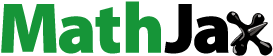
ABSTRACT
The aim of this work was to evaluate the potential anti-inflammatory effect of protein hydrolysate and peptide fractions from Salvia hispanica L. seeds. Protein isolate was obtained using defatted flour of mucilage-free seeds. Hydrolysis process was conducted by enzymatic digestion. The hydrolysate was fractionated using ultrafiltration membranes to obtain the peptide fractions (<1, 1-3, 3-5, 5-10, and >10 kDa). Protein derivatives were evaluated by in vitro activation of murine peritoneal macrophages. The anti-inflammatory activity was determined as NO production, H2O2 release and pro- and anti-inflammatory cytokines (TNF-α, IL-1β, IL-6, and IL-10) production. All the peptides exerted an anti-inflammatory activity, but peptide fraction between 1 - 3 kDa showed the highest anti-inflammatory effect. This fraction was evaluated on in vivo murine models of TPA-induced ear edema and DNFB-induced delayed-type hypersensitivity, exhibiting inhibitory effects. Hence, the results demonstrated that protein derivatives from S. hispanica L. seeds have in vitro and in vivo anti-inflammatory effects.
1. Introduction
Inflammation has been described as a response to infection or injury. The inflammatory process is universally associated with diabetes, cancer and cardiovascular or neurodegenerative diseases (Okin & Medzhitov, Citation2012). The first cellular signs of inflammation include infiltration of monocytes, dendritic cells, and neutrophils in the site of injury (Nathan & Ding, Citation2010). The inflammatory response begins with the neutrophils, which destroy and remove foreign particles and damaged tissue. Later, macrophages appear in the site of inflammation and continue the process of phagocytosis (Velnar, Bailey, & Smrkolj, Citation2009). Among inflammation-related processes, macrophages play an important role in inflammatory diseases through secretion of reactive oxygen species (ROS), for example, hydrogen peroxide (H2O2) (Mittal, Siddiqui, Tran, Reddy, & Malik, Citation2014). In addition, these cells produce other inflammation mediators including prostaglandins and reactive nitrogen species (RNS), such as the nitric oxide (NO) (Lugrin, Rosenblatt-Velin, Parapanov, & Liaudet, Citation2014). When macrophages are stimulated, these activated cells release cytokines involved in the regulation of inflammation, such as interleukin 1β (IL-1β), interleukin 6 (IL-6), interleukin 10 (IL-10) and tumor necrosis factor α (TNF-α), which mediate intercellular communication and orchestrate a variety of processes ranging from the cellular proliferation to metabolism, chemotaxis and inhibition of other cytokines (Arango Duque & Descoteaux, Citation2014; Lawrence & Fong, Citation2010; Zhang & An, Citation2007).
It has been found that there are many different types of drugs, with relevant clinical applications, to treat the inflammatory disorders. However, these drugs have been reported to be associated with undesirable side effects in some patients and a high cost of treatment. Therefore, natural products may be a good source for the identification of bioactive compounds and their possible development in drugs for treating inflammation (Gautam & Jachak, Citation2009). Functional foods are a kind of natural products that have a beneficial effect on one or more functions of the body. Also, functional foods improve the state of health and/or reduce the risk of disease (Doyon & Labrecque, Citation2008). It has been shown that a variety of bioactive compounds of functional foods are capable to modulate inflammatory responses, interacting with a wide array of inflammatory mediators (Kim, Young, Bobe, Colburn, & Milner, Citation2009). Among these compounds, proteins and bioactive peptides derived from animal and plant foods are the most studied components. By definition, bioactive peptides are food protein-derived peptides that possess beneficial pharmacological properties. Currently, there is a growing trend in the use of food protein-derived peptides as intervention agents against chronic diseases or the maintenance of general well-being (Udenigwe & Aluko, Citation2012).
Salvia hispanica L., whose common name is chia, is a functional food and potential source of bioactive peptides (Segura-Campos, Salazar-Vega, Chel-Guerrero, & Betancur-Ancona, Citation2013). S. hispanica L. is an annual herbaceous plant that belongs to the Lamiaceae family and is native from Mexico (Ixtaina, Nolasco, & Tomás, Citation2008). Various bioactive compounds have been identified in the seeds, including phenolic compounds and essential fatty acids. These molecules are responsible for antidiabetic, antioxidant and anti-inflammatory activities (Rangaraju & Kumar, Citation2013). Actually, the anti-inflammatory effect of proteins and peptides isolated from the seeds of this plant has not been reported. Because of this, the aim of the present study was to examine the anti-inflammatory activity of protein hydrolysate and peptide fractions isolated from S. hispanica L. seeds.
2. Materials and methods
2.1. Materials
Sodium hydroxide (NaOH), hydrochloric acid (HCl), sulfuric acid (H2SO4), Pepsin®, Pancreatin®, hexane, L-serine, O-phthaldialdehyde (OPA), thiazolyl blue tetrazolium bromide (MTT), dimethyl sulfoxide (DMSO), bovine serum albumin (BSA), trypan-blue dye, lipopolysaccharides (LPS) from Escherichia coli (0111:B4), Griess reagent, sodium nitrite (NaNO2), H2O2, dextrose, phenol red, type I horseradish peroxidase (HRP), acetone, indomethacin, 12-O-tetradecanoylphorbol 13-acetate (TPA) and 1-fluoro-2,4-dinitrobenzene (DNFB) were purchased from Sigma-Aldrich® (MO, USA). Dulbecco’s modified Eagle’s medium (DMEM), fetal bovine serum (FBS) and penicillin–streptomycin were purchased from Gibco-BRL® (NY, USA). Murine IL-1β enzyme-linked immunosorbent assay (ELISA) development kit (900-K47), murine IL-6 ELISA development kit (900-K50), murine IL-10 ELISA development kit (900-K53) and murine TNF-α ELISA development kit (900-K54) were obtained from Peprotech® (London, UK).
2.2. Plant material
S. hispanica L. seeds were obtained in December 2016 in Mérida, Yucatán, México. The seeds were identified by a specialist (Biol. José Luis Tapia Muñoz, CICY) and a sample were reserved at the herbarium of the Centro de Investigación Científica de Yucatán (CICY) for future reference (voucher number 69494).
