Abstract
The genus Bifidobacterium is well known to have beneficial health effects. We discovered that quercetin and related polyphenols enhanced the secretion of anti-inflammatory substances by Bifidobacterium adolescentis. This study investigated characteristics of the anti-inflammatory substances secreted by B. adolescentis. The culture supernatant of B. adolescentis with quercetin reduced the levels of inflammatory mediators in activated macrophages. Spontaneous quercetin degradant failed to increase anti-inflammatory activity, while the enhancement of anti-inflammatory activity by quercetin was sustained after washout of quercetin. Physicochemical treatment of the culture supernatant indicated that its bioactive substances may be heat-stable, non-phenolic, and acidic biomolecules with molecular weights less than 3 kDa. Acetate and lactate have little or no effect on nitric oxide production. Taken together, the anti-inflammatory substances secreted by B. adolescentis may be small molecules but not short chain fatty acids. In agreement with these findings, stearic acid was tentatively identified as a bioactive candidate compound.
The anti-inflammatory substances secreted by B. adolescentis may be unique biomolecules but not short chain fatty acids.
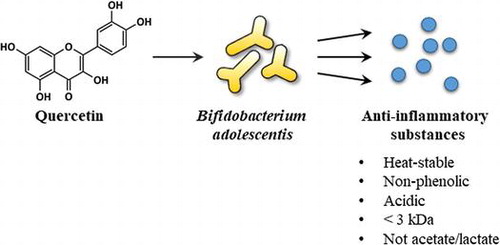
Abbreviations:
- BAD, Bifidobacterium adolescentis with DMSO
- BAQ, B. adolescentis with quercetin
- DMEM, Dulbecco’s modified Eagle’s medium
- DMSO, dimethyl sulfoxide
- ELISA, enzyme-linked immunosorbent assay
- FBS, fetal bovine serum
- IL-1β, interleukin 1β
- iNOS, inducible nitric oxide synthase
- LC-MS, liquid chromatography-mass spectrometry
- MW, molecular weight
- NO, nitric oxide
- PGE2, prostaglandin E2
- QUE quercetin
- TNF-α, tumor necrosis factor-α
Edible plants richly contain non-nutrient bioactive fibers and phytochemicals including polyphenols widely accepted as beneficial for maintaining health and preventing diseases. Dietary fibers are known to control the absorption of sugar and lipids as well as improve intestinal environments, such as bowel habits and microflora components. The intestinal microbiome is composed of around 500–1000 species, and its pathophysiological functions recently have attracted a great deal of interest. The genus Bifidobacterium is a major component of intestinal microflora, and some species are widely used in probiotic products similar to lactic acid bacteria [Citation1]. This genus of bacteria essentially digests fibers to produce acetic and lactic acid; thus, oligosaccharides known as prebiotics mainly increase the number of bifidobacteria [Citation1]. Acetic and lactic acids are important sources of other short chain fatty acids, propionic acid and butyric acid [Citation2,3]. The Japanese gut microbiome contains most abundantly the genus Bifidobacterium among 12 nations on 6 continents [Citation4]. This may be partly because traditional Japanese foods have a high content of dietary fiber. However, the gut microbiome in the Hadza of Tanzania, who are hunter-gatherers mainly consuming wild foods but not agricultural products, completely lack bifidobacterial species [Citation5]. In addition to producing organic acids, bifidobacteria are known to produce health promoting metabolites including vitamins [Citation6], conjugated linoleic acid [Citation7], bacteriocins [Citation8], and exopolysaccarides [Citation9], as well as largely unknown biomolecules with anti-inflammatory activity [Citation10Citation[11]Citation[12]−Citation13].
Dietary polyphenols are widely thought to exhibit physiological functions following absorption from the intestine and then distribution into specific tissues. However, a small portion (5–10%) of total polyphenol intake is estimated to be absorbed at the small intestine, while the remaining portion probably moves into the colon and is catabolized by the intestinal microbiota [Citation14]. Polyphenols are essentially subjected to reactions including hydrolysis, reduction, dehydroxylation, demethylation, and ring fission, and then catabolized to small phenolic compounds, such as hydroxycinnamates, γ-valerolactones, and phloroglucinol [Citation15,16]. Polymerized polyphenols may be initially depolymerized into monomers. Furthermore, some polyphenols including daidzein, ellagitannins, and lignans are converted to more active compounds such as equol, urolithins, and enterodiol/enterolactone, respectively. Small phenolic catabolites were shown to be absorbed from the colon and have antioxidant activity, anti-microbial, and anti-inflammatory activity in vitro as well as anti-carcinogenic activity in vivo, suggesting that these catabolites may exert biological activities, which are unique actions of polyphenols in the body [Citation17Citation[18]−Citation19].
