ABSTRACT
A growing number of people choose plant-based milk products to replace dairy for health reasons or veganism. Since fermentation is a promising method to improve the nutritional value of plant-based milk, this study aimed to establish the process of oat milk fermentation and to evaluate the effect of fermented oat milk (FOM) on modulating antigen-specific immune response in ovalbumin (OVA)-sensitized mice. The FOM was prepared by fermentation of oat milk with Streptococcus thermophilus, Lactobacillus bulgaricus and proteolytic L. rhamnosus. Addition of 1% glucose improved the process of fermentation. FOM was stable while stored at 4°C within seven days. Oral administration with FOM (0.4 and 2 g/kg body weight) did not alter the serum level of OVA-specific IgG and spleen index. However, FOM had comparable potency with commercial dairy yogurt to lower stimulation index, IL-2, IFN-γ and IL-4 production and to promote TGF-β and IL-10 secretion by OVA-stimulated splenocytes.
Introduction
Fermented dairy products, including yogurts, are consumed as vehicle for delivering probiotics to consumers. Yogurt is formed through the coagulation of milk protein by lactic acid, which is produced by the fermentation of LAB with Lactobacillus bulgaricus and Streptococcus thermophilus being the most common strains (Chandan, Citation2006). Yogurt is not only highly recommended for those suffering from gastrointestinal disorders and lactose intolerance, but is beneficial to boost the immune system (Boyle & Tang, Citation2006; Ranadheera et al., Citation2017). All the time, fermented dairy products are the predominant commercial food matrices in the probiotic market. However, vegan or people who had allergy to milk proteins, lactose intolerance or hyperlipidaemia are eager for alternatives of dairy products (Silanikove et al., Citation2015). Therefore, the development of new natural components and novel non-dairy products is of great interest in the food industry. For example, drinks from various vegetables and cereals were made in order to replace traditional dairy drinks (Coda et al., Citation2012). The effectiveness of probiotics is associated with their cell viability, which could be improved by adding prebiotics (Terpou et al., Citation2019). Compared to other types of cereals, oat is rich in dietary fibre, including β-glucan, as prebiotic matrix for improving cell viability of probiotics. (Chen, Mah, et al., Citation2021). Both β-glucan and oat peptides were also demonstrated as the major bioactive components beneficial for immune systems (Chen, Mah, et al., Citation2021; Comino et al., Citation2016).
With respect to the common microorganisms utilized for yogurt fermentation, both L. bulgaricus and S. thermophilus are well-known probiotics with immunomodulatory activities (Dargahi et al., Citation2021). In addition, the immunomodulatory activities of L. rhamnosus strains have been mentioned. For example, oral administration of L. rhamnosus CRL1505 to mice would beneficially regulate the balance between pro-inflammatory mediators and IL-10, allowing an effective inflammatory response against infection and avoiding tissue damage (Villena et al., Citation2012). L. rhamnosus LA68 treatment increased responsiveness of splenocytes, activation of the T helper (Th)1 immune response in mice (Dimitrijevic et al., Citation2014) L. rhamnosus JB-1 supplementation increased all T lymphocyte subset proportions in laying hen pullets (Mindus et al., Citation2021). In this study, L. rhamnosus BCRC 10940 was utilized for oat milk fermentation owing to its proteolysis ability (Chen, Hsieh, et al., Citation2021), although the impact of L. rhamnosus BCRC 10940 on immune responses remains unclear.
Due to the increasing prevalence of allergic and autoimmune diseases, clinicians and scientists are urgent to develop novel strategies that elicit antigen-specific immune tolerance but keep immune reactivity to infectious pathogens and tumour antigens (Nazimek & Bryniarski, Citation2020). Antigen-specific T cell cytokines are mediators produced by Th cells and regulatory T (Treg) cells, which perform the function of these cells in an antigen-specific immune response (Nazimek & Bryniarski, Citation2020). Antibodies are major components of humoral immunity, and the classes of antibody expression were also influenced by T cell cytokines. IgG is the main class of antibody in serum known to prevent against pathogen infection (Barker, Citation2019). Moreover, IgG targeting to important IgE epitopes could be induced by antigen-specific immunotherapy, leading to competition with IgE and prevention of type I hypersensitivity (Shamji et al., Citation2021). The balance between the subsets of T cells, both effectors, such as Th cells, and regulatory T (Treg) cells, play a crucial role in the control of inflammatory and allergic conditions (Jäger & Kuchroo, Citation2010). An important feature of T cell activation is the production of cytokines. For example, interleukin (IL)-2 leads to T cell clonal expansion; interferon (IFN)-γ elicits different inflammatory responses that are characteristics of certain autoimmune diseases and delayed-type hypersensitivity; IL-4 results in immunoglobulin (Ig)E isotype switching and the subsequent type I hypersensitivity; IL-10 and TGF-β limit host immune response and maintain immune tolerance (Jäger & Kuchroo, Citation2010). Spleen is the largest secondary lymphoid organ and rich in T and B cells. Spleen weight index is an indicator of immune strength in mammals, and stimulation index of splenocytes in response to antigen is a common indicator for evaluating antigen-specific cell proliferation (Corbin et al., Citation2008).