2.3. Production of mucilage-free defatted flour
The production of mucilage-free defatted flour from S. hispanica L. was done according to the method described by Orona-Tamayo, Valverde, Nieto-Rendón, and Paredes-López (Citation2015). Flour was produced from 3 kg of S. hispanica L. seeds by first removing all impurities and damaged seeds. Later, seeds were mixed in distilled water in a 1:40 (w/v) ratio for 90 min at room temperature to allow mucilage extraction. Mucilage was separated from the mixture by filtering in a mesh. The mucilage-free seeds were dried in a stove during 24 h at 50°C and ground in a Thomas-Wiley® mill (model A) with a 0.5 mm screen, obtaining the flour. Oil extraction from the mucilage-free flour was done with hexane in a Soxhlet system for 4 h at 70°C and the defatted flour was dried in the stove for 24 h at 50°C. Finally, defatted flour was sifted for 20 min using a Ro-Tap® agitation system (model E) with a 140 µm mesh, obtaining mucilage-free defatted flour with a high concentration of protein.
2.4. Production of protein isolate
The production of protein isolate from S. hispanica L. was carried out following the protocol reported by Segura-Campos, Salazar-Vega, et al. (Citation2013). An alkaline extraction and acid precipitation of proteins method was employed, where 5 g of mucilage-free defatted flour was dispersed in 1 L of distilled water. The solution was adjusted to pH 12 with 2 N NaOH and mixed for 30 min at room temperature. The obtained solution was centrifuged 1 h at 2500 rpm and 10°C and the supernatant was collected. The supernatant was adjusted to pH 4 with 2 N HCl, mixed for 30 min at room temperature and centrifuged 1 h at 2500 rpm and 10°C. The precipitated was recovered and freeze-dried at 522 × 10−3 bar and −47°C.
2.5. Moisture and protein content
The moisture (method 925.09) and protein (method 954.01) contents in protein isolate from S. hispanica L. were determined following the standard procedures of the Association of Official Analytical Chemists (Cunniff, Citation1997). Moisture content was measured based on samples weight loss after stove-drying during 2 h at 130°C. Protein content on samples was quantified as nitrogen (N2) content after digestion with H2SO4 and catalysts in SpeedDigester® K-436 system, distillation in KjelFlex® K-360 system and titration with 0.1 N HCl.
2.6. Production of protein hydrolysate
The production of protein hydrolysate from S. hispanica L. was done according to the previously described method by Herrera Chalé, Ruiz Ruiz, Acevedo Fernández, Betancur Ancona, and Segura Campos (Citation2014). Hydrolysis of the protein isolate in distilled water at 5% (w/v) was carried out by sequential treatment with Pepsin® and Pancreatin®. Protein suspension was adjusted to pH 2 using 6 N HCl and hydrolyzed by treatment with Pepsin® for 45 min, followed by treatment with Pancreatin® for another 45 min after adjusting to pH 7.5 using 6 N NaOH. The temperature was kept at 37°C throughout the whole process and the hydrolysis was done in a reaction vessel equipped with a stirrer, thermometer and pH electrode. The reaction was stopped by heating to 80°C during 20 min, followed by centrifuging for 20 min at 2,500 rpm and 4°C to remove the insoluble portion.
2.7. Degree of hydrolysis
The degree of hydrolysis (DH) was measured according to Joshi, Sudhakar, and Nazeer (Citation2016). Protein hydrolysate was solubilized at 10 mg/mL in deionized water and a 1:10 dilution was done. Immediately, a 1:3 dilution was prepared from the previous dilution and aliquots of 200 µL were mixed with 1.5 mL of OPA reagent. The mixtures were shaking during 5 s and incubated for 2 min. The absorbances were measured at 340 nm in a Thermo Scientific® UV-Vis spectrophotometer and the number of amino groups was determined by comparison with an L-serine standard curve (0–100 µg/mL). The DH was calculated using the following equation:where: H is the number of hydrolyzed bonds and HTot is the total number of peptide bonds per protein equivalent. The HTot factor was dependent on raw material amino acid composition.
2.8. Isolation of peptide fractions
The protein hydrolysate was submitted to ultrafiltration process using the protocol of Laviada-Castillo et al. (Citation2019). Protein hydrolysate was passed through ultrafiltration membranes with molecular weight cut-off (MWCO) of 1, 3, 5 and 10 kDa using a stirred ultrafiltration system. Ultrafiltration was performed sequentially: first through the 10 kDa MWCO membrane and no-retentate material passed through 5 kDa. No-retentate material from 5 kDa was passed through the 3 kDa whose no-retentate material was passed through the 1 kDa. The retentate material from each MWCO membrane was collected as >10, 5–10, 3–5, 1–3 and <1 kDa peptide fractions, respectively.
2.9. Animals
Balb/c male mice (6–8 week of age, 20 ± 5 g of weight) were obtained from Centro de Investigaciones Regionales (CIR) “Dr. Hideyo Noguchi” of Universidad Autónoma de Yucatán. This study was done in accordance with the principles of the Statement on Animal Use in Biomedical Research from the World Medical Association and the Mexican Official Norm for Animal Care and Handing (NOM-062-ZOO-1999). Animals were maintained in standard polypropylene cages, with access to special food and purified water ad libitum, a free environment of pathogen and stress, controlled room with 12 h light/dark cycle and temperature of 22 ± 2°C.
2.10. In vitro assays
2.10.1. Isolation and treatment of peritoneal murine macrophages
The isolation and treatment of peritoneal murine macrophages were carried out according to the method described by Arana-Argáez et al. (Citation2017). Macrophages were isolated from the Balb/c mice by lavages of the peritoneal cavity using 10 mL of cold phosphate buffered saline (PBS). The cells were collected by aspiration of the lavage fluid and centrifugation for 15 min at 1800 rpm and 4°C. Cells were re-suspended in supplemented DMEM media with 10% FBS and 1% penicillin–streptomycin.
For cell viability assay, 1 × 104 cells/well were incubated in 96-well plates with 200 μL of supplemented DMEM media. The plates were placed in a humidified incubator during 48 h, 37°C and 5% CO2. Later, macrophages were treated with the protein hydrolysate and peptide fractions from S. hispanica L. at 1, 10, 100 and 200 μg/mL dissolved in supplemented DMEM media. The plates were incubated for 48 h, 37°C and 5% CO2. In this test, macrophages with 100% DMSO were employed as a positive control, while macrophages without treatment or stimuli were used as a negative control.