Although polyphenols may regulate components of the microflora via suppressing growth of pathogenic bacteria with lesser effects on probiotic bacteria [Citation20], the biological effects on the gut microbiota have been not fully investigated. We have found that some polyphenols, such as quercetin and (−)-epigallocatechin-3-gallate enhance in vitro anti-inflammatory activity of B. adolescentis [Citation21,22]. The culture supernatant of B. adolescentis with quercetin markedly suppressed levels of nitric oxide (NO) production by lipopolysaccharide (LPS)-stimulated RAW264 cells. Quercetin spontaneously and equally degraded during the incubation period regardless of the presence of the bifidobacteria in our experimental conditions. Phenolic acids known as major catabolites of quercetin failed to decrease NO production. Additionally, anti-inflammatory activity of the culture supernatant increased in a bacterial number-dependent manner [Citation21]. Hence, we speculated that quercetin promotes the secretion of anti-inflammatory substances from B. adolescentis, which may secrete it spontaneously at various levels. Several studies have already reported the anti-inflammatory activity of the culture supernatant from bifidobacterial species [Citation10–13], while, to our knowledge, the enhancement of a biological function of the microbiota by polyphenols was first demonstrated. Furthermore, our previous investigation suggested that the anti-inflammatory substances are not acetic or lactic acids, indicating the presence of unidentified biomolecules [Citation22]. Therefore, the aim of this study was to investigate physicochemical and functional aspects of the anti-inflammatory substances secreted from B. adolescentis.
Materials and methods
Reagents
GAM broth was purchased from Nissui Pharmaceutical (Tokyo, Japan). Dulbecco’s modified Eagle’s medium (DMEM, high glucose), penicillin, and streptomycin were obtained from Sigma-Aldrich (St. Louis, MO, USA). Fetal bovine serum (FBS) was purchased from Thermo Fisher Scientific (Waltham, MA, USA). Quercetin, prostaglandin E2 (PGE2) Express EIA kits, and primary antibodies for inducible nitric oxide synthase (iNOS) and cyclooxygenase (COX)-2 were obtained from Cayman Chemical (Ann Arbor, MI, USA). Enzyme-linked immunosorbent assay (ELISA) kits for tumor necrosis factor-α (TNF-α) and interleukin 1β (IL-1β) were bought from Diaclone (Besancon, France) and R&D systems (Minneapolis, MN, USA), respectively. Anti-pan-actin antibody and horseradish peroxidase-conjugated anti-rabbit IgG antibody were purchased from Cell Signaling Technology (Beverly, MA, USA). Stearic acid was obtained from Tokyo Chemical Industry (Tokyo, Japan). All other reagents were purchased from WAKO Pure Chemicals (Osaka, Japan), unless otherwise specified.
Culture conditions. B. adolescentis
JCM1275T and murine macrophage RAW264 cells (RCB0535) were provided by the RIKEN BRC through the National Bio-Resource Project of the Ministry of Education, Culture, Sports, Science and Technology, Japan. B. adolescentis was cultured in GAM broth supplemented with 10 g/L of glucose at 37 °C for 22 h under static and anaerobic conditions using the AnaeroPack system (Mitsubishi Gas Chemical, Tokyo, Japan). RAW264 cells were maintained in DMEM supplemented with 10% FBS, 100 U/mL of penicillin, and 100 μg/mL streptomycin at 37 °C in a humidified atmosphere with 5% CO2.
Preparation of the culture supernatants
B. adolescentis was cultured with dimethyl sulfoxide (DMSO, final conc. 0.05%) or quercetin dissolved in DMSO (final 25 μM; final 0.05% DMSO) in 1 mL of serum and antibiotic-free DMEM at 37 °C for 3 h under anaerobic and static conditions. Quercetin-preincubated medium was prepared by the anaerobic incubation of quercetin-supplemented DMEM for 2 h just before addition of the bacteria. Wash out of quercetin was performed by washing B. adolescentis, which had been incubated with quercetin for 3 h, twice with PBS and then culturing the bacteria in fresh DMEM for a further 3 h. The bacteria in the culture media were removed by two rounds of centrifugation (5000 ×g for 5 min; 10,000 ×g for 5 min), and the obtained culture supernatants were used for the cell experiments. The heating of the culture supernatants was performed using a dry heat block kept at 100 °C for 30 min. Polyclar VT (polyvinylpolypyrrolidone, 10 mg/mL), which is used as a convenient precipitant of phenolic compounds to remove polyphenols from beverage products [Citation23], was added to the culture supernatant to remove phenolic compounds and incubated at 4 °C for 90 min, and then removed by centrifugation at 20,000 ×g for 5 min. The fractionation of the culture supernatants was performed using ultrafiltration devices with 3 kDa molecular weight cut-off membrane (Amicon ultra, Millipore, Bedford, MA, USA). Following the prewash of the membrane by centrifugation with 0.5 mL of ultrapure water at 14,000 ×g for 14 min, the culture supernatants (0.5 mL) were filtrated at 14,000 ×g for 7 min. Upper (>3 kDa) and lower (<3 kDa) fractions filled to 0.5 mL with serum and antibiotic-free DMEM were used for the cell experiments.
Ethyl acetate partition of the culture supernatants
The pH of the culture supernatants was adjusted by adding hydrogen chloride or sodium hydroxide (final conc. 0.1 M) followed by the ethyl acetate partition twice. The ethyl acetate layer was collected and dried in a centrifugal evaporator, and then reconstituted with ultrapure water as 50-fold concentrate, which was added to the cell culture media as described below.