Although the immunomodulatory activity of traditional yogurt (fermented cow milk; FCM) has been reported, limited information pertaining to the influence of plant-based milk and even yogurt on antigen-specific immune responses is available. Because of the immunomodulatory properties of β-glucan, L. bulgaricus and S. thermophilus and proteolysis ability of L. rhamnosus BCRC 10940, it was hypothesized that oat milk fermented with mix strains of L. bulgaricus, S. thermophilus and L. rhamnosus might exert influence on modulating antigen-specific immune response. The process and storage stability of fermented oat milk (FOM) were optimized and evaluated, respectively. Further, ovalbumin (OVA)-sensitized mice were employed to understand the impact of FOM on OVA-specific IgG production and T cell-associated cytokine secretion. The results obtained from FOM-treated mice were compared to that from commercial fermented cow milk (FCM)-treated mice.
Materials and methods
Bacterial strains, chemicals, reagents, detection kits and oat
Lactobacillus bulgaricus BCRC 10696, S. thermophilus BCRC 12268 and L. rhamnosus BCRC 10940 were purchased from Food Industry Research and Development Institute (Hsinchu, Taiwan). Chemicals and reagents, including ovalbumin (grade V), were purchased from Sigma Chemical (St. Louis, MO, USA). The reagents for bacterial culture and cell culture were purchased from Difco Laboratories (Detroit, MI, USA) and GE Healthcare Life Sciences (Marlborough, MA, USA). The enzyme-linked immunosorbent assay (ELISA) kits for cytokine and immunoglobulin (Ig) measurement were purchased from eBioscience, Inc. (San Diego, CA, USA). Rolled oats (Avena sativa L.) were purchased from Kugi Food Corporation (New Taipei, Taiwan) and commercial yogurt (FCM; raw milk fermented with S. thermophilus, L. bulgaricus, L. acidophilus and B. lactis) were purchased from Uni-President Enterprises Corporation (Tainan, Taiwan).
Preparation and fermentation of oat milk
The strains of L. bulgaricus, S. thermophilus and L. rhamnosus were kept in glycerol-containing MRS (50%, v/v) individually at −80°C. Before inoculation, the strains were subcultured twice in fresh MRS broth without glycerol at 37°C for 48 h. To prepare the oat milk, 120 g of oats and 1 L of distilled water were blended using a Mixer (KJSC15G, Sampo Corporation, Taoyuan, Taiwan) for 1 min, and the mixture was then strained to extract the milk (Raikos et al., Citation2020). For fermentation, the oat milk was heated at 90°C for 30 min followed by inoculation of L. bulgaricus, S. thermophilus and L. rhamnosus at 2% (v/v) to yield an initial concentration of about 107 cells per mL. The mixture was incubated at 37°C for 48 h. While the pH value reached to 4.4–4.6, part of the FOM was kept at 4°C for storage test, and the other was used for animal experiment.
Determination of LAB count, pH value, titratable acidity, protein and peptide concentration of FOM
At the 0, 6, 12, 18, 24, 36 and 48 h of fermentation and the 0, 3, 7 and 14 day of storage, 2 mL of FOM was isolated to determine the pH value using the pH metre (Thermo Eutech pH 510, Thermo Fisher Scientific Inc., Waltham, MA, USA), and 5 mL of FOM was isolated to determine the titratable acidity according to the methods described in the previous study (O’Connor, Citation1995). Plate count in MRS agar plate was employed to determine the LAB count. The concentration of protein was determined by using the method reported by Lowry et al., and bovine serum albumin was employed as the standard (Lowry, Citation1951). The concentration of peptide was determined by using the method reported by Chen et al., and casein tryptone was utilized as the standard (Chen et al., Citation2007).
Ethics statement and animal experiments
Specific pathogen-free BALB/c mice (female, five weeks old) were obtained from the National Laboratory Animal Center of Taiwan. All mice were housed for one week at the laboratory animal facility of the National Taiwan Ocean University (NTOU). The study was conducted according to the guidelines of the Declaration of Helsinki, and approved by the Institutional Animal Care and Use Committee of the National Taiwan Ocean University (NTOU-109072).
The mice were divided into naïve group (NA), vehicle group (VH) and treatment groups (six mice per group). In the VH group, mice were daily treated with 0.1 mL of phosphate-buffered saline (PBS) by gavage. The mice in treatment groups were daily administered with 0.8 or 2 g/kg body weight of FOM (FOML or FOMH) or with 2 g/kg body weight of FCM samples dissolved in 0.1 mL of PBS by gavage. Except for mice in the NA group, the mice were sensitized by intraperitoneally injected with 50 μg of OVA and 1 mg of aluminium hydroxide adjuvant on day 4 and a double dose of OVA and aluminium adjuvant on day 18. On day 31, the mice were euthanized, and the blood and spleen samples were harvested to prepare serum and splenocytes suspensions for the following experiments (Wei et al., Citation2022).