For the NO, H2O2, and cytokines determination (IL-1β, IL-6, IL-10, and TNF-α), 1 × 105 cells/well were incubated in 24-well plates with 500 μL of supplemented DMEM media. The plates were also incubated during 48 h, 37°C and 5% CO2. Subsequently, cells were treated with the protein hydrolysate and peptide fractions from S. hispanica L. at 1, 10, 100 and 200 μg/mL dissolved in supplemented DMEM media. The plates were incubated for 48 h, 37°C and 5% CO2. Finally, macrophages were activated by adding LPS at 1 μg/mL and were incubated (48 h, 37°C and 5% CO2). In these assays, macrophages with LPS (1 μg/mL) were used as a positive control and macrophages without treatment or stimuli were employed as a negative control.
2.10.2. Cell viability
The cell viability was determined following the procedure of Debnath, Kim, Nath, and Lee (Citation2017). Supernatants of a 96-well plate with treated macrophages were removed and 20 μL of MTT solution (5 mg/mL in PBS) was added with 180 μL of supplemented DMEM media. Cells were incubated for 4 h, 37°C and 5% CO2. Supernatants were removed and 100 μL of 100% DMSO were added to dissolving the produced- formazan crystals. The absorbances were measured at 490 nm in a Bio-Rad® iMark microplate reader and the cell viability percentage (%CV) was calculated as:where: AbsTxs is the absorbance of treated macrophages with protein derivatives and AbsC(−) is the absorbance of the negative control.
2.10.3. Measurement of NO production
The NO production was measured according to Suleria, Addepalli, Masci, Gobe, and Osborne (Citation2017). Briefly, 50 µL of supernatants from a 24-well plate with treated and activated macrophages were mixed with 50 µL of Griess reagent in a 96-well plate. The plate was incubated during 10 min at room temperature and the absorbances were measured at 490 nm on the microplate reader. Nitrites concentration was determined by comparison with a NaNO2 standard curve (0–200 μM). NO inhibition percentage (%INO) was calculated by the formula:where: NOTxs is the NO concentration of treated macrophages with protein derivatives and NOC(+) is the NO concentration of the positive control.
2.10.4. Measurement of H2O2 release
The H2O2 release was measured using the protocol of Toffoli-Kadri et al. (Citation2014). Aliquots of 100 µL of supernatants from a 24-well plate with treated and activated macrophages were mixed with 100 µL of a fresh phenol red solution (5.5 mM dextrose, 0.056 g phenol red and 8.5 U/mL type I HRP in PBS) in a 96-well plate. The plate was incubated during 3 h at room temperature and the reaction was stopped by adding 10 μL of 1 N NaOH. The absorbances were measured at 620 nm on the microplate reader. H2O2 concentration was determined by comparison with an H2O2 standard curve (0–50 μM). H2O2 inhibition percentage (%IH2O2) was calculated using the formula:where: H2O2Txs is the H2O2 concentration of treated macrophages with protein derivatives and H2O2C(+) is the H2O2 concentration of the positive control.
2.10.5. Determination of cytokines production
The determination of IL-1β, IL-6, IL-10, and TNF-α production was done according to the manufacturer’s instructions for commercial ELISA kits (Peprotech®, London, UK). A capture antibody was used at 2 μg/mL for IL-1β, IL-6 or IL-10 and 1 μg/mL for TNF-α. Serial dilutions of recombinant IL-1β (0–4000 pg/mL), IL-6 (0–4000 pg/mL), IL-10 (0–3000 pg/mL) or TNF-α (0–2000 pg/mL) were used as standard curve. By last, cytokines present in the supernatants of the macrophages were incubated with a detection antibody (0.5 μg/mL for IL-1β, IL-6 or IL-10, 0.25 μg/mL for TNF-α) and avidin-peroxidase. The absorbances were measured at 490 nm using the microplate reader. Cytokines concentration was determined by comparison with the standard curve. Inhibition percentages of IL-1β, IL-6, and TNF-α (%ICytokine) were calculated as:where: CytokineTxs is the IL-1β, IL-6 or TNF-α concentration of treated macrophages with protein derivatives and CytokineC(+) is the IL-1β, IL-6 or TNF-α concentration of the positive control.
2.10.6. In vitro screening
The protein derivative with the highest in vitro anti-inflammatory effect was the 1–3 kDa fraction. Then, the in vivo activity of this fraction was assessed in murine models of TPA-induced ear edema and DNFB-induced delayed-type hypersensitivity.
2.11. In vivo assays
2.11.1. Preparation of samples
An experimental group, a positive control group and a negative control group were used in the in vivo tests (five animals per group). Animals of the experimental group were treated with 100 µL of 1–3 kDa fraction (100 µg/kg) dissolved in 0.9% physiological saline solution (PSS). Mice of the positive control group received 100 µL of indomethacin (10 mg/kg) in 0.9% PSS, a non-steroidal anti-inflammatory drug (NSAID) used as reference substance. Mice of the negative control group only received 100 µL of 0.9% PSS. The samples were administered intragastrically for five days.
2.11.2. TPA-induced ear edema
The induction of ear edema was done according to Gómez and Gil (Citation2011). In the last day of treatments administration, ears of animals were measured with a digital precision micrometer. Immediately, the treatments were administered to the animal groups and 1 h later, TPA (2 µg) in acetone (20 µL) were applied topically to the inner and outer surface of the right ear of each mouse (10 µL to each side). The right ears were measured at 1, 2, 3, 4 and 5 h after topical application. Difference between right and left ears thickness was reported as edema expression. Inhibition percentage of ear edema (%IEE) was calculated using the formula:where: EETxs is the ear thickness in the experimental group and EEC(−) is the ear thickness in the negative control group.