Liquid chromatography–mass spectrometry (LC-MS) analysis
Ethyl acetate layer dried up by a centrifugal evaporator was dissolved in 80% acetonitrile with 0.1% formic acid, and then subjected to LC-MS analysis using an EASY-nLC 1000 coupled to an LTQ-Orbitrap Velos Pro (Thermo Fisher Scientific, Waltham, MA, USA). Mobile phase A was water with 0.1% (v/v) formic acid, and mobile phase B was acetonitrile with 0.1% (v/v) formic acid. The samples were separated using an Acclaim PepMap100 C18 column (3 μm, 0.075 × 150 mm; Thermo Fisher Scientific) at 200 nL/min flow rate with a linear gradient program of 80–95% B for 80 min. Mass spectra from m/z 100 to 500 were obtained in the ESI positive-ion mode.
Evaluation of in vitro anti-inflammatory activity of the culture supernatant
Following incubation of RAW264 cells (3.5 × 104 cells in 0.14 mL) in a 96-well microplate for 24 h, the cells were treated with samples (50-fold concentrate of the culture supernatant, organic acids, and stearic acid) for 20 min and then stimulated by 100 ng/mL LPS. After 24 h of incubation, the medium was used to measure the NO production using the Griess assay [Citation24] and the inflammatory mediators using ELISA kits following the manufacturer’s instructions. The concentration of NO was calculated from the standard curve prepared using a sodium nitrite solution. All of the tested compounds and conditioned media showed no cytotoxicity to RAW264 cells in any experiment.
Western blotting
Following LPS treatment of RAW264 cells in the culture supernatant for 24 h, the cells were lysed using RIPA buffer supplemented with Halt Protease and Phosphatase Inhibitor (Thermo Fisher Scientific, Waltham, MA, USA). Total protein (5 μg) was separated by sodium dodecyl sulfate-polyacrylamide gel electrophoresis using 4–20% Mini-PROTEAN TGX Precast gel (Bio-Rad, Hercules, CA, USA), and then transferred onto Immobilon-P polyvinylidene difluoride membranes (Merck Millipore, Darmstadt, German). Following the blocking of the membrane using washing buffer (0.1 M Tris-HCl, pH7.5, 0.15 M NaCl, 0.1% Tween20) containing 5% (w/v) bovine serum albumin for 30 min, the membrane was incubated in the solution of primary antibodies for iNOS, COX-2 or pan-actin (1:2,000) diluted in 5% bovine serum albumin for 1 h with gentle shaking at room temperature. The membrane was washed with washing buffer six times for 5 min, then incubated in the solution of secondary antibody (1:10,000) in 5% bovine serum albumin for 1 h with gentle shaking at room temperature. After washing, the membrane was reacted with ImmunoStar LD (WAKO Pure Chemicals), and its chemiluminescence was detected and quantified using Fusion SL4 imaging system (Vilber Lumat, Marne-la-Vallée, France).
Statistical analysis
Data are shown as the mean ± SD from three independent experiments. The statistical analyses (Tukey–Kramer test and Student’s t-test) were performed using GraphPad Prism7 for Windows (version 7.02, GraphPad Software, Inc., La Jolla, CA, USA). A p-value of less than 0.05 was defined as statistically significant.
Results
The culture supernatant of B. adolescentis with quercetin had suppressive effects on the production of inflammatory mediators in LPS-stimulated RAW264 cells
We have shown that the culture supernatant of B. adolescentis with polyphenols more potently inhibits the NO production in LPS-stimulated RAW264 cells. As shown in Figure (A), the culture supernatant from quercetin-treated B. adolescentis markedly inhibited NO production by 60%, even though single incubation with quercetin or B. adolescentis had no effect. However, its suppressive effects on other inflammatory mediators were unclear. Therefore, we investigated changes in the production of TNF-α, IL-1β, and PGE2 in the activated macrophages that were incubated in the culture supernatant. LPS treatment in the culture supernatant of B. adolescentis with DMSO remarkably induced the production of these inflammatory mediators (Figure (B)–(D)). The culture supernatant of B. adolescentis with quercetin reduced the levels of PGE2, TNF-α, and IL-1β by 40, 27, and 47%, respectively, with a statistically significant difference. Additionally, the protein levels of iNOS and COX-2 were markedly decreased by 61 and 37%, respectively (Figure (E)).
Figure 1. The culture supernatant of B. adolescentis with quercetin suppressed the production of the inflammatory mediators from LPS-stimulated macrophages. B. adolescentis was incubated with DMSO (final concentration 0.05%, 0 μM quercetin) or quercetin (25 μM) in serum-free DMEM at 37 °C for 3 h under static and anaerobic conditions. The culture supernatants were prepared by the centrifugation twice, and then added to RAW264 cells. Following 24 h of LPS treatment, (A) NO (left, production level; right, inhibition ratio), (B) PGE2, (C) TNF-α, and (D) IL-1β were measured with the Griess assay or ELISA kits. Additionally, protein levels of iNOS and COX-2 were analyzed using western blotting (E). Intensities of these bands were quantified and corrected with the band intensity of pan-actin as an internal standard. Data are shown as means ± SD (n = 3) and were analyzed for statistically significant differences by multiple comparison testing (Tukey–Kramer test and Student’s t-test). Indicated images are representative data. Different letters indicate significant differences (p < 0.05). BA, B. adolescentis; IL, interleukin; LPS, lipopolysaccharide; PG, prostaglandin; QUE, quercetin; TNF, tumor necrosis factor.
