ABSTRACT
Objectives
Inadequate dietary magnesium (Mg) intake is a growing public health concern. Mg is critical for diverse metabolic processes including energy production, macromolecule biosynthesis, and electrolyte homeostasis. Inadequate free Mg2+ ion concentration ([Mg2+]) in the brain is associated with several neurological and behavioral disorders. Elevating [Mg2+]in the brain using oral Mg supplementation has proven to be challenging due to the tight regulation of Mg2+ transport to the brain. This study explored the effect of short-term moderate reduction in dietary Mg intake (87% of normal Mg diet for 30 days) on [Mg2+] in the cerebrospinal fluid (CSF) ([Mg2+]CSF) and red blood cells (RBCs) ([Mg2+]RBC) in adult male rats. In addition, we investigated the effectiveness of magnesium-rich blend of Swiss chard and buckwheat extracts (SC/BW extract) in increasing brain [Mg2+] compared to various Mg salts commonly used as dietary supplements.
Methods
Animals were assigned to either normal or low Mg diet for 30 - 45 days. Following this, animals maintained on low Mg diet were supplemented with various Mg compounds. [Mg2+]CSF and [Mg2+]RBC were measured at baseline and following Mg administration. Anxiety-like behavior and cognitive function were also evaluated.
Results
The present study showed that a short-term and moderate reduction in Mg dietary intake results in a significant decline in [Mg2+]CSF and [Mg2+]RBC and the emergence of anxiety-like behavior in comparison to animals maintained on normal Mg diet. Supplementation with SC/BW extract significantly elevated [Mg2+]CSF and improved animal performance in the novel object recognition test in comparison with animals maintained on reduced Mg intake and supplemented with various Mg compounds.
Discussion
These observations indicate that brain [Mg2+] is more sensitive to a short-term and moderate reduction in Mg dietary intake than previously thought and emphasizes the importance of dietary Mg in replenishing brain Mg2+ reserves.
Introduction
Magnesium (Mg) compounds commonly used in dietary supplements have demonstrated poor delivery of Mg to the central nervous system (CNS) [Citation1,Citation2]. Bioavailability of either organic or inorganic Mg compounds is dependent on its solubility, stability constant, and the general health of the subject’s gastrointestinal tract (GI). Patients are often unaware of the status of their GI health and/or the presence of any GI condition(s) that impede the proper absorption of the Mg compound they may select as a dietary supplement [Citation3]. The assessment of Mg status is challenging, as commonly used Mg measurements in the clinic do not reflect Mg status in various tissues especially in the brain [Citation4,Citation5].
There is a substantial need for effective dietary supplementation of Mg in the United States population. The recommended dietary allowance for Mg is 320 and 420 mg for adult females and males, respectively [Citation6]. In the past 100 years, the dietary intakes of Mg in the United States have declined from about 450–500 mg/day to 175–225 mg/day [Citation3,Citation7]. Further, it is estimated that about 60% of adults in the United States consumed below the established recommended daily allowance and that 45% are Mg deficient [Citation5,Citation6,Citation8].
Subclinical Mg deficiency is common in the general population and is associated with the development and progression of several diseases including cardiovascular, neurological, and metabolic diseases and early mortality [Citation3,Citation9–19]. Suboptimal Mg status is the result of either inadequate dietary Mg intake, poor absorption and/or increased elimination from the body. However, insufficient dietary Mg intake is arguably the leading factor accounting for suboptimal Mg status.
The effect of moderate reduction in dietary Mg intake on general health and particularly on CNS function is often underestimated. It has been previously shown that short-term (2–4 weeks) moderate reduction in dietary Mg intake (30–35% normal diet) leads to progressive decrease in brain intracellular free Mg ion concentrations ([Mg2+]) in a rat model [Citation20]. In humans, a reduction in brain [Mg2+] is associated with neurological disturbances and increased susceptibility to brain injury [Citation2,Citation19,Citation21–26].
[Mg2+] is higher in the cerebrospinal fluid (CSF) ([Mg2+]CSF) than in plasma. The active transport system that maintains this concentration gradient limits the amount of Mg2+ that crosses the blood brain barrier. Human and animal studies show that boosting brain [Mg2+] via chronic oral Mg supplementation is challenging [Citation27–30]. The intravenous infusion of Mg sulfate (MgSO4) in rats for 5 days, increased plasma [Mg2+] three-fold but failed to elevate [Mg2+] in the brain [Citation28]. Intravenous infusion of MgSO4 for 60 min in 2-day and 40-day old new born swine showed significant increases in plasma [Mg2+] in both age groups, but had no effect on brain [Mg2+] [Citation31]. In humans, a 100%–300% increase in blood [Mg2+] via intravenous infusion of MgSO4 resulted in only 10%–19% elevation in [Mg2+]CSF [Citation32]. Mercieri et al. showed that increasing serum [Mg2+] over 80% of the baseline value did not change [Mg2+]CSF in neurologically healthy patients [Citation33]. Taken together, this raises questions on the selection of Mg supplement(s) suitable for replenishing and maintaining healthy brain Mg2+ stores in individuals with suboptimal dietary Mg intake.
A recently developed Mg compound (magnesium-L-threonate, Mg threonate) significantly increased [Mg2+] in the brain when added to drinking water [Citation2] or administered as a bolus gavage in adult rats [Citation1]. This is a significant finding as Mg salts commonly used in dietary supplements have poor delivery of Mg2+ to the brain [Citation1,Citation2].
Mg intake from diet remains the ideal approach to maintain optimal Mg status. Mg in food matrices is bound to various organic and inorganic compounds (multiform of Mg) and may provide a more effective approach to improve Mg status in the brain and various other tissues [Citation34–38]. About 15–20% of Mg in mature leaves is bound to chlorophyl and about 75% is associated with protein synthesis through its roles in ribosomal structure and function [Citation34,Citation36]. A small percentage of leaf Mg is either free, ionic Mg2+, or Mg complexed with organic acids, amino acids, and adenosine triphosphate (ATP) [Citation36,Citation38]. Based on recent NHANES 2015–2016 data, the mean daily amounts of dietary Mg consumed for male and female aged 20 years and over are 345 and 272 mg, respectively [Citation39]. This represents a deficit in established recommended daily allowance of about 17% for male and 15% for female aged 20 years and over. Having this, we posed the two questions. (1) does a short-term moderate reduction (13%) in Mg dietary intake alter [Mg2+]CSF and [Mg2+] in red blood cell (RBC) ([Mg2+]RBC)and impact behavioral outcomes (2) would a plant-based supplement made from Swiss chard and buckwheat extracts (SC/BW extract) rich in naturally occurring Mg elevate [Mg2+]CSF and [Mg2+]RBC?
