ABSTRACT
Effects of dietary concentrate level and grass hay source were determined to evaluate a method (www2.luresext.edu/goats/research/suppconc.html) of predicting negative associative effects between feedstuffs in Boer goat wethers. Forage DM intake (g/kg BW0.75) was similar (P = .157) between growing and yearling wethers (34.9 and 30.8) and ranked 0 and 15 > 30 > 45 g/kg BW0.75 of concentrate dry matter (48.5, 41.8, 25.9, and 15.2, respectively). Age and concentrate level interacted in neutral detergent fibre digestibility (57.3%, 60.6%, 61.4%, and 58.4% for growing and 56.6, 62.9, 56.8, and 30.0% for yearling wethers with 0, 15, 30, and 45 g/kg BW0.75, respectively). There was a tendency (P = .074) for an interaction in metabolizable energy (ME) intake between age and concentrate level (361, 530, 634, and 709 for growing and 363, 547, 541, and 555 kJ/kg BW0.75 for yearling wethers with 0, 15, 30, and 45 g/kg BW0.75); values predicted for treatments with concentrate were 563, 631, and 619 for growing and 575, 684, and 697 kJ/kg BW0.75 for yearling wethers with 15, 30, and 45 g/kg BW0.75, respectively. In conclusion, ME intake was accurately predicted for the low level of concentrate with both animal types and the moderate level with growing wethers.
1. Introduction
In most ruminant production systems, reproducing females acquire the majority of nutrients and energy from grazed or harvested forage (Minson Citation1990). This is also generally true for weaned animals, although there are settings where diets high in concentrate are fed, such as finishing feedlot cattle in the USA (Galyean & Goetsch Citation1993). For forage-based diets, however, in many cases dietary inclusion of concentrate feedstuffs is necessary to achieve the desired level of performance, with the amount and composition of the supplement depending on characteristics of the basal forage and target animal (Goetsch & Gipson Citation2014). Because of the different types of microorganisms in the rumen and effects on the quantity and array of nutrients absorbed in relation to tissue needs, concentrate inclusion can impact intake and(or) digestion of basal dietary forage (McCollum & Horn Citation1990). In order to maximize efficiency of utilization of forage and concentrate in mixed diets, there is need to accurately predict both negative and positive associative effects between different types of feedstuffs (Sahlu et al. Citation2009).
A web-based nutrient requirement calculation system was developed at Langston University (Langston University Interactive Nutrient Calcultors, LINC; www2.luresext.edu/goats/research/nutreqgoats.html) to apply NRC (Citation2007) metabolizable energy (ME) and protein requirements of goats, as described by Goetsch and Gipson (Citation2014). There are calculations to determine the quantity and composition of a supplement needed to exactly meet the energy and protein needs recommended by NRC (Citation2007) for specific animal and basal forage conditions. A method of predicting feedstuff associative effects in goats is included so that projections of ME intake with mixed concentrate-forage diets are more accurate than otherwise. Though it is known that associative effects result from potential change in both intake and digestion of basal forage, the decreases in forage intake (i.e. substitution) predicted by LINC are intended to encompass the sum effect of decreases in forage intake and(or) digestion so that total ME intake is predicted accurately.
The LINC equation for predicting negative associative effects is:where SUBN is the decrease in forage intake expressed as a fraction of supplement intake, DMIBWS is the supplement intake as % body weight [BW; dry matter (DM) basis], DMIBWS2 is the DMISBW squared, DMIBWS3 is the DMISBW cubed, and DIGF is the digestibility of forage [i.e. organic matter (OM) digestibility or total digestible nutrient concentration as a percentage]. provides examples of predictions with this equation. Values shown are for average BW of growing and yearling goat wethers in the present experiment. also displays that negative associative effects are assumed absent when supplement DM intake is less than 0.5% BW, and that predicted decreases in basal forage intake as a percentage of supplement intake increase with increasing (1) level of supplementation and (2) digestibility of basal forage consumed without supplementation. Conversely, prediction of positive associative effects, which is not addressed in this study, occurs with supplement intake less than 0.5% BW, forage less than 6% crude protein (CP), and supplement at least 15% CP. Similarly, projected increases in forage intake are designed to encompass effects of potential increases in both basal forage intake and digestion.
Table 1. Predictions of decreases in basal forage intake by growing and yearling Boer goat wethers to account for effects of supplemental concentrate on intake of ME resulting from potential decreases in forage intake and digestion with the web-based goat nutrient requirement calculation system for goats of Langston University (LINC).
The LINC system for predicting negative feedstuff associative effects in goats has not been evaluated for accuracy or need for modification or development of other methods. Factors of particular relevance are basal forage composition, level of supplementation, and animal characteristics that impact nutrient demand. Therefore, objectives of this experiment were to evaluate effects of different levels of supplemental concentrate and forage sources on intake, digestion, and behaviour of growing and yearling Boer goat wethers and compare findings with predictions of the LINC system.