2.11.3. DNFB-induced delayed-type hypersensitivity
The DNFB-induced delayed-type hypersensitivity assay was carried out following the protocol of Fu, Zhang, Peng, Guo, and Chen (Citation2015). In the last day of treatments administration, both ears of animals were measured using the digital precision micrometer. The treatments were administered to the animal groups and 1 h later, the abdomen of mice was shaved. Subsequently, 20 µL of 0.5% DNFB in acetone:olive oil (4:1 v/v) were applied topically to the abdomen of each mouse (first challenge). After 24 h, the abdomen of mice was challenged by application of 20 µL of 0.5% DNFB solution (second challenge). Five days later, the shaved abdomen of mice was challenged again with 20 µL of 0.5% DNFB solution (third challenge), while 10 µL of 0.2% DNFB solution was applied topically to the inner surfaces of right ears. After 48 h, ears of mice were measured and the difference between right and left ears thickness was reported as edema expression. Inhibition percentage of edema (%IE) was calculated as:where: ETxs is the ear thickness in the experimental group and EC(−) is the ear thickness in the negative control group.
2.12. Statistical analysis
Moisture content, protein content and DH results were expressed as the mean ± standard deviation (SD) of each determination by triplicate. In vitro experimental values were expressed as the mean ± SD of three independent experiments in triplicate. Data were analyzed by one-way analysis of variance (ANOVA) with Dunnett’s post hoc test. In vivo experimental values were expressed as the mean ± standard error (SE) of each group. Data were analyzed by two-way ANOVA with Dunnett’s post hoc test. In the biological assays, levels of p < 0.05 were used as a criterion of statistical significance in contrast with the control group. All calculations were done using GraphPad Prism® version 7.00 software (GraphPad Software Inc., CA, USA).
3. Results
3.1. Moisture and protein content
Moisture content in protein isolate from S. hispanica L. was 7.84 ± 0.08%. Also, protein content was determined, getting 90.29 ± 1.33% on dry basis.
3.2. Degree of hydrolysis
As described above, the protein hydrolysate was obtained from protein isolate and the DH was determined, obtaining an average value of 38.31 ± 3.90%.
3.3. Effect of protein derivatives on cell viability
The results demonstrated that protein derivatives did not affect the viability of peritoneal murine macrophages at different concentrations (). Also, the percentages did not show significant differences in comparison to negative control composed by macrophages without treatment or stimuli (100.00%).
Figure 1. Effect of protein derivatives from S. hispanica L. seeds on cell viability. The results represent the mean ± SD of three independent experiments in triplicate. Data were analyzed using one-way ANOVA followed by Dunnett’s post hoc test. Levels of p < 0.05 were considered as statistically significant in contrast to C(−). C(−): negative control, C(+): positive control, H: protein hydrolysate, F: peptide fraction.

3.4. Effect of protein derivatives on NO production and H2O2 release
Values of NO production showed a concentration-dependent manner decrement by treated macrophages with some protein derivatives ((A)). Protein hydrolysate, 3–5 kDa fraction and 1–3 kDa fraction showed this decrement from 10 to 200 µg/mL. The 1–3 kDa fraction presented concentrations of 132.80, 111.30 and 101.30 µM at 10, 100 and 200 µg/mL, respectively. H2O2 release was assessed on treated macrophages, watching different values in each protein derivative ((B)). Protein hydrolysate, >10 kDa fraction and <1 kDa fraction exhibited low concentrations at 200 µg/mL. Only the 1–3 kDa fraction presented a concentration-dependent manner decrease, with 18.74 (8.74% inhibition), 18.23 (11.24% inhibition), 14.80 (27.97% inhibition) and 14.56 µM (29.13% inhibition) at evaluated concentrations.
Figure 2. Effect of protein derivatives from S. hispanica L. seeds on NO (A) and H2O2 (B) production. The results represent the mean ± SD of three independent experiments in triplicate. Data were analyzed using one-way ANOVA followed by Dunnett’s post hoc test. Levels of p < 0.05 were considered as statistically significant in contrast to C(+). C(+): positive control, C(−): negative control, H: protein hydrolysate, F: peptide fraction.

3.5. Effect of protein derivatives on cytokines production
IL-1β concentrations exhibited different trends in all assessed protein derivatives ((A)). Only the >10 kDa fraction and 1–3 kDa fraction demonstrated a concentration-dependent manner inhibitory effect. Nevertheless, the 1–3 kDa fraction showed the IL-1β lowest levels, with 1604.00 (10.25% inhibition), 1141.00 (36.18% inhibition), 884.20 (50.50% inhibition) and 732.20 pg/mL (59.00% inhibition) at evaluated concentrations. Unlike the previous cytokine, IL-6 levels showed similar trends in the protein hydrolysate and peptide fractions ((B)). All protein derivatives presented a concentration-dependent manner decrement, but the 1–3 kDa fraction exerted the highest inhibition of this cytokine, with levels of 1811.00 (14.46% inhibition), 1481.00 (30.05% inhibition), 1069.00 (49.54% inhibition) and 812.50 pg/mL (61.63% inhibition) at 1, 10, 100 and 200 µg/mL, respectively. TNF-α values also followed similar trends in all evaluated protein derivatives ((C)). Once again, the 1–3 kDa fraction showed a concentration-dependent manner decrease, whose values were 778.50 (16.27% inhibition), 655.50 (29.52% inhibition), 547.50 (41.14% inhibition) and 488.50 pg/mL (47.49% inhibition) at evaluated concentrations.
Figure 3. Effect of protein derivatives from S. hispanica L. seeds on IL-1β (A), IL-6 (B), TNF-α (C) and IL-10 (D) production. The results represent the mean ± SD of three independent experiments in triplicate. Data were analyzed using one-way ANOVA followed by Dunnett’s post hoc test. Levels of p < 0.05 were considered as statistically significant in contrast to C(+). C(+): positive control, C(−): negative control, H: protein hydrolysate, F: peptide fraction.

Finally, IL-10 concentrations produced by the cells were determined ((D)). All protein derivatives demonstrated a concentration-dependent manner increase. The highest level of this cytokine was obtained at the maximum concentration evaluated for 1–3 kDa fraction (200 µg/mL), with 1410.00 pg/mL. The concentrations of IL-10 were compared with the positive control, whose production was lower in response to antigenic stimuli (in this case, the LPS) and whose value was 129.00 pg/mL.