We utilized a proprietary blend of organic buckwheat and Swiss chard extracts as a plant-based multiform source of Mg to leverage both the benefits of organic cultivation and the natural mineral composition of these cultivars. Both Swiss chard and buckwheat plants are rich in Mg [Citation40–44]. Organically grown foods tend to have more dry matter and higher levels of certain minerals such as Mg in comparison to conventionally grown produce [Citation45,Citation46].
Materials and methods
Animals
Healthy male Sprague Dawley rats (150–175g weight on arrival; Envigo (Indianapolis, IN)) were used in the study. Animals were assigned unique identification numbers and group housed (2–3 rats per cage) in ventilated cages with micro-isolator filter tops. All rats were maintained at a temperature between 20°C and 23°C with 50% humidity on a 12h light/dark cycle. Food and water were available ad libitum. Testing was performed during the light part of the cycle. All studies were approved by the Institutional Animal Care and Use Committee (IACUC) established at PsychoGenics Inc. according to the rules set out by the Public Health Service Office of Laboratory Animal Welfare (IACUC 180_0313 and IACUC 204_0613).
Study design
Two studies were conducted with different groups of animals. In both studies, the animals were assigned to one of two diets obtained from LabDiet (St. Louis, MO, U.S.A.): normal dietary Mg (LabDiet 5001, containing 0.23% Mg) (Control diet) or 87% normal dietary Mg (LabDiet 5008, containing 0.2% Mg) (Mg deficient diet (MgD)). The control diet (LabDiet 5001) contains 25% protein, 5% fat, 5.3% fiber and provides 4.09 Kcal/g. The Mg deficient diet (LabDiet 5008) contains 23.5% protein, 6.5% fat, 3.8% fiber and provides 4.15 Kcal/g. In the first study, the animals were kept on their assigned diet for 30 days after which the first CSF collection (baseline, day 0) was performed. Twenty-four hours after the initial CSF baseline collection, animals maintained on 87% normal dietary Mg (MgD) were divided into 5 treatment groups and received either vehicle (MgD + vehicle), SC/BW extract (MgD + SC/BW extract), Mg threonate (MgD + Mg threonate), Mg citrate (MgD + Mg citrate) or Mg glycinate (MgD + Mg glycinate). Animals maintained on normal Mg diet received vehicle (control diet + vehicle). Animals received 14 days of treatment and on the 15th day (post-intervention, day 15), the second CSF collection was performed.
In the second study (), the animals were kept on their assigned diet for 30 days and behavioral tests were conducted on days 30–44 (baseline tests, day 0). Twenty-four hours after the completion of the last behavioral test, animals maintained on low Mg diet were divided into 4 treatment groups and received either vehicle (MgD + vehicle), SC/BW extract (MgD + SC/BW extract), Mg threonate (MgD + Mg threonate), or Mg citrate (MgD + Mg citrate). Animals maintained on normal Mg diet also received vehicle as treatment (control diet + vehicle). Animals received 45 days of treatment with behavioral tests conducted during the last 14 days (post-intervention, day 45) ().
Table 1. Study design and timeline for behavioral tests.
In both studies, animals were maintained on their assigned diets throughout the study. All treatments were given twice per day (BID) via oral gavage (PO). Water was used as the vehicle. A total of 14–15 rats were used in each group. The administration of the treatments twice per day ensures optimal bioavailability of Mg and minimizes possible GI adverse events. Intestinal absorption of Mg from food and supplements is affected by the amount of Mg ingested where the percent of Mg absorbed decreases as the dose of Mg ingested increases [Citation47].
Magnesium compounds
The following Mg compounds were used: magnesium threonate (Magceutics Inc., U.S.A.), magnesium citrate (Jost Chemical CO., U.S.A.), and magnesium glycinate (Innophos Nutrition, U.S.A.). The SC/BW juice extract containing 15.75 mg elemental Mg/g dry weight (1.575% w/w) was obtained from Standard Process Inc. (Palmyra, WI, U.S.A.). The Swiss chard and buckwheat used in this study are grown on the Standard Process Inc. organic farm in Wisconsin. The green leaves of the Swiss chard and whole buckwheat plant (aerial parts) are juiced and dried on site using Standard Process Inc. proprietary processes. The main micro and macronutrient content in one-gram dry weight of the SC/BW extract: fat (0.086 g), carbohydrate (0.83 g), protein (0.17 g), vitamin K1 (6.21 mcg), Calcium (3.45 mg), iron (1.03 mg), phosphorus (5.2 mg), and potassium (65.5 mg). All Mg compounds and SC/BW powder were dissolved in water and administered via oral gavage at a dose of 20 mg elemental Mg/kg twice per day (40 mg/kg/day).
CSF and RBC collections
Baseline collections (day 0): Rats were anaesthetized with isoflurane and placed in a stereotaxic frame. The atlanto-occipital membrane was exposed, and a microneedle was inserted into the cisterna magna via the atlanto-occipital membrane. Approximately 25–50 µL of CSF was slowly extracted and immediately frozen on dry ice and then stored at −80°C. A small piece of moist collagen foam was used to seal the punctured membrane. The exposed muscle and skin were sutured in layers and the animal placed in a warmed chamber to recover from anaesthesia before returning to home cage. Animals received 5 mg/kg carprofen sub-cutaneous as post-operative analgesic and again at 24 h. For RBC isolation, 200 ul of blood from tail vein was collected into lithium heparin tubes. The whole blood was centrifuged at 500× g for 10 min at 4°C. The haematocrit and plasma levels were marked. The plasma and buffy coat were discarded, and the tube filled with saline to the original plasma level, capped and gently inverted to mix. The RBCs were centrifuged at 500× g for 10 min at 4°C and the supernatant was aspirated and discarded. This saline wash was repeated two more times for a total of 3 saline washes. The RBC pellet was then frozen on dry ice. All samples were stored at −80°C and shipped on dry ice to Pronexus Analytical (Bromma, Sweden) for [Mg2+]CSF and [Mg2+]RBC analysis.
Terminal collections (day 15): In the morning of the day after the completion of 14 days of treatment (day 15), animals were anaesthetized with Pentobarbital (60 mg/kg) prior to terminal CSF and RBC collections. The atlanto-occipital membrane was exposed and ∼0.1 mL of CSF was extracted from the cisterna magna and frozen on dry ice. For RBC isolation, 1.5 mL of blood collected by cardiac puncture was transferred into lithium heparin tubes. Whole blood was centrifuged at 500× g for 10 min at 4°C. The haematocrit and plasma levels were marked. Plasma and buffy coat were discarded, and the tube filled with saline to the original plasma level, capped and gently inverted to mix. The RBCs were centrifuged at 500× g for 10 min at 4°C and the supernatant was aspirated and discarded. The saline wash was repeated two more times for a total of three washes. The RBC pellet was then frozen on dry ice. All samples were stored at −80°C and shipped on dry ice to Pronexus Analytical for [Mg2+]CSF and [Mg2+]RBC analysis.