2. Materials and methods
2.1. Animals and preliminary period
The experimental protocol was approved by the Langston University Animal Care and Use Committee. Twelve growing Boer goat wethers (initial age of 188 ± 2.6 days and BW of 24.0 ± 0.48 kg) and 12 yearling Boer goat wethers (573 ± 3.2 days and 46.7 ± 1.03 kg) were used. There was a 3-wk period for adaptation to individual housing in 1.05 × 0.55 m elevated pens with a plastic-coated expanded metal floor, with free-choice feeding of a medium quality grass hay (source B) coarsely ground to pass a 3.8-cm screen. In addition, a supplement of 80% ground corn, 19% soybean meal, and 1% ammonium chloride was offered at 30 g/kg BW0.75 (DM basis) determined at the beginning of the period. Hay refusals were collected before 07:00 h and equal quantities of feedstuffs were given at 07:00 h and 15:00 h, with hay given after all supplement was consumed. Hay was offered for refusals of approximately 20% of that offered.
A small amount (8–10 g/day; as fed basis) of a mixture of 95% soybean meal and 5% trace mineral premix (275 mg/kg Co, 2000 mg/kg I, 43,746 mg/kg Fe, 750 mg/kg Se, 18,748 mg/kg Cu, 68,744 mg/kg Zn, and 19,998 mg/kg Mn) was top-dressed on hay. Also, each wether had free access to a small trace mineralized salt block (NaCl: 96.5–99.5%; Zn: 4000 mg/kg; Fe: 1600 mg/kg; Mn: 1200 mg/kg; Cu: 260–390 mg/kg; I: 100 mg/kg; and Co: 40 mg/kg) in the bottom of each feeder.
Wethers were vaccinated against clostridial organisms with Covexin 8® (Schering-Plough Animal Health Corp., Omaha, NE, USA) and orally treated for internal parasites with 12 mg/kg of levamisole (Prohibit®, AgriLabs, St. Joseph, MO, USA), 0.5 mg/kg of moxidectin (Cydectin®; Boehringer Ingelheim Vetmedica, Inc., St. Joseph, MO, USA), and 10 mg/kg of albendazole (Valbazen®; Pfizer Animal Health, New York, NY, USA). Albendazole treatment was repeated 24 h later.
2.2. Treatments and measures
The experimental design was a split-plot with eight simultaneous 3 × 3 Latin squares. The treatment design was a 2 × 4 × 3 factorial, with main plots of animal age (growing vs. yearling wethers) and level of supplementation with the same concentrate mixture given previously (0, 15, 30, and 45 g/kg BW0.75; DM basis) and a subplot of forage source (three sources of grass hay, referred to as A, B, and C). The wide range in level of concentrate was to achieve large differences in predicted associative effects to address robustness. Relatedly, supplementation was based on BW0.75 in order to achieve a higher level for both animal types than possible if based on BW1.0. Furthermore, although few current caprine production systems entail use of very high concentrate diets, converse to beef cattle and sheep in some areas of the world, it is conceivable that future prevalence will increase in some locations.
Four of the Latin squares were with growing wethers and four were with yearlings. One square (three wethers) of each animal age was assigned to the same level of concentrate in each of the three 3-wk periods, with different forage sources fed in the periods for ad libitum intake. Much longer periods would have been necessitated if animals within squares were switched to different concentrate levels from period to period. Samples of these and other available grass hay sources were subjected to preliminary chemical analyses for selection of ones intended to differ appreciably in quality. Based on visual appearance before harvest, the hay sources chosen consisted primarily of different quantities of johnsongrass (Sorghum halepense) and bermudagrass (Cynodon dactylon).
One-half of the wethers of each age, with one or two from each square, were allocated to one animal set and others were assigned to a second set that started 1 wk later than the first so that samples were collected and measurements made simultaneously on 12 animals. On day 10, ruminal fluid was sampled by stomach tube 4 h after the morning meal. Only one ruminal fluid sample was collected to avoid potential negative effects on forage intake, although it is realized that one sample is not adequate to characterize conditions throughout the day. The pH was measured with a digital meter and then 4 ml were placed into a tube with 1 ml of a 25% (wt/vol) metaphosphoric acid solution and frozen at −20° C for later volatile fatty acid (VFA) analysis. Likewise, 3 ml were placed into a tube with 2 ml of 3 M HCl and frozen at −20°C for ammonia analysis. Analyses of VFA and ammonia N were by procedures of Lu et al. (Citation1990) and Broderick and Kang (Citation1980), respectively.
Behaviour of 12 wethers (6 per animal type) per day was monitored on days 13 and 14 by CCTV cameras (VC-C50i; Canon U.S.A., Inc., Lake Success, NY, USA) with use of infrared lighting. Recordings (mpeg) were coded using the Observer XT Version 10.5 (Noldus Information Technology, Wageningen, The Netherlands). Two behavioural states were defined as Feeding, which consisted of three mutually exclusive behaviour events of eating, ruminating, or not eating or ruminating (i.e. idle), and Position that entailed two mutually exclusive behaviour events of lying or standing. The Observer XT automatically calculated event duration in seconds for every behavioural state and the observation files were exported as csv files. Because some of the video files were slightly less than 24 h, total event duration was expressed as a percentage.