3.6. Effect of 1-3 kDa fraction on ear edema
Ear edema in the animals of the experimental group exhibited a significant decrease after TPA topical application, with 0.25 mm thickness and 83.87% inhibition at 2 h (). The highest edema volume was produced in the negative control group (1.55 mm thickness at 2 h), while ear edema of the positive control group showed a maximum inhibition at the same time (0.13 mm thickness and 91.39% inhibition).
Figure 4. Effect of 1–3 kDa fraction from S. hispanica L. seeds on TPA-induced ear edema. The results represent the mean ± SE of each group. Data were analyzed using two-way ANOVA followed by Dunnett’s post hoc test. Levels of p < 0.05 were considered as statistically significant in contrast to C(−). C(−): negative control group, C(+): positive control group, F1-3 (100 µg/kg): experimental group.

3.7. Effect of 1-3 kDa fraction on delayed-type hypersensitivity assay
By last, the effect of 1–3 kDa fraction from S. hispanica L. was analyzed on DNFB-induced delayed-type hypersensitivity assay, obtaining the difference in edema thickness of each animal at days 0 and 8 after the hapten application (). A decrease in ear edema of the experimental group was observed, with 0.95 mm and 22.97% inhibition. The edema inhibition was statistically significant in comparison to the negative control group (1.23 mm thickness). Results of the positive control group were also considered as the maximum inhibition, with 0.75 mm thickness and 39.18% inhibition.
Figure 5. Effect of 1–3 kDa fraction from S. hispanica L. seeds on DNFB-induced delayed hypersensitivity assay. The results represent the mean ± SE of each group. Data were analyzed using two-way ANOVA followed by Dunnett’s post hoc test. Levels of p < 0.05 were considered as statistically significant in contrast to C(−). C(−): negative control group, C(+): positive control group, F1-3 (100 µg/kg): experimental group.

4. Discussion
The aim of this study was to determine the anti-inflammatory effects of protein derivatives isolated from S. hispanica L. seeds. Firstly, the protein content (90.29%) of protein isolate was determined, which was similar in comparison with the protein concentration of other S. hispanica L. derivatives evaluated in several studies. For example, Chim-Chi, Gallegos-Tintoré, Jiménez-Martínez, Dávila-Ortiz, and Chel-Guerrero (Citation2018) analyzed the proximate composition of a protein concentrate isolated from defatted flour, obtaining a protein content of 77.26%. In another study, Timilsena, Adhikari, Barrow, and Adhikari (Citation2016) also determined the protein percentage of a protein isolate obtained from the seeds, whose value was 92.50%. The slight variations can be attributed to various reasons. In our research, components such as carbohydrates or oils were removed through the mucilage extraction and defatting with the solvent. Even, the remaining non-protein components were removed by sifting with a 140 μm mesh. In the same way, the method of alkaline solubilization and isoelectric precipitation used in this work helped to obtain the protein isolate with a high concentration of protein, making it a good material for the production of the protein hydrolysate.
The DH is a fundamental property of a protein hydrolysate because it helps to determine its characteristics and possible use. Previous studies have described that hydrolysates can be classified into three types: hydrolysates with a low DH (between 1% and 10%, for improvement of functional properties), hydrolysates with variable DH (for use as flavorings) and extensive hydrolysates with DH higher than 10% (for use in specialized food because of its nutritional, physiological or biological properties) (Benítez, Ibarz, & Pagan, Citation2008). Production of extensive hydrolysates requires more than one protease because a single enzyme cannot achieve such high DHs within a reasonable time period (Segura Campos, Peralta González, Chel Guerrero, & Betancur Ancona, Citation2013). For this reason, in the present work was used a Pepsin®-Pancreatin® system to produce the hydrolysate from S. hispanica L. Also, the DH calculated for the protein hydrolysate allowed to categorize it as an extensive hydrolysate.
On the other hand, the percentage obtained in this study are comparable to values of DH reported in other investigations with S. hispanica L. Segura-Campos, Salazar-Vega, et al. (Citation2013) obtained a DH of 43.80% using Alcalase® and Flavourzyme®, for a reaction time of 150 min. In another study carried out by Segura Campos, Peralta González, et al. (Citation2013), the Alcalase®-Flavourzyme® system was also used during 150 min for the production of a protein hydrolysate and the DH was calculated, obtaining 51.60%. The slight variations observed between DHs may be due mainly to reaction time since a long time can generate a higher DH. However, these data support the fact that S. hispanica L. is a good natural resource to obtain extensive protein hydrolysates with possible anti-inflammatory activity. Additionally, a protein hydrolysate with the described characteristics represents an important source of bioactive peptides with different molecular weight, so the hydrolysate was subjected to an ultrafiltration fractionation step through membranes of different MWCO, obtaining the peptide fractions. Then, the in vitro anti-inflammatory activity of these protein derivatives was assessed over peritoneal murine macrophages.
In the development of the oxidative stress and inflammation, the excessive production of reactive species (for example, NO and H2O2) plays a crucial role. Likewise, the oxidative stress contributes to the pathophysiology of a wide number of illnesses, such as cardiovascular diseases, cancer or diabetes (Lugrin et al., Citation2014). In this study, some protein derivatives isolated from S. hispanica L. seeds exhibited a concentration-dependent manner decrement in the NO and H2O2 release by cells. Besides, other studies have demonstrated the suppressive effect of protein derivatives from commercial-importance grains over the production of ROS or RNS. For example, Ndiaye, Vuong, Duarte, Aluko, and Matar (Citation2012) demonstrated that <3 kDa fraction isolated from Pisum sativum L. seeds (yellow field pea) caused an inhibition in NO production by activated RAW 264.7 macrophages. Udenigwe, Lu, Han, Hou, and Aluko (Citation2009) evaluated the NO production by RAW 264.7 macrophages treated with low molecular weight peptide fractions (<1 kDa) obtained of defatted flour from Linum usitatissimum (flaxseed), concluding that peptide fractions exerted an inhibitory effect over NO.
These studies establish that low molecular weight peptide fractions exert significant effects in the decrease of RNS. Low molecular weight peptide fractions from S. hispanica L. showed a similar trend and it is possible that present inhibitory effects on other related markers, such as the superoxide radical (O2.-) production, superoxide dismutase (SOD) expression or inducible nitric oxide synthase (iNOS) expression, which would clarify the mechanism in the inhibition of RNS and ROS.