Treatment of splenocytes and measurement of cytokine and Ig
Splenocytes (5 × 106 cells/mL) were seeded into 96-well culture plates (0.1 mL/well) and incubated in the presence of OVA (10 μg/mL) for 68 h. A 3-(4,5-dimethylthiazol-2-yl)-2,5-diphenyl-tetrazolium bromide (MTT) stock solution (5 mg/mL in PBS) was added to each well (10 μL/well) and incubated for 4 h. At the end of incubation, the formed formazan was dissolved with 10% dimethyl sulphoxide, and then the plates were read at 570 nm (Wei et al., Citation2022). On the other hand, the supernatants of splenocytes cultured in the presence of OVA (10 μg/mL) were collected, and the levels of IFN-γ, IL-2, IL-4, IL-10 and TGF-β, in supernatants were measured by enzyme-linked immunosorbent assay (ELISA) as described below (Wei et al., Citation2022). Moreover, the level of OVA-specific IgG in serum was also measured by ELISA. According to the supplier’s instruction of ELISA kits, 100 μL of OVA (0.5 mg/mL) or capture antibody solution was added into each well of 96-well plate and incubated at 4°C overnight. After washing with PBS three times, 100 μL of ELISA diluent was added into each well and incubated at room temperature (RT) for 1 h. After washing, 100 μL of diluted standard, serum or supernatant samples were added into the wells and incubated at RT for 2 h. After washing, 100 μL of detection antibody solution was added into each well and incubated at RT for 1 h. After washing, 100 μL of HRP solution was added into each well and incubated at RT for 30 min. Finally, the wells were washed seven times, and 100 μL of tetramethylbenzidine substrate solution was added into each well. After incubation for 15 min, 50 μL of sulphuric acid (6 N) was added into each well to stop the colour reaction. The absorbance value at 450 nm of absorbance wavelength was measured by a multi-detection microplate reader (Synergy HT, Agilent Technologies, Inc., Santa Clara, CA, USA). The absorbance value of each supernatant sample was calculated from the standard curve to obtain the concentrations of cytokines.
Statistical analysis
Data are expressed as mean ± standard deviation. P-value <.05 was considered a statistically significant difference analysed by one-way ANOVA followed by Duncan’s multiple range test using IBM SPSS Statistics software (International Business Machines Corporation 2017; Armonk, NY, USA).
Results
Supplement of 1% glucose improved the fermentation of oat milk
To compare immune activity of FOM to traditional FCM, typical bacterial strains, including L. bulgaricus, S. thermophilus as well as L. rhamnosus were utilized for oat milk fermentation. Within 48 h of fermentation, the number of LAB was increased less than 1 log, and the level of pH and titratable acidity was altered limitedly ((A–C)). To improve the fermentation rate, 1% glucose was added in the mixture to elevate the level of carbon source for LAB. Compared to that without glucose supplement, significantly increased LAB number and titratable acidity and decreased pH value were observed in oat milk fermented with 1% glucose supplement ((A–C)). Additionally, protein concentration of oak milk fermented with 1% glucose supplement was higher than that without glucose supplement, although protein concentration of these two samples was sharply declined after 36 h of fermentation ((D)).
Figure 1. Influence of 1% glucose addition on oat milk fermentation. Homogenized oat milk was inoculated with S. thermophilus, L. bulgaricus and L. rhamnosus followed by incubation at 37°C for 48 h. Analysis of (A) LAB count, (B) pH value, (C) titratable acidity and (D) protein concentration of fermented oat milk was performed at the anticipated time points. Results are expressed as means ± standard deviation (n = 3).
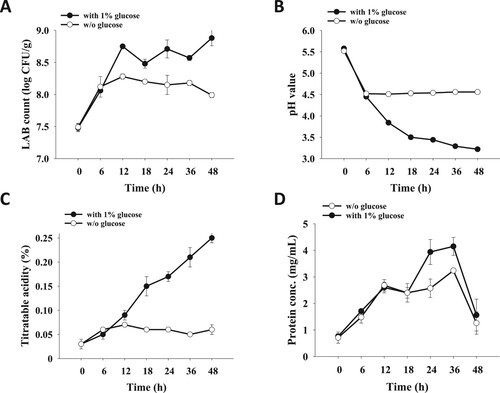
Storage stability of FOM
While the pH value reached to 4.4–4.6, the oat milk fermented with 1% glucose supplement was further kept at 4°C for 14 days to understand the storage stability by measuring LAB count, pH value, titratable acidity and peptide concentration kinetically. The LAB count of FOM was increased in the first three days, whereas it was eminently decreased after seven days of storage ((A)). In parallel, the pH value and titratable acidity of FOM were diminished and raised, respectively, over time ((B,C)). Concordantly, the peptide concentration of FOM was reduced in the first three days and kept at a stable level in the following 11 days ((D)).
Figure 2. Storage test of fermented oat milk at 4°C. While the pH value reached to 4.4–4.6, the fermented oak milk was storage at 4°C, and (A) LAB count, (B) pH value, (C) titratable acidity and (D) protein concentration of fermented oat milk was determined at the anticipated time points. Results are expressed as means ± standard deviation (n = 3).
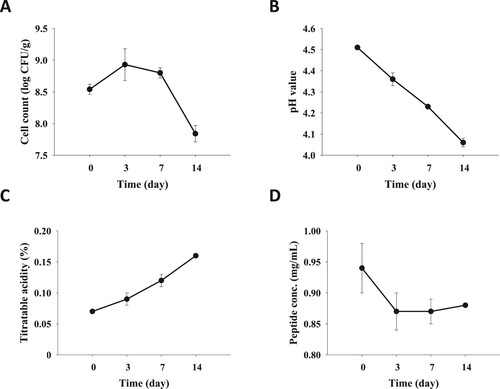
Daily supplement of FOM modulated antigen-specific T cell responses
To understand the impact of FOM on antigen-specific immune response, OVA-sensitized mice were daily treated with FOM, and the levels of serum antibody and splenic cytokine production of FOM-treated mice were measured and compared to that of commercial FCM-treated mice. Compared to that of normal mice, OVA sensitization elicited the production of OVA-specific IgG in serum. Neither FOM nor FCM treatment altered the level of OVA-specific IgG ((A)). Consistently, the spleen index was comparable between each group ((B)). Further, the splenocyte suspensions were prepared and incubated in the presence of OVA to understand the profile of antigen-specific T cell responses via investigating stimulation index and cytokine production. Compared to that of NA group, the stimulation index of VH group was significantly elevated. FOM treatment alleviated stimulation index in a dose-dependent manner, although FCM was most potent to reduce stimulation index ((C)). Since IL-2 is the key cytokine to induce clonal expansion, the concentration of IL-2 within the supernatants of cultured splenocytes was measured. In line with the results of stimulation index, OVA sensitization promoted IL-2 production, and FOM treatment attenuated IL-2 production in a dose-dependent manner ((D)).