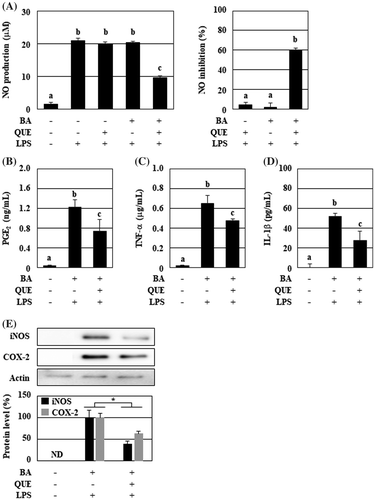
Quercetin itself, but not its decomposition products, enhanced the anti-inflammatory activity of B. adolescentis
Quercetin enhances the secretion of anti-inflammatory substances from B. adolescentis, and rapidly and spontaneously decomposes less than 10% of the dosage in the media for 1-h incubation, suggesting the possibility of decomposition products of quercetin as a bifidobacteria-stimulating factor. We next investigated the effect of quercetin-preincubated medium on B. adolescentis. Simultaneous addition of B. adolescentis and quercetin into the medium showed marked increase of anti-inflammatory activity (Figure , left column), whereas the addition of the bifidobacteria into the medium that was preincubated with quercetin for 2 h failed to suppress the NO production (Figure , center column). The bifidobacteria washed out of quercetin after a 3-h incubation retained the production of anti-inflammatory substances (Figure , right column).
Figure 2. Quercetin, but not decomposed products, persistently enhanced the anti-inflammatory activity of B. adolescentis. The samples used for the experiments were as follows: (left column) B. adolescentis and quercetin were simultaneously added to the medium, followed by incubation for 3 h; (center column) B. adolescentis was added to the quercetin-preincubated medium, followed by incubation for 3 h; (right column) B. adolescentis was incubated with quercetin for 3 h, washed with PBS, and then incubated in fresh medium for a further 3 h. The final concentration of quercetin was 25 μM. DMSO (0.05%) was used as a control. Supernatants of the incubated media were collected and evaluated for suppressive effects on NO production in LPS-stimulated macrophages. Data are shown as means ± SD (n = 3) and were analyzed for statistically significant differences by multiple comparison testing (Tukey–Kramer test). Different letters indicate significant differences (p < 0.05). BA, B. adolescentis; LPS, lipopolysaccharide; QUE, quercetin.
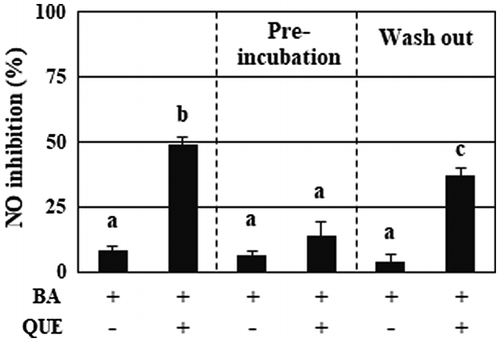
Acetate and lactate may not be principal components of quercetin-enhanced anti-inflammatory activity of B. adolescentis
The bifidobacteria metabolize glucoses into adenosine triphosphate as well as acetate and lactate. Acetate are reported to suppress the production of inflammatory mediators, such as NO, TNF-α, and IL-1β, in LPS-treated RAW264 cells [Citation25]. Hence, we confirmed the NO suppressive activity of acetate and lactate in our laboratory. Sodium acetate and sodium lactate dissolved in MilliQ water and filter-sterilized were mixed with serum and antibiotic-free DMEM at final concentrations of 1.25–20 mM and then added to the cells. Acetate, but not lactate, inhibited NO production in a dose-dependent manner (Figure ). The suppressive activity of the combination was similar to that of acetate. Acetate and the combination at 20 mM suppressed the NO production by 31% and 36%, respectively, whereas these acids at 5 mM, which are the same level of acetate and lactate in the culture supernatant of B. adolescentis with quercetin [Citation22], were 9–12% suppression.
Figure 3. Acetate and lactate had a weak suppression of NO production. RAW264 cells were pretreated with acetate, lactate (final concentration 1.25–20 mM), and a combination for 20 min, and then stimulated by LPS for 24 h. After incubation, the suppressive effects of NO production of the organic acids were evaluated. Data are shown as means ± SD (n = 3). NO, nitric oxide.
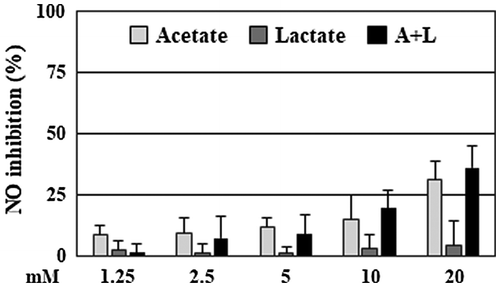
The anti-inflammatory substance of B. adolescentis was a non-phenolic and heat stable compound with a low molecular weight
Although some studies reported in vitro anti-inflammatory activity of bifidobacteria, its material information has been limited including organic acids, DNA, and a protein [Citation10,12]. To estimate active substances secreted from quercetin-treated B. adolescentis, we confirmed changes of anti-inflammatory activity of the culture supernatant that were physicochemically treated. Removal of phenolic compounds by Polyclar VT showed little effect on the NO suppressive activity of the culture supernatant of B. adolescentis with quercetin and only 9% reduction with a statistically significant difference (Figure (A)). The heat treatment of the culture supernatant had no effect on NO suppression (Figure (B)). Additionally, the fraction less than 3 kDa of the culture supernatant prepared by the ultrafiltration retained NO suppressive activity (Figure (C)).