Spectrophotometric analysis of free Mg2+ ions in the CSF and RBC
[Mg2+]CSF and [Mg2+]RBC were quantified with spectrophotometric calmagite method using a commercially available kit (Biolabo, France). Briefly, 200 µl of calmagite reagent was pipetted into each well of a 96-well plate (ThermoFisher Scientific, U.S.A.), thereafter 2 µl of standard Mg2+ solution (for calibrations) prepared in artificial CSF or 2 µl of either CSF or RBC samples were pipetted to the respective wells. The plate was centrifuged at 2500 rpm for 60 s and shaken in an orbital shaker for 3 min. The plate was placed into a Labrox Multimode reader (Labrox, Finland) and the absorbance was read at 530 nm. The calibration standards and the samples were analyzed in triplicate. Pronexus Analytical (Bromma, Sweden) conducted the analysis of [Mg2+]CSF and [Mg2+]RBC.
Behavioral tests
Elevated plus maze
The elevated plus maze (EPM) test measures anxiety-like behavior in rodents [Citation48]. The maze (Hamilton Kinder) consists of two closed arms (15.75 cm h × 10.8 cm w × 50.17 cm l) and two open arms (10.8 cm w × 50.17 cm l) forming a cross, with a square center platform (10.8 × 10.8 cm). All visible surfaces were made of black acrylic. Each arm of the maze was placed on a support column 85.09 cm above the floor. Antistatic black vinyl curtains (7’ tall) surrounded the EPM to make a 7’ × 7’ enclosure. Animals were allowed to acclimate to the experimental room for at least 1 h before the test. Following the appropriate pre-treatment time, rats were then placed in the center of the EPM facing the open arm for a 5-minute run. The time spent, distance traveled, and the number of entries were automatically recorded by the computer. The EPM was thoroughly cleaned after each test. Data of EPM test were expressed as the ratio of time spent in open arms over total time in open or enclosed arms, and the ratio of entries in open arms over total entries in open or enclosed arms. Anxious animals limit their open arm exploration. Animals with time in open arm less than 0.5% in either baseline or post intervention were regarded as outliers and eliminated from data analysis.
Novel object recognition
The Novel Object Recognition (NOR) is a test of recognition learning and memory retrieval, which takes advantage of the spontaneous preference of rodents to investigate a novel object compared with a familiar one [Citation49]. NOR test was conducted in an open-field arena (40 × 40 cm) placed in a sound-attenuated room under dimmed lighting. Each rat was tested separately. All training and testing trials were videotaped and scored by an observer blind to treatments. On Days 1 and 2, rats were allowed to freely explore the arena (no objects inside) for a 5-minute habituation period. On Day 3 (training and testing day), rats were placed in the test arena in the presence of two identical objects (Object A–A or Object B–B) for 3 min (first test, T1). One hour later, the rats were again placed into the test arena in the presence of one familiar object and one novel object (Object A and Object B) for 3 min, and the time spent exploring both objects is recorded. The presentation order and position of the objects (left/right) was randomized between rats to prevent bias from order or place preference. Data of NOR test (second test, T2) were expressed as Recognition Index (RI), which is defined as the ratio of the time spent exploring the novel object over the total time spent exploring both objects (Novel/(Familiar + Novel) × 100%) during the test session. Rats with recognition index above 90% or below 30% during the first minute of T2 were eliminated because they suggest strong (non-memory) bias between two objects. In addition, the animals whose data fell above or below two standard deviations from the mean were removed as outliers from the final analysis.
Spontaneous alternation
Spontaneous alternation is a test to evaluate the animal’s spatial working memory [Citation50]. The plus-shaped maze used in the present study consisted of four clear Plexiglas arms (each arm measures 60 × 15 × 15 cm, with a 15 × 15 cm central hub). The maze is set on a rotating table and is randomly rotated after each testing trial. The maze is also cleaned with 70% ethanol after each trial. The test was conducted in a lit testing room containing a moderate density of extra maze cues between 9 am and 3 pm. The rats were tested with a counterbalanced order in different testing days.
After being placed in the central hub, each rat was permitted to move freely through the maze for 10 min. During that time, the number and sequence of entries were manually recorded by the researcher. An alternation is defined as entry into four different arms on overlapping sets of five consecutive entries.
The percent alternations were calculated as the ratio of number of alternations over total possible alternations multiplied by 100. Data on the total number of arm entries were also analyzed. A rat must have at least 12 arm entries to get a valid alternation score. When the animal’s short-term memory is normal, it is unlikely to enter an arm that was just visited. When memory is impaired, the spontaneous alternation score decreases.
Statistical analysis
Data presented in , were analyzed using two-way mixed analysis of variance (ANOVA) with day as repeated measure followed by simple main effects with Tukey’s post hoc test where indicated. Unpaired Student t-test was used for comparing [Mg2+]CSF and [Mg2+]RBC and elevated plus maze data between control and MgD diets ((a–d)). Tukey’s pairwise comparison was used for data presented in . Data are presented as mean ± SEM (standard error of the mean). Statistical significance was defined as p < 0.05. Significant differences in figures are marked with different letters or with an asterisk (*). No significant violation of model assumptions (normal distribution, homogenous variance and sphericity) was observed. All statistical evaluations were performed using R Statistical Software Package Version 3.6.0. (R Core Team, 2019) for Microsoft Windows.
Figure 1. Effect of a short-term moderate reduction of dietary Mg intake (87% normal dietary Mg) on [Mg2+]CSF (a) and [Mg2+]RBC (b). (c) and (d) shows the effect on anxiety-like behavior as assessed by percent time spent in open arms and percent entries to open arms of the elevated plus maze. Unpaired t test, *p < 0.05, **p < 0.01. Data presented as mean ± SEM.