Cytokines are low molecular weight proteins that play a vital role in regulating the inflammatory response. Each cytokine binds to a specific cell surface receptor, generating a signalling cascade that affects the cell function. This may stimulate the production of other cytokines, increase the number of cell surface receptors for other molecules or suppress the cytokine’s own effect (Arango Duque & Descoteaux, Citation2014). In our work, the protein derivatives obtained from S. hispanica L. decreased values of pro-inflammatory cytokines (IL-1β, IL-6, and TNF-α) and increased levels of an anti-inflammatory cytokine (IL-10). The 1–3 kDa fraction caused a concentration-dependent manner decrement in the production of all evaluated pro-inflammatory cytokines, exhibiting the lowest levels. The greatest possible inhibitory effect was observed at the maximum concentration evaluated, which indicates that the low molecular weight peptides contained in this fraction possess important anti-inflammatory properties at high doses. On the other hand, several studies have published the effect of peptides obtained from commercial-interest seeds over the production of inflammatory cytokines. For example, Ndiaye et al. (Citation2012) showed that <3 kDa fraction from P. sativum L. seeds exerted a decrement in IL-6 and TNF-α levels secreted by RAW 264.7 macrophages. In the same way, Udenigwe, Je, Cho, and Yada (Citation2013) evaluated the protein hydrolysate and peptide fractions isolated from almond, demonstrating that >5 kDa fraction inhibited the production of IL-1β, IL-6, and TNF-α by RAW 264.7 macrophages. Even, this fraction also inhibited gene expression of the same cytokines.
These works establish a relation between the size of peptides contained in the protein derivatives and the anti-inflammatory activity, due to low molecular weight peptide fractions presented important effects. Consequently, peptides in the low molecular weight fractions from S. hispanica L. also could follow a similar mechanism by the inhibition of the expression of nuclear factor κB (NF-κB) or another transcription factor, as well as the expression of genes for IL-1β, IL-6, and TNF-α. Additionally, it would be important to evaluate other factors related to the production of these mediators. First, a concentration-dependent increase in the production of IL-10 was observed, so the evaluation of other anti-inflammatory cytokines related to macrophages, such as transforming growth factor β (TGF-β), would provide important information on this type of mediators. Likewise, evaluation of cytokines related to other cells of the immune system, such as interleukin 4 (IL-4), interleukin 5 (IL-5) or interferon γ (IFN-γ), would help to know the effect of protein derivatives on other processes involved in inflammation. The obtained results in NO, H2O2 and cytokines production were analyzed, demonstrating that 1–3 kDa fraction had the highest anti-inflammatory effect on these markers. Therefore, this fraction was selected and it is in vivo activity was evaluated in different murine models.
TPA is an inducing agent of inflammation that is applied topically to mice ears and stimulates cellular infiltration with the release of mediators like prostaglandins, which increase vascular permeability and promote the edema formation within 1–4 h (Bralley, Greenspan, Hargrove, Wicker, & Hartle, Citation2008). Cyclooxygenases (COXs) are enzymes involved in prostaglandins synthesis. Therefore, the inflammation on TPA-induced ear edema is decreased with NSAIDs (as indomethacin) since inhibiting COXs, block prostaglandins production and decrease the inflammation (Gómez & Gil, Citation2011). Currently, there are no reports about the evaluation of proteins and peptides obtained from animal or vegetable sources on the TPA-induced ear edema test. However, some studies have reported the anti-inflammatory activity of protein derivatives isolated from several sources on other similar in vivo models. De Carvalho-Silva et al. (Citation2012) analyzed the effect of a whey protein hydrolysate on mice with paw edema, which caused an inhibition in edema development 3 h after carrageenan induction. Tavares et al. (Citation2013) showed that peptide concentrate obtained from whey proteins exerted a decrement on carrageenan-induced paw edema after 4 h.
These studies attribute the anti-inflammatory effect to inhibition of involved enzymes in prostaglandins production (as COXs). Thus, the significant inhibitory effect in ear edema of mice group treated with the 1–3 kDa fraction could be produced at this level. The analysis of other inflammatory mediators, such as COXs expression or prostaglandins production by immune system cells (for example, macrophages) would be useful to clarify the mechanism of inflammation inhibition.
Cell-mediated immunity regulates the delayed-type hypersensitivity reactions (type IV), whose beginning involves the participation of CD4+ and CD8+ T lymphocytes. The mechanism is initiated as a response to a variety of viruses, fungi, and parasites, as well as graft rejection and contact skin sensitivity to chemical agents (Kobayashi, Kaneda, & Kasama, Citation2001). Contact hypersensitivity has been widely studied on murine models of delayed-type hypersensitivity and consists of two phases, named sensitization and elicitation phases (Honda, Egawa, Grabbe, & Kabashima, Citation2013). In this sense, DNFB has been the most used hapten on contact hypersensitivity models (Fu et al., Citation2015). In the sensitization phase, the mouse abdominal skin is exposed to hapten for the first time. Haptens are non-immunogenic molecules by themselves but they form highly immunogenic complexes upon binding to skin proteins. The complexes are recognized by antigen-presenting cells (APCs) and presented to T lymphocytes as haptenated peptides. Activated T lymphocytes proliferate and circulate between lymphoid organs and skin. The elicitation phase occurs after re-exposure of the animal skin to hapten, in a different site to first contact (as the ear). This phase implicates the release of cytokines by circulating cells (IFN-γ, TNF-α, etc.) and recruitment of neutrophils and specific T lymphocytes, resulting to vasodilation, inflammation, and edema 24–48 h after antigenic challenge (Christensen & Haase, Citation2012).
Today, there are no studies about the activity of proteins and peptides on DNFB-induced delayed-type hypersensitivity model. However, the inhibition in edema of animals treated with the 1–3 kDa fraction could be attributed to inhibition of the signaling pathways factors involved in pro-inflammatory cytokines production (such as NF-κB) or the suppression of chemotactic process mediators. Therefore, the evaluation of these parameters would provide information on the role of this protein derivative in delayed-type hypersensitivity reactions.