Figure 4. The anti-inflammatory substance of B. adolescentis was a non-phenolic and heat stable compound with low molecular weight. The culture supernatants of B. adolescentis with DMSO or quercetin were treated with (A) Polyclar VT to remove phenolic compounds, (B) heated at 100 °C for 30 min, or (C) fractionated by ultrafiltration as described in the Materials and Methods. Then, the suppressive effects of NO production of the culture supernatant were evaluated. (D) The pH of the culture supernatant was modified by adding hydrogen chloride or sodium hydroxide, and then partitioned by ethyl acetate. The original supernatant (Pre) and the reconstitutes (pH = 2–12: 50-fold concentrates) of the organic layer prepared as described in the Materials and Methods. The reconstitutes were diluted to 1× concentration with serum and antibiotic-free DMEM and then added to RAW264 cells. After 24 h of LPS treatment, the inhibition of the NO production was evaluated. Data are shown as means ± SD (n = 3). BAD, B. adolescentis with DMSO; BAQ, B. adolescentis with quercetin; MW, molecular weight; NO, nitric oxide.
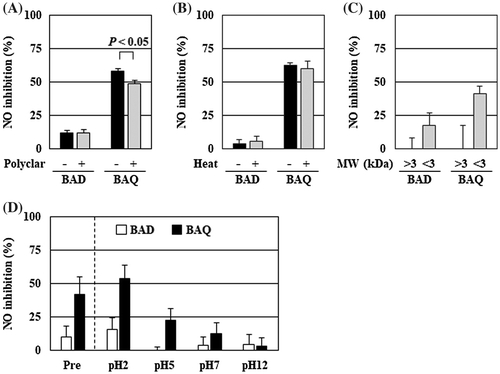
Next, to isolate active substances, liquid–liquid partition of the culture supernatant with ethyl acetate was performed. Following the partition using the culture supernatant just after preparation, the organic layer was collected, reconstituted, and estimated the in vitro anti-inflammatory activity; it failed to inhibit NO production (data not shown). Hence, the partition was performed using the culture supernatant that modified the pH with hydrogen chloride or sodium hydroxide. Interestingly, the NO suppressive activity of the organic layer changed in a pH-dependent manner (Figure (D)). The organic layer with low pH showed increased activity; pH = 2 was more potent, while pH = 12 was inactive.
Stearic acid was tentatively identified as a candidate of anti-inflammatory substances
The organic layer with low pH was analyzed using LC-MS technique for the tentative identification of bioactive substances. LC-MS data showed some differences in the peaks between DMSO- and quercetin-treated bifidobacteria (Figure (A)). Among them, the broad peak around 56 min contained a major compound with [M + H]+ m/z 285.2785 (Δ −0.3 mmu), which suggested stearic acid (C18H36O2) as a tentative biomolecule candidate. Additionally, stearic acid, which was dissolved in DMSO and mixed with serum and antibiotic-free DMEM at final concentrations of 1.5–50 μM, suppressed NO production in a dose-dependent manner without cytotoxicity (Figure (B)).
Figure 5. Tentative identification of anti-inflammatory substances showed stearic acid as the biomolecule candidate. (A) The organic layers prepared by the culture supernatant with low pH were analyzed using LC-MS system described in Materials and methods. (B) RAW264 cells were pretreated with stearic acid (final concentration 1.5–50 μM) for 20 min, and then stimulated by LPS for 24 h. After incubation, the suppressive effects of NO production of stearic acid were evaluated. Data are shown as means ± SD (n = 3). BAD, B. adolescentis with DMSO; BAQ, B. adolescentis with quercetin; NO, nitric oxide.
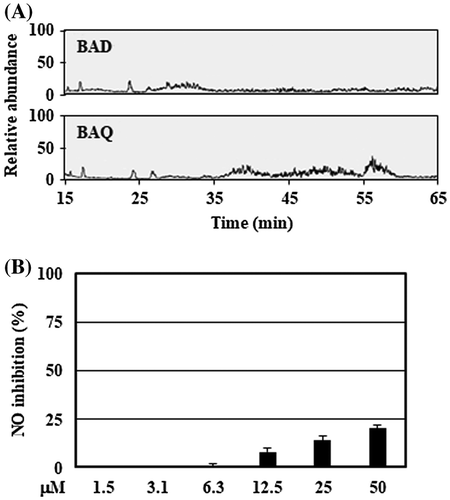
Discussion
Biotransformation of polyphenols by intestinal bacteria has become important parts of the study of bioavailability, and the biological activities of its metabolites have received attention. We have reported that some polyphenols including quercetin enhanced in vitro anti-inflammatory activity of B. adolescentis [Citation21,22]. According to our follow-up experiments to investigate the change in anti-inflammatory activity of the culture supernatant by modifying quercetin concentrations, culture duration, and bacterial number, we assumed that anti-inflammatory substances in the culture supernatant were derived from B. adolescentis primed by quercetin, but not quercetin metabolites by B. adolescentis. Additionally, (-)-epigallocatechin gallate, phloretin, and (+)-taxifolin stimulated the production of acetate and lactate by B. adolescentis [Citation22]. To our knowledge, polyphenol-induced secretion of functional molecules by bifidobacteria is a newly emerged functional interaction between dietary factors and intestinal microbiota. Therefore, in the present study, we investigated the functional properties of the anti-inflammatory culture supernatant of B. adolescentis with quercetin to obtain more information on the active substances and attempted to identify them.