Wethers were placed in metabolism crates (0.7 × 1.2 m; plastic-coated expanded metal floor) on days 17–21 of each period for total collection of faeces and urine. Urine was acidified with 20 ml of 20% (vol/vol) H2SO4 placed in each collection vessel to maintain pH below 3.0. Composite samples of faeces and urine were formed by collecting 10% daily aliquots. Feed and refusal samples were collected daily in wk 3 to form composite samples. Samples were analysed for DM (ID 967.03), ash (942.05), Kjeldahl N (976.096; AOAC Citation2006), neutral detergent fibre (NDF) with use of heat stable amylase and containing residual ash, acid detergent fibre (ADF) (ID 973.18), and acid detergent lignin (ADL; filter bag technique of ANKOM Technology Corp., Fairport, NY, USA). Samples were also analysed for gross energy using a bomb calorimeter (Parr 6300; Parr Instrument Co., Inc., Moline, IL, USA).
Values for individual animals receiving concentrate were coupled with means of observations without concentrate to estimate effects of supplementation on measures such as basal forage NDF digestion and ME intake and to evaluate predictions of the LINC system. For the former, 65.6% digestibility of concentrate NDF was assumed. This value was based on fermentable fibre levels of 66.7% and 64.3% in corn and soybean meal (Thonney & Hogue Citation2013). However, it should be noted that higher values, such as 72.9% and 78.0% for corn and soybean meal, respectively, have been reported (Nocek & Russell Citation1988). Digested concentrate NDF was subtracted from total digested NDF to predict digested forage NDF. Intake of ME was estimated from digestible OM intake, digestible energy (DE) of 19.33 kJ/kg of digested OM (NRC Citation1981), and ME as 82% of DE (Garrett et al. Citation1959). As noted earlier, ME rather than digested OM or DE was addressed because of NRC (Citation2007) recommendations for ME, although it is realized that the same metabolizability is assumed regardless of dietary treatment.
2.3. Statistical analyses
The composition of hay was analysed with the GLM procedure of SAS (Citation2008). Other data were analysed with a mixed effects model consisting of animal age, supplement level, forage source, and their interactions (SAS Citation2008). The random effect was animal within age × supplement level, with a repeated measure of period and subject of animal within age × supplement level. The model for variables with one value per period was:where Yijklm is the dependent variable, µ the overall mean, Ai the fixed effect of animal age, Sj the fixed effect of level of supplementation, ASij the fixed effect of the interaction between Ai and Sj, Pk the fixed effect of period, Fl the fixed effect of forage source, AFil the fixed effect of the interaction between Ai and Fl, SFjl the fixed effect of the interaction between Sj and Fl, ASFijl the fixed effect of the three-way interaction of Ai, Sj, and Fl, ANm(ij) the random effect of animal within Ai × Sij, and eijklm residual error. Animal within age × supplementation level (16 degrees of freedom, df) was the error term to test effects of Ai (1 df), Sj (1 df), and ASij (1 df), and residual error (eijklm; 30 df) was the error for testing other sources of variation (2, 2, 6, and 6 df for AFil, SFjl, ASFijl, and ANm(ij), respectively). Moreover, for analysis of findings with concentrate inclusion in the diet based on mean results without concentrate, data form the six Latin squares with concentrate were analysed similarly. The covariance structure assumed was variance components.
Means were separated by least significant difference when overall treatment effects were significant (P < .05). For the level of supplementation, separation by orthogonal contrasts was considered but not found preferable in terms of interpretation clarity. The P values for effects are listed in tables and tendencies (i.e. P > .05 and P < .10) are also presented in the text. Means are presented in accordance with the significance of effects. That is, interaction means are listed when an interaction was significant. Main effect means are included when the factor was not involved in an interaction regardless of significance of the main effect.
For behavioural variables, mean event duration (%) for each hour was analysed with a mixed effects model consisting of animal age, supplement level, forage source, hour, and their interactions. Random effects were animal within age × supplement level and within age × supplement level × forage source. The repeated measure was period × hour with the subject of animal within age × supplement level.
3. Results
3.1. Feedstuff composition
The level of CP in the A forage source () was lower than expected based on the pre-trial analyses of samples collected from large round bales. However, inclusion of soybean meal in the concentrate should have averted associative effects resulting from the creation or accentuation of a deficiency of ruminally available nitrogenous compounds. Concentrations of NDF in samples during the experiment were quite similar for B and C (P > .10). But, the ADL concentration 3% greater for C vs. B (P < .05) implies lower ruminally availability of fibre for C.
Table 2. Composition of feedstuffs consumed by growing and yearling Boer goat wethers.