Figure 3. Influence of fermented oat milk supplement on antigen-specific IgG production and antigen-induced splenocytes proliferation. Mice in the naïve (NA) group did not receive any treatment throughout the experiment. The other mice (six mice per group) were sensitized with ovalbumin twice with a two-week interval and daily treated with PBS (vehicle control; VH), fermented oat milk (0.4 or 2 mg/kg body weight; FOML or FOMH), or commercial cow milk yogurt (2 mg/kg body weight; FCM) by gavage through the experiment. Fourteen days after the second sensitization, the mice were sacrificed to individually collect the (A) serum samples for OVA-specific IgG determination and the spleen samples for (B) spleen index calculation. The prepared splenocytes were cultured in the presence of OVA for 24 h to determine the level of (C) stimulation index and (D) IL-2 production. Results are expressed as SEM of triplicate experiments. Different letters indicate statistical difference (p < .05) between each group.
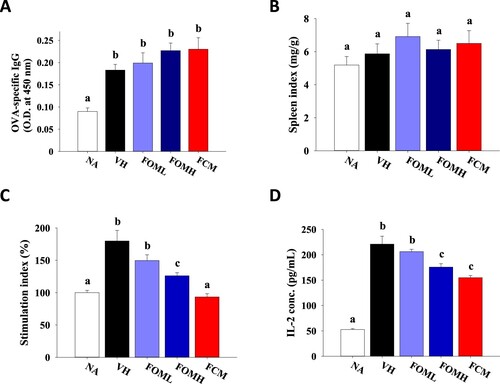
To understand the influence of FOM on modulating Th subsets activation, the level of relevant cytokines majorly secreted by Th1, Th2 and Treg cells was determined. Compared to that in the NA group, the concentration of IFN-γ and IL-4 in the VH group was markedly elevated ((A,B)). However, FOM treatment down-regulated the production of IFN-γ and IL-4, indicating the effect of FOM on suppressing both Th1 and Th2 activation. On the other hand, the level of TGF-β and IL-10, inhibitory cytokines primarily secreted from Treg cells, was up-regulated in FOM treatment groups compared to that in the VH group, revealing the potential of FOM on promoting the activity of Treg cells ((C,D)). Most important, the level of these cytokines was not significantly different between FOMH and FCM groups, suggesting comparable potency of FOM and FCM to modulate antigen-specific T cell responses ((D) and ).
Figure 4. Influence of fermented oat milk supplement on antigen-induced T cell-associated cytokine secretion from splenocytes. The mice (six mice per group) were treated as described in the “Materials and methods.” Fourteen days after the second sensitization, the mice were sacrificed to harvest the spleen samples. The prepared splenocytes were cultured in the presence of OVA for 24–72 h. The supernatants were collected to determine the levels of (A) IFN-γ, (B) IL-4, (C) TGF-β and (D) IL-10 production. Results are expressed as SEM of triplicate experiments. Different letters indicate statistical difference (p < .05) between each group.
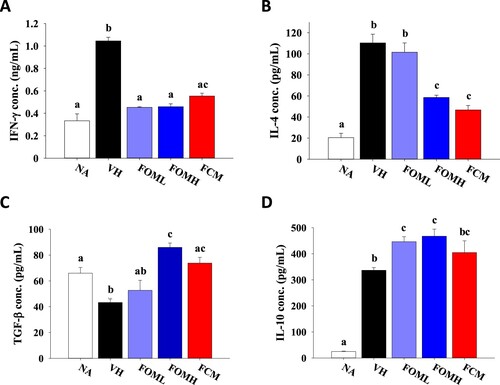
Discussion
In this study, the process of oat milk fermentation was established by employing traditional strains for dairy yogurt, and its storage stability was explored. Most important, we provided the first evidence to substantiate the modulatory effect of FOM on antigen-specific immune response. Daily treatment of FOM did not alter plasma level of OVA-specific IgG and spleen index in OVA-sensitized mice. However, stimulation index of splenocytes and splenic secretion of IL-2 was down-regulated. Furthermore, the potency of FOM and FCM was comparable to alleviate IFN-γ and IL-4 production and to elevate IL-10 and TGF-β secretion, revealing the potential of FOM to boost regulatory immune responses.
