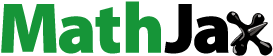
Abstract
Chokeberries, elderberries, blueberries, and blackberries are highly nutritious native fruits in the US Midwest region. Their high moisture content, delicate structure easily leads to fruit loss/waste. This review focuses on different drying methods for whole fruits and juices to preserve their quality and improve their shelf life. Solar drying, hot-air drying, spray drying, freeze-drying, vacuum-drying, electromagnetic drying, and osmotic dehydration are the commonly used dehydrating methods for berries. Berries are photo, heat-sensitive, and rich source of essential nutrients. Texture, flavor, color, water content, phytonutrients, physicochemical properties can be influenced by dryer and processing parameter selection. Drying is a complex dynamic process, due to structural differences among various foods, combined thermal and non-thermal techniques could improve fruit quality. Hence, knowledge of drying behavior and degradation kinetics is vital for optimizing the process parameters to enhance the fruit quality. Freeze drying and spray drying showed better preservation of nutrients. Existing research suggests that chokeberries (Aronia) are underutilized compared to blueberries and blackberries. Aronia fruit has a lot of potentials containing health-promoting compounds and is yet to be explored. Future research suggestions have been put forward for the efficient use of drying techniques and to improve the fruit quality.
1. Introduction
As of 2019, more than 12.2 million tons of berries have been produced globally, and its production has nearly doubled in the last 15 years (6.3 million tons in 2005) (FAOSTAT Citation2021; Zhao Citation2007). The consumption and popularity of berries have grown tremendously in recent years because of their distinctive taste, flavor, color, and abundant phytonutrients. Currently, the berries grown in North America include strawberry, blueberry, raspberry, red and black currants, blackberry, elderberry, and chokeberry (Aronia). Some of these berries such as blueberry, blackberry, elderberry, and chokeberry (Aronia) are cultivated in the Midwest region. However, blueberries and blackberries have been widely commercialized throughout North America. They have well-established market potential and could be found in a range of products - from beverages to supplements to baby foods. On the other hand, chokeberry (Aronia), which is a lesser-known fruit, has received a lot of attention in recent years due to its high health benefits. Hence, we have selected two well-known berries and one underutilized berry that has got a high potential with limited scientific data available. We have summarized the work that could be applied, highlighting the three selected berries. represents the acres harvested for blueberries, blackberries, and chokeberries in the United States.
Figure 1. Acres cultivated for bluberries, blackberries and chokeberries (Aronia) in the United States. Source: USDA, National Agricultural Statistics Service (2021).
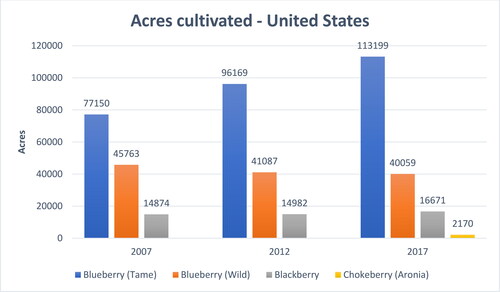
The majority of berries can be eaten as fresh fruits, but cranberries and chokeberries have a tart taste, and hence it is consumed as processed fruit juices, syrups, jams, wines, marmalades, jellies, purees, and ice creams (Tylewicz et al. Citation2018). Sustainable food processing focuses on the prevention of food loss/waste. Reduction of food loss/waste is of prime importance as millions of people suffer from hidden hunger and poverty. Technological strategies such as new product development and/or sustainable food processing/packaging could be used to address food loss/waste of fresh produce. Hence food processing technologies can be adapted for preserving native berries and increasing the shelf life and market potentials for newly developed products.
Blueberries (Vaccinium) belong to the family Ericaceae and is categorized as highbush, lowbush, and rabbiteye varieties. They are distinguished as small, round berries with intense purple color and have a sweet/sour taste. The fruit is indigenous to North America and parts of Europe (Reque et al. Citation2014). The United States is the world’s largest producer of blueberries (308,760 tonnes in 2019) and blueberry has become the second-largest consumed berry after strawberries (FAOSTAT Citation2021; Farneti et al. Citation2017). They are a good source of vitamins, minerals, flavonoids, phenolic acids, anthocyanins, and dietary fiber. It is categorized as one the richest fruits in vitamin C (10 − 100 mg/100 g fresh weight) and antioxidants (8.1 − 38.3 μmol Trolox equivalents (TE)/g fresh weight). shows the nutrient composition of blueberries. Regular intake of blueberries has been linked to preventing and controlling various diseases such as Alzheimer’s disease, aging, mascular degeneration, cardiovascular diseases, diabetes, and even certain cancers (Nile and Park Citation2014; Skrovankova et al. Citation2015; Zhao Citation2007).
Figure 2. Nutrient composition of blueberries in (wetbasis, wb %) (FoodData Central Citation2021).
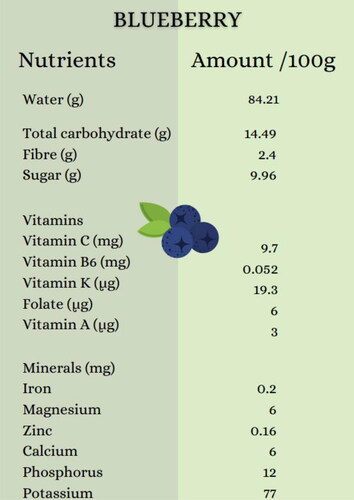
Blackberry (Rubus), belonging to the family of Rosaceae (Rose), is a small shrub composed of several little drupelets around a single seed. The fruit is characterized as soft, juicy and the color varies from bright red-hue to black as they ripen. They have a distinct sweet taste with a tad of sourness. Blackberry is native to Asia and North America stands as the largest producer followed by Europe. The cultivation has also extended to temperate regions of South America, South Africa, and Oceania (Ferrari, Germer, and Aguirre 2012; Zhao Citation2007). Blackberries are usually harvested between late spring and summer. There is a growing popularity among consumers because of their high nutritive value. shows blackberry’s nutritional profile. Among the berry varieties, blackberry has an abundant source of anthocyanins (93 − 280 mg/100g) (Hassimotto et al. Citation2008). The phytochemicals have a health-promoting effect and are found to reduce blood pressure, cholesterol, maintain eye health, prevent aging, cancer, and liver injury (Koner et al. Citation2019; Nogueira et al. Citation2020). Blackberry is a highly perishable fruit because of its high water content (92%), high respiratory rate, and delicate structure, thereby minimizing its consumption and distribution (Nogueira et al. Citation2020). The berries start to decay after 2 − 3 days of harvest even when stored at 0 °C and thus improper post-harvest handling can lead to about 60% production loss (Ferreira Nogueira et al. Citation2018).
Figure 3. Nutrient composition of blackberries in (wetbasis, wb %) (FoodData Central Citation2021).
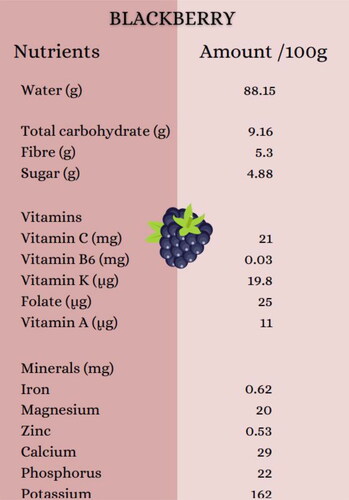
Chokeberry (Aronia) is a deciduous shrub from the family of Rosaceae (Rose) and is classified as Aronia arbutifolia (red chokeberry) and Aronia melanocarpa (black chokeberry). The fruit is indigenous to North America, and its cultivation expanded to Canada, Russia, and Poland. Chokeberry (Aronia) is highly nutritious and has a pleasant flavor. Its nutritional composition is detailed in . Its functional properties find applications in the food and pharmaceutical industries, but its astringency and bitterness limit its consumption as raw fruit. Among the berry groups, including grapes, black chokeberry (Aronia) has the highest content of total polyphenols, and hence its extract is added to dietary supplements (Bednarska and Janiszewska-Turak Citation2020; Tylewicz et al. Citation2018). Due to its strong antioxidant activity, around 3 to 4 times higher than blackberry, raspberry, and blackcurrant, consumption of the fruit has been found to effectively fight against heart diseases, gastrointestinal and muscular disorders, hypertension, inflammation, and diabetes (Gawałek and Domian Citation2020; Petkovic et al. Citation2020).
Figure 4. Nutrient composition of chokeberries in (wetbasis, wb %) (Karakashova et al. Citation2016; Kulling and Rawel Citation2008).
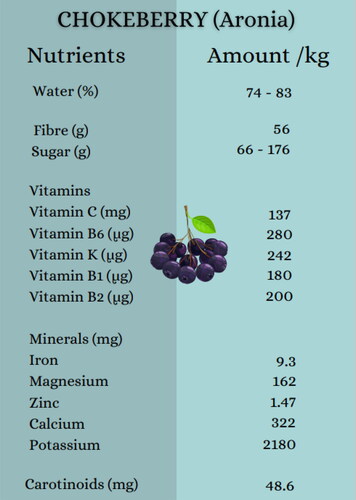
Berries are a potent source to the food and beverage industries due to their unmatched sensorial and nutritional qualities (Farías-Cervantes et al. Citation2020). But they are highly susceptible to mechanical damage because of their fragile structure and are deteriorated quickly. Due to its shorter shelf life and limited seasonal availability, different food processing operations can be widely employed to preserve either as intact/raw fruit or as value-added products. However, the phytochemicals present in the berries are thermal and light-sensitive (Correia et al. Citation2017). Hence the selection of appropriate food processing technologies is essential to preserve its health benefits. Although there are few reviews available on the drying of fruits (Deng et al. Citation2019; Fernandes et al. Citation2011; Karam et al. Citation2016; Sagar and Suresh Kumar Citation2010) there is no recent review focused on preservation techniques of native North American-Indian berries. Therefore, this article focuses on the commonly used food processing methods for blueberries and blackberries, that could be applied to underexplored chokeberries (Aronia), and other native berries like elderberries in the Midwest region. There are excellent reviews available on drying kinetics of agricultural products (Bassey, Cheng, and Sun Citation2021; Li et al. Citation2019; Natesan et al. Citation2020; Nayak, Liu, and Tang Citation2015; Onwude et al. Citation2016). The purpose of this article is to summarize the work that has been carried out on berries from different cultivars and create awareness to all the stakeholders like farmers, producers, and engineers to apply these techniques to native berries in the Midwest region. The theories of drying kinetics, process optimization using the mathematical tool, and the degradation kinetics of berries have also been discussed briefly. Furthermore, possible research gaps are suggested, to further improve the product quality.