3.2. Intake and digestion
Hay and total DM intake in g/kg BW0.75 were similar (P = .157 and .165, respectively) for growing and yearling wethers, and intakes of hay and total DM in g/kg BW0.75 and g/day were greatest among forage sources for B ( and ; P < .05). There was an interaction (P = .026) between animal age and supplement level in g/day intake of hay DM. In general, the 30 and 45 g/kg BW0.75 levels of supplementation, compared with 0 and 15 levels, decreased hay intake by yearling wethers much more than by growing wethers, although the magnitude of nonsignificant difference between 30 and 45 g/kg BW0.75 levels was similar for both ages. Total DM intake in g/kg BW0.75 was numerically (P = .103) lowest among supplement levels for 0, although there was a difference in g/day. Digestibility of DM was lower for B than for A and C forage sources. However, the magnitude of difference was less than in g/day of DM intake, resulting in maintenance of greatest digestible DM intake for B.
Table 3. P values for effects of animal age, supplement level, and forage source on intake and digestion by Boer goat wethers.
Table 4. Effects of animal age, supplement level, and forage source on intake and digestion by Boer goat wethers.
Results for OM were generally in accordance with those for DM ( and ). However, one difference was an interaction between animal age and level of supplementation in OM digestibility, which appeared primarily the result of a greater value for growing vs. yearling wethers with the 45 g/kg BW0.75 supplement level.
As alluded earlier, CP concentrations in total DM consumed of 14.4%, 12.9%, and 14.8% for A, B, and C forage sources suggest that there was no appreciable deficiency of ruminally available nitrogenous compounds required for microbial growth and unhindered fibre digestion, which is supported by the lack of interactions between forage source and supplement treatment ( and ). Total tract digestion of CP was fairly similar to that expected based on findings of Moore et al. (Citation2004) of true protein digestibility of 88% and metabolic faecal CP of 2.67% of DM intake, with predictions of 69.5%, 67.3%, and 70.0% for A, B, and C, respectively. Based on N balance means, it would appear that all wethers were decreasing in BW during the experiment, with lower N balance (greater N loss) for yearling vs. growing wethers and for C than for A and B forages. However, the relatively short length of periods, faecal and urine collections in the last week of 3-wk periods, and normal measurement errors should be considered when evaluating such findings.
The interaction in NDF intake between animal age and level of concentrate is in accordance with that in hay DM intake in g/day ( and ). The reason for the interaction between age and supplementation level in NDF digestibility was a much lower value for yearling wethers and the 45 g/kg BW0.75 level compared with that for growing wethers and all other age × supplementation level treatments. The interaction between forage source and supplementation level in NDF digestibility reflects varying susceptibilities of forage fibre to decreases resulting from different levels of concentrate. That is, there was a decline with the C source when concentrate was raised from 15 to 30 g/kg BW0.75, whereas decreases occurred with A and B when the feeding rate of concentrate was increased from 30 to 45 g/kg BW0.75. But, these values address the sum effect of digestibility of forage and concentrate. Nonetheless, the magnitude of change in total diet NDF digestibility in response to increasing level of concentrate was much greater for A (i.e. 29 percentage unit decrease from 30 to 45 g/kg BW0.75) than for B (11 percentage unit decrease with 30–45 g/kg BW0.75) and C (15 percentage unit decrease with 15–30 g/kg BW0.75). Treatment effects on intake and digestibility of ADF were similar to those for NDF.
3.3. Ruminal fluid
Ruminal fluid pH was similar between animal ages and among forage sources, but ranked 0 and 15 > 30 > 45 g/kg BW0.75 of concentrate ( and ). However, the mean for the concentrate level of 45 g/kg BW0.75 was above 6.0. There were no effects of animal age, forage source, or supplement level on the level of total VFA, but there were many interactions in molar percentages of individual VFA. The interaction between forage source and level of concentrate in the molar percentage of acetate was because of a smaller decline with increasing concentrate level for the B than for A or C forage sources, which corresponds to the interaction in the molar percentage of propionate and opposite magnitudes of change. The three-way interaction in the molar percentage of butyrate was somewhat similar in nature to the two-way interactions in acetate and propionate levels. However, for butyrate a relatively small increase in the molar percentage occurred as concentrate level increased with growing but not with yearling wethers.
Table 5. P values for effects of animal age, supplement level, and forage source on ruminal fluid characteristics of Boer goat wethers.
Table 6. Effects of animal age, supplement level, and forage source on ruminal fluid characteristics of Boer goat wethers.
The increase in ruminal fluid concentration of ammonia N with increasing level of concentrate was expected given the CP level in the concentrate, although the difference between the 30 and 45 g/kg BW0.75 levels was not significant ( and ). It is not possible to conclusively discern the reason for the greater ammonia N concentration for growing vs. yearling wethers.
3.4. Feeding behaviour
The proportion of time spent eating was affected by animal age, hour of the day, and age × hour ( and ; ). Also, the P value for the effect of level of concentrate was .053. Likewise, time spent ruminating was influenced by age, supplement level, hour, and a three-way interaction among supplement level, forage source, and hour (). The same factors influenced time spent idle, except there was only a tendency for a three-way interaction (P = .061). Time spent standing and lying varied with hour, supplement level × hour, and supplement level × forage source × hour. However, because it is not possible to clearly address these latter three-way interactions, these data are not shown in figures.