So far, few studies pertaining to the FOM or oat milk yogurt have been reported. Most of these published studies focused on physicochemical properties, texture and probiotic survivability of oat-based yogurt. For example, Raikos et al. fermented oat milk (12% w/w oats) with 5% (w/w) lactose and 0.25% (w/w) Yo-Mix® ABY yogurt culture (Bifidobacterium lactis, L. acidophilus, L. bulgaricus, L. lactis and S. thermophilus). 1.5% or 3% aquafaba and vegetable oil were added as ingredients and fermented at 43°C for 6 h. Both aquafaba and vegetable had a markedly impact on synaeresis, water holding capacity, hardness and the formation of the gel network structure of the yogurt (Raikos et al., Citation2020). Luca and Oroian evaluated the influence of synbiotic microcapsules on oat yogurt’s properties. Vegetable oat beverage was fermented with FD-DVS YC-350 YoFlex® (L. bulgaricus and S. thermophilus) and synbiotic microcapsules (12 log cfu/100 mL) at 45°C for 16 h. The addition of synbiotic microcapsules did not change the quality, texture or rheological parameters of the product, but increased the number of probiotics after storage (Luca & Oroian, Citation2022). In addition, oat protein concentrate has been used for fermentation with L. bulgaricus and S. thermophilus at 45°C for 24 h. The fermented product was mildly sour (pH 4.2) with 3.3 ± 0.2 g/kg of lactic acid and 4.9 × 106 cfu/g of Lactobacillus. Some of the proteolytic enzymes present in the yoghurt culture cleaved oat protein and released peptides (Brückner-Gühmann et al., Citation2019). With respect to the studies evaluating biological activity of FOM, dietary supplement of 35% symbiotic oat-based frozen yogurt, containing 4.1% protein, 8.5% fat, 2.1% fibre, and Bifidobacterium and L. acidophilus, improved hyperlipidaemia in rats fed with high cholesterol diet (Yang et al., Citation2019). Besides, limited information pertaining to the bioactivities and even immunomodulatory activities of FOM or oat milk yogurt is available.
Oat per se contains several nutrients, including dietary fibre, β-glucan, copper, iron, selenium, zinc, polyphenolics, proteins and peptides, with immunomodulatory activities (Chen, Mah, et al., Citation2021). After dehulling, the fibre content of oat groats is 10–12% with roughly 40% as soluble fibre, mainly β-glucan, and 60% as insoluble fibre (Menon et al., Citation2016). Modulation of dietary fibres on the immune system via reshaping gut microbiota and short-chain fatty acids (SCFAs) production has been proposed, although the underlying mechanism is not fully illustrated (Neuhouser et al., Citation2012; Yang et al., Citation2020). The influence of SCFA butyrate on suppressing pro-inflammatory cytokines and enhancing regulatory cytokines was observed in both human and animal studies (Iddir et al., Citation2020; Liu et al., Citation2018). Oat β-glucan is also a well-known stimulator for the innate immune system (Pan et al., Citation2020). Moreover, mineral ingredients, including the metal ions iron, zinc and copper, are recognized crucial nutrients for maintaining normal function of immune system (Chen, Mah, et al., Citation2021). It has been reported that ferulic acid, the preponderant polyphenol in oats, improved survival rate and mitigated weight loss in mice infected with influenza virus by activating TLR-7 and TLR-9, and increasing IFN production (Zhu et al., Citation2019). Proteins are known crucial for the production of immune cells and antibodies. Furthermore, previous studies have reported the impact of oat peptides on T cell proliferation and circulating dendritic cells from coeliac patients (Comino et al., Citation2011, Citation2016). However, the impact of above oat nutrients on antigen-specific immunity remains unclear.
The immunomodulatory activities of LAB strains for yogurt fermentation have been reported in numerous studies. For example, anti-inflammatory properties of S. thermophilus 285 were observed in peripheral blood mononuclear cells (Dargahi et al., Citation2021). Oral administration of S. thermophilus ME553 to C57BL/6 mice significantly promoted IFN-γ and IL-17 production, whereas L. bulgaricus ME552 only elevated IL-17 production. These two strains exerted distinguished extent to elicit IFN-γ production (Kamiya et al., Citation2016). Mice fed with live LAB (L. bulgaricus and S. thermophilus)-containing yogurt for 7 and 14 days had a higher percentage of B cells than that fed with cow milk (Meydani & Ha, Citation2000). In a similar experiment, intestinal lymphocytes from mice fed with live LAB-containing yogurt had a higher proliferative response to ConA and LPS (Puri et al., Citation1996). Production of IFN-γ from splenocytes of mice fed with live LAB (L. bulgaricus and S. thermophilus) was up-regulated (Muscettola et al., Citation1994). De Simone et al. reported that human lymphocytes cultured with L. bulgaricus and S. thermophilus produced more IFN-γ when stimulated with ConA (De Simone et al., Citation1986). In human study, increased production of IFN-γ from T cells was observed in adults consuming yogurt containing live L. bulgaricus and S. thermophilus (Halpern et al., Citation1991). Makino et al. demonstrated that extracellular polysaccharides produced by L. bulgaricus OLL1073R-1 could enhance IFN-γ production from murine splenocytes and augmented NK cell activity following oral administration (Makino et al., Citation2006). Elderly volunteers received at least 9 × 107 L. bulgaricus 8481 per day for six months, and an increase in the percentage of T cells and NK cells was observed. In the other research, L. rhamnosus GG was more potent in inducing maturation of dendritic cells, and L. bulgaricus provoked a higher secretion of pro-inflammatory cytokines as well as IL-10 (Elmadfa et al., Citation2010). Orally administration of L. rhamnosus CRL1505 to mice increased the levels of IFN-γ, IL-10 and IL-6 in the respiratory tract and the number of lung CD3+CD4+IFN-γ+ T cells (Villena et al., Citation2012). Co-culture of mononuclear cells with L. rhamnosus GG-derived soluble factors increased Th1 cytokine profile (Fong et al., Citation2016; Ludwig et al., Citation2018). Immunosuppressive mice, L. rhamnosus 1.0320 treatment enhanced the cellular immune function and humoral immune function and up-regulated IL-6, IL-10, IL-12, TNF-α and IgG levels in serum (Gao et al., Citation2021). Administration with L. rhamnosus (JB-1) to mice increased the population of CD4 + CD25 + Foxp3+ Treg cells with decreased TNF and IFN-γ production in mesenteric lymph nodes (Karimi et al., Citation2012). Treatment of L. rhamnosus GG (ATCC 53103) enhanced Th1 cellular immunity, but rather than humoral immunity, in a human gut microbiota transplanted porcine model (Wen et al., Citation2014). Moreover, this strain also increased the number of intestinal CD3+CD4+ T cells in weaned piglets (Shonyela et al., Citation2020). So far, the impact of L. rhamnosus BCRC 10940, which was employed in this study, on immune responses has not been reported. Based on the results of above studies, the immunomodulatory effect of S. thermophilus, L. bulgaricus and L. rhamnosus is strain-specific, and limited studies focused on the influence of these strains on antigen-specific immunity. In addition, the reported effects of oat ingredients and LAB for yogurt fermentation on immune responses are inconsistent. Therefore, further investigation will be required to elucidate structure–activity association of oat ingredients and LAB metabolites in the FOM for inducing regulatory immune responses.