Because we estimated the in vitro anti-inflammatory activity of the culture supernatant as the suppressive activity on the NO production, we examined the effects of the culture supernatant on other inflammatory mediators including PGE2, TNF-α, and IL-1β. These mediators were reduced by the treatment of the culture supernatant by 40, 27, and 47%, respectively, with a significant difference, while the suppression ratios were relatively weaker than that of the NO production (60%) (Figure ). Because the cell membrane-bound TNF-α is stocked on dormant macrophages and rapidly secreted as soluble TNF-α via shedding by proteases as an acute inflammatory response [Citation26], the suppressive effects of the culture supernatant on TNF-α production was especially weak among the tested mediators. The culture supernatant reduced the levels of iNOS and COX-2 protein, suggesting that anti-inflammatory substances in the culture supernatant may inhibit LPS-induced inflammatory signaling in RAW264 cells.
Pre-incubated quercetin failed to enhance the anti-inflammatory activity of B. adolescentis. Additionally, the enhanced ability of the bacteria was kept after wash out of quercetin (Figure ). These findings indicate that quercetin itself, but not its decomposed compounds, is probably essential for increasing the ability of B. adolescentis, and that the effect of quercetin on the bacteria may persist for a few hours. Quercetin must penetrate a cell wall composed of a thick peptidoglycan layer with teichoic acids to reach the plasma membrane and then cause anti-inflammatory signaling in Gram-positive bifidobacteria. Because B. adolescentis cultured with quercetin has a light yellow color, quercetin may penetrate the peptidoglycan layer. Electrostatic characteristics of the cell wall may affect the permeation of quercetin into the bacteria [Citation27]. Whether quercetin elicits anti-inflammatory signaling in the bifidobacteria and, if so, how it interacts with bacterial proteins in the plasma membrane, as well as what proteins are involved in the intracellular signaling, is of great interest.
Acetate and lactate are major products in the metabolism of carbohydrates in bifidobacteria. These acids are used by intestinal bacteria to produce propionate and butyrate [Citation3]. Acetate, propionate, and butyrate, namely short chain fatty acids, have a variety of physiological effects regulating functions of several tissue including colon, brain, and liver [Citation28]. Other groups reported that short chain fatty acids at 1.2 mM suppressed NO production in LPS-treated RAW264 cells by around 50% [Citation25]. However, we confirmed weak suppression by acetate, lactate, and a combination at 5 mM (12, 1, and 9%, respectively) compared with the culture supernatant (Figure ). Our previous study showed that the culture supernatant of B. adolescentis with quercetin contained 5 mM acetate and 4 mM lactate, which were similar levels in the supernatant of the bifidobacteria plus DMSO. That is, quercetin had no effect on the production of these acids [Citation22]. Therefore, we considered that acetate and lactate are probably not major anti-inflammatory substances secreted from B. adolescentis.
The verification in this study demonstrated that anti-inflammatory substances secreted from quercetin-stimulated B. adolescentis are heat-stable and non-phenolic compounds with a molecular weight less than 3 kDa (Figure (A)–(C)). Furthermore, the active substances moved to ethyl acetate from the acidic, but not neutral and alkaline, culture supernatant, indicating the anti-inflammatory substances have an acidic feature. Other groups identified a 50 kDa protein secreted from Bifidobacterium animalis subsp. lactis BB12 as an inhibitor of interleukin-8 production in TNF-α-treated Caco-2 cells. More recently, Faecalibacterium prausnitzii was identified as an anti-inflammatory commensal bacterium [Citation29], and found to secrete a 15 kDa protein with a microbial anti-inflammatory molecule [Citation30]. The anti-inflammatory effects of this protein and derived peptides were demonstrated by in vitro and in vivo models [Citation30]. The isolated peptide (VTLVGNTFLQSTINRTIGVL, [M + H]+ m/z 2146.94) was composed of mainly hydrophobic and neutral amino acids and without acidic amino acids. Its related protein and peptides were not found in BLAST search results of Bifidobacterial proteins, indicating that this peptide is most likely not involved in our findings. On the other hand, an experiment using a proteinase was attempted but was not successful due to a cytotoxic effect of the proteinase treatment (data not shown). To rule out the possibility that a proteinaceous substance is involved in the activity, another digestion experiment will be necessary.
