants, was also considered. In this study, the inhibitory effect on oxidative damage was assessed by measuring the viability of H2O2-damaged HepG2 cells treated with O-methylated TFs, which were expected to be effective for the factors. TFs and O-methylated TFs, except for 4MeTF3′G, 3,4diMeTF3′G, and 4MeTF3,3′G, were also examined. H2O2-treatment of HepG2 cells significantly decreased cell viability, which depended on H2O2 concentration (Fig. (A)). In the cells treated with TF3,3′G and all O-methylated TFs, viabilities were significantly high compared with the cells treated with DMSO (80.2%), which was the compound vehicle (p < 0.05), and were improved to approximately 100%; TF3,3′G (96.1%), 3MeTF3′G (100.4%), 3MeTF3,3′G (107.3%), 3,5diMeTF3′G (98.9%), and 3,5diMeTF3,3′G (111.9%) (Fig. (B)). However, the viabilities of the cells treated with TF3′G (90.0%) tended to be higher but not significantly different from the cells treated with DMSO. The difference of viabilities in relation to the number of methyl groups among the other O-methylated TF3′Gs was not assessed. It was suggested that the inhibitory effect on oxidative damage was potentiated by O-methylation or O-galloylation of TFs because 3,5diMeTF3,3′G improved cell viability compared with the corresponding TF3,3′G and 3,5diMeTF3′G (p < 0.05).
Fig. 3. Inhibitory effect of O-methylated TFs on oxidative damage in H2O2-damaged HepG2 cells.
Notes: (A) Estimation of cell viability by H2O2-treatment. (B) Inhibitory effects of 1–3, 5, 7, and 9 on oxidative damage in H2O2-damaged HepG2 cells. The values were calculated as a percentage of the control cells, and shown as means ± SEM (n = 6). Different symbols above bars indicate significant difference (p < 0.05).
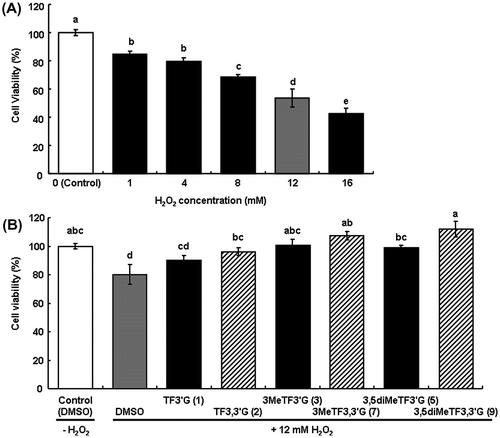
In conclusion, this study revealed that metabolic stability and the inhibitory effect on oxidative damage were potentiated by O-methylation or O-galloylation of TFs. A previous study suggests that O-methylation of polyphenol improves absorption and accumulation of polyphenol in cells,Citation15) and reportedly, the O-galloylation of TF3G and 3MeTF3G increases DPPH radical scavenging activity and lipid solubility.Citation6) It was suggested that not only the radical scavenging activity but also the metabolic stability, absorption, and accumulation of antioxidants were possibly involved in the inhibitory effect on oxidative damage in cells.
Notes
Abbreviations: TF, theaflavin; TF3′G, theaflavin 3′-O-gallate; TF3,3′G, theaflavin 3-O-gallate,3′-O-gallate; DMSO, dimethylsulfoxide; DPPH, 2,2-diphenyl-1-picrylhydrazyl; 3MeTF3G, theaflavin 3-O-(3-O-methyl)gallate; EC, epicatechin; ECG, epicatechin 3-O-gallate; EGCG3′′Me, epigallocatechin 3-O-(3-O-methyl)gallate; HepG2, hepatoblastoma G2; TOFMS, Time-of-flight mass spectrometry; NMR, Nuclear magnetic resonance; HSQC, heteronuclear single quantum coherence; HMBC, heteronuclear multiple bond correlation; ASA, L-(+)-ascorbic acid; SAM, S-adenosyl-L-methionine; SEM, standard error of the mean; DMEM, Dulbecco’s modified Eagle medium; 3MeTF3′G, theaflavin 3′-O-(3-O-methyl)gallate; 4MeTF3′G, theaflavin 3′-O-(4-O-methyl)gallate; 3,5diMeTF3′G, theaflavin 3′-O-(3,5-di-O-methyl)gallate; 3,4diMeTF3′G, theaflavin 3′-O-(3,4-di-O-methyl)gallate; 3MeTF3,3′G, theaflavin 3-O-gallate,3′-O-(3-O-methyl)gallate; 4MeTF3,3′G, theaflavin 3-O-gallate,3′-O-(4-O-methyl)gallate; 3,5diMeTF3,3′G, theaflavin 3-O-gallate,3′-O-(3,5-di-O-methyl)gallate.