![Figure 1. Effect of a short-term moderate reduction of dietary Mg intake (87% normal dietary Mg) on [Mg2+]CSF (a) and [Mg2+]RBC (b). Figure 1(c) and (d) shows the effect on anxiety-like behavior as assessed by percent time spent in open arms and percent entries to open arms of the elevated plus maze. Unpaired t test, *p < 0.05, **p < 0.01. Data presented as mean ± SEM.](/cms/asset/6bc93ad6-98d4-4110-b86f-3beaf83f4a3e/ynns_a_1995119_f0001_ob.jpg)
Results
Short-term moderate reduction in dietary intake of magnesium reduced [Mg2+]CSF and [Mg2+]RBC and the emergence of anxiety-like behavior
Adult male rats maintained on 87% normal dietary Mg intake for only 30 days (MgD diet group) demonstrated a significant fall in measurable [Mg2+]CSF (p < 0.0001, n = 72) and [Mg2+]RBC (p = 0.0344, n = 72) in comparison to animals maintained on control diet (n = 14–15) ((a,b)). Interestingly, animals maintained on what would be considered a moderate MgD diet showed signs of anxiety-like behavior as assessed in the elevated plus maze test. The MgD diet group showed a significant decrease in the percent time spent in the open arms (p = 0.0089, n = 60) and the percent entries to open arms (p = 0.0307, n = 60) in comparison to animals maintained on control diet (n = 14) ((c,d)). There were no significant changes between MgD and control diet groups in body weight (data not shown), the novel object recognition test (, day 0) or spontaneous alternation (, day 0), indicating that a moderate reduction in dietary Mg intake does not result in overt behavioral changes. These findings indicate that the emergence of anxiety-like behavior maybe the first symptom of reduction in brain [Mg2+].
Effect of various Magnesium compounds on [Mg2+]CSF and [Mg2+]RBC
CSF and RBC samples were collected at baseline (day 0) and 24 h after the completion of a 14 days treatment with various Mg compounds (day 15). Two-way mixed ANOVA for [Mg2+]CSF indicated significant effects of treatment (p = 0.0006) and treatment x time interaction (p = 0.0029) but no significant time effect (p = 0.715) (). Simple main effect of treatment indicated a significant treatment effect at day 0 (p < 0.0001) and 15 (p = 0.0279). Baseline [Mg2+]CSF (day 0) for MgD diet group was significantly reduced in comparison control diet (p < 0.05) (see also analysis presented in (a)). At day 15, MgD + SC/BW extract group showed significantly higher [Mg2+]CSF in comparison to MgD + vehicle (p = 0.039). On the other hand, simple main effect of time indicated a significant increase in [Mg2+]CSF in MgD + SC/BW extract (p = 0.0129) group and a decrease in control diet (p = 0.0184) (day 0 versus 15). It is interesting to note that although it did not reach significance, [Mg2+]CSF in MgD + Mg threonate group was higher at day 15 in comparison to baseline values ().
Figure 2. [Mg2+]CSF for animals maintained on magnesium deficient diet (MgD) or control diet before (day 0) and after treatment (day 15) with various magnesium compounds. (a) significantly different from MgD + vehicle (p < 0.05); (b) significantly different from control diet (p < 0.05); (c) significantly different from day 0 (p < 0.02); (d) significantly different from day 0 (p < 0.02). n = 13–15 per group. Data presented as mean ± SEM.
![Figure 2. [Mg2+]CSF for animals maintained on magnesium deficient diet (MgD) or control diet before (day 0) and after treatment (day 15) with various magnesium compounds. (a) significantly different from MgD + vehicle (p < 0.05); (b) significantly different from control diet (p < 0.05); (c) significantly different from day 0 (p < 0.02); (d) significantly different from day 0 (p < 0.02). n = 13–15 per group. Data presented as mean ± SEM.](/cms/asset/f9ea0265-b11a-48dd-8a2a-e0d0b33a3057/ynns_a_1995119_f0002_ob.jpg)
Data from were calculated and presented in as a percentage of baseline level and provide visual comparison to [Mg2+]CSF data reported by Slutsky et. al. (2010) [Citation2]. At day 15, [Mg2+]CSF was 9.5 and 8.3% higher than baseline for MgD + SC/BW extract and Mg threonate groups, respectively (p < 0.05) (). In agreement with previous published work [Citation2], repeated CSF sampling in rats that did not receive any magnesium supplementation (the two control groups) resulted in a 5-7% decrease in [Mg2+]CSF by the second sampling point (day 15). Taking into account this reduction in [Mg2+]CSF associated with repeated CSF collection (about 5%), the actual increase in [Mg2+]CSF with SC/BW extract and Mg threonate should be around 14.5 and 13.3%, respectively. Other magnesium compounds tested showed only marginal elevation of [Mg2+]CSF in comparison to vehicle controls ().
Figure 3. Elevation of [Mg2+]CSF before (day 0) and after treatment (day 15) with different magnesium compounds. Data from were calculated and presented as a percentage of baseline level. *Significantly different from MgD + vehicle and control diet groups, p < 0.05. Data presented as mean ± SEM.
![Figure 3. Elevation of [Mg2+]CSF before (day 0) and after treatment (day 15) with different magnesium compounds. Data from Figure 2 were calculated and presented as a percentage of baseline level. *Significantly different from MgD + vehicle and control diet groups, p < 0.05. Data presented as mean ± SEM.](/cms/asset/8d12e460-e77d-4cec-abb8-bbadc29e4160/ynns_a_1995119_f0003_ob.jpg)
For [Mg2+]RBC, two-way mixed ANOVA indicated a significant effect of time (p < 0.0001) and no significant effect of treatment (p = 0.1171) or treatment x time interaction (p = 0.2846). The [Mg2+]RBC levels were significantly higher at day 15 when compared to baseline (). The observed increase in [Mg2+]RBC in all groups at day 15 is interesting. This study measured the physiologically active free ionic Mg2+ levels that tend to fluctuate based on various factors such as the type of anesthesia [Citation33], stress [Citation33] and the circadian rhythm [Citation51]. In this study, the anesthesia used at baseline (survival procedure) and day 15 (non-survival, terminal procedure) were different. In addition, animals were gavaged twice per day for 14 days, which is considered a mild stress that may have an impact on [Mg2+]RBC. [Mg2+]CSF seemed more tightly regulated than [Mg2+]RBC and was not affected by these factors. Nevertheless, we noted that at day 15, [Mg2+]RBC for animals treated with SC/BW extract tends to be higher than MgD + vehicle and within the range observed with control diet + vehicle group ().
Effect of various magnesium compounds on percent time spent in open arms and percent entries in open arms of the elevated plus maze
Two-way mixed ANOVA for percent time spent in the open arms () indicated significant main effects of treatment (p = 0.0021) and time (p = 4.202e-08). No significant effect of treatment x time interaction (p = 0.988) was observed. Post hoc analysis of the treatment main effect indicated that the percent time spent in open arms was significantly reduced for animals maintained at MgD diet treated with vehicle (p = 0.0045), SC/BW extract (p = 0.0049) and Mg citrate (p = 0.0144) in comparison to control diet at both day 0 and 45. Supplementation of various Mg compounds did not reverse the anxiety-like profile induced by reduction of Mg intake from the diet.