Conclusion
The process of oat milk fermentation and storage stability of FOM are provided in this study. The results of in vivo experiments substantiate the modulatory effect of FOM on antigen-specific T cell responses, and the potency of FOM and commercial FCM was comparable, suggesting FOM is potential to be developed as vegan alternatives to animal products for treating immune disorders.
Disclosure statement
No potential conflict of interest was reported by the author(s).
Data availability statement
The datasets used and/or analysed during the current study are available from the corresponding author on request.
Additional information
Funding
References
- Barker, T. (2019). Applied immunology and biochemistry. Scientific e-Resources.
- Boyle, R., & Tang, M. (2006). The role of probiotics in the management of allergic disease. Clinical & Experimental Allergy, 36(5), 568–576. https://doi.org/10.1111/j.1365-2222.2006.02472.x
- Brückner-Gühmann, M., Vasil’eva, E., Culetu, A., Duta, D., Sozer, N., & Drusch, S. (2019). Oat protein concentrate as alternative ingredient for non-dairy yoghurt-type product. Journal of the Science of Food and Agriculture, 99(13), 5852–5857. https://doi.org/10.1002/jsfa.9858
- Chandan, R. C. (2006). History and consumption trends. Manufacturing Yogurt and Fermented Milks, 1(3.21), 5400. https://doi.org/10.1002/9780470277812
- Chen, G.-W., Tsai, J.-S., & Pan, B. S. (2007). Purification of angiotensin I-converting enzyme inhibitory peptides and antihypertensive effect of milk produced by protease-facilitated lactic fermentation. International Dairy Journal, 17(6), 641–647. https://doi.org/10.1016/j.idairyj.2006.07.004
- Chen, H.-Y., Hsieh, C.-W., Chen, P.-C., Lin, S.-P., Lin, Y.-F., & Cheng, K.-C. (2021). Development and optimization of djulis sourdough bread fermented by lactic acid bacteria for antioxidant capacity. Molecules, 26(18), 5658. https://doi.org/10.3390/molecules26185658
- Chen, O., Mah, E., Dioum, E., Marwaha, A., Shanmugam, S., Malleshi, N., Sudha, V., Gayathri, R., Unnikrishnan, R., Anjana, R. M., & Krishnaswamy, K. (2021). The role of oat nutrients in the immune system: A narrative review. Nutrients, 13(4), 1048. https://doi.org/10.3390/nu13041048
- Coda, R., Lanera, A., Trani, A., Gobbetti, M., & Di Cagno, R. (2012). Yogurt-like beverages made of a mixture of cereals, soy and grape must: Microbiology, texture, nutritional and sensory properties. International Journal of Food Microbiology, 155(3), 120–127. https://doi.org/10.1016/j.ijfoodmicro.2012.01.016
- Comino, I., Bernardo, D., Bancel, E., Moreno, M. D., Sánchez, B., Barro, F., Šuligoj, T., Ciclitira, P. J., Cebolla, Á, Knight, S. C., & Branlard, G. (2016). Identification and molecular characterization of oat peptides implicated on coeliac immune response. Food & Nutrition Research, 60(1), 30324. https://doi.org/10.3402/fnr.v60.30324
- Comino, I., Real, A., de Lorenzo, L., Cornell, H., López-Casado, MÁ, Barro, F., Lorite, P., Torres, M. I., Cebolla, Á, & Sousa, C. (2011). Diversity in oat potential immunogenicity: Basis for the selection of oat varieties with no toxicity in coeliac disease. Gut, 60(7), 915–922. https://doi.org/10.1136/gut.2010.225268
- Corbin, E., Vicente, J., Martin-Hernando, M., Acevedo, P., Perez-Rodriguez, L., & Gortazar, C. (2008). Spleen mass as a measure of immune strength in mammals. Mammal Review, 38(1), 108–115. https://doi.org/10.1111/j.1365-2907.2007.00112.x
- Dargahi, N., Johnson, J. C., & Apostolopoulos, V. (2021). Immune modulatory effects of probiotic Streptococcus thermophilus on human monocytes. Biologics, 1(3), 396–415. https://doi.org/10.3390/biologics1030023
- De Simone, C., Salvadori, B. B., Negri, R., Ferrazzi, M., Baldinelli, L., & Vesely, R. (1986). The adjuvant effect of yogurt on production of gamma-interferon by con A-stimulated human peripheral blood lymphocytes. Nutrition Reports International (USA).