Tentative identification by LC-MS analysis showed the possibility of stearic acid as a candidate of the anti-inflammatory substance from B. adolescentis (Figure (A)). Additionally, the base peak from 35 to 55 min suggested the presence of unique compounds in the culture supernatant of quercetin-treated bacteria, but it was difficult to estimate the structure of compounds because of the trace amount of the extract. Interestingly, stearic acid had a weak, but not negligible, suppressive activity against NO production and no cytotoxicity (Figure (B)). Because DMEM does not contain stearic acid, this fatty acid is probably derived from the bacterial components. Saturated fatty acids including stearic acid are known to induce inflammatory responses via activation of Toll-like receptor [Citation4,31], whereas our finding indicates an anti-inflammatory action of stearic acid. Physicochemical properties of the culture supernatant were closely matched to the chemical characteristics of stearic acid. However, considering its weak activity, the culture supernatant most likely contained other types of major active biomolecules. Stearic acid is possibly a component of one or more of these active biomolecules. Establishment of an efficient method for large-scale preparation of the extract from the culture supernatant may provide more information to identify the anti-inflammatory substances of B. adolescentis.
In conclusion, quercetin stimulated the secretion from B. adolescentis of heat-stable, non-phenolic, and acidic anti-inflammatory substances with a molecular weight less than 3 kDa. Stearic acid was tentatively identified as a candidate of anti-inflammatory substance from B. adolescentis, while major active biomolecules remain to be explored. Additionally, action mechanisms of quercetin and the characteristics of the active substances are the most intriguing questions, which may lead us to new insights into functional interactions of host and bifidobacteria as the strategies for maintaining health. We will address these questions in the near future.
Author contributions
Kyuichi Kawabata conceived and designed the experiments, analyzed the results, and wrote the manuscript with assistance from Yoshimitsu Hamano, Shu Taira, Shigeru Murakami, and Hajime Ohigashi. Nobuyuki Baba and Taiken Sakano performed the experiments. Akira Tamura, Seigo Baba, Midori Natsume, and Takeshi Ishii contributed reagents, materials, and analytical tools. All authors reviewed the results and approved the final version of the manuscript.
Disclosure statement
No potential conflict of interest was reported by the authors.
Funding
This work was supported by grants to K. K. from the Ministry of Education, Culture, Sports, Science and Technology of Japan under a Grant-in-Aid for Young Scientists (B, numbers 22780120 and 26850085), and the Food Science Institute Foundation (Ryoushoku-kenkyukai), Japan.
Acknowledgment
We thank Joel Anderson PhD, from Edanz Group (www.edanzediting.com/ac) for editing a draft of this manuscript.
References
- Rastall RA, Gibson GR, Gill HS, et al. Modulation of the microbial ecology of the human colon by probiotics, prebiotics and synbiotics to enhance human health: An overview of enabling science and potential applications. FEMS Microbiol Ecol. 2005;52(2):145–152.10.1016/j.femsec.2005.01.003
- Reichardt N, Duncan SH, Young P, et al. Phylogenetic distribution of three pathways for propionate production within the human gut microbiota. ISME J. 2014;8(6):1323–1335.10.1038/ismej.2014.14
- Belenguer A, Duncan SH, Calder AG, et al. Two routes of metabolic cross-feeding between Bifidobacterium adolescentis and butyrate-producing anaerobes from the human gut. Appl Environ Microbiol. 2006;72(5):3593–3599.10.1128/AEM.72.5.3593-3599.2006
- Nishijima S, Suda W, Oshima K, et al. The gut microbiome of healthy Japanese and its microbial and functional uniqueness. DNA Res. 2016;23(2):125–133.10.1093/dnares/dsw002
- Schnorr SL, Candela M, Rampelli S, et al. Gut microbiome of the Hadza hunter-gatherers. Nat Commun. 2014;5:3654.