Figure 5. Percent time spent in the open arms of the elevated plus maze before (day 0) and after (day 45) treatment with different magnesium compounds. *Significantly different from control diet group, p < 0.05. n = 14–15 per group. Data presented as mean ± SEM.
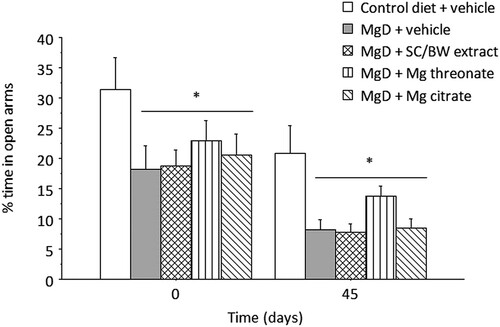
Two-way mixed ANOVA for number of entries in open arms () indicated significant main effects of treatment (p = 0.0051) and time (p = 0.0021). No significant effect of treatment x time interaction (p = 0.67) was observed. Animals in MgD + vehicle group showed significantly fewer entries in comparison to animals maintained on control diet (p = 0.0015).
Effect of various magnesium compounds on Novel Object Recognition index
Two-way mixed ANOVA for recognition index (RI) in the novel object recognition test () indicated a significant main effect of treatment (p = 0.0266) and no significant effects of time (p = 0.431) or treatment × time interaction (p = 0.237). The RI for animals treated with SC/BW extract was significantly higher than MgD + vehicle group (p = 0.0358). Although not significant, it is interesting to note that the RI for animals treated with Mg citrate was higher than other groups.
Effect of various magnesium compounds on spontaneous alternation
Two-way mixed ANOVA for percent spontaneous alternation () did not indicate a significant main effect of treatment (p = 0.6988), time (p = 0.7751) or treatment × time interaction (p = 0.0853).
Discussion
Dietary Mg intake in the US population is suboptimal and the resulting health consequences of poor Mg status are often underestimated [Citation9,Citation52,Citation53]. Subclinical Mg deficiency is of particular concern with regard to CNS health. Poor Mg status is considered a risk factor for the development and progression of various neurological diseases [Citation17–19,Citation21–23,Citation25,Citation54]. Elevation of Mg in the CNS with oral supplementation is challenging due to the tight regulation of Mg transport across the blood brain barrier [Citation2,Citation30–32,Citation55]. [Mg2+] in the brain is more sensitive to a reduction in dietary Mg intake than was previously thought [Citation20,Citation56]. Altura et al. reported that rats maintained on 30-35% normal dietary Mg intake for only 2–4 weeks showed a significant decrease in brain [Mg2+] [Citation20].
In the present study, rats maintained on 87% normal Mg dietary intake for 4 weeks have demonstrated a significant decrease in [Mg2+]CSF ((a)). The 87% normal Mg dietary intake (representing only 13% reduction in dietary Mg intake) is particularly relevant as it matches the estimated Mg dietary gap in the general population [Citation6,Citation39]. Slutsky et al. showed a drop in [Mg2+]CSF associated with CSF sampling [Citation2]. It is estimated that each CSF sampling led to 15-30% loss of CSF Mg2+ and that this decrease in [Mg2+]CSF is not readily replenished. We observed a similar drop in [Mg2+]CSF due to repeated CSF sampling as demonstrated from the data obtained from control rats (MgD + vehicle and control diet + vehicle) (). These observations emphasize the challenges in maintaining a healthy CNS [Mg2+] and the importance of adequate dietary intake of Mg.
The ideal source of Mg is that obtained from whole food. The present study showed that Mg in food matrices (multiform of Mg) is an effective approach to improving Mg status in the brain and eliminate the often-daunting task of selecting a suitable Mg compound as a dietary supplement.
Bioavailability of Mg compounds (organic or inorganic) is dependent on solubility, stability constant of the complex (the strength of the bond between Mg and the organic/inorganic molecule bound to it) and the general health of the subject’s GI tract. The patient’s GI health status plays an important role in determining the bioavailability of the Mg compound selected. Approximately 45% of Mg obtained from the diet is actually absorbed by the body and of this 45%, about 30% is absorbed in the ileum and colon and 15% in the jejunum and duodenum [Citation57]. Clinical conditions that affect the health of the ileum and colon such as Crohn’s disease and ileitis (inflammation of the ileum) may reduce the bioavailability of Mg compounds that are mainly absorbed at the ileum and colon such as Mg oxide, Mg taurinate or Mg lactate.
The relationship between GI health and the selection of Mg compounds has been demonstrated with a study by Schuette et al. who compared the bioavailability of Mg glycinate versus Mg oxide in patients who had undergone an ileal resection (surgical removal of part of the ileum) procedure. This study found that patients with ileal resection were at risk for Mg deficiency. Patients with the largest ileum segment removed responded poorly to Mg oxide (absorbed mainly at ileum) and had a better outcome with Mg glycinate (absorbed mainly at duodenum as a dipeptide). Patients with a small ileum section removed responded equally to Mg oxide and Mg glycinate indicating that both of these forms have similar bioavailability in subjects with a healthy GI tract [Citation58].
We report, for the first time, that rats maintained on only 87% normal dietary Mg intake (13% reduction) for 4 weeks showed a significant decline in [Mg2+]CSF ((a) and ) and the emergence of anxiety-like behavior ((c,d), and ) in comparison to control animals placed on a normal Mg diet. The MgD groups supplemented with SC/BW extract and Mg threonate showed a significant increase from baseline in [Mg2+]CSF in comparison to MgD + vehicle and control diet + vehicle groups (). As expected, Mg citrate and Mg glycinate treatment showed only a marginal and not significant elevation of [Mg2+]CSF in comparison to MgD + vehicle and control diet + vehicle groups. The present data indicates that naturally occurring multiform Mg found in the plant-based supplement SC/BW extract is effective in elevating [Mg2+]CSF similar to Mg threonate. These results highlight the advantage of the whole food matrix as a preferred source of Mg for the whole body and in particular for the CNS. Whole food-based Mg supplements such as SC/BW extract may provide an advantage over dietary supplements with one form of Mg salt especially for individuals with poor GI health status that impacts Mg bioavailability.
Several studies showed that suboptimal Mg status is associated with anxiety [Citation21,Citation23,Citation59–62]. Our results are in agreement with the reported correlation between Mg dietary intake and anxiety. In the present study, rats maintained on a moderate Mg deficient diet spent significantly less time in and made fewer entries into the open arms of the elevated plus maze compared to rats maintained on control diet ((c,d), and ). There were no significant differences in body weight, novel object recognition (), and spontaneous alternation in the plus-maze () between the MgD and control diet groups at baseline (day 0). This indicates that a moderate reduction in dietary Mg intake does not result in overt behavioral changes. The emergence of anxiety-like behavior maybe the first observable behavioral symptom of suboptimal dietary Mg intake and [Mg2+] reduction in the brain.