Figure 1. Effects of animal type on time spent eating throughout the day and the hourly pattern of time idle.
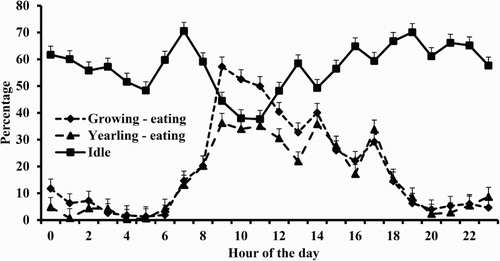
Figure 2. Effects of level of supplemental concentrate and forage source on time spent ruminating throughout the day. Panel A = A forage source, Panel B = B forage source, and Panel C = C forage source.
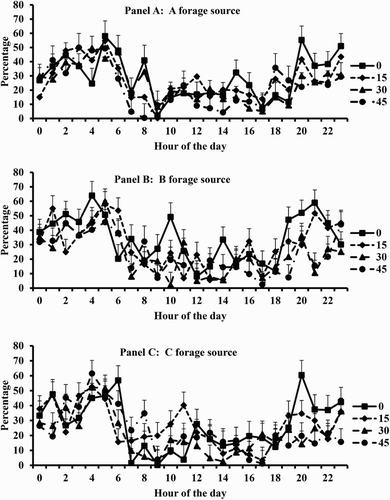
Table 7. P values for effects of animal age, supplement level, and forage source on feeding behaviour and position.
Table 8. Effects of animal age, supplement level, and forage source on feeding behaviour and position of Boer goat wethers.
Growing wethers spent 55 and 72 min longer eating and ruminating, respectively, than did yearling wethers ( and ). The difference in total eating time was primarily attributable to the 5-h period of 09:00–13:00 h (). There was only a tendency for a comparable animal age × hour interaction in time ruminating (P = .099), with a high P value for this interaction in time idle (P = .781). Thus, during that 5 -h period when growing wethers spent more time eating than did yearlings, they tended to ruminate less ().
The tendency for an effect of concentrate level on eating time involved trends for values approximately 5 percentage units lower for 30 and 45 vs. 0 and 15 g/kg BW0.75 ( and ). Similarly, time ruminating was less for 30 and 45 vs. 0 g/kg BW0.75, with an intermediate value for 15 g/kg BW0.75. In accordance, time idle averaged 11 percentage units greater for the two highest levels of concentrate than for the two lowest levels. There were no significant main effects or two-way interactions in feeding behaviour involving forage source. Therefore, the significant three-way interactions among supplement level, forage source, and hour observed were not anticipated.
4. Discussion
4.1. Feed intake
With greater potential for growth and nutrient utilization by growing than yearling wethers, a significant difference in forage intake may have been expected, at least with diets of moderate to high quality (Jarrige et al. Citation1986; Horn & McCollum Citation1987; NRC Citation2007). Intake on a g/kg BW0.75 basis of the B forage that was 44 and 66% greater than for A and C, respectively, was somewhat surprising given the relatively small differences in NDF concentration. However, the ADF concentration was 11 percentage units lower for B vs. A. The ADF concentration for B and C was fairly similar, but perhaps the substantial difference in the level of ADL relative to NDF, implying less cell wall lignification, was involved in the difference in forage intake (Jarrige et al. Citation1986; Van Soest Citation1994; Coleman et al. Citation2003).
Effects of concentrate inclusion in the diet on forage intake were generally as expected, with the magnitude increasing as level of concentrate rose (Jarrige et al. Citation1986; Horn & McCollum Citation1987; Paterson et al. Citation1994). Based on observations of animals consuming diets containing concentrate and averages of forage intake without concentrate, supplementation decreased forage intake by 7, 23, and 33 g/kg BW0.75 with 15, 30, and 45 g/kg BW0.75 (standard error of the mean; SEM = 2.6), equating to 44, 79, and 77% of concentrate intake, respectively. Predicted decreases in forage intake with the LINC system were 22, 60, and 96% of concentrate intake for growing wethers and 9, 39, and 78% for yearlings with concentrate at 15, 30, and 45 g/kg BW0.75, respectively. These values differ between animal ages because the quantity of supplement in this experiment was based on BW0.75 and BW1.0 is used in the LINC equation. It is also notable that the levels of supplement of 0.68% and 0.56% BW with the 15 g/kg BW0.75 level for growing and yearling wethers, respectively, were both above the LINC threshold of 0.5% BW, below which negative associative effects are presumed absent and positive effects are possible.