Finally, obtained results on the in vitro and in vivo assays showed that low molecular weight peptide fractions exerted significant effects on inflammation. The excessive release of NO and H2O2 is involved in the inflammatory process, so ear edema decrement could be due to the inhibition of reactive species. In the same way, decrease in the production of pro-inflammatory cytokines (IL-1β, IL-6, and TNF-α) could be correlated with the inhibition in the development of edema, since cytokines stimulate the chemotactic process and the entry of cells to the inflammation site. In addition, this is the first study that evaluated the anti-inflammatory properties of protein derivatives isolated from S. hispanica L. seeds, establishing a basis for future research with this plant. Nevertheless, more pharmacological studies are necessary before considering its future application in inflammatory diseases.
5. Conclusion
The results demonstrated that protein hydrolysate and peptide fractions isolated from S. hispanica L. seeds possess anti-inflammatory effects without affecting the viability of macrophages. The enzymatic hydrolysis and ultrafiltration process helped to obtain the protein derivatives with significant biological activity. Nevertheless, the 1–3 kDa fraction exhibited the highest anti-inflammatory effect on NO, H2O2, IL-1β, IL-6, IL-10 and TNF-α production. Also, the 1–3 kDa fraction showed significant inhibitory effects on TPA-induced ear edema and DNFB-induced delayed-type hypersensitivity model.
Disclosure statement
No potential conflict of interest was reported by the authors.
Additional information
Funding
References
- Arana-Argáez, V. E., Chan-Zapata, I., Canul-Canche, J., Fernández-Martín, K., Martín-Quintal, Z., Torres-Romero, J. C., … Ramírez-Camacho, M. A. (2017). Immunosuppresive effects of the methanolic extract of Chrysophyllum cainito leaves on macrophage functions. African Journal of Traditional, Complementary and Alternative Medicines, 14(1), 179–186.
- Arango Duque, G., & Descoteaux, A. (2014). Macrophage cytokines: Involvement in immunity and infectious diseases. Frontiers in Immunology, 5(491), 1–12.
- Benítez, R., Ibarz, A., & Pagan, J. (2008). Hidrolizados de proteína: procesos y aplicaciones. Acta Bioquímica Clínica Latinoamericana, 42(2), 227–236.
- Bralley, E. E., Greenspan, P., Hargrove, J. L., Wicker, L., & Hartle, D. K. (2008). Topical anti-inflammatory activity of Polygonum cuspidatum extract in the TPA model of mouse ear inflammation. Journal of Inflammation, 5(1), 1–7. doi: 10.1186/1476-9255-5-1
- Chim-Chi, Y., Gallegos-Tintoré, S., Jiménez-Martínez, C., Dávila-Ortiz, G., & Chel-Guerrero, L. (2018). Antioxidant capacity of Mexican chia (Salvia hispanica L.) protein hydrolyzates. Journal of Food Measurement and Characterization, 12(1), 323–331. doi: 10.1007/s11694-017-9644-9
- Christensen, A. D., & Haase, C. (2012). Immunological mechanisms of contact hypersensitivity in mice. Acta Pathologica, Microbiologica et Immunologica Scandinavica, 120(1), 1–27. doi: 10.1111/j.1600-0463.2011.02832
- Cunniff, P. (1997). Official methods of analysis of AOAC international. Gaithersburg, MD: AOAC International.
- Debnath, T., Kim, E. K., Nath, N. C. D., & Lee, K. G. (2017). Therapeutic effects of Ligularia stenocephala against inflammatory bowel disease by regulating antioxidant and inflammatory mediators. Food and Agricultural Immunology, 28(6), 1142–1154. doi: 10.1080/09540105.2017.1332008
- De Carvalho-Silva, L. B., Bertoldo Pacheco, M. T., Bertoldo, R., De Carvalho Veloso, C., Costa Teodoro, L., Giusti-Paiva, A., … Soncini, R. (2012). Anti-inflammatory activities of enzymatic (alcalase) hydrolysate of a whey protein concentrate. African Journal of Biotechnology, 11(12), 2993–2999.
- Doyon, M., & Labrecque, J. (2008). Functional foods: A conceptual definition. British Food Journal, 110(11), 1133–1149. doi: 10.1108/00070700810918036
- Fu, R., Zhang, Y., Peng, T., Guo, Y., & Chen, F. (2015). Phenolic composition and effects on allergic contact dermatitis of phenolic extracts Sapium sebiferum (L.) Roxb. leaves. Journal of Ethnopharmacology, 162, 176–180. doi: 10.1016/j.jep.2014.12.072
- Gautam, R., & Jachak, S. M. (2009). Recent developments in anti-inflammatory natural products. Medicinal Research Reviews, 29(5), 767–820. doi: 10.1002/med.20156
- Gómez, M., & Gil, J. F. (2011). Topical anti-inflammatory activity of Calea prunifolia HBK (Asteraceae) in the TPA model of mouse ear inflammation. Journal of the Brazilian Chemical Society, 22(12), 2391–2395. doi: 10.1590/S0103-50532011001200021
- Herrera Chalé, F. G., Ruiz Ruiz, J. C., Acevedo Fernández, J. J., Betancur Ancona, D. A., & Segura Campos, M. R. (2014). ACE inhibitory, hypotensive and antioxidant peptide fractions from Mucuna pruriens proteins. Process Biochemistry, 49(10), 1691–1698. doi: 10.1016/j.procbio.2014.06.021
- Honda, T., Egawa, G., Grabbe, S., & Kabashima, K. (2013). Update of immune events in the murine contact hypersensitivity model: Toward the understanding of allergic contact dermatitis. Journal of Investigative Dermatology, 133(2), 303–315. doi: 10.1038/jid.2012.284
- Ixtaina, V. Y., Nolasco, S. M., & Tomás, M. C. (2008). Physical properties of chia (Salvia hispanica L.) seeds. Industrial Crops and Products, 28(3), 286–293. doi: 10.1016/j.indcrop.2008.03.009