- LeBlanc JG, Milani C, de Giori GS, et al. Bacteria as vitamin suppliers to their host: a gut microbiota perspective. Curr Opin Biotechnol. 2013;24(2):160–168.10.1016/j.copbio.2012.08.005
- Coakley M, Ross RP, Nordgren M, et al. Conjugated linoleic acid biosynthesis by human-derived Bifidobacterium species. J Appl Microbiol. 2003;94(1):138–145.10.1046/j.1365-2672.2003.01814.x
- Martinez FAC, Balciunas EM, Converti A, et al. Bacteriocin production by Bifidobacterium spp. A review. Biotechnol Adv. 2013;31(4):482–488.10.1016/j.biotechadv.2013.01.010
- van Geel-Schutten GH, Flesch F, ten Brink B, et al. Screening and characterization of Lactobacillus strains producing large amounts of exopolysaccharides. Appl Microbiol Biotechnol. 1998;50(6):697–703.10.1007/s002530051353
- Imaoka A, Shima T, Kato K, et al. Anti-inflammatory activity of probiotic Bifidobacterium: Enhancement of IL-10 production in peripheral blood mononuclear cells from ulcerative colitis patients and inhibition of IL-8 secretion in HT-29 cells. World J Gastroenterol. 2008;14(16):2511–2516.10.3748/wjg.14.2511
- Okada Y, Tsuzuki Y, Hokari R, et al. Anti-inflammatory effects of the genus Bifidobacterium on macrophages by modification of phospho-IκB and SOCS gene expression. Int J Exp Pathol. 2009;90(2):131–140.10.1111/iep.2009.90.issue-2
- Wang Z, Wang J, Cheng Y, et al. Secreted factors from Bifidobacterium animalis subsp. lactis inhibit NF-κB-mediated interleukin-8 gene expression in Caco-2 cells. Appl Environ Microbiol. 2011;77(22):8171–8174.10.1128/AEM.06145-11
- Khokhlova E V, Smeianov V V, Efimov B a, et al., Anti-inflammatory properties of intestinal Bifidobacterium strains isolated from healthy infants. Microbiol Immunol. 2012;56(1): 27–39.10.1111/mim.2012.56.issue-1
- Clifford MN. Diet-derived phenols in plasma and tissues and their implications for health. Planta Med. 2004;70(12):1103–1114.10.1055/s-2004-835835
- Selma MV, Espín JC, Tomás-Barberán Fa. Interaction between phenolics and gut microbiota: role in human health. J Agric Food Chem. 2009;57(15):6485–6501.10.1021/jf902107d
- Monagas M, Urpi-Sarda M, Sánchez-Patán F, et al. Insights into the metabolism and microbial biotransformation of dietary flavan-3-ols and the bioactivity of their metabolites. Food Funct. 2010;1(3):233–253.10.1039/c0fo00132e
- Williamson G, Clifford MN. Colonic metabolites of berry polyphenols: the missing link to biological activity? Br J Nutr. 2010;104(S3):S48–S66.10.1017/S0007114510003946
- Tuohy KM, Conterno L, Gasperotti M, et al. Up-regulating the human intestinal microbiome using whole plant foods, polyphenols, and/or fiber. J Agric Food Chem. 2012;60(36):8776–8782.10.1021/jf2053959
- Gasperotti M, Passamonti S, Tramer F, et al. Fate of microbial metabolites of dietary polyphenols in rats: is the brain their target destination? ACS Chem Neurosci. 2015;6(8):1341–1352.10.1021/acschemneuro.5b00051
- Etxeberria U, Fernández-Quintela A, Milagro FI, et al. Impact of polyphenols and polyphenol-rich dietary sources on gut microbiota composition. J Agric Food Chem. 2013;61(40):9517–9533.10.1021/jf402506c
- Kawabata K, Sugiyama Y, Sakano T, et al. Flavonols enhanced production of anti-inflammatory substance(s) by Bifidobacterium adolescentis : Prebiotic actions of galangin, quercetin, and fisetin. BioFactors. 2013;39(4):422–429.10.1002/biof.1081
- Kawabata K, Kato Y, Sakano T, et al. Effects of phytochemicals on in vitro anti-inflammatory activity of Bifidobacterium adolescentis. Biosci Biotechnol Biochem. 2015;79(5):799–807.10.1080/09168451.2015.1006566
- McMurrough I, Madigan D, Smyth MR. Adsorption by polyvinylpolypyrrolidone of catechins and proanthocyanidins from beer. J Agric Food Chem. 1995;43(10):2687–2691.10.1021/jf00058a025
- Green LC, Wagner DA, Glogowski J, et al. Analysis of nitrate, nitrite, and [15 N]nitrate in biological fluids. Anal Biochem. 1982;126(1):131–138.10.1016/0003-2697(82)90118-X
- Liu T, Li J, Liu Y, et al. Short-Chain fatty acids suppress lipopolysaccharide-Induced production of nitric oxide and proinflammatory cytokines through inhibition of NF-?B Pathway in RAW264.7 cells. Inflammation. 2012;35(5):1676–1684.10.1007/s10753-012-9484-z
- Gearing AJ, Beckett P, Christodoulou M, et al. Processing of tumour necrosis factor-α precursor by metalloproteinases. Nature. 1994;370(6490):555–557.10.1038/370555a0
- Malanovic N, Lohner K. Gram-positive bacterial cell envelopes: The impact on the activity of antimicrobial peptides. Biochim Biophys Acta - Biomembr. 2016;1858(5):936–946.10.1016/j.bbamem.2015.11.004
- Koh A, De Vadder F, Kovatcheva-Datchary P, et al. From dietary fiber to host physiology: short-chain fatty acids as key bacterial metabolites. Cell. 2016;165(6):1332–1345.10.1016/j.cell.2016.05.041
- Sokol H, Pigneur B, Watterlot L, et al. Faecalibacterium prausnitzii is an anti-inflammatory commensal bacterium identified by gut microbiota analysis of Crohn disease patients. Proc Natl Acad Sci USA. 2008;105(43):16731–16736.10.1073/pnas.0804812105
- Quévrain E, Maubert MA, Michon C, et al. Identification of an anti-inflammatory protein from Faecalibacterium prausnitzii, a commensal bacterium deficient in Crohn’s disease. Gut. 2016;65(3):415–425.10.1136/gutjnl-2014-307649
- Milanski M, Degasperi G, Coope A, et al. Saturated fatty acids produce an inflammatory response predominantly through the activation of TLR4 Signaling in hypothalamus: implications for the pathogenesis of obesity. J Neurosci. 2009;29(2):359–370.10.1523/JNEUROSCI.2760-08.2009