The effect of forage source on the decrease in forage intake in response to supplementation, calculated as noted above, was nearly significant (P = .063), with a numerical ranking of B > C > A (17, 23, and 22 g/kg BW0.75, respectively; SEM = 2.11), equivalent to 52%, 70%, and 78% of supplement intake for forage sources A, B, and C, respectively. Predictions with the LINC system were 50%, 39%, and 64% of supplement intake for forages A, B, and C, respectively. Predictions differ among forage sources because of inclusion of forage digestibility in the equation.
4.2. Digestion and ruminal characteristics
Lower OM digestion for forage source B vs. A and C sources disagrees with highest intake of B. Presumably ruminal digesta retention time was shortest for source B because of greatest intake, thus limiting extent of digestion (Galyean & Owens Citation1991; Van Soest Citation1994; Coleman et al. Citation2003). In accordance, there were considerable differences in rate of NDF intake, with values of 1.52, 1.80, and 1.26 g/min for A, B, and C, respectively (SEM = 0.141). These findings may reflect disparate and interacting influences of forage characteristics on the host of factors affecting intake and digestion that are difficult to predict (Teixeira et al. Citation2011).
There was an interaction between animal age and level of concentrate in digestibility of basal forage NDF based on values for individual animals consuming diets with concentrate and averages for the 0 level of concentrate. Values were 60.4%, 60.3%, and 55.4% for growing wethers and 62.5%, 51.8%, and 6.5% for yearlings with 15, 30, and 45 g/kg BW0.75 concentrate levels, respectively (SEM = 5.86). Hence, the low and moderate levels of concentrate did not negatively affect basal forage NDF digestion, and it appears that there were small positive effects of concentrate at 15 g/kg BW0.75 and the moderate level with growing wethers as noted in other instances (Mould et al. Citation1983; Uden Citation1984). However, the negative effect with yearling wethers of concentrate at 45 g/kg BW0.75 was substantial, and the very low value that was not different from 0 suggests that digestion of concentrate NDF also was depressed by ruminal conditions created by this high dietary level of concentrate.
Reasons why the highest level of concentrate decreased basal forage NDF digestion with the yearling but not growing wethers cannot be conclusively discerned. Nonetheless, though the animal age × supplement level interaction in g/kg BW0.75 intake of forage was not significant, there was an interaction in intake as g/day, with the 30 and 45 g/kg BW0.75 concentrate levels having much greater effect for yearling than growing wethers. Perhaps the lesser nutrient demand and capacity for utilization by yearling vs. growing wethers contributed to lower forage intake relative to BW1.0 (Horn & McCollum Citation1987; NRC Citation2007) and, thus, concentrate being a greater percentage of the total diet with the two highest levels (0%, 28.7%, 49.9%, and 71.5% for growing wethers and 0%, 28.7%, 59.2%, and 78.2% for yearling wethers with 0, 15, 30, and 45 g/kg BW0.75, respectively; SEM = 2.85). In accordance, there was a numerical interaction in ruminal pH between animal type and supplement level (P = .122), with lower values for yearling than for growing wethers for the two highest levels of concentrate (6.59, 6.53, 6.45, and 6.25 for growing wethers and 6.67, 6.51, 6.28, and 6.01 for yearling wethers with 0, 15, 30, and 45 g/kg BW0.75 of concentrate, respectively; SEM = 0.067). Although ruminal fluid was sampled only at one time, these values suggest that low pH could have contributed to limited NDF digestion in yearling wethers at least with concentrate at 45 g/kg BW0.75, with likely effects of preferential starch utilization by fibrolytic microorganisms in addition to low pH (Mertens & Loften Citation1980; Hoover Citation1986). Both of these animal age × supplementation level interaction means (i.e. percentage of concentrate in the diet and ruminal pH) agree with an interaction in the molar percentage of propionate, with a greater value for concentrate at 45 g/kg BW0.75 with yearling than growing wethers and a trend for a similar difference (P = .067) between ages with concentrate at 30 g/kg BW0.75.
4.3. Feeding behaviour
More time eating and ruminating for growing vs. yearling wethers probably relates to numerically higher DM intake by growing wethers relative to BW0.75 (Dulphy et al. Citation1980; Lechner-Doll et al. Citation1991; Coleman et al. Citation2003). Positive correlations between NDF intake and time spent eating (r = 0.34; P = .004) and ruminating (r = 0.32; P = .007) suggest that the tendency for differences in eating and rumination times between the two lowest and two highest levels of concentrate involved differences in fibre intake (Dulphy et al. Citation1980; Lechner-Doll et al. Citation1991; Coleman et al. Citation2003). However, rates of NDF intake of 1.90, 1.65, 1.48, and 1.09 g/min for supplement at 0, 15, 30, and 45 g/kg BW0.75, respectively (SEM = 0.194) and a correlation between time spent eating and NDF intake rate of –0.38 (P = .001) reflect an importance of other factors influencing eating time. Although eating time was not partitioned into that associated with concentrate and forage, based on casual observations of very rapid concentrate ingestion, it would seem that chewing during eating and rumination relative to hay and NDF intakes was considerably greater for concentrate levels of 30 and 45 vs. 0 and 15 g/kg BW0.75, which is supported by low rates of NDF intake.