- Joshi, I., Sudhakar, S., & Nazeer, R. A. (2016). Anti-inflammatory properties of bioactive peptide derived from gastropod influenced by enzymatic hydrolysis. Applied Biochemistry and Biotechnology, 180(6), 1128–1140. doi: 10.1007/s12010-016-2156-y
- Kim, Y. S., Young, M. R., Bobe, G., Colburn, N. H., & Milner, J. A. (2009). Bioactive food components, inflammatory targets, and cancer prevention. Cancer Prevention Research, 2(3), 200–208. doi: 10.1158/1940-6207.CAPR-08-0141
- Kobayashi, K., Kaneda, K., & Kasama, T. (2001). Immunopathogenesis of delayed-type hypersensitivity. Microscopy Research and Technique, 53(4), 241–245. doi: 10.1002/jemt.1090
- Laviada-Castillo, R. E., Segura-Campos, M. R., Chan-Zapata, I., Torres-Romero, J. C., Guillermo-Cordero, J. L., & Arana-Argáez, V. E. (2019). Immunosuppressive effects of protein derivatives from Mucuna pruriens on a streptozotocin-induced type 1 diabetes murine model. Journal of Food Biochemistry, 43(5), 1–10. doi: 10.1111/jfbc.12834
- Lawrence, T., & Fong, C. (2010). The resolution of inflammation: Anti-inflammatory roles for NF-κB. The International Journal of Biochemistry & Cell Biology, 42(4), 519–523. doi: 10.1016/j.biocel.2009.12.016
- Lugrin, J., Rosenblatt-Velin, N., Parapanov, R., & Liaudet, L. (2014). The role of oxidative stress during inflammatory processes. Biological Chemistry, 395(2), 203–230. doi: 10.1515/hsz-2013-0241
- Mittal, M., Siddiqui, M. R., Tran, K., Reddy, S. P., & Malik, A. B. (2014). Reactive oxygen species in inflammation and tissue injury. Antioxidants & Redox Signaling, 20(7), 1126–1167. doi: 10.1089/ars.2012.5149
- Nathan, C., & Ding, A. (2010). Nonresolving inflammation. Cell, 140(6), 871–882. doi: 10.1016/j.cell.2010.02.029
- Ndiaye, F., Vuong, T., Duarte, J., Aluko, R. E., & Matar, C. (2012). Anti-oxidant, anti-inflammatory and immunomodulating properties of an enzymatic protein hydrolysate from yellow field pea seeds. European Journal of Nutrition, 51(1), 29–37. doi: 10.1007/s00394-011-0186-3
- Okin, D., & Medzhitov, R. (2012). Evolution of inflammatory diseases. Current Biology, 22(17), R733–R740. doi: 10.1016/j.cub.2012.07.029
- Orona-Tamayo, D., Valverde, M. E., Nieto-Rendón, B., & Paredes-López, O. (2015). Inhibitory activity of chia (Salvia hispanica L.) protein fractions against angiotensin I-converting enzyme and antioxidant capacity. LWT - Food Science and Technology, 64(1), 236–242. doi: 10.1016/j.lwt.2015.05.033
- Rangaraju, A., & Kumar, U. M. (2013). A pharmacognostic study on Salvia hispanica. American Journal of Pharmacy & Health Research, 1(9), 27–37.
- Segura Campos, M. R., Peralta González, F., Chel Guerrero, L., & Betancur Ancona, D. (2013). Angiotensin I-converting enzyme inhibitory peptides of chia (Salvia hispanica) produced by enzymatic hydrolysis. International Journal of Food Science, 2013, 1–8. doi: 10.1155/2013/158482
- Segura-Campos, M. R., Salazar-Vega, I. M., Chel-Guerrero, L. A., & Betancur-Ancona, D. A. (2013). Biological potential of chia (Salvia hispanica L.) protein hydrolysates and their incorporation into functional foods. LWT - Food Science and Technology, 50(2), 723–731. doi: 10.1016/j.lwt.2012.07.017
- Suleria, H. A. R., Addepalli, R., Masci, P., Gobe, G., & Osborne, S. A. (2017). In vitro anti-inflammatory activities of blacklip abalone (Haliotis rubra) in RAW 264.7 macrophages. Food and Agricultural Immunology, 28(4), 711–724. doi: 10.1080/09540105.2017.1310186
- Tavares, T. G., Spindola, H., Longato, G., Pintado, M. E., Carvalho, J. E., & Malcata, F. X. (2013). Antinociceptive and anti-inflammatory effects of novel dietary protein hydrolysate produced from whey by proteases of Cynara cardunculus. International Dairy Journal, 32(2), 156–162. doi: 10.1016/j.idairyj.2013.05.010
- Timilsena, Y. P., Adhikari, R., Barrow, C. J., & Adhikari, B. (2016). Physicochemical and functional properties of protein isolate produced from Australian chia seeds. Food Chemistry, 212(1), 648–656. doi: 10.1016/j.foodchem.2016.06.017
- Toffoli-Kadri, M. C., Carollo, C. A., Días Lourenço, L., Felipe, J. L., Brandini Néspoli, J. H., Campos Wollf, L. G., … de Siqueira, J. M. (2014). In vivo and in vitro anti-inflammatory properties of Achyrocline alata (Kunth) DC. Journal of Ethnopharmacology, 153(2), 461–468. doi: 10.1016/j.jep.2014.03.008
- Udenigwe, C. C., & Aluko, R. E. (2012). Food protein-derived bioactive peptides production, processing, and potential health benefits. Journal of Food Science, 71(1), 11–24. doi: 10.1111/j.1750-3841.2011.02455
- Udenigwe, C. C., Je, J. Y., Cho, Y. S., & Yada, R. Y. (2013). Almond protein hydrolysate fraction modulates the expression of proinflammatory cytokines and enzymes in activated macrophages. Food & Function, 4, 777–783. doi: 10.1039/C3FO30327F
- Udenigwe, C. C., Lu, Y. H., Han, C. H., Hou, W. C., & Aluko, R. E. (2009). Flaxseed protein-derived peptide fractions: Antioxidant properties and inhibition of lipopolysaccharide-induced nitric oxide production in murine macrophages. Food Chemistry, 116(1), 277–284. doi: 10.1016/j.foodchem.2009.02.046
- Velnar, T., Bailey, T., & Smrkolj, V. (2009). The wound healing process: An overview of the cellular and molecular mechanisms. Journal of International Medical Research, 37(5), 1528–1542. doi: 10.1177/147323000903700531
- Zhang, J. M., & An, J. (2007). Cytokines, inflammation and pain. International Anesthesiology Clinics, 45(2), 27–37. doi: 10.1097/AIA.0b013e318034194e