2. Dehydrating methods for whole fruits
2.1. Solar drying
Solar drying is one of the ancient methods for preserving agricultural produce and being an inexpensive dehydrating technique, it is still practiced in several developing countries. Commercial food industries use high-tech industrial dryers which require high initial investments. Because of the affordability and easy-to-use solar dryers, this is the preferred method for many small-scale industries (Prakash and Kumar Citation2013). Based on the equipment design and mechanism of energy transfer, solar dryers are classified as (a) direct solar dryers, (b) indirect solar dryers (c) hybrid dryers and (d) mixed-mode dryers (Fudholi et al. Citation2010). shows the illustration of a direct solar dryer. This process has been illustrated to share with the community stakeholders, as a relatively simple technology that can be used by small-scale food processors and producers near the farm. The foremost benefit of using solar dryers is that it uses renewable energy resources. Nevertheless, large-scale drying has a few drawbacks, such as storing energy for off-seasons, and small intensity of solar radiation. Hence, numerous researches have been conducted to advance solar dryers (Bhesh Bhandari Citation2015). Acır, Aktaş, and Danışman (Citation2014) investigated the effect of a novel solar energy collector on the drying efficiency using blueberries as a case study. Samples were dried at different mass flow rates and air temperatures. The highest efficiency was found to be 71.59% for a flow rate of 0.052 kg/s and the moisture content was reduced from 2.18 g H2O/g dry matter to 0.672 g H2O/g dry matter. Arellanes et al. (Citation2017) studied the effect of blanching pretreatment and solar dehydration on the quality parameters of blueberries. Results indicated that blanching pretreatment reduced the time taken to reach 12.5% moisture level by 1.5 hours. The rehydration capacity of berries after blanching was better compared to the non-blanched samples. There were no significant changes in total phenols, total anthocyanins, and total antioxidant capacity based on the pretreatment but on comparing with the fresh samples, dehydration showed a loss of bioactive compounds. The effect of sun drying, oven drying, and freeze drying on the chemical properties of chokeberries were compared by Do Thi and Hwang (Citation2016). Sun-dried chokeberries exhibited a major loss of bioactive and anti-oxidant compounds, because of their long drying time
Figure 5. Schematic diagram of a direct solar drying. Adapted from (Prakash and Kumar Citation2013).
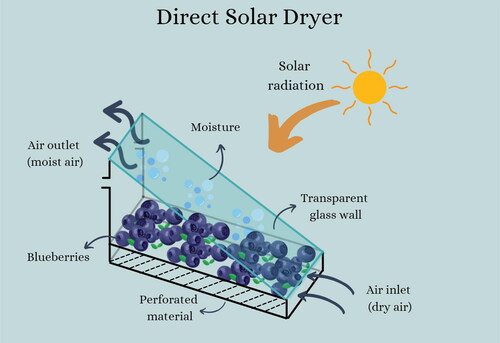
2.2. Convective drying
Hot air drying is one of the most commonly used conventional methods for drying fruits and vegetables. Currently, the batch oven drying method is mostly used among farmers and small-scale processing communities in the Midwest region, and continuous dryers are used by medium to large scale industries which has better throughput. shows the schematic diagram of a batch oven dryer. Berry samples are placed on a tray and preheated air is circulated inside the drying chamber. Firstly, the unbound water from the inner regions of the food material is transferred to the surface by moisture diffusion and surface evaporation takes place. Secondly, heat from the surrounding hot air is transferred from the surface to the center of food material involving simultaneous heat and mass transfer. In continuous dryers, the food material is placed on a conveyor belt which moves continuously through a drying chamber, and hot gas is passed through it. The drying air is passed in co-current, counter-current, or mixed flow mode. The speed of the drying air is adjusted according to the desired drying duration. Although labor and energy requirements are less compared to batch systems, continuous systems require high initial investment and maintenance costs. Even though this technique is widely used for the dehydration of berries, prolonged drying time can result in quality degradation of the final product (Feng, Yin, and Tang Citation2012).
Figure 6. Schematic diagram of Conventional Air Drying – Oven drying. Adapted from (Kassem et al. Citation2011).
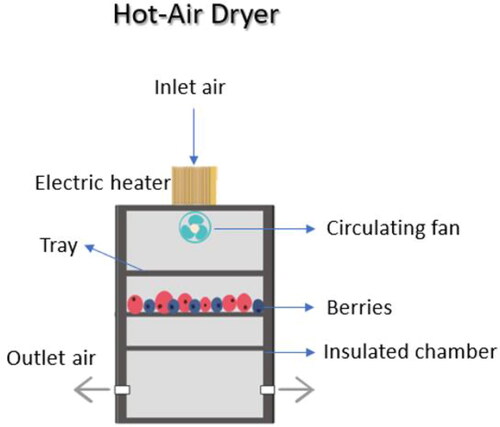
Several studies had been conducted to evaluate the nutritional aspects of oven-dried berries. Değirmencioğlu et al. (Citation2017) found that syringic acid was the dominant phenolic compound and its contents were significantly high in dried blueberries. A large variation in total phenolics and anthocyanin content was observed among fresh and dried blueberries and the antioxidant capacity increased following hot air drying at 50 °C for 2 hours. Yuan et al. (Citation2011) evaluated antioxidant activity, total phenolics, and anthocyanins in blueberry samples of 19 genotypes. Hot air drying at 65 °C for two weeks reduced their contents by 56.6–73.2%, 47.6–69.3%, 38.1–67.4%, respectively, in comparison to fresh samples. Samoticha, Wojdyło, and Lech (Citation2016) conducted oven drying at three different temperatures, time combinations (50 °C for 23 hours; 60 °C for 17 hours; 70 °C for 11 hours) and compared based on the quality parameters of chokeberry (Aronia) fruit. Although samples dried at 50 °C showed the least water activity, prolonged drying durations caused a significant loss in polyphenolic compounds and antioxidant capacity. depicts the correlation between antioxidant activity and total phenolic content. The antioxidant capacity increased with an increase in total phenolics irrespective of anthocyanin content. The interdependence might be because, during drying, some of the anthocyanins could have been converted into phenolic compounds with high antioxidant capacity (Değirmencioğlu et al. Citation2017). Furthermore, the direct relationships between these two parameters could be observed in various studies (Aryal et al. Citation2019; Balasundram, Sundram, and Samman Citation2006; El Jemli et al. Citation2016; Kumar, Sandhir, and Ojha Citation2014; Soong and Barlow Citation2004). Vitamin C is a vital nutrient for humans and berries are a rich source of it. Because of its high sensitivity to heat, drying temperature and time play a significant role in determining its retention capacity. Sadowska, Jadwiga, and Klóska (Citation2017) studied the effect of air-drying (50 °C, for 48 hours) on the vitamin C content of different berries. In black chokeberry (Aronia) and blackberry, their content decreased by 30% and 28% respectively compared to fresh berries. Another study conducted by Nemzer et al. (Citation2018) showed that oven-dried blueberries had a significant loss of vitamin C, total vitamin B, and Pyridoxine (vitamin B6) but retained the maximum amount of riboflavin (vitamin B2) compared to freeze-drying and refractance window drying. The color of the samples was also affected during drying. L* parameter decreased indicating the darkening of product color. shows a comparison of vitamin C retention in blueberries, blackberries, and chokeberries (Aronia). Though chokeberry (Aronia) and blackberry did not have a significant difference, blueberry showed a major loss compared to freeze drying, which could be because of the longer drying time. The effects of blanching pretreatment on the quality parameters of two different blueberry cultivars were investigated by Sablani et al. (Citation2011). The phytochemical contents were better retained after blanching and the effective moisture diffusivity also increased.
Figure 7. Effect of oven drying (at different temperature and time) on total phenolics, anthocyanin content and anti-oxidant capacity (indicated in the secondary vertical label) in chokeberries. Fresh sample contents are also shown for comparison. Data (Samoticha, Wojdyło, and Lech Citation2016).
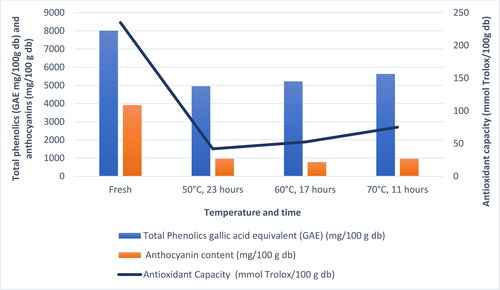
Figure 8. Comparison of oven and freeze drying on vitamin C retention of berries (Chokeberry- Aronia; Blueberry & Blackberry).
Date from: Nemzer et al. (Citation2018) and Sadowska, Jadwiga, and Klóska (Citation2017).
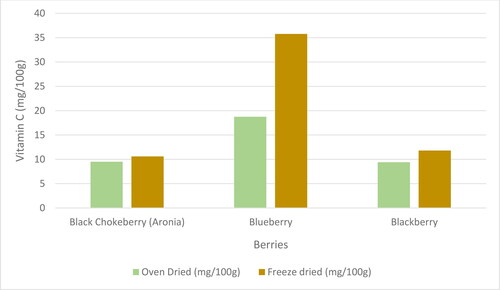
2.3. Vacuum drying (VD)
Vacuum drying is a batch process ideally suited for drying oxygen- and heat-sensitive compounds. Vacuum drying could be considered as a better alternative to hot air drying because of its unique characteristics such as high energy efficiency, less drying time and temperature, and minimal heat damages (Ngamwonglumlert and Devahastin Citation2018; Parikh Citation2015). In the vacuum drying process, the berries are dried under reduced pressure, and heat is transferred either by conduction or radiation. Sumic et al. (Citation2015) optimized the vacuum drying conditions to analyze the influence of drying on phytochemical compounds of blueberries. The results showed that maximum retention of vitamin C, total phenolics, and anthocyanins with minimal color change occurred at a temperature and pressure of 60 °C and 100 mbar. Horszwald, Julien, and Andlauer (Citation2013) evaluated the effects of VD at different temperatures on the physicochemical properties of chokeberry (Aronia) powders. The findings evidenced that increase in drying temperature decreased the nutritional quality of chokeberry (Aronia) powders. Vitamin C is thermally sensitive and is degraded easily by oxidative reactions (Sapei and Hwa Citation2014). The vitamin C content of chokeberry (Aronia) powder obtained by milling followed by vacuum drying was nearly twice that of oven drying (Sadowska et al. Citation2019). Conductive vacuum drying follows the phenomena of surface heat transfer, resulting in a slower drying rate. Hence, vacuum drying is usually combined with microwave drying to increase the drying rate. The properties of the blueberry powder obtained by ultrasonic spray drying and microwave vacuum drying were compared by Candia-Muñoz et al. (Citation2015). Powders produced by ultrasonic spray drying were spherical with a uniform size distribution, and the flowability of powders was better than commercially obtained blueberry powders. With regards to the retention of bioactive compounds and antioxidant capacity, microwave vacuum dried samples showed better conservation than ultrasonic spray drying.