- Dimitrijevic, R., Ivanovic, N., Mathiesen, G., Petrusic, V., Zivkovic, I., Djordjevic, B., & Dimitrijevic, L. (2014). Effects of Lactobacillus rhamnosus LA68 on the immune system of C57BL/6 mice upon oral administration. Journal of Dairy Research, 81(2), 202–207. https://doi.org/10.1017/S0022029914000028
- Elmadfa, I., Klein, P., & Meyer, A. L. (2010). Immune-stimulating effects of lactic acid bacteria in vivo and in vitro. Proceedings of the Nutrition Society, 69(3), 416–420. https://doi.org/10.1017/S0029665110001710
- Fong, F. L. Y., Kirjavainen, P. V., & El-Nezami, H. (2016). Immunomodulation of Lactobacillus rhamnosus GG (LGG)-derived soluble factors on antigen-presenting cells of healthy blood donors. Scientific Reports, 6(1), 1–8. https://doi.org/10.1038/s41598-016-0001-8
- Gao, D., Liu, Z., Liu, F., Chen, L., Wang, W., Ma, J., Xu, C., Jiang, Z., & Hou, J. (2021). Study of the immunoregulatory effect of Lactobacillus rhamnosus 1.0320 in immunosuppressed mice. Journal of Functional Foods, 79(12), 104423. https://doi.org/10.1016/j.jff.2021.104423
- Halpern, G., Vruwink, K., Van de Water, J. A., Keen, C. L., & Gershwin, M. E. (1991). Influence of long-term yoghurt consumption in young adults. International Journal of Immunotherapy, 7(4), 205–210.
- Iddir, M., Brito, A., Dingeo, G., Fernandez Del Campo, S. S., Samouda, H., La Frano, M. R., & Bohn, T. (2020). Strengthening the immune system and reducing inflammation and oxidative stress through diet and nutrition: Considerations during the COVID-19 crisis. Nutrients, 12(6), 1562. https://doi.org/10.3390/nu12061562
- Jäger, A., & Kuchroo, V. K. (2010). Effector and regulatory T-cell subsets in autoimmunity and tissue inflammation. Scandinavian Journal of Immunology, 72(3), 173–184. https://doi.org/10.1111/j.1365-3083.2010.02432.x
- Kamiya, T., Watanabe, Y., Makino, S., Kano, H., & Tsuji, N. M. (2016). Improvement of intestinal immune cell function by lactic acid bacteria for dairy products. Microorganisms, 5(1), 1. https://doi.org/10.3390/microorganisms5010001
- Karimi, K., Kandiah, N., Chau, J., Bienenstock, J., & Forsythe, P. (2012). A Lactobacillus rhamnosus strain induces a heme oxygenase dependent increase in Foxp3+ regulatory T cells.
- Liu, H., Wang, J., He, T., Becker, S., Zhang, G., Li, D., & Ma, X. (2018). Butyrate: A double-edged sword for health? Advances in Nutrition, 9(1), 21–29. https://doi.org/10.1093/advances/nmx009
- Lowry, O. H. (1951). Protein measurement with the Folin phenol reagent. Journal of Biological Chemistry, 193(1), 265–275. https://doi.org/10.1016/S0021-9258(19)52451-6
- Luca, L., & Oroian, M. (2022). Oat Yogurts enriched with synbiotic microcapsules: Physicochemical, microbiological, textural and rheological properties during storage. Foods, 11(7), 940. https://doi.org/10.3390/foods11070940
- Ludwig, I. S., Broere, F., Manurung, S., Lambers, T. T., Van der Zee, R., & Van Eden, W. (2018). Lactobacillus rhamnosus GG-derived soluble mediators modulate adaptive immune cells. Frontiers in Immunology, 9, 1546. https://doi.org/10.3389/fimmu.2018.01546
- Makino, S., Ikegami, S., Kano, H., Sashihara, T., Sugano, H., Horiuchi, H., Saito, T., & Oda, M. (2006). Immunomodulatory effects of polysaccharides produced by Lactobacillus delbrueckii ssp. bulgaricus OLL1073R-1. Journal of Dairy Science, 89(8), 2873–2881. https://doi.org/10.3168/jds.S0022-0302(06)72560-7
- Menon, R., Gonzalez, T., Ferruzzi, M., Jackson, E., Winderl, D., & Watson, J. (2016). Oats – from farm to fork. Advances in Food and Nutrition Research, 77, 1–55. https://doi.org/10.1016/bs.afnr.2015.12.001
- Meydani, S. N., & Ha, W.-K. (2000). Immunologic effects of yogurt. The American Journal of Clinical Nutrition, 71(4), 861–872. https://doi.org/10.1093/ajcn/71.4.861
- Mindus, C., van Staaveren, N., Fuchs, D., Gostner, J. M., Kjaer, J. B., Kunze, W., Mian, M. F., Shoveller, A. K., Forsythe, P., & Harlander-Matauschek, A. (2021). L. rhamnosus improves the immune response and tryptophan catabolism in laying hen pullets. Scientific Reports, 11(1), 1–15. https://doi.org/10.1038/s41598-020-79139-8
- Muscettola, M., Massai, L., Tanganelli, C., & Grasso, G. (1994). Effects of Lactobacilli on interferon production in young and aged mice a. Annals of the New York Academy of Sciences, 717(1), 226–232. https://doi.org/10.1111/j.1749-6632.1994.tb12092.x
- Nazimek, K., & Bryniarski, K. (2020). Approaches to inducing antigen-specific immune tolerance in allergy and autoimmunity: Focus on antigen-presenting cells and extracellular vesicles. Scandinavian Journal of Immunology, 91(6), e12881. https://doi.org/10.1111/sji.12881
- Neuhouser, M. L., Schwarz, Y., Wang, C., Breymeyer, K., Coronado, G., Wang, C. Y., Noar, K., Song, X., & Lampe, J. W. (2012). A low-glycemic load diet reduces serum C-reactive protein and modestly increases adiponectin in overweight and obese adults. The Journal of Nutrition, 142(2), 369–374. https://doi.org/10.3945/jn.111.149807
- O’Connor, C. (1995). Rural dairy technology (Vol. 1). ILRI (aka ILCA and ILRAD).