References
- Yang Z, Tu Y, Xia H, Jie G, Chen X, He P. Suppression of free-radicals and protection against H2O2-induced oxidative damage in HPF-1 cell by oxidized phenolic compounds present in black tea. Food Chem. 2007;105:1349–1356.10.1016/j.foodchem.2007.05.006
- Lin JK, Chen PC, Ho CT, Lin-Shiau SY. Inhibition of xanthine oxidase and suppression of intracellular reactive oxygen species in HL-60 cells by theaflavin-3,3′-digallate, (-)-epigallocatechin-3-gallate, and propyl gallate. J. Agric. Food Chem. 2000;48:2736–2743.10.1021/jf000066d
- Feng Q, Torii Y, Uchida K, Nakamura Y, Hara Y, Osawa T. Black tea polyphenols, theaflavins, prevent cellular DNA damage by inhibiting oxidative stress and suppressing cytochrome P450 1A1 in cell cultures. J. Agric. Food Chem. 2002;50:213–220.10.1021/jf010875c
- Nishimura M, Ishiyama K, Watanabe A, Kawano S, Miyase T, Sano M. Determination of theaflavins including methylated theaflavins in black tea leaves by solid-phase extraction and HPLC analysis. J. Agric. Food Chem. 2007;55:7252–7257.10.1021/jf070312m
- Tanaka Y, Kirita M, Miyata S, Abe Y, Tagashira M, Kanda T, Maeda-Yamamoto M. O-Methylated theaflavins suppress the intracellular accumulation of triglycerides from terminally differentiated human visceral adipocytes. J. Agric. Food Chem. 2013;61:12634–12639.10.1021/jf404446h
- Ishiyama K, Nishimura M, Deguchi M, Terasaka E, Miyase T, Sano M. Enzymatic preparation of methylated theaflavins and their antioxidant activities. Nippon Shokuhin Kagaku Kogaku Kaishi (in Japanese). 2013;60:339–346.10.3136/nskkk.60.339
- Wen X, Walle T. Methylated flavonoids have greatly improved intestinal absorption and metabolic stability. Drug Metabol. Dispos. 2006;34:1786–1792.10.1124/dmd.106.011122
- Puiggròs F, Llópiz N, Ardévol A, Bladé C, Arola L, Salvadó MJ. Grape seed procyanidins prevent oxidative injury by modulating the expression of antioxidant enzyme systems. J. Agric. Food Chem. 2005;53:6080–6086.10.1021/jf050343m
- Okushio K, Suzuki M, Matsumoto N, Nanjo F, Hara Y. Methylation of tea catechins by rat liver homogenates. Biosci. Biotechnol. Biochem. 1999;63:430–432.10.1271/bbb.63.430
- Dou J, Lee VSY, Tzen JTC, Lee MR. Identification and comparison of phenolic compounds in the preparation of oolong tea manufactured by semifermentation and drying processes. J. Agric. Food Chem. 2007;55:7462–7468.10.1021/jf0718603
- Gao S, Feng N, Yu S, Yu D, Wang X. Vasodilator constituents from the roots of Lysidice rhodostega. Planta Med. 2004;70:1128–1134.10.1055/s-2004-835839
- Watanabe M. Catechins as antioxidants from buckwheat (Fagopyrum esculentum Moench) groats. J. Agric. Food Chem. 1998;46:839–845.10.1021/jf9707546
- Mulder TPJ, Van Platerink CJ, Wijnand Schuyl PJ, Van Amelsvoort JMM. Analysis of theaflavins in biological fluids using liquid chromatography-electrospray mass spectrometry. J. Chromatogr. B. 2001;760:271–279.10.1016/S0378-4347(01)00285-7
- Kirita M, Honma D, Tanaka Y, Usui S, Shoji T, Sami M, Yokota T, Tagashira M, Muranaka A, Uchiyama M, Kanda T, Maeda-Yamamoto M. Cloning of a novel O-methyltransferase from camellia sinensis and synthesis of O-methylated EGCG and evaluation of their bioactivity. J. Agric. Food Chem. 2010;58:7196–7201.10.1021/jf100493s
- Spencer JPE, Abd El Mohsen MM, Rice-Evans C. Cellular uptake and metabolism of flavonoids and their metabolites: Implications for their bioactivity. Arch. Biochem. Biophys. 2004;423:148–161.10.1016/j.abb.2003.11.010