2.4. Freeze drying (FD)
Freeze drying also referred to as lyophilization, is a drying method that follows the phenomenon of sublimation. The freeze-drying process occurs in three stages- freezing, primary drying (ice sublimation), and secondary drying (removal of unbound moisture). In the first stage, berries are frozen rapidly at a very low temperature. In the second stage, the samples are kept in vacuum conditions and sublimation of ice takes place. The final stage begins when there are no ice crystals in the dried product and moisture is removed from the partially bound water. The dehydrated berries, upon rehydration, regain their original characteristics (Berk Citation2013). illustrate the process flow of a freeze dryer, and the various stages in the freeze drying process of blueberries respectively. These figures have been visualized to provide different unit operations that are involved in the freeze-drying process and to explain the underlying mechanism during freeze drying. Although the freeze-drying method is considered an expensive process, it has become a widely accepted preservation technique because of its unmatched benefits over conventional drying. Since the process is operated at a very low temperature undesirable chemical reactions could be inhibited, and several heat-sensitive nutrients could be preserved. Also, the minimal structural changes or shrinkage of the dried product results in better consumer acceptability. Nemzer et al. (Citation2018) reported that freeze-dried blueberries showed less shrinkage whereas air-dried samples had a distorted cell structure. Moreover, a considerably higher glass transition temperature was observed in freeze drying samples indicating a better shelf life of the product at room temperatures than the hot air drying. Sadowska, Świderski, et al. (Citation2017) compared the properties of chokeberry (Aronia) powder obtained by freeze drying and fluidized-bed jet milling with drying (FJMD). The chokeberry (Aronia) powder obtained by freeze drying had maintained its cell structure with a better water holding capacity (812 g H2O/100g dry matter), lower moisture content (3.01%), and lower water activity (0.202) than FMJD. However, the powder obtained by FJMD had retained higher amounts of anthocyanin and thus the powder was lighter with a more intense red and yellow color. Several drying methods such as freeze-drying, microwave vacuum drying, hot air drying, and combined hot air-microwave drying techniques were evaluated by (Mejia-Meza et al. Citation2008; Samoticha, Wojdyło, and Lech Citation2016) on the physiochemical properties of chokeberries and blueberries. The results showed that freeze drying was the most effective technique in preserving the total phenolics, anthocyanins, and antioxidant activity. represents the comparison of vitamin C retention between freeze drying and hot air drying in different berries. Vitamin C is a heat-sensitive nutrient; is better preserved in drying processes where the operating temperature is kept very low (Sadowska, Jadwiga, and Klóska Citation2017; Nemzer et al. Citation2018). A study conducted by Sablani et al. (Citation2011) manifested that freeze-dried blueberries had an increase in antioxidant capacity by 1–36% (dry matter) than fresh berries. The high antioxidant capacity in berries after drying might be because partially oxidized polyphenols can have a better antioxidant capacity than non-oxidized polyphenols (López-Nicolás and García‐Carmona Citation2009). Additionally, this increase might be associated with the formation of Maillard reaction products (MRPs) (Kamiloglu and Capanoglu Citation2015). Generally, vacuum freeze dryers are preferred because of their high product quality. But the major drawback is its high operating cost; associated with the longer drying time. Besides, freeze drying is mostly performed as a batch process which incurs additional expenses. The cost of freeze drying is two to five times higher than oven drying and nearly six times higher than spray drying (Bhandari et al. Citation2013). The major contributor to increased operating costs is high energy consumption. In conventional freeze dryers, heat is transferred from the conduction trays to the product from only one side resulting in slower drying. To overcome this drawback, infrared or microwave-assisted vacuum freeze-drying can be implemented which yields similar product quality as traditional freeze drying. The use of alternative energy sources aids in rapid heat dissipation through the product, thereby increasing the rate of heat and mass transfer. Reyes et al. (Citation2011) explored the impact of infrared assisted vacuum or conventional freeze drying on functional properties of blueberries. The use of infrared radiation significantly decreased the drying time. The final moisture content was reduced below 0.1 in less than 12 hours of drying time. A mathematical model has been proposed by Stratta et al. (Citation2020) to approximate the total cost of a freeze-drying cycle and to examine the factors that contribute to the operating and capital expenses. The study illustrates that the initial investment (raw materials and factory utilities) rather than the freeze-drying process is the prime factor for the increased production cost. Since the operating cost is extremely small (9%) compared to the capital cost (91%) for a 7-day working basis, the optimized conditions would be to run more production cycles within the lifespan of the equipment. In this way, the capital costs will reduce while the production efficiency will be improved. Application of freeze drying on blueberries, blackberries and chokeberries (Aronia) has been summarized in .
Table 1. Application of freeze drying for blueberries, blackberries and chokeberries (Aronia).
2.5. Osmotic dehydration (OD)
Osmotic dehydration is a simultaneous mass transfer process that is used for the removal of water from fruits/vegetables. When the material is immersed in an aqueous solution (sugar or brine), the water moves from a region of low concentration solute (food material) to a region of high concentration (hypertonic solution) through the cell wall membrane until an equilibrium is reached. The movement of water and solute molecules takes place due to the difference in osmotic pressure. Osmotic dehydration can also be used as a prior treatment to normal drying as it reduces 30 − 70% moisture content, reduces heat damages, preserves color and flavor, and minimizes energy costs (Akbarian, Ghasemkhani, and Moayedi Citation2013; Yadav and Singh Citation2014). However, due to the mechanical resistance of cell tissues to mass flow, a longer drying time is required to reduce the moisture content (Zielinska and Michalska Citation2016). Hence before osmotic dehydration, different treatments such as pulsed electric field (PEF), vacuum, high hydrostatic pressure, blanching, gamma irradiation can be applied to promote permeability of the cell membrane (Selvi, Gurunathan, and Singh Citation2014). Fresh blueberries were subjected to pulsed electric field treatment before osmotic dehydration and their effects on nutritional quality were studied by Yu, Jin, Fan, et al. (Citation2017). The results showed that pretreatment significantly reduced the drying time from 120 h to 48 h without negatively affecting its nutritional and microbiological qualities. The influence of different pretreatments (immersion in boiling water and NaOH, ultrasound treatment, and enzymatic immersion) on the polyphenol and dry matter content of blueberries were investigated by Kucner, Klewicki, and Sójka (Citation2013). It was reported that fruit immersed in pectinolytic and lipolytic enzyme solutions gave the desired results. The dry matter content increased to 26% and almost 96% of the phenolic contents were retained.
2.6. Alternative drying methods
Unlike conventional drying methods which use an electric heater or exhaust gas to transfer heat to the food product, electromagnetic field-based drying uses an electromagnetic spectrum to induce thermal energy in the food material. Electromagnetic drying includes microwave, infrared, and radiofrequency drying. These methods are novel emerging technologies, developed to improve the drying process. Various studies have been conducted in drying blueberries, blackberries, and chokeberries using microwave and infrared techniques.
Microwave (microwave-assisted) drying uses frequency in the range of 0.3 GHz to 3 GHz with the most common frequency being 915 MHz (for industrial application) and 2450 MHz (for home scale applications). This technique has received a lot of attention in recent years because of higher drying rates, more energy efficiency, and uniform heating (Sutar and Prasad Citation2008; Wray and Ramaswamy Citation2015). The drying process is based on volumetric heating, where the water molecules present in the food act as electric dipoles. As the electromagnetic waves, generated by the magnetron, passes through wet material, the bipolar molecules rotate to align with the alternating electric field. This rapid molecular movement creates friction, thereby generating heat in the food material. However, it is difficult to control the heat and drying rate, and hence, microwave drying is usually combined with other techniques to prevent product damage (Joardder, Karim, and Kumar Citation2013). Hot air combined with microwave drying preserved the color and showed less shrinkage when compared to other pretreatments in blackberry samples. The least color change (ΔE = 12.15) and shrinkage (28.12%) was observed at 360 W with an inlet air temperature of 70 °C (Kaveh, Taghinezhad, and Aziz Citation2020). Rodriguez et al. (Citation2016) compared microwave drying with two different pretreatments (osmotic dehydration and hot air drying) along with control drying (hot air drying) in blueberry samples. Hot air-microwave drying showed a better drying rate and had a shorter drying time when compared with osmotic-microwave drying. The firmness and stiffness of blueberries decreased with increasing microwave power irrespective of the pretreatments. Blueberries dried at 622.5 W and 750 W were softer and less firm than at 562.5 W. Significant changes in h and a* values were observed for both processes. Samples pretreated with hot air drying (blue coloration) showed desired color characteristics than osmotic pretreatment (purple-reddish). However, the lightness of samples was not significantly affected for both the pretreatments. Both the drying process had a major impact on the rehydration capacity of blueberry samples. However, hot air-microwave drying showed 20% more rehydration capacity than controlled drying but the rehydrated samples were less firm and stiff when compared with natural berries. In a study conducted by Calín-Sánchez et al. (Citation2015), chokeberries dried by microwave-vacuum had a better drying rate and exhibited better sensory (less sour, bitter, and astringent taste) and microstructural (low hardness, bulk density, and high porosity) properties.
In infrared (IR) drying, the IR radiation directly penetrates the food product to a few depths and heats the product without heating the surrounding air. This technique has several advantages over conventional methods such as faster drying rate, uniform heating, proper control over the product temperature, and better preservation of product quality (Huang et al. Citation2021). Shi et al. (Citation2008) compared IR drying of fresh and sugar-infused blueberries with hot air drying. The drying temperatures did not make any significant effect on the texture and color of the samples dried by IR. IR-sugar-infused blueberries had better textural properties than hot air-dried berries and the dried blueberries exhibited darker, redder, and less blue color compared to the fresh samples. Sometimes, IR is not suitable for heat-sensitive nutrients. Hence it is combined with other techniques for further improvement of the drying process. Taghinezhad et al. (Citation2021) investigated the combined effects of hot air-infrared and ultrasound pretreatment. The total color change (ΔE) decreased with the increase of ultrasound pretreatment time and inlet air temperature. The lowest color change (ΔE = 17.99) was observed at 70 °C and for a pretreatment time of 45 minutes.
2.7. Hybrid drying
For decades, conventional drying has been widely employed for the drying of fruits and vegetables. However, there are a few limitations such as poor product quality, increased drying time, and low energy efficiency (Li et al. Citation2019). Hence novel drying techniques that minimize these drawbacks have increased the attention in the past few years. Hybrid drying technology has developed a synergetic effect as it combines the benefit of several individual drying and this could therefore be considered a better alternative to conventional drying. Several combinations such as microwave – vacuum, infrared – freeze-drying, hot air – microwave, hot air – infrared, hot air – freeze-drying have been developed by various researchers and the results had proven that hybrid drying is cost-effective, with improved product quality and drying efficiency (Chen et al. Citation2017; Hnin et al. Citation2019).
Moreno et al. (Citation2016) evaluated the effect of ohmic heating and pulsed vacuum as a pretreatment for osmotic dehydration of blueberries. Compared to the untreated samples, the treated samples showed improved mass transfer rates with high polyphenol retention. Zielinska, Sadowski, and Błaszczak (Citation2016) combined hot air and microwave drying on blueberry samples and found that the combined technology had significantly improved the fruit quality and the process was also more economical. Case hardening, slow drying rate, loss of flavor, aroma, and bioactive compounds are some of the major disadvantages of hot air drying (Li et al. Citation2019). But microwave drying combined with hot air drying drastically reduced the drying time and retained the quality attributes of the blueberry pomace as well (Zielinska and Michalska Citation2018).
Properties such as color and rehydration ratio are important factors to access the quality of dried food materials. Color plays a vital role in consumers’ acceptance of the product and a high rehydration capacity means the dried food material is of good quality. shows the impact of various drying on the color and rehydration ratio of blueberries, blackberries, and chokeberries (Aronia).
Table 2. Impact of drying on optical properties and rehydration potential of blueberries, blackberries and chokeberries (Aronia).
3. Dehydrating method for juices
3.1. Spray drying (SD)
Spray drying is one of the frequently implemented encapsulating techniques to preserve the bioactive compounds having therapeutic properties. The spray drying process involves four fundamental stages: (1) atomization, (2) droplet air contact, (3) droplet drying, (4) separation. depicts the process flow of a spray dryer. The liquid feed is first atomized through the spray nozzle as small droplets or mist. Preheated air is circulated inside the drying chamber, and as the fine spray comes in contact with the hot air, moisture is evaporated rapidly. The particles are passed on to the cyclone separator where the gas stream is removed through the exhaust vent and the final product will be collected at the bottom. The particle morphology substantially impacts the physical characteristics such as reconstitution properties and flow behavior of the powder (Both, Boom, and Schutyser Citation2020). The size and shape of powder particles largely depend on the type of encapsulation technique implemented (physical method – microspheres; chemical method – microcapsules) (Quintero et al. Citation2017). shows the spray drying mechanism to convert berry juice into berry powder and similar mechanism forms as the basis for encapsulation of liquid formulations with berry matrix to create morphologies at micro/nano scale level (Krishnaswamy and Orsat Citation2017). The properties of the spray-dried powder can be typically altered by the design and fabrication of the spray dryer, the liquid feed characteristics, and the process parameters. outlines the impact of spray drying process parameters on the final product quality. Of all the parameters, drying air temperature is the crucial factor influencing the powder properties. An increase in inlet air temperature increases the product yield, particle size, solubility and decreases the moisture content and bulk density (Phisut Citation2012; Shishir and Chen Citation2017). Ferrari, Germer, and de Aguirre (Citation2012) compared the effects of inlet temperature and maltodextrin concentration on the physicochemical properties of blackberry powder. An increase in inlet temperature (140 − 180 °C) decreased the moisture content but increased the particle size and the loss of bioactive compounds caused by thermal degradation. The blackberry powder color was negatively affected by the addition of the carrier agent. The powders became paler with the increased inclusion of maltodextrin due to its intrinsic whitish color.