4.4. Prediction of ME intake
As noted before, the LINC system for both negative and positive associative effects was designed to project changes in basal forage intake that will predict the sum effect on ME intake of changes in forage intake and digestibility. To evaluate accuracy of projections of negative associative effects in this experiment, actual and predicted means of observations with dietary inclusion of concentrate are presented in . Because of tendencies for interactions in actual ME intake between animal age and concentrate level for all observations and ones only with diets including concentrate (P = .074 and .060, respectively), the latter interaction means are included. Prediction was fairly accurate for both animal types with the low level of supplementation (33 and 28 kJ/kg BW0.75 differences for growing and yearling wethers, respectively), with concentrate averaging 29% of total DM intake for both animal types. Accurate prediction occurred despite an under-estimation of the decrease in basal forage intake relative to supplement intake, because of the compensatory slight improvement in forage digestion. Prediction also was accurate for the moderate concentrate level and growing wethers (3 kJ/kg BW0.75 difference), with concentrate at 50% of the diet. Conversely, there was under-prediction for growing wethers consuming diets with the highest level of concentrate (72% of DM intake; 90 kJ/kg BW0.75 difference) and considerable over-prediction for yearlings fed diets with both the moderate and high levels of concentrate (59 and 78% of DM intake, respectively; differences of 143 and 142 kJ/kg BW0.75, respectively). However, these settings with inaccurate prediction would not be common for goats in present production systems because of the very high levels of dietary concentrate with the 45 g/kg BW0.75 level, as would also be true for use of this moderate level with yearling wethers because of their nutrient requirements primarily restricted to maintenance.
Table 9. Actual and predicted ME intake (kJ/kg BW0.75) for Boer goat wethers.
Based on main effect means, prediction was highly to moderately accurate for growing wethers (20 kJ/kg BW0.75 difference), concentrate levels of 15 and 45 g/kg BW0.75 (39 and 26 kJ/kg BW0.75 differences, respectively), and forage sources A and C (5 and 24 kJ/kg BW0.75 differences, respectively). There were over-estimates of 104, 70, and 107 kJ/kg BW0.75 for yearling wethers, the moderate dietary level of concentrate, and forage source B, respectively. Over-prediction with yearlings resulted from moderate and high levels of concentrate and that with the moderate level of concentrate was because of yearling rather than growing wethers. Over-prediction with forage source B suggests less accuracy with forages having characteristics promoting relatively high intake that perhaps limits extent of digestion.
5. Conclusion
Effects of dietary level of concentrate on ad libitum intake of forage had much greater influence on digested nutrient intake than changes in digestion. Low and moderate levels of concentrate did not depress fibre digestion in growing wethers. Conversely, fibre digestion by yearlings was markedly depressed by the high concentrate level perhaps because of lower forage intake in g/day. Prediction of ME intake with the LINC system was reasonably accurate, slightly more so with growing vs. yearling wethers. Inaccurate prediction for both animal types with the highest level of concentrate is perhaps of little practical importance since this would not be a typical production setting at the present time, and the same consideration exists for the moderate level with yearlings. Development of a larger database, particularly with multiple levels of supplementation slightly below and above the low level of this experiment and a wider array of forage qualities, would be beneficial for further evaluation of the prediction of negative associative effects with the LINC system.
Disclosure statement
No potential conflict of interest was reported by the authors.
Additional information
Funding
References
- AOAC. 2006. Official methods of analysis. 18th ed. Gaithersburg (MD): AOAC International.
- Broderick GA, Kang JH. 1980. Automated simultaneous determination of ammonia and total amino acids in rumen fluid and in vitro media. J Dairy Sci. 63:64–75. doi: 10.3168/jds.S0022-0302(80)82888-8
- Coleman SW, Hart SP, Sahlu T. 2003. Relationships among forage chemistry, rumination and retention time with intake and digestibility of hay by goats. Small Rumin Res. 50:129–140. doi: 10.1016/S0921-4488(03)00116-0
- Dulphy JP, Remond B, Theriez M. 1980. Ingestive behavior and related activities in ruminants. In: Ruckebusch Y, Thivend Y. editors. Digestive physiology and metabolism in ruminants. Westport (CT): AVI Publishing Company Inc; p. 103–122.
- Galyean ML, Goetsch, AL. 1993. Utilization of forage fiber by ruminants. In: Jung HG, Buxton DR, Hatfield RD, Ralph J. editors. Forage cell wall structure and digestibility. Madison (WI): Am. Soc. Agron., Crop Sci. Soc. Am., Soil Sci. Soc. Am; p. 33–72.
- Galyean ML, Owens FN. 1991. Effects of diet composition and level of feed intake on site and extent of digestion in ruminants. In: Tsuda T, Sasaki Y, Kawashima R. editors. Physiological aspects of digestion and metabolism in ruminants. New York (NY): Academic Press Inc.; p. 483–514.
- Garrett WN, Mayer JH, Lofgreen GP. 1959. The comparative energy requirements of sheep and cattle for maintenance and gain. J Anim Sci. 18:528–547.