Although SC/BW extract and Mg threonate treatments elevated [Mg2+]CSF, they failed to reduce the established anxiety observed following the reduction in dietary Mg intake. A lengthier treatment may be required to reverse anxiety or Mg replenishment may be necessary but not sufficient. A higher dose might be needed; however, this is not likely. The dose of elemental Mg used in this study was 40 mg/kg/day which is equivalent to about 400 mg/day for a subject with a body weight of 65 kg (using a conversion factor of 6.2 rat – human) [Citation63]. This is a significant and adequate dose for rats maintained at only 87% of normal Mg intake. Additionally, SC/BW extract and Mg threonate, at the dose used, resulted in a significant elevation in [Mg2+]CSF but did not reverse the anxiety profile.
SC/BW extract significantly improved RI in the novel object recognition test in comparison to control diet group (). Slutsky et al. showed that Mg threonate treatment in rats improves RI [Citation2]. In the present study, Mg threonate treatment in rats maintained on reduced Mg diet did not improve the RI (). There are notable differences between our study and that of Slutsky et al. which could explain this discrepancy. In the present study, animals were maintained on a reduced dietary Mg intake during the course of the study and Mg compounds were given via oral gavage twice per day at a dose of 40 mg elemental Mg/day. In Slutsky et al. the rats were maintained on a normal Mg diet and Mg threonate was given via drinking water at an estimated dose of 50 mg elemental Mg/day. It is important to note that the observed beneficial effect on learning and memory with the group that received the SC/BW extract treatment might be the result of elevating brain [Mg2+] and the possible effects of various other beneficial nutrients in these cultivars such as polyphenols, antioxidants, minerals and vitamins.
Conclusions
Our results show that a short-term and moderate reduction in dietary Mg intake within the estimated gap of Mg intake reported in the United States population leads to a significant decrease in [Mg2+]CSF and the emergence of anxiety-like behavior in adult male rats. Naturally occurring Mg found in SC/BW extract elevated [Mg2+]CSF and improved learning and memory. Although SC/BW extract and Mg threonate elevated brain [Mg2+], they failed to reverse the observed anxiety-like behavior. This study indicates that brain [Mg2+] is more sensitive to a short-term and moderate reduction in Mg dietary intake than previously thought and emphasizes the importance of dietary Mg in replenishing brain Mg2+ reserves.
Acknowledgements
Conceptualization, B.F.E.-K, Q.C., T.H. and Y.R.L.; methodology, B.F.E.-K., and Q.C.; statistical analysis, B.F.E.-K and W.Z.; formal analysis, B.F.E.-K, Q.C. and W.Z.; investigation, B.F.E.-K.; writing – original draft preparation, K.J., C.P., Y.R.L. and B.F.E.-K; writing – review and editing, K.J., C.P., Y.R.L., W.Z. and B.F.E.-K. All authors have read and agreed to the published version of the manuscript.
Disclosure statement
K.J., W.Z., Y.R.L., C.P. and B.F.E.-K. are employees of Standard Process Inc. Standard Process Inc. filed a composition patent for SC/BW extract. The remaining authors declare that they have nothing to disclose.
Data availability statement
The data that support the findings of this study are available from the corresponding author, B.F.E.-K., upon reasonable request.
Additional information
Funding
Notes on contributors
Bassem F. El-Khodor
Bassem F. El-Khodor received his Ph.D. in Neurological Sciences from McGill University, and is the Director of Discovery Science & Innovation at Standard Process Inc.
Karma James
Karma James received her Ph.D. in Food Science from Penn State University and is the Product Development Manager at Standard Process Inc.
Qing Chang
Qing Chang received his Ph.D. in Physiological Psychology from Peking University, and is an Associate Fellow at PsychoGenics Inc.
Wei Zhang
Wei Zhang received her Ph.D. in Macromolecular Science & Engineering from Virginia Tech and is a Nutrition Scientist/Biostatistician at Standard Process Inc.
Yvette R. Loiselle
Yvette R. Loiselle received her M.Sc. in Agriculture from the University of Wisconsin-River Falls and is the Coordinator of Scientific Content at Standard Process Inc.
Chinmayee Panda
Chinmayee Panda received her Ph.D. in Molecular Genetics from North Carolina State University and is a Clinical Nutrition Scientist at Standard Process Inc.
Taleen Hanania
Taleen Hanania received her Ph.D. in Pharmacology at the University of Texas Medical Branch and is the EVP, External Scientific Affairs at PsychoGenics Inc.
References
- Sadir S, Tabassum S, Emad S, Liaquat L, Batool Z, Madiha S, et al. Neurobehavioral and biochemical effects of magnesium chloride (MgCl2), magnesium sulphate (MgSO4) and magnesium-L-threonate (MgT) supplementation in rats: a dose dependent comparative study. Pak J Pharm Sci. 2019;32:277–283.
- Slutsky I, Abumaria N, Wu LJ, Huang C, Zhang L, Li B, et al. Enhancement of learning and memory by elevating brain magnesium. Neuron. 2010;65:165–177. doi:10.1016/j.neuron.2009.12.026.
- Cazzola R, Della Porta M, Manoni M, Iotti S, Pinotti L, Maier JA. Going to the roots of reduced magnesium dietary intake: a tradeoff between climate changes and sources. Heliyon. 2020;6:e05390. doi:10.1016/j.heliyon.2020.e05390.
- Razzaque MS. Magnesium: are we consuming enough? Nutrients. 2018;10. doi:10.3390/nu10121863.
- Workinger JL, Doyle RP, Bortz J. Challenges in the diagnosis of magnesium status. Nutrients. 2018;10. doi:10.3390/nu10091202.
- Moshfegh AG, Ahuja J, Rhodes D, LaComb R. What we eat in America, NHANES 2005-2006: usual nutrient intakes from food and water compared to 1997 dietary reference intakes for vitamin D, calcium, phosphorus, and magneisum. U.S. Department of Agriculture, Agriculture Research Service, Beltsville Human Research Center, Beltsville, MD; 2009.
- Altura BM. Introduction: importance of Mg in physiology and medicine and the need for ion selective electrodes. Scand J Clin Lab Invest Suppl. 1994;217:5–9.
- Quann EE, Fulgoni VL, Auestad N. Consuming the daily recommended amounts of dairy products would reduce the prevalence of inadequate micronutrient intakes in the United States: diet modeling study based on NHANES 2007-2010. Nutr J. 2015;14:90. doi:10.1186/s12937-015-0057-5.