Figure 12. Influence of spray drying operating conditions on powder characteristics. Data from: (Phisut Citation2012; Shishir and Chen Citation2017).
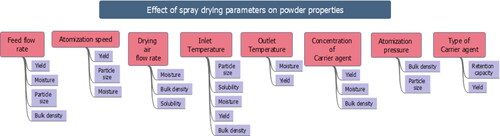
3.1.1. Recent applications in spray drying with bioactive compounds
Dietary patterns have changed across the globe over the years, and in recent years, consumer understanding of eating functional foods has risen tremendously. Because of the consumer’s willingness to consume food with health-promoting compounds, industries are largely focusing on innovative products incorporated with essential nutrients (vitamins, minerals, and phytochemical compounds) (Ali and Rahut Citation2019; Jafari and McClements Citation2017). But direct inclusion of these health-promoting ingredients in food and beverages is quite challenging because of their instability and degradation on exposure to oxygen and heat. Hence encapsulation of nutrients could be the best approach to overcome these drawbacks (Sharif, Khoshnoudi-Nia, and Jafari Citation2020). Among the different encapsulation techniques (i.e., spray drying, liposome entrapment, coacervation, nano emulsions, extrusion), spray drying has been widely implemented for several decades (Yamamoto et al. Citation2012).
One of the major challenges in spray drying of fruit juices is the tendency of powders to form lumps, caking, or stickiness on the walls of the drying chamber which ultimately decreases the product yield (Shishir and Chen Citation2017). This is because fruit juices mainly consist of sugars (glucose, sucrose, fructose) and organic acids (citric, malic acids) which have low molecular weight and glass transition temperature (Tg) (Ruiz-Cabrera and Schmidt Citation2015; Viljakainen, Visti, and Laakso Citation2002). To overcome this issue, carrier agents such as carbohydrate polymers (starch, maltodextrin, gums, pectin, chitosan, cellulose, and derivatives), lipids (wax, paraffin), and proteins (caseins, soy protein, wheat gluten, whey protein) are blended with the fruit extract. The encapsulating agents chosen must meet some of the fundamental criteria such as high molecular weight, Tg above 100 C, and high solubility. Most commonly, carbohydrate polymers are preferred because they are inexpensive when compared to proteins and lipids (Gawałek and Domian Citation2020). Alternatively, for sugar and acid-rich materials, a modified spray dryer (dehumidified spray drying system) could also be used, in which a dehumidifier is connected to the inlet air. This could result in a better product yield and powder properties (Goula and Adamopoulos Citation2005). Several authors have examined the effectiveness of different carrier materials on the physicochemical properties of the powder. Wilkowska et al. (Citation2017) compared HP-β-cyclodextrin (HP-CD), maltodextrin (MD), and a blend of Arabic gum with maltodextrin (AG + MD) as the carrier material on the storage stability of antioxidants and polyphenols in chokeberry (Aronia) juice and wine. The findings evidenced that the type of wall material had a remarkable effect on the microstructures, encapsulation efficiency, and stability of bioactive compounds. HP-CD encapsulated powder showed the smallest impact on the loss of antioxidant capacity under all storage conditions. Furthermore, encapsulation of wine polyphenols exhibited better antiradical activity than juice polyphenols. Spray drying has been the frequently implemented method for encapsulation followed by freeze-drying (Sharif, Khoshnoudi-Nia, and Jafari Citation2020). This method has the edge over freeze drying because of its scalability and cost-effectiveness. Despite its benefits, spray drying, however, solely pertains to only thermally stable nutrients (Rezvankhah, Emam-Djomeh, and Askari Citation2020). This fact corroborates with the study conducted by Franceschinis et al. (Citation2014). Although spray-dried blackberry powder showed better physical stability, freeze-dried formulations showed the least loss of bioactive compounds and exhibited the strongest antioxidant activity. This can be attributed to the high product temperatures in the spray drying process. However, the findings of Horszwald, Julien, and Andlauer (Citation2013) debunks the fact that spray drying is limited to thermo-sensitive compounds. Spray-dried chokeberry (Aronia) powder retained the highest content of bioactive compounds compared to freeze drying and vacuum drying because of the shorter drying time. shows the recent applications of various carrier types on blueberries, blackberries, and chokeberries.
Table 3. Application of different carrier material for spray drying of blueberries, blackberries and chokeberries (Aronia).
Conventional spray dryers use atomizers that rely on mechanical energy to shear the liquid have a few shortcomings such as broad droplet size distribution, inability to control the velocity of particle and droplet size, and increased likelihood of orifice clogging. Such shortcomings could be overcome by an ultrasonic spray nozzle that uses minimal vibrational energy to atomize the fluid and produces more uniform, spherical particles with smaller droplet sizes (Gogate Citation2015; Shishir and Chen Citation2017). The influence of conventional and ultrasonic-assisted spray drying was compared by Tatar Turan et al. (Citation2016). Powders produced by ultrasonic atomizer showed higher encapsulation efficiency, with uniform size distribution, and exhibited better retention of phytochemical compounds than the conventional nozzles.
4. Drying kinetics and mathematical modeling
Drying is an essential unit operation for the food industries during post-harvest handling to reduce moisture and provide adequate storage conditions. There are more than hundreds of dryers available intended for various uses (Klemeš, Smith, and Kim Citation2008). Each dryer works on different principles based on the modes of heat and mass transfer. Hence it is vital to choose an appropriate drying technique considering various factors such as final product quality, desired moisture level, energy consumption, and capital cost. Moreover, because of the variations in structure and composition of different fruits and vegetables, the measurement of process parameters during drying becomes a challenge (Castro, Mayorga, and Moreno Citation2018). Hence in such cases, mathematical modeling can be an important tool that helps to predict the behavior of a process under different conditions. Various studies have been conducted to develop models and predict the drying kinetics of fruits, vegetables, and sea products using different dryers (Afolabi and Agarry Citation2014).
4.1. Thin-layer drying
Thin-layer drying has been frequently used for several food materials to optimize the drying time (Kadam, Goyal, and Gupta Citation2011). It is to dry a single layer of the product with constant width. Thus, the temperature profile can be assumed as uniform, and lumped parameter models can be applied (Onwude et al. Citation2016). Thin-layer drying equations are classified as (a) theoretical, (b) semi-theoretical, and (c) empirical. Theoretical equations are deduced from Fick’s second law of diffusion and it is based on both internal and external resistance to mass transfer. Although theoretical models can be used to predict the drying kinetics, too many assumptions on the diffusion mechanism led to increased errors. On the other hand, semi-theoretical models are sub-classified as Newton’s law of cooling and Fick’s second law based on their derivations. Semi-theoretical and empirical models consider external resistance to mass transfer and they are widely employed as they both depend on experimental data and thus include lesser assumptions (Erbay and Icier Citation2010; Kucuk, Kilic, and Midilli Citation2014; Onwude et al. Citation2016). The classification of thin-layer models has been illustrated in . For most of the agricultural produce, Lewis, Page, Midilli–Kucuk, Wang and Singh, Henderson–Pabis, Two Term, modified Page, logarithmic, Thompson, and modified Henderson–Pabis models were successful in predicting the drying behavior (Aregbesola et al. Citation2015; Vega-Galvez et al. Citation2012).
To understand the drying characteristics of a material, a drying curve is obtained. The drying curve is different for different food materials and it is based on the drying conditions such as velocity, temperature, and pressure. The drying curve can be described in 4 stages (). Critical moisture is the point at which the constant drying rate period ends and the falling drying rate period starts. The constant drying rate period is mostly dependent on the rate of evaporation from the food material. This could be improved by increasing the inlet air temperature and contact time. The falling drying rate period is based on moisture diffusion from the food material and this can be improved by increasing the material’s temperature which increases the diffusivity. To predict the drying behavior of food materials, it is vital to precisely choose a model that has a reasonably good fit of the experimental data. Different food products such as cereal grains, dehydrated fruits, and vegetables have different moisture content. Grains with too much moisture can deteriorate easily, dehydrated fruits and vegetables are at increased risk of browning, and egg powders are subject to increased risk of oxygen absorption (Pomeranz and Meloan Citation1994). Hence, a knowledge of moisture content is important as it plays a critical role in the processing, handling, storage, and quality of the product. The moisture ratio is calculated according to EquationEquation (1)(1)
(1) .
(1)
(1)
– Dimensionless moisture ratio;
– equilibrium moisture content;
– moisture content at any time t;
– initial moisture content.
A plot of vs t is obtained for the chosen models and by using regression analysis, the model coefficients/constants (a, b, c, n), drying constants (k), and regression coefficient (R2) are determined. Root mean square error (RMSE), reduced chi-square (
) and regression coefficient (R2) are some of the statistical parameters used to validate the selected models. (R2) is considered as a crucial parameter for model validation and generally, a high R2 and low value of
RMSE are preferred (Eminoğlu, Yegül, and Sacilik Citation2019; Erbay and Icier Citation2010; Kiliç and Çinar Citation2019).
Zielinska and Markowski (Citation2016) investigated the influence of microwave–convective drying on the rehydration properties of blueberries. Convective pre-drying resulted in effective drying and rehydration rate. Mathematical models were used to study the drying kinetics of moisture gain and solid loss of blueberries. Based on the statistical results, the Peleg model and first-order kinetic model were found to be a good fit to describe rehydration behavior. Song et al. (Citation2020) studied the effects of osmotic dehydration pretreatment combined with microwave vacuum drying on the phytochemical properties of blueberries. The mass transfer process during osmotic dehydration was effectively described by a two-parameter mathematical model. The validation of the model was corroborated by regression analysis which showed high values of R2 (>0.96). Several researchers have simulated drying curves for different dehydrating techniques on blueberries, blackberries, and chokeberries which are detailed in . For blueberry samples, different models were found to best describe the drying characteristics of convective drying. For convective drying at different temperatures: 30, 40, and 50 °C, the page model showed the best fit but when the temperature was raised to 70 °C and combined with different pretreatments Weibull and modified Henderson – Pabis model best described the drying curve. Furthermore, when a microwave component was added, R2 was greater than 0.97 for Linear Model and Henderson – Pabis model. To improve the drying process and product quality, pretreatments are combined with the drying process. This approach will influence the drying kinetics directly and so different models were found to have the best fit. For the blackberry samples dried by solar drying, Midilli–Kuçuk model and Page model best described the drying behavior. This difference could be attributed to the change in air velocity and temperature during drying.
Table 4. Recent applications of thin layer models to describe the drying kinetics for blueberries, blackberries and chokeberries (Aronia).