- Pan, W., Hao, S., Zheng, M., Lin, D., Jiang, P., Zhao, J., Shi, H., Yang, X., Li, X., & Yu, Y. (2020). Oat-derived β-glucans induced trained immunity through metabolic reprogramming. Inflammation, 43(4), 1323–1336. https://doi.org/10.1007/s10753-020-01211-2
- Puri, P., Rattan, A., Bijlani, R., Mahapatra, S., & Nath, I. (1996). Splenic and intestinal lymphocyte proliferation response in mice fed milk or yogurt and challenged with Salmonella typhimurium. International Journal of Food Sciences and Nutrition, 47(5), 391–398. https://doi.org/10.3109/09637489609006952
- Raikos, V., Juskaite, L., Vas, F., & Hayes, H. E. (2020). Physicochemical properties, texture, and probiotic survivability of oat-based yogurt using aquafaba as a gelling agent. Food Science & Nutrition, 8(12), 6426–6432. https://doi.org/10.1002/fsn3.1932
- Ranadheera, C. S., Vidanarachchi, J. K., Rocha, R. S., Cruz, A. G., & Ajlouni, S. (2017). Probiotic delivery through fermentation: Dairy vs. non-dairy beverages. Fermentation, 3(4), 67. https://doi.org/10.3390/fermentation3040067
- Shamji, M. H., Valenta, R., Jardetzky, T., Verhasselt, V., Durham, S. R., Würtzen, P. A., & van Neerven, R. J. (2021). The role of allergen-specific IgE, IgG and IgA in allergic disease. Allergy, 76(12), 3627–3641. https://doi.org/10.1111/all.14908
- Shonyela, S. M., Feng, B., Yang, W., Yang, G., & Wang, C. (2020). The regulatory effect of Lactobacillus rhamnosus GG on T lymphocyte and the development of intestinal villi in piglets of different periods. Amb Express, 10(1), 1–11. https://doi.org/10.1186/s13568-020-00980-1
- Silanikove, N., Leitner, G., & Merin, U. (2015). The interrelationships between lactose intolerance and the modern dairy industry: Global perspectives in evolutional and historical backgrounds. Nutrients, 7(9), 7312–7331. https://doi.org/10.3390/nu7095340
- Terpou, A., Papadaki, A., Lappa, I. K., Kachrimanidou, V., Bosnea, L. A., & Kopsahelis, N. (2019). Probiotics in food systems: Significance and emerging strategies towards improved viability and delivery of enhanced beneficial value. Nutrients, 11(7), 1591. https://doi.org/10.3390/nu11071591
- Villena, J., Chiba, E., Tomosada, Y., Salva, S., Marranzino, G., Kitazawa, H., & Alvarez, S. (2012). Orally administered Lactobacillus rhamnosus modulates the respiratory immune response triggered by the viral pathogen-associated molecular pattern poly (I: C). BMC Immunology, 13(1), 1–15. https://doi.org/10.1186/1471-2172-13-53
- Wei, Y. J., Fang, R. E., Ou, J. Y., Pan, C. L., & Huang, C. H. (2022). Modulatory effects of Porphyra-derived polysaccharides, oligosaccharides and their mixture on antigen-specific immune responses in ovalbumin-sensitized mice. Journal of Functional Foods, 96(1-2), 105209. https://doi.org/10.1016/j.jff.2022.105209
- Wen, K., Tin, C., Wang, H., Yang, X., Li, G., Giri-Rachman, E., Kocher, J., Bui, T., Clark-Deener, S., & Yuan, L. (2014). Probiotic Lactobacillus rhamnosus GG enhanced Th1 cellular immunity but did not affect antibody responses in a human gut microbiota transplanted neonatal gnotobiotic pig model. PLoS One, 9(4), e94504. https://doi.org/10.1371/journal.pone.0094504
- Yang, H., Sun, Y., Cai, R., Chen, Y., & Gu, B. (2020). The impact of dietary fiber and probiotics in infectious diseases. Microbial Pathogenesis, 140(8), 103931. https://doi.org/10.1016/j.micpath.2019.103931
- Yang, R., Wang, C., Ye, H., Gao, F., Cheng, J., Zhang, T., & Guo, M. (2019). Effects of feeding hyperlipidemia rats with symbiotic oat-based frozen yogurt on serum triglycerides and cholesterol. Food Science & Nutrition, 7(3), 1096–1103. https://doi.org/10.1002/fsn3.949
- Zhu, Y., Shao, Y., Qu, X., Guo, J., Yang, J., Zhou, Z., & Wang, S. (2019). Sodium ferulate protects against influenza virus infection by activation of the TLR7/9-MyD88-IRF7 signaling pathway and inhibition of the NF-κB signaling pathway. Biochemical and Biophysical Research Communications, 512(4), 793–798. https://doi.org/10.1016/j.bbrc.2019.03.113