- Goetsch AL, Gipson TA. 2014. Review: use of a web-based nutrient-requirement-calculation system to assess potential influences of various factors on nutrient needs of goats while grazing. Prof Anim Sci. 30:192–214.
- Hoover WH. 1986. Chemical factors involved in ruminal fiber digestion. J Dairy Sci. 69:2755–2766. doi: 10.3168/jds.S0022-0302(86)80724-X
- Horn GW, McCollum FT. 1987. Energy supplementation of grazing ruminants. In: Proceedings of the grazing livestock nutrition conference. Laramie: University of Wyoming; p. 125–136.
- Jarrige R, Demarquilly C, Dulphy JP, Hoden A, Robelin J, Beranger C, Geay Y, Journet M, Malterre C, Micol D, Petit M. 1986. The INRA “fill unit” system for predicting the voluntary intake of forage-based diets in ruminants: A review. J Anim Sci. 63:1737–1758.
- Lechner-Doll M, Kaske M, Engelhardt WV. 1991. Factors influencing mean retention time of particles in the forestomach of ruminants and camelids. In: Tsuda T, Sasaki Y, Kawashima R. editors. Physiological aspects of digestion and metabolism in ruminants. New York (NY): Academic Press Inc.; p. 455–482.
- Lu CD, Potchoiba MJ, Sahlu T, Fernandez JM. 1990. Performance of dairy goats fed isonitrogenous diets containing soybean meal or hydrolyzed feather meal during early lactation. Small Rumin Res. 3:425–434. doi: 10.1016/0921-4488(90)90073-F
- McCollum FT, Horn GW. 1990. Protein supplementation of grazing livestock: a review. Prof Anim Sci. 6(2):1–16.
- Mertens DR, Loften JR. 1980. The effect of starch on forage fiber digestion kinetics in vitro. J Dairy Sci. 63:1437–1446. doi: 10.3168/jds.S0022-0302(80)83101-8
- Minson DJ. 1990. Forage in ruminant research. New York (NY): Academic Press.
- Moore JE, Goetsch AL, Luo J, Owens FN, Galyean ML, Johnson ZB, Sahlu T, Ferrell CL. 2004. Prediction of fecal crude protein excretion of goats. Small Rumin Res. 53:275–292. doi: 10.1016/j.smallrumres.2004.04.008
- Mould FL, Ørskov ER, Mann SO. 1983. Associative effects of mixed feeds. I. Effects of type and level of supplementation and the influence of the rumen fluid pH on cellulolysis in vivo and dry matter digestion of various roughages. Anim Feed Sci Technol. 10:15–30. doi: 10.1016/0377-8401(83)90003-2
- Nocek JE, Russell JB. 1988. Protein and energy as integrated systems. Relationship of ruminal protein and carbohydrate availability to microbial synthesis and milk production. J Dairy Sci. 71:2070–2107. doi: 10.3168/jds.S0022-0302(88)79782-9
- NRC. 1981. Nutrient requirements of goats: Angora, dairy, and meat goats in temperate and tropical countries. Washington (DC): National Academy Press.
- NRC. 2007. Nutrient requirements of small ruminants. Sheep, goats, cervids, and New World camelids. Washington (DC): National Academy Press.
- Paterson JA, Belyea FL, Bowman JP, Kerley MS, Williams JE. 1994. The impact of forage quality and supplementation regimen on ruminant animal intake and performance. In: Fahey GC, Jr. editor. Forage quality, evaluation, and utilization. Madison (WI): Am. Soc. Agron., Crop Sci. Soc. Am., Soil Sci. Soc. Am; p. 59–114.
- Sahlu T, Dawson LJ, Gipson TA, Hart SP, Merkel RC, Puchala R, Wang Z, Zeng S, Goetsch AL. 2009. Impact of animal science research on U.S. goat production and predictions for the future. J Anim Sci. 87:400–418. doi: 10.2527/jas.2008-1291
- SAS. 2008. SAS/STAT® 9.2 user’s guide. Cary (NC): SAS Inst. Inc.
- Teixeira IAMA, St-Pierre N, Resende KT, Cannas A. 2011. Prediction of intake and average daily gain by different feeding systems for goats. Small Rumin Res. 98:93–97. doi: 10.1016/j.smallrumres.2011.03.018
- Thonney ML, Hogue DE. 2013. Fermentable fiber for diet formulation. In: Proceedings of the 2013 Cornell nutrition conference for feed manufacturers. Ithaca (NY): Cornell University; p. 174–189. Available from http://www.ansci.cornell.edu/pdfs/CN2013_complete.pdf
- Uden P. 1984. Digestibility and digesta retention in dairy cows receiving hay or silage at varying concentrate levels. Anim Feed Sci Technol. 11:279–291. doi: 10.1016/0377-8401(84)90043-9
- Van Soest PJ. 1994. Nutritional ecology of the ruminant. Ithaca (NY): Cornell University Press.