- DiNicolantonio JJ, O'Keefe JH, Wilson W. Subclinical magnesium deficiency: a principal driver of cardiovascular disease and a public health crisis. Open Heart. 2018;5:e000668. doi:10.1136/openhrt-2017-000668.
- Del Gobbo LC, Imamura F, Wu JH, de Oliveira Otto MC, Chiuve SE, Mozaffarian D. Circulating and dietary magnesium and risk of cardiovascular disease: a systematic review and meta-analysis of prospective studies. Am J Clin Nutr. 2013;98:160–173. doi:10.3945/ajcn.112.053132.
- Efstratiadis G, Sarigianni M, Gougourelas I. Hypomagnesemia and cardiovascular system. Hippokratia. 2006;10:147–152.
- Galland L. Magnesium, stress and neuropsychiatric disorders. Magnes Trace Elem. 1991;10:287–301.
- Glasdam SM, Glasdam S, Peters GH. The importance of magnesium in the human body: a systematic literature review. Adv Clin Chem. 2016;73:169–193. doi:10.1016/bs.acc.2015.10.002.
- Guasch-Ferré M, Bulló M, Estruch R, Corella D, Martínez-González MA, Ros E, et al. Dietary magnesium intake is inversely associated with mortality in adults at high cardiovascular disease risk. J Nutr. 2014;144:55–60. doi:10.3945/jn.113.183012.
- Hruby A, Meigs JB, O'Donnell CJ, Jacques PF, McKeown NM. Higher magnesium intake reduces risk of impaired glucose and insulin metabolism and progression from prediabetes to diabetes in middle-aged Americans. Diabetes Care. 2014;37:419–427. doi:10.2337/dc13-1397.
- Ikee R. Cardiovascular disease, mortality, and magnesium in chronic kidney disease: growing interest in magnesium-related interventions. Renal Replac Therapy. 2018;4.; doi:10.1186/s41100-017-0142-7.
- Kirkland AE, Sarlo GL, Holton KF. The role of magnesium in neurological disorders. Nutrients. 2018;10. doi:10.3390/nu10060730.
- Nayak R, Attry S, Ghosh SN. Serum magnesium as a marker of neurological outcome in severe traumatic brain injury patients. Asian J Neurosurg. 2018;13:685–688. doi:10.4103/ajns.AJNS_232_16.
- Xue W, You J, Su Y, Wang Q. The effect of magnesium deficiency on neurological disorders: a narrative review article. Iran J Public Health. 2019;48:379–387.
- Altura BM, Gebrewold A, Zhang A, Altura BT, Gupta RK. Short-term reduction in dietary intake of magnesium causes deficits in brain intracellular free Mg2+ and [H + ]i but not high-energy phosphates as observed by in vivo 31P-NMR. Biochim Biophys Acta. 1997;1358:1–5. doi:10.1016/s0167-4889(97)00077-3.
- Singewald N, Sinner C, Hetzenauer A, Sartori SB, Murck H. Magnesium-deficient diet alters depression- and anxiety-related behavior in mice–influence of desipramine and Hypericum perforatum extract. Neuropharmacology. 2004;47:1189–1197. doi:10.1016/j.neuropharm.2004.08.010.
- Morris ME. Brain and CSF magnesium concentrations during magnesium deficit in animals and humans: neurological symptoms. Magnes Res. 1992;5:303–313.
- Sartori SB, Whittle N, Hetzenauer A, Singewald N. Magnesium deficiency induces anxiety and HPA axis dysregulation: modulation by therapeutic drug treatment. Neuropharmacology. 2012;62:304–312. doi:10.1016/j.neuropharm.2011.07.027.
- Haensch C-A. Cerebrospinal fluid magnesium level in different neurological disorders. Neurosci Med. 2010;01:60–63. doi:10.4236/nm.2010.12009.
- Serita T, Miyahara M, Tanimizu T, Takahashi S, Oishi S, Nagayoshi T, et al. Dietary magnesium deficiency impairs hippocampus-dependent memories without changes in the spine density and morphology of hippocampal neurons in mice. Brain Res Bull. 2019;144:149–157. doi:10.1016/j.brainresbull.2018.11.019.
- Altura BM, Altura BT. Role of magnesium in patho-physiological processes and the clinical utility of magnesium ion selective electrodes. Scand J Clin Lab Invest Suppl. 1996;224:211–234. doi:10.3109/00365519609088642.
- Ates M, Kizildag S, Yuksel O, Hosgorler F, Yuce Z, Guvendi G, et al. Dose-dependent absorption profile of different magnesium compounds. Biol Trace Elem Res. 2019;192:244–251. doi:10.1007/s12011-019-01663-0.
- Kim YJ, McFarlane C, Warner DS, Baker MT, Choi WW, Dexter F. The Effects of plasma and brain magnesium concentrations on lidocaine-induced seizures in the rat. Anesth Analg. 1996;83:1223-8.
- Vink R. Magnesium in the CNS: recent advances and developments. Magnes Res. 2016;29:95–101. doi:10.1684/mrh.2016.0408.
- Wary C, Brillault-Salvat C, Bloch G, Leroy-Willig A, Roumenov D, Grognet JM, et al. Effect of chronic magnesium supplementation on magnesium distribution in healthy volunteers evaluated by 31P-NMRS and ion selective electrodes. Br J Clin Pharmacol. 1999;48:655–662. doi:10.1046/j.1365-2125.1999.00063.x.
- Gee JB, Corbett RJ, Perlman JM, Laptook AR. Hypermagnesemia does not increase brain intracellular magnesium in newborn swine. Pediatr Neurol. 2001;25:304–308. doi:10.1016/s0887-8994(01)00317-4.
- McKee JA, Brewer RP, Macy GE, Phillips-Bute B, Campbell KA, Borel CO, et al. Analysis of the brain bioavailability of peripherally administered magnesium sulfate: A study in humans with acute brain injury undergoing prolonged induced hypermagnesemia. Crit Care Med. 2005;33:661–666. doi:10.1097/01.ccm.0000156293.35868.b2.
- Mercieri M, De Blasi RA, Palmisani S, Forte S, Cardelli P, Romano R, et al. Changes in cerebrospinal fluid magnesium levels in patients undergoing spinal anaesthesia for hip arthroplasty: does intravenous infusion of magnesium sulphate make any difference? A prospective, randomized, controlled study. Br J Anaesth. 2012;109:208–215. doi:10.1093/bja/aes146.
- Karley AJ, White PJ. Moving cationic minerals to edible tissues: potassium, magnesium, calcium. Curr Opin Plant Biol. 2009;12:291–298. doi:10.1016/j.pbi.2009.04.013.