4.2. Need for process optimization
Drying is a complex interactive dynamic process with several processing parameters that can impact the quality, shelf life, and microbial safety of the product (Patil, Chauhan, and Singh Citation2014). Previously, to optimize such a process, a one-factor-at-a-time approach was used. This optimization was laborious as a series of experimental trials were needed and it failed to consider the interactive effects between various factors (Shishir and Chen Citation2017). Different statistical tools such as full-factorial design and response surface methodology can be used for process optimization. However, the major drawback of the full-factorial design is the difficulty in interrupting and experimenting with more factors and variables. In such cases, response surface methodology could be an efficient model that could analyze and relate the influence of multiple variables using experimental data (Anwar et al. Citation2016). RSM is desirable over other optimization methods because the number of data required to design, analyze and optimize decreases the trials needed (Krishnaiah et al. Citation2015). The general equation of RSM can be expressed by EquationEquation (2)(2)
(2) (Mumtaz et al. Citation2017).
(2)
(2)
y – response variable, x1 and x2 – independent variable, e – experimental error
The two important models of RSM which are currently used are the first-order model and the second-order model. First-order designs include – 2k factorial, Plackett–Burman, and simplex designs (Khuri and Mukhopadhyay Citation2010). The first-order model is usually employed at the beginning of trials to determine the important parameters that influence the drying process. It is mathematically expressed in the form of a linear Equation (3)
– constant,
– Regression coefficients, xi – independent variable, e – experimental error
If the response surface has a curvature, then the second-order model is used to identify the key parameters in the experiment, and then to evaluate and analyze its significance, and finally reach an optimized condition. The mathematical expression of the second-degree model is given by Equation (4) (Khuri Citation2017).
– constant,
– Linear, cross product, and quadratic regression coefficients, xi, xj – independent variable, e – experimental error. The regression coefficients are obtained by the least square method.
Central Composite Design (CCD), 3k factorial, and Box Behnken Design (BBD) are the most widely used second-order designs (Khuri and Mukhopadhyay Citation2010). However, CCD is more robust with high accuracy compared to the other methods and it is categorized into three types – Circumscribed, inscribed, and face-centered (Islam Shishir et al. Citation2016).
The CCD generally comprises of 2k factorial design and each variable is evaluated at two levels and the factors are coded at one level high (+1) and one level low (−1) for the corresponding variable. It also consists of a 2k axial runs – two points are considered at a distance α on the axis for each control parameter and nc center points (determines the number of replicates needed at the center). The experimental data are collected and fit into the polynomial equations to evaluate the regression coefficients, predict the response and finally, the adequacy of the model is checked using statistical analysis such as lack of fit, prediction error sum of squares (PRESS), R2, adjusted and predicted R2, and adequate precision. R2 closer to unity is considered a good fit. Analysis of variance (ANOVA) is performed to study the interaction among multiple parameters and its response and statistical significance test must be performed at a 95% confidence level (Behera et al. Citation2018; Islam Shishir et al. Citation2016; Khuri Citation2017; Khuri and Mukhopadhyay Citation2010). The main advantage of using RSM is that it can be used before the experiment to predict the response of a process that enables sustainably obtaining a high product quality without any overuse of raw materials (Anwar et al. Citation2016).
4.3. Degradation kinetics
Berries are a rich source of antioxidants, vitamins, mineral, and because of their seasonal availability and perishability, their everyday consumption is minimal (Sarkis et al. Citation2013). Several factors such as oxygen, light, temperature, and enzymes could cause degradation of bioactive compounds but amongst this, the temperature is the main affecting factor. However, one of the commonly employed techniques for preservation and extension of shelf life is thermal processing. Hence, knowledge of degradation kinetics is paramount to preserve the nutritional value during processing (Kechinski et al. Citation2010). The degradation kinetics for bioactive compounds such as polyphenols, vitamins, minerals can be characterized by using zero-order (EquationEquation 5(5)
(5) ), first-order (EquationEquation 6
(6)
(6) ), or Weibull model (EquationEquation 7
(7)
(7) ) and the dependency of reaction rate constant with temperature can be studied by using Arrhenius equation (EquationEquation 8
(8)
(8) ), Ball model (EquationEquation 9
(9)
(9) ) and temperature coefficient (Q10) (EquationEquation 10
(10)
(10) ). Thermodynamic functions can also be used to describe the temperature dependency of anthocyanin degradation. This is given by the Eyring model (Equation 11) (Martynenko and Chen Citation2016; Sarkis et al. Citation2013).
Zero-order model
(5)
(5)
First-order model
(6)
(6)
Weibull model
(7)
(7)
Arrhenius equation
(8)
(8)
Temperature coefficient (Q10) model
(9)
(9)
Eyring model
(10)
(10)
– Degradation rate constant (min−1);
– Rate constant at T0; Ct – Concentration at time t; C0 – Initial concentration;
– activation energy; n – reaction order; R – Gas constant; ΔH - activation enthalpy; ΔS – activation entropy; h – Planck’s constant.
Various studies have been conducted to analyze the stability of anthocyanins upon exposure to heat. Celli et al. (Citation2016) studied the degradation kinetics of freeze-dried blueberry powder. The anthocyanin degradation followed first-order kinetics. The Arrhenius model and thermodynamic functions were used to determine the mechanism of anthocyanin degradation. A positive ΔH has been obtained which implies that the degradation rate increases with temperature and the change in degradation rate for a 10 rise in temperature were found to be in the range of 1.77 − 3.11. shows some of the recent research on the degradation kinetics of anthocyanins and vitamins in blueberries and blackberries. However, no research has been done for chokeberries and this is an under-explored native fruit that has a lot of potentials.
Table 5. Degradation kinetics of vitamins and anthocyanins in blueberries and blackberries during drying.
5. Conclusion and future scope
Berries are filled with nutritional value, and it is considered as one of the healthiest foods that could be incorporated into our everyday diet. With their appealing colors, flavors, and functional properties, consumer’s demand for berries is increasing rapidly. However, berries are seasonal fruits, and they are highly prone to microbial spoilage and thus have a shorter shelf-life. This limited supply of the market makes it difficult to consume nearly every day. Hence this paper reviews different dehydrating techniques for preserving blueberries, blackberries, and chokeberries. By employing a suitable dehydrating method, the quality factors such as texture, aroma, color, taste, and nutritive value could be preserved. Although conventional drying methods preserve the product quality there are few limitations. For instance, solar drying needs a longer drying time and requires high initial investments. For hot air drying, the longer drying period degrades the nutritional quality, and for vacuum drying, a high production cost is needed. Hence, a combination of thermal and non-thermal techniques such as the osmotic dehydration assisted oven/vacuum drying can be used that minimizes these drawbacks. Electromagnetic drying has received considerable attention in recent years because of its high energy efficiency and low drying time. However, scaling up of these techniques is difficult and their full potential is yet to be explored. Compared to the other drying techniques, freeze-drying and spray drying showed the best preservation of phytochemical properties. But considering the affordability, ease of operation, production scaling, and material handling, spray drying could be considered as a better alternative to freezing drying. Studies have shown that spray drying is very effective in encapsulating micronutrients which can be further used to fortify foods. To the best of our knowledge, no research has been conducted on the incorporation of vitamins and minerals into berries which could make it a value-added functional fruit. The majority of the studies conducted on spray drying of blueberries, blackberries, and chokeberries, did not include data on the efficiency of encapsulation, which is an important parameter to determine the stability of heat-sensitive nutrients. Evidence on encapsulation efficiency could be useful to analyze and effectively choose appropriate encapsulating material. Mathematical modeling is an important tool to study the behavior of a process, the degradation kinetics, and to optimize the process parameters to achieve maximum product quality. Although research has been done to understand the degradation kinetics of active ingredients in blueberries and blackberries, no work has been conducted for chokeberries. More research on this is essential for the appropriate selection of drying methods and to minimize the loss of health-promoting compounds of this native North American berry. In the past decade, very few studies have focused on the optimization of spray drying parameters using the RSM tool for blueberries and blackberries while no such research has been carried out for chokeberries. Hence, future studies could emphasize these research gaps to further improve the product quality, prevent food loss and develop novel value-added products from native North American berries.
Author contributions
Karishma Sri Ravichandran: Investigation, Writing-Original Draft, Visualization. Kiruba Krishnaswamy: Conceptualization, Resources, Visualization, Writing – Review & Editing, Supervision, Project administration, Funding acquisition.
Disclosure statement
The authors declare no competing interest.
Additional information
Funding
References
- Acır, A., M. Aktaş, and F. Danışman. 2014. Investigation of drying characteristics of blueberry using a new solar air collector design. In Progress in exergy, energy, and the environment, ed. I. Dincer, A. Midilli, and H. Kucuk, 695–701. New York: Springer International Publishing. doi: 10.1007/978-3-319-04681-5_66.
- Afolabi, T., and S. Agarry. 2014. Mathematical modelling and simulation of the mass and heat transfer of batch convective air drying of tropical fruits. Chemical and Process Engineering Research 23:9–18.
- Akbarian, M., N. Ghasemkhani, and F. Moayedi. 2013. Osmotic dehydration of fruits in food industrial: A review. International Journal of Biosciences 3:1–16. doi: 10.12692/ijb/4.1.42-57.
- Ali, A., and D. B. Rahut. 2019. Healthy foods as proxy for functional foods: Consumers’ awareness, perception, and demand for natural functional foods in Pakistan. International Journal of Food Science 2019:6390650–12. doi: 10.1155/2019/6390650.
- An, K., M. Fu, H. Zhang, D. Tang, Y. Xu, and G. Xiao. 2019. Effect of ethyl oleate pretreatment on blueberry (Vaccinium corymbosum L.): Drying kinetics, antioxidant activity, and structure of wax layer. Journal of Food Science and Technology 56 (2):783–91. doi: 10.1007/s13197-018-3538-7.
- Anwar, K., K. Anwar Mohamad Said, M. Afizal, and M. Amin. 2016. Overview on the response surface methodology (RSM) in extraction processes. Journal of Applied Science & Process Engineering 2 (1):8–17. doi: 10.33736/jaspe.161.2015.
- Araujo-Díaz, S. B., C. Leyva-Porras, P. Aguirre-Bañuelos, C. Álvarez-Salas, and Z. Saavedra-Leos. 2017. Evaluation of the physical properties and conservation of the antioxidants content, employing inulin and maltodextrin in the spray drying of blueberry juice. Carbohydrate Polymers 167:317–25. doi: 10.1016/j.carbpol.2017.03.065.
- Aregbesola, O. A., B. S. Ogunsina, A. E. Sofolahan, and N. N. Chime. 2015. Mathematical modeling of thin layer drying characteristics of Dika (Irvingia gabonensis) nuts and kernels. Nigerian Food Journal 33 (1):83–9. doi: 10.1016/j.nifoj.2015.04.012.
- Arellanes, N., P. Benito, M. E. Pérez, B. H. Zárate, and M. G. Alonso. 2017. Solar dehydration of blueberries (Vaccinium corymbosum L.). Acta Horticulturae 1180 (1180):491–6. doi: 10.17660/ActaHortic.2017.1180.69.
- Aryal, S., M. K. Baniya, K. Danekhu, P. Kunwar, R. Gurung, and N. Koirala. 2019. Total phenolic content, flavonoid content and antioxidant potential of wild vegetables from Western Nepal. Plants 8 (4):96. doi: 10.3390/plants8040096.
- Balasundram, N., K. Sundram, and S. Samman. 2006. Phenolic compounds in plants and agri-industrial by-products: Antioxidant activity, occurrence, and potential uses. Food Chemistry 99 (1):191–203. doi: 10.1016/j.foodchem.2005.07.042.
- Barba, F. J., H. Jäger, N. Meneses, M. J. Esteve, A. Frígola, and D. Knorr. 2012. Evaluation of quality changes of blueberry juice during refrigerated storage after high-pressure and pulsed electric fields processing. Innovative Food Science & Emerging Technologies 14:18–24. doi: 10.1016/j.ifset.2011.12.004.
- Bassey, E. J., J.-H. Cheng, and D.-W. Sun. 2021. Novel nonthermal and thermal pretreatments for enhancing drying performance and improving quality of fruits and vegetables. Trends in Food Science & Technology 112:137–48. doi: 10.1016/j.tifs.2021.03.045.
- Bednarska, M. A., and E. Janiszewska-Turak. 2020. The influence of spray drying parameters and carrier material on the physico-chemical properties and quality of chokeberry juice powder. Journal of Food Science and Technology 57 (2):564–77. doi: 10.1007/s13197-019-04088-8.
- Behera, S. K., H. Meena, S. Chakraborty, and B. C. Meikap. 2018. Application of response surface methodology (RSM) for optimization of leaching parameters for ash reduction from low-grade coal. International Journal of Mining Science and Technology 28 (4):621–9. doi: 10.1016/j.ijmst.2018.04.014.
- Berk, Z. 2013. In Food process engineering and technology, 2nd ed., 1–690. San Diego: Academic Press. doi: 10.1016/C2011-0-05296-1.
- Bhandari, B. 2015. Handbook of industrial drying, fourth edition edited by A. S. Mujumdar. Drying Technology 33 (1):128–9. doi: 10.1080/07373937.2014.983704.
- Bhandari, B., N. Bansal, M. Zhang, and P. Schuck. 2013. In Handbook of food powders: Processes and properties, 1–660. Cambridge: Woodhead Publishing Limited. doi: 10.1533/9780857098672.
- Both, E. M., R. M. Boom, and M. A. I. Schutyser. 2020. Particle morphology and powder properties during spray drying of maltodextrin and whey protein mixtures. Powder Technology 363:519–24. doi: 10.1016/j.powtec.2020.01.001.
- Buckow, R., A. Kastell, N. S. Terefe, and C. Versteeg. 2010. Pressure and temperature effects on degradation kinetics and storage stability of total anthocyanins in blueberry juice. Journal of Agricultural and Food Chemistry 58 (18):10076–84. doi: 10.1021/jf1015347.
- Busso Casati, C., R. Baeza, and V. Sánchez. 2017. Comparison of the kinetics of monomeric anthocyanins loss and colour changes in thermally treated blackcurrant, Maqui berry and blueberry pulps from Argentina. Journal of Berry Research 7 (2):85–96. doi: 10.3233/JBR-170151.
- Calín-Sánchez, Á., A. Kharaghani, K. Lech, A. Figiel, A. Carbonell-Barrachina, and E. Tsotsas. 2015. Drying kinetics and microstructural and sensory properties of black chokeberry (Aronia melanocarpa) as affected by drying method. Food and Bioprocess Technology 8 (1):63–74. doi: 10.1007/s11947-014-1383-x.
- Candia-Muñoz, N., M. Ramirez-Bunster, Y. Vargas-Hernández, and L. Gaete-Garretón. 2015. Ultrasonic spray drying vs high vacuum and microwaves technology for blueberries. Physics Procedia 70. Proceedings of the 2015 ICU International Congress on Ultrasonics, Metz, France. 867–871. doi: 10.1016/j.phpro.2015.08.178.
- Castro, A. M., E. Y. Mayorga, and F. L. Moreno. 2018. Mathematical modelling of convective drying of fruits: A review. Journal of Food Engineering 223:152–67. doi: 10.1016/j.jfoodeng.2017.12.012.
- Celli, G. B., R. Dibazar, A. Ghanem, and M. S.-L. Brooks. 2016. Degradation kinetics of anthocyanins in freeze-dried microencapsulates from lowbush blueberries (Vaccinium angustifolium Aiton) and prediction of shelf-life. Drying Technology 34 (10):1175–84. doi: 10.1080/07373937.2015.1099546.
- Chen, Q., Z. Li, J. Bi, L. Zhou, J. Yi, and X. Wu. 2017. Effect of hybrid drying methods on physicochemical, nutritional and antioxidant properties of dried black mulberry. LWT 80:178–84. doi: 10.1016/j.lwt.2017.02.017.
- Colín-Cruz, M. A., D. J. Pimentel-González, H. Carrillo-Navas, J. Alvarez-Ramírez, and A. Y. Guadarrama-Lezama. 2019. Co-encapsulation of bioactive compounds from blackberry juice and probiotic bacteria in biopolymeric matrices. LWT 110:94–101. doi: 10.1016/j.lwt.2019.04.064.
- Correia, R., M. H. Grace, D. Esposito, and M. A. Lila. 2017. Wild blueberry polyphenol-protein food ingredients produced by three drying methods: Comparative physico-chemical properties, phytochemical content, and stability during storage. Food Chemistry 235:76–85. doi: 10.1016/j.foodchem.2017.05.042.
- Ćujić, N., N. Stanisavljević, Š. Katarina, A. Kalušević, V. Nedovic, J. Samardzic, and T. Janković. 2018. Application of gum Arabic in the production of spray-dried chokeberry polyphenols, microparticles characterisation and in vitro digestion method. Lekovite Sirovine 38:10–8. doi: 10.1364/leksir1838009C.
- Ćujić-Nikolić, N., N. Stanisavljević, K. Šavikin, A. Kalušević, V. Nedović, J. Samardžić, and T. Janković. 2019. Chokeberry polyphenols preservation using spray drying: effect of encapsulation using maltodextrin and skimmed milk on their recovery following in vitro digestion. Journal of Microencapsulation 36 (8):693–703. doi: 10.1080/02652048.2019.1667448.
- Darniadi, S., P. Ho, and B. S. Murray. 2018. Comparison of blueberry powder produced via foam-mat freeze-drying versus spray-drying: Evaluation of foam and powder properties. Journal of the Science of Food and Agriculture 98 (5):2002–10. doi: 10.1002/jsfa.8685.
- Değirmencioğlu, N., O. Gürbüz, G. Karatepe, and R. Irkin. 2017. Influence of hot air drying on phenolic compounds and antioxidant capacity of blueberry (Vaccinium myrtillus) fruit and leaf. Journal of Applied Botany and Food Quality 90:115–25. doi: 10.5073/JABFQ.2017.090.014.
- Deng, L.-Z., A. S. Mujumdar, Q. Zhang, X.-H. Yang, J. Wang, Z.-A. Zheng, Z.-J. Gao, and H.-W. Xiao. 2019. Chemical and physical pretreatments of fruits and vegetables: Effects on drying characteristics and quality attributes – A comprehensive review. Critical Reviews in Food Science and Nutrition 59 (9):1408–32. doi: 10.1080/10408398.2017.1409192.
- Do Thi, N., and E.-S. Hwang. 2016. Effects of drying methods on contents of bioactive compounds and antioxidant activities of black chokeberries (Aronia melanocarpa). Food Science and Biotechnology 25 (1):55–61. doi: 10.1007/s10068-016-0008-8.
- El Jemli, M., R. Kamal, I. Marmouzi, A. Zerrouki, Y. Cherrah, and K. Alaoui. 2016. Radical-scavenging activity and ferric reducing ability of Juniperus thurifera (L.), J. Oxycedrus (L.), J. phoenicea (L.) and Tetraclinis articulata (L.). Advances in Pharmacological Sciences 2016:6392656. doi: 10.1155/2016/6392656.
- Eminoğlu, M. B., U. Yegül, and K. Sacilik. 2019. Drying characteristics of blackberry fruits in a convective hot-air dryer. HortScience 54 (9):1546–50. doi: 10.21273/HORTSCI14201-19.
- Erbay, Z., and F. Icier. 2010. A review of thin layer drying of foods: Theory, modeling, and experimental results. Critical Reviews in Food Science and Nutrition 50 (5):441–64. doi: 10.1080/10408390802437063.
- Food and Agriculture Organization. FAOSTAT. 2021. Accessed February 3, 2021. http://www.fao.org/faostat/en/#data/QC.
- Farías-Cervantes, V. S., Y. Salinas-Moreno, A. Chávez-Rodríguez, G. Luna-Solano, H. Medrano-Roldan, and I. Andrade-González. 2020. Stickiness and agglomeration of blackberry and raspberry spray dried juices using agave fructans and maltodextrin as carrier agents. Czech Journal of Food Sciences 38 (No. 4):229–36. doi: 10.17221/350/2018-CJFS.
- Farneti, B., I. Khomenko, M. Grisenti, M. Ajelli, E. Betta, A. A. Algarra, L. Cappellin, E. Aprea, F. Gasperi, F. Biasioli, et al. 2017. Exploring blueberry aroma complexity by chromatographic and direct-injection spectrometric techniques. Frontiers in Plant Science 8:617. doi: 10.3389/fpls.2017.00617.
- Feng, H., Y. Yin, and J. Tang. 2012. Microwave drying of food and agricultural materials: Basics and heat and mass transfer modeling. Food Engineering Reviews 4 (2):89–106. doi: 10.1007/s12393-012-9048-x.
- Fernandes, F. A. N., S. Rodrigues, C. L. Law, and A. S. Mujumdar. 2011. Drying of exotic tropical fruits: A comprehensive review. Food and Bioprocess Technology 4 (2):163–85. doi: 10.1007/s11947-010-0323-7.
- Ferrari, C. C., S. P. M. Germer, and J. M. de Aguirre. 2012. Effects of spray-drying conditions on the physicochemical properties of blackberry powder. Drying Technology 30 (2):154–63. doi: 10.1080/07373937.2011.628429.
- Ferreira Nogueira, G., L. G. Pereira Martin, F. M. Fakhouri, and R. Augustus de Oliveira. 2018. Microencapsulation of blackberry pulp with arrowroot starch and gum arabic mixture by spray drying. Journal of Microencapsulation 35 (5):482–93. doi: 10.1080/02652048.2018.1538264.
- U.S. Department of Agriculture. FoodData Central. 2021. Accessed February 6, 2021. https://fdc.nal.usda.gov/.
- Fracassetti, D., C. Del Bo’, P. Simonetti, C. Gardana, D. Klimis-Zacas, and S. Ciappellano. 2013. Effect of time and storage temperature on anthocyanin decay and antioxidant activity in wild blueberry (Vaccinium angustifolium) powder. Journal of Agricultural and Food Chemistry 61 (12):2999–3005. doi: 10.1021/jf3048884.
- Franceschinis, L., D. M. Salvatori, N. Sosa, and C. Schebor. 2014. Physical and functional properties of blackberry freeze- and spray-dried powders. Drying Technology 32 (2):197–207. doi: 10.1080/07373937.2013.814664.
- Fudholi, A., K. Sopian, M. H. Ruslan, M. A. Alghoul, and M. Y. Sulaiman. 2010. Review of solar dryers for agricultural and marine products. Renewable and Sustainable Energy Reviews 14 (1):1–30. doi: 10.1016/j.rser.2009.07.032.
- Gancel, A.-L., A. Feneuil, O. Acosta, A. M. Pérez, and F. Vaillant. 2011. Impact of industrial processing and storage on major polyphenols and the antioxidant capacity of tropical highland blackberry (Rubus adenotrichus). Food Research International 44 (7):2243–51. doi: 10.1016/j.foodres.2010.06.013.
- Gawałek, J., and E. Domian. 2020. Tapioca dextrin as an alternative carrier in the spray drying of fruit juices – A case study of chokeberry powder. Foods 9 (8):1125. doi: 10.3390/foods9081125.
- Gogate, P. R. 2015. 30 – The use of ultrasonic atomization for encapsulation and other processes in food and pharmaceutical manufacturing. In Power ultrasonics, ed. J.A. Gallego-Juárez and K.F. Graff, 911–35. Oxford: Woodhead Publishing. doi: 10.1016/B978-1-78242-028-6.00030-2.
- Goula, A., and K. Adamopoulos. 2005. Spray drying of tomato pulp in dehumidified air. II. The effect on power properties. Journal of Food Engineering - Engineering 66 (1):35–42. doi: 10.1016/j.jfoodeng.2004.02.031.
- Grabowski, S., M. Marcotte, D. Quan, A. R. Taherian, M. R. Zareifard, M. Poirier, and T. Kudra. 2007. Kinetics and quality aspects of Canadian blueberries and cranberries dried by osmo-convective method. Drying Technology 25 (2):367–74. doi: 10.1080/07373930601120563.
- Hassimotto, N., R. Mota, B. Cordenunsi, and F. Lajolo. 2008. Physicochemical characterization and bioactive compounds of blackberry fruits (Rubus Sp.) grown in Brazil. Ciencia E Tecnologia De Alimentos – Ciencia Tecnol Aliment 28 (3): 702–08. doi: 10.1590/S0101-20612008000300029.
- Hnin, K. K., M. Zhang, A. S. Mujumdar, and Y. Zhu. 2019. Emerging food drying technologies with energy-saving characteristics: A review. Drying Technology 37 (12):1465–80. doi: 10.1080/07373937.2018.1510417.
- Horszwald, A., H. Julien, and W. Andlauer. 2013. Characterisation of Aronia powders obtained by different drying processes. Food Chemistry 141 (3):2858–63. doi: 10.1016/j.foodchem.2013.05.103.
- Huang, D., P. Yang, X. Tang, L. Luo, and B. Sunden. 2021. Application of infrared radiation in the drying of food products. Trends in Food Science & Technology 110:765–77. doi: 10.1016/j.tifs.2021.02.039.
- Islam Shishir, M. R., Taip, F. S. N. Ab. Aziz, R. A. Talib, and Md, and S. Hossain Sarker. 2016. Optimization of spray drying parameters for pink guava powder using RSM. Food Science and Biotechnology 25 (2):461–8. doi: 10.1007/s10068-016-0064-0.
- Jafari, S. M., and D. J. McClements. 2017. Nanotechnology approaches for increasing nutrient bioavailability. Advances in Food and Nutrition Research 81:1–30. doi: 10.1016/bs.afnr.2016.12.008.
- Janiszewska-Turak, E., A. Sak, and D. Witrowa-Rajchert. 2019. Influence of the carrier material on the stability of chokeberry juice microcapsules. International Agrophysics 33 (4):517–25. doi: 10.31545/intagr/113530.
- Jiménez-Aguilar, D. M., A. E. Ortega-Regules, J. D. Lozada-Ramírez, M. C. I. Pérez-Pérez, E. J. Vernon-Carter, and J. Welti-Chanes. 2011. Color and chemical stability of spray-dried blueberry extract using mesquite gum as wall material. Journal of Food Composition and Analysis 24 (6):889–94. doi: 10.1016/j.jfca.2011.04.012.
- Joardder, M. U. H., A. Karim, and C. Kumar. 2013. Effect of temperature distribution on predicting quality of microwave dehydrated food. Journal of Mechanical Engineering and Sciences 5:562–8. doi: 10.15282/jmes.5.2013.2.0053.
- Kadam, D., R. Goyal, and M. Gupta. 2011. Mathematical modeling of convective thin layer drying of basil leaves. Journal of Medicinal Plant Research 5 (19):4721–30.
- Kamiloglu, S., and E. Capanoglu. 2015. Polyphenol content in Figs (Ficus carica L.): Effect of sun-drying. International Journal of Food Properties 18 (3):521–35. doi: 10.1080/10942912.2013.833522.
- Karakashova, L., F. Babanovska-Milenkovska, M. Stojanova, and B. Karakashov. 2016. Comparison of qualities properties of fresh, frozen and solar dried chokeberry fruits. 25th International Scientific-Experts Congress on Agriculture and Food Industry 2014, Izmir. doi: 10.13140/RG.2.1.4506.1209.
- Karam, M. C., J. Petit, D. Zimmer, E. B. Djantou, and J. Scher. 2016. Effects of drying and grinding in production of fruit and vegetable powders: A review. Journal of Food Engineering 188:32–49. doi: 10.1016/j.jfoodeng.2016.05.001.
- Kassem, A. S., A. Z. Shokr, A. R. El-Mahdy, A. M. Aboukarima, and E. Y. Hamed. 2011. Comparison of drying characteristics of Thompson seedless grapes using combined microwave oven and hot air drying. Journal of the Saudi Society of Agricultural Sciences 10 (1):33–40. doi: 10.1016/j.jssas.2010.05.001.
- Kaveh, M., E. Taghinezhad, and M. Aziz. 2020. Effects of physical and chemical pretreatments on drying and quality properties of blackberry (Rubus Spp.) in hot air dryer. Food Science & Nutrition 8 (7):3843–56. doi: 10.1002/fsn3.1678.
- Kechinski, C. P., P. V. R. Guimarães, C. P. Z. Noreña, I. C. Tessaro, and L. D. F. Marczak. 2010. Degradation kinetics of anthocyanin in blueberry juice during thermal treatment. Journal of Food Science 75 (2):C173–6. doi: 10.1111/j.1750-3841.2009.01479.x.
- Khuri, A. 2017. Response surface methodology and its applications in agricultural and food sciences. Biometrics & Biostatistics International Journal 5 (5):155–63. doi: 10.15406/bbij.2017.05.00141.
- Khuri, A. I., and S. Mukhopadhyay. 2010. Response surface methodology. Wiley Interdisciplinary Reviews: Computational Statistics 2 (2):128–49. doi: 10.1002/wics.73.
- Kiliç, E. E., and İ. Çinar. 2019. Convective hot airdrying characteristics of selected vegetables. International Advanced Researches and Engineering Journal 3 (1):7–13.
- Klemeš, J., R. Smith, and J.-K. Kim. 2008. Handbook of water and energy management in food processing, 1–1029. Cambridge: Woodhead Publishing.
- Koner, S., P. Dash, V. Priya, and V. D. Rajeswari. 2019. 15 – Natural and artificial beverages: Exploring the pros and cons. In Natural Beverages, ed. A. M. Grumezescu and A. M. Holban, 427–45. Cambridge: Academic Press. doi: 10.1016/B978-0-12-816689-5.00015-8.
- Krishnaiah, D., A. Bono, R. Sarbatly, R. Nithyanandam, and S. M. Anisuzzaman. 2015. Optimisation of spray drying operating conditions of Morinda citrifolia L. Fruit extract using response surface methodology. Journal of King Saud University – Engineering Sciences 27 (1):26–36. doi: 10.1016/j.jksues.2012.10.004.
- Krishnaswamy, K., and V. Orsat. 2017. Chapter 2. Sustainable delivery systems through green nanotechnology. In Nano- and microscale drug delivery systems: Design and fabrication. ed. A. M. Grumezescu, 17–32. Cambridge: Elsevier Science. doi: 10.1016/B978-0-323-52727-9.00002-9.
- Kucner, A., R. Klewicki, and M. Sójka. 2013. The influence of selected osmotic dehydration and pretreatment parameters on dry matter and polyphenol content in highbush blueberry (Vaccinium corymbosum L.) fruits. Food and Bioprocess Technology 6 (8):2031–47. doi: 10.1007/s11947-012-0997-0.
- Kucuk, H., A. Kilic, and A. Midilli. 2014. Common applications of thin layer drying curve equations and their evaluation criteria. In Progress in exergy, energy, and the environment, ed, eds. I. Dincer, A.Midilli, and H. Kucuk, 669–80. Cham: Springer. doi: 10.1007/978-3-319-04681-5_63.
- Kulling, S. E., and H. M. Rawel. 2008. Chokeberry (Aronia melanocarpa) – A review on the characteristic components and potential health effects. Planta Medica 74 (13):1625–34. doi: 10.1055/s-0028-1088306.
- Kumar, S., R. Sandhir, and S. Ojha. 2014. Evaluation of antioxidant activity and total phenol in different varieties of Lantana camara leaves. BMC Research Notes 7:560. doi: 10.1186/1756-0500-7-560.
- Lo, A., J. C. Gallardo Brigido, A. Silva-Norman, I. Pilatowsky, O. García-Valladares, and J. Ramírez. 2018. Moisture content modeling and effective moisture diffusivity determination during convective solar drying of blackberry (Rubus Spp) and Basil (Ocimum basilicum L.). Paper presented at the IDS 2018. 21st International Drying Symposium Proceedings. doi: 10.4995/IDS2018.2018.7841.
- Leyva-Porras, C., M. Z. Saavedra-Leos, E. Cervantes-González, P. Aguirre-Bañuelos, M. B. Silva-Cázarez, and C. Álvarez-Salas. 2019. Spray drying of blueberry juice-maltodextrin mixtures: evaluation of processing conditions on content of resveratrol. Antioxidants (Basel, Switzerland) 8 (10):437. doi: 10.3390/antiox8100437.
- Li, K., M. Zhang, A. S. Mujumdar, and B. Chitrakar. 2019. Recent developments in physical field-based drying techniques for fruits and vegetables. Drying Technology 37 (15):1954–73. doi: 10.1080/07373937.2018.1546733.
- López-Nicolás, J., and F. García‐Carmona. 2009. Enzymatic and nonenzymatic degradation of polyphenols. In Fruit and vegetable phytochemicals, eds. L.D. L.A. Rosa, E. Alvarez-Parrilla, G. A. González-Aguilar, 101–29. New Jersey: John Wiley & Sons, Ltd.
- López‐Vidaña, E. C., I. P. Figueroa, E. G. A. Marcos, A. Navarro‐Ocaña, L. Hernández‐Vázquez, and J. A. Santiago‐Urbina. 2019. Solar drying kinetics and bioactive compounds of blackberry (Rubus fruticosus). Journal of Food Process Engineering 42 (4):e13018. doi: 10.1111/jfpe.13018.
- Martín-Gómez, J., M. Varo, J. Merida, and M. Serratosa. 2020. Influence of drying processes on anthocyanin profiles, total phenolic compounds and antioxidant activities of blueberry (Vaccinium corymbosum). LWT 120:108931. doi: 10.1016/j.lwt.2019.108931.
- Martynenko, A., and Y. Chen. 2016. Degradation kinetics of total anthocyanins and formation of polymeric color in blueberry hydrothermodynamic (HTD) processing. Journal of Food Engineering 171:44–51. doi: 10.1016/j.jfoodeng.2015.10.008.
- Mejia-Meza, E., J. Yáñez, N. Davies, B. Rasco, F. Younce, C. Remsberg, and C. Clary. 2008. Improving nutritional value of dried blueberries (Vaccinium corymbosum L.) combining microwave-vacuum, hot-air drying and freeze drying technologies. International Journal of Food Engineering 4 (5). doi: 10.2202/1556-3758.1364.
- Moreno, J., M. Gonzales, P. Zúñiga, G. Petzold, K. Mella, and O. Muñoz. 2016. Ohmic heating and pulsed vacuum effect on dehydration processes and polyphenol component retention of osmodehydrated blueberries (Cv. Tifblue). Innovative Food Science & Emerging Technologies 36:112–9. doi: 10.1016/j.ifset.2016.06.005.
- Mumtaz, M. W., A. Adnan, H. Mukhtar, U. Rashid, and M. Danish. 2017. Chapter fifteen – Biodiesel production through chemical and biochemical transesterification: Trends, technicalities, and future perspectives. In Clean energy for sustainable development, ed. M. G. Rasul, A. Kalam Azad, and S. C. Sharma, 465–85. London: Academic Press. doi: 10.1016/B978-0-12-805423-9.00015-6.
- Natesan, V. T., P. Mani, T. J. S. Prasad, J. M. Krishna, and S. Sekar. 2020. Applications of thin layer modelling techniques and advances in drying of agricultural products. AIP Conference Proceedings 2311:090025. doi: 10.1063/5.0033995.
- Nayak, B., R. H. Liu, and J. Tang. 2015. Effect of processing on phenolic antioxidants of fruits, vegetables, and grains – A review. Critical Reviews in Food Science and Nutrition 55 (7):887–918. doi: 10.1080/10408398.2011.654142.
- Nemzer, B., L. Vargas, X. Xia, M. Sintara, and H. Feng. 2018. Phytochemical and physical properties of blueberries, tart cherries, strawberries, and cranberries as affected by different drying methods. Food Chemistry 262:242–50. doi: 10.1016/j.foodchem.2018.04.047.
- Ngamwonglumlert, L., and S. Devahastin. 2018. 8 – Microstructure and its relationship with quality and storage stability of dried foods. In Food microstructure and its relationship with quality and stability, ed. S. Devahastin, 139–59. Woodhead publishing series in food science, technology and nutrition. Cambridge: Woodhead Publishing. doi: 10.1016/B978-0-08-100764-8.00008-3.
- Nile, S. H., and S. W. Park. 2014. Edible berries: Bioactive components and their effect on human health. Nutrition 30 (2):134–44. doi: 10.1016/j.nut.2013.04.007.
- Nogueira, G. F., C. T. Soares, L. G. P. Martin, F. M. Fakhouri, and R. A. de Oliveira. 2020. Influence of spray drying on bioactive compounds of blackberry pulp microencapsulated with arrowroot starch and gum Arabic mixture. Journal of Microencapsulation 37 (1):65–76. doi: 10.1080/02652048.2019.1693646.
- Oancea, S., and F. Călin. 2016. Changes in total phenolics and anthocyanins during blackberry, raspberry and cherry jam processing and storage. Romanian Biotechnological Letters 21:11232–7.
- Onwude, D. I., N. Hashim, R. B. Janius, N. M. Nawi, and K. Abdan. 2016. Modeling the thin-layer drying of fruits and vegetables: A review. Comprehensive Reviews in Food Science and Food Safety 15 (3):599–618. doi: 10.1111/1541-4337.12196.
- Parikh, D. 2015. Vacuum drying: Basics and application. Chemical Engineering 122 (4).
- Patil, V., A. K. Chauhan, and R. P. Singh. 2014. Optimization of the spray-drying process for developing guava powder using response surface methodology. Powder Technology 253:230–6. doi: 10.1016/j.powtec.2013.11.033.
- Petkovic, M., Đurović, I. N. Miletic, A. Lukyanov, Е. Ключка, J. Radovanović, and D. Donskoy. 2020. Model of convective drying of black chokeberry (Aronia melanocarpa l.). XXV Savetovanje O Biotehnologiji 2:563–9.
- Petkovic, M., I. Đurović, N. Miletic, and J. Radovanović. 2019. Effect of convective drying method of chokeberry (Aronia melanocarpa L.) on drying kinetics, bioactive components and sensory characteristics of bread with chokeberry powder. Periodica Polytechnica Chemical Engineering 63 (4):600–8. doi: 10.3311/PPch.13783.
- Phisut, N. 2012. Spray drying technique of fruit juice powder: Some factors influencing the properties of product. International Food Research Journal 19:1297–306.
- Pomeranz, Y., and C. E. Meloan. 1994. Determination of moisture. In Food analysis: Theory and practice, ed. Y. Pomeranz and C. E. Meloan, 575–601. Boston, MA: Springer US. doi: 10.1007/978-1-4615-6998-5_34.
- Prakash, O., and A. Kumar. 2013. Historical review and recent trends in solar drying systems. International Journal of Green Energy 10 (7):690–738. doi: 10.1080/15435075.2012.727113.
- Quintero, J., A. Naranajo, G. Ciro, and J. Rojas. 2017. Vegetable proteins: Non-sensitizing encapsulation agents for bioactive compounds. Allergen. 4: 43–65. doi: 10.5772/intechopen.70378.
- Reque, P., R. Steffens, A. Martins da Silva, A. Jablonski, S. Flôres, A. Rios, and E. Jong. 2014. Characterization of blueberry fruits (Vaccinium Spp.) and derived products. Food Science and Technology (Campinas) 34 (4):773–9. doi: 10.1590/1678-457X.6470.
- Reyes, A., A. Evseev, A. Mahn, V. Bubnovich, R. Bustos, and E. Scheuermann. 2011. Effect of operating conditions in freeze-drying on the nutritional properties of blueberries. International Journal of Food Sciences and Nutrition 62 (3):303–6. doi: 10.3109/09637486.2010.534078.
- Rezvankhah, A., Z. Emam-Djomeh, and G. Askari. 2020. Encapsulation and delivery of bioactive compounds using spray and freeze-drying techniques: A review. Drying Technology 38 (1–2):235–58. doi: 10.1080/07373937.2019.1653906.
- Rigon, R. T., and C. P. Zapata Noreña. 2016. Microencapsulation by spray-drying of bioactive compounds extracted from blackberry (Rubus fruticosus). Journal of Food Science and Technology 53 (3):1515–24. doi: 10.1007/s13197-015-2111-x.
- Rodriguez, A., M. J. Zaro, M. L. Lemoine, and R. H. Mascheroni. 2016. Comparison of two alternatives of combined drying to processing of blueberries (ÓNeal): Evaluation of the final quality. Drying Technology 34 (8):974–85. doi: 10.1080/07373937.2015.1089886.
- Ruiz-Cabrera, M. A., and S. J. Schmidt. 2015. Determination of glass transition temperatures during cooling and heating of low-moisture amorphous sugar mixtures. Journal of Food Engineering 146:36–43. doi: 10.1016/j.jfoodeng.2014.08.023.
- Sablani, S. S., P. K. Andrews, N. M. Davies, T. Walters, H. Saez, and L. Bastarrachea. 2011. Effects of air and freeze drying on phytochemical content of conventional and organic berries. Drying Technology 29 (2):205–16. doi: 10.1080/07373937.2010.483047.
- Sadowska, K., A. Jadwiga, and Ł. Klóska. 2017. Influence of freezing, lyophilisation and air-drying on the total monomeric anthocyanins, vitamin C and antioxidant capacity of selected berries. International Journal of Food Science & Technology 52 (5):1246–51. doi: 10.1111/ijfs.13391.
- Sadowska, A., F. Świderski, R. Rakowska, and E. Hallmann. 2017. The functional properties of chokeberry and kale powders obtained by an innovative method of fluidised-bed jet milling with drying compared to freeze drying. International Journal of Food Engineering 13 (6). doi: 10.1515/ijfe-2016-0310.
- Sadowska, A., F. Świderski, R. Rakowska, and E. Hallmann. 2019. Comparison of quality and microstructure of chokeberry powders prepared by different drying methods, including innovative fluidised bed jet milling and drying. Food Science and Biotechnology 28 (4):1073–81. doi: 10.1007/s10068-019-00556-1.
- Sagar, V. R., and P. Suresh Kumar. 2010. Recent advances in drying and dehydration of fruits and vegetables: A review. Journal of Food Science and Technology 47 (1):15–26. doi: 10.1007/s13197-010-0010-8.
- Samoticha, J., A. Wojdyło, and K. Lech. 2016. The influence of different the drying methods on chemical composition and antioxidant activity in chokeberries. LWT – Food Science and Technology 66:484–9. doi: 10.1016/j.lwt.2015.10.073.
- Sapei, L., and L. Hwa. 2014. Study on the kinetics of vitamin c degradation in fresh strawberry juices. Procedia Chemistry 9. Bali: International Conference and Workshop on Chemical Engineering UNPAR 2013 (ICCE UNPAR 2013), 62–8. doi: 10.1016/j.proche.2014.05.008.
- Sarkis, J. R., D. P. Jaeschke, G. D. Mercali, I. C. Tessaro, and L. D. F. Marczak. 2013. Degradation kinetics of anthocyanins in blackberry pulp during ohmic and conventional heating. International Food Research Journal 26 (1):87–97.
- Selvi, N. J., B. Gurunathan, and A. Singh. 2014. Effect of various pretreatment methods on osmotic dehydration of fruits for qualitative and quantitative advantage. International Journal of ChemTech Research 6 (12):4995–5001.
- Sharif, N., S. Khoshnoudi-Nia, and S. M. Jafari. 2020. Nano/microencapsulation of anthocyanins; a systematic review and meta-analysis. Food Research International 132:109077. doi: 10.1016/j.foodres.2020.109077.
- Shi, J., Z. Pan, T. H. McHugh, D. Wood, E. Hirschberg, and D. Olson. 2008. Drying and quality characteristics of fresh and sugar-infused blueberries dried with infrared radiation heating. LWT – Food Science and Technology 41 (10):1962–72. doi: 10.1016/j.lwt.2008.01.003.
- Shishir, M. R. I., and W. Chen. 2017. Trends of spray drying: A critical review on drying of fruit and vegetable juices. Trends in Food Science & Technology 65:49–67. doi: 10.1016/j.tifs.2017.05.006.
- Skrovankova, S., D. Sumczynski, J. Mlcek, T. Jurikova, and J. Sochor. 2015. Bioactive compounds and antioxidant activity in different types of berries. International Journal of Molecular Sciences 16 (10):24673–706. doi: 10.3390/ijms161024673.
- Song, C., Z. Li, T. Wu, V. Raghavan, X. Ma, and H. Chen. 2020. Mass transfer during osmotic dehydration and its effect on anthocyanin retention of microwave vacuum-dried blackberries. Journal of the Science of Food and Agriculture 100 (1):102–9. doi: 10.1002/jsfa.9999.
- Soong, Y.-Y., and P. J. Barlow. 2004. Antioxidant activity and phenolic content of selected fruit seeds. Food Chemistry 88 (3):411–7. doi: 10.1016/j.foodchem.2004.02.003.
- Stratta, L., L. C. Capozzi, S. Franzino, and R. Pisano. 2020. Economic analysis of a freeze-drying cycle. Processes 8 (11):1399. doi: 10.3390/pr8111399.
- Sumic, M., N. Tepic, D. Jokic, and V. Malbasa. 2015. Optimization of frozen wild blueberry vacuum drying process. Hemijska Industrija 69 (1):77–84. doi: 10.2298/HEMIND131212022S.
- Sutar, P., and S. Prasad. 2008. Microwave drying technology-recent developments and R&D needs in India. Proceedings of 42nd ISAE Annual Convention, Bhopal. 1–3.
- Taghinezhad, E., M. Kaveh, E. Khalife, and G. Chen. 2021. Drying of organic blackberry in combined hot air-infrared dryer with ultrasound pretreatment. Drying Technology 39 (14):2075–17. doi: 10.1080/07373937.2020.1753066.
- Taşkın, O. 2020. Evaluation of freeze drying for whole, half cut and puree black chokeberry (Aronia melanocarpa L.). Heat and Mass Transfer 56:2503–13. doi: 10.1007/s00231-020-02867-0.
- Tatar Turan, F., A. Cengiz, D. Sandıkçı, M