- White PJ, Broadley MR. Biofortification of crops with seven mineral elements often lacking in human diets–iron, zinc, copper, calcium, magnesium, selenium and iodine. New Phytol. 2009;182:49–84. doi:10.1111/j.1469-8137.2008.02738.x.
- Wilkinson SR, Welch RM, Mayland HF, Grunes DL. Magnesium in plants: uptake, distribution, function, and utilization by man and animals. Met Ions Biol Syst. 1990;26:33–56.
- Maathuis FJ. Physiological functions of mineral macronutrients. Curr Opin Plant Biol. 2009;12:250–258. doi:10.1016/j.pbi.2009.04.003.
- Gout E, Rebeille F, Douce R, Bligny R. Interplay of Mg2+, ADP, and ATP in the cytosol and mitochondria: unravelling the role of Mg2+ in cell respiration. Proc Natl Acad Sci U S A. 2014;111:E4560–4567. doi:10.1073/pnas.1406251111.
- U.S. Department of Agriculture, Agricultural Research Service. (2018). Nutrient intakes from food and beverages: mean amounts consumed per individual, by gender and age, what we eat in America, NHANES 2015-2016. 2018.
- Colonna E, Rouphael Y, Barbieri G, De Pascale S. Nutritional quality of ten leafy vegetables harvested at two light intensities. Food Chem. 2016;199:702–710. doi:10.1016/j.foodchem.2015.12.068.
- Gamba M, Raguindin PF, Asllanaj E, Merlo F, Glisic M, Minder B, et al. Bioactive compounds and nutritional composition of Swiss chard (Beta vulgaris L. var. cicla and flavescens): a systematic review. Crit Rev Food Sci Nutr. 2020: 1–16. doi:10.1080/10408398.2020.1799326.
- Mzoughi Z, Chahdoura H, Chakroun Y, Camara M, Fernandez-Ruiz V, Morales P, et al. Wild edible Swiss chard leaves (Beta vulgaris L. var. cicla): nutritional, phytochemical composition and biological activities. Food Res Int. 2019;119:612–621. doi:10.1016/j.foodres.2018.10.039.
- Klepacka J, Najda A, Klimek K. Effect of buckwheat groats processing on the content and bioaccessibility of selected minerals. Foods. 2020;9. doi:10.3390/foods9060832.
- Huda MN, Lu S, Jahan T, Ding M, Jha R, Zhang K, et al. Treasure from garden: Bioactive compounds of buckwheat. Food Chem. 2021;335:127653. doi:10.1016/j.foodchem.2020.127653.
- Crinnion WJ. Organic foods contain higher levels of certain nutrients, lower levels of pesticides, and may provide health benefits for the consumer. Altern Med Rev. 2010;15:4–12.
- Lairon D. Nutritional quality and safety of organic food. Agron. Sustain Dev. 2010;30:33–41. doi:10.1051/agro/2009019.
- Fine KD, Santa Ana CA, Porter JL, Fordtran JS. Intestinal absorption of magnesium from food and supplements. J Clin Invest. 1991;88:396–402. doi:10.1172/JCI115317.
- Walf AA, Frye CA. The use of the elevated plus maze as an assay of anxiety-related behavior in rodents. Nat Protoc. 2007;2:322–328. doi:10.1038/nprot.2007.44.
- Antunes M, Biala G. The novel object recognition memory: neurobiology, test procedure, and its modifications. Cogn Process. 2012;13:93–110. doi:10.1007/s10339-011-0430-z.
- Lalonde R. The neurobiological basis of spontaneous alternation. Neurosci Biobehav Rev. 2002;26:91–104. doi:10.1016/s0149-7634(01)00041-0.
- Newhouse IJ, Johnson KP, Montelpare WJ, McAuliffe JE. Variability within individuals of plasma ionic magnesium concentrations. BMC Physiol. 2002;2:6. doi:10.1186/1472-6793-2-6.
- Rosanoff A, Weaver CM, Rude RK. Suboptimal magnesium status in the United States: are the health consequences underestimated? Nutr Rev. 2012;70:153–164. doi:10.1111/j.1753-4887.2011.00465.x.
- Rosanoff A, Dai Q, Shapses SA. Essential nutrient interactions: does low or suboptimal magnesium status interact with vitamin D and/or calcium status? Adv Nutr. 2016;7:25–43. doi:10.3945/an.115.008631.
- McIntosh TK, Vink R, Yamakami I, Faden AI. Magnesium protects against neurological deficit after brain injury. Brain Res. 1989;482:252–260. doi:10.1016/0006-8993(89)91188-8.
- Brewer RP, Parra A, Borel CO, Hopkins MB, Reynolds JD. Intravenous magnesium sulfate does not increase ventricular CSF ionized magnesium concentration of patients with intracranial hypertension. Clin Neuropharmacol. 2001;24:341–345. doi:10.1097/00002826-200111000-00005.
- Chutkow JG. Compendium on magnesium and its role in biology, nutrition, and physiology. In: H Siegel, A Siegel, editors. New York: Marcel Dekker; 1990. p. 441–462.
- McCarthy J., Kumar R. Divalent cation metabolism: magnesium. In: Schrier RW, editor. The schrier atlas of diseases of the kidney. Hoboken: Wiley-Blackwell; 2000. p. 1–12.
- Schuette SA, Lashner BA, Janghorbani M. Bioavailability of magnesium diglycinate vs magnesium oxide in patients with ileal resection. J Parenter Enteral Nutr. 1994;18:430–435. doi:10.1177/0148607194018005430.
- Boyle NB, Lawton C, Dye L. The effects of magnesium supplementation on subjective anxiety and stress – a systematic review. Nutrients. 2017;9. doi:10.3390/nu9050429.
- Pyndt Jorgensen B, Winther G, Kihl P, Nielsen DS, Wegener G, Hansen AK, Sorensen DB. Dietary magnesium deficiency affects gut microbiota and anxiety-like behaviour in C57BL/6N mice. Acta Neuropsychiatr. 2015;27:307–311. doi:10.1017/neu.2015.10.
- Grases G, Perez-Castello JA, Sanchis P, Casero A, Perello J, Isern B, et al. Anxiety and stress among science students. Study of calcium and magnesium alterations. Magnes Res. 2006;19:102–106.
- Laarakker MC, van Lith HA, Ohl F. Behavioral characterization of A/J and C57BL/6J mice using a multidimensional test: association between blood plasma and brain magnesium-ion concentration with anxiety. Physiol Behav. 2011;102:205–219. doi:10.1016/j.physbeh.2010.10.019.
- Nair AB, Jacob S. A simple practice guide for dose conversion between animals and human. J Basic Clin Pharm. 2016;7:27–31. doi:10.4103/0976-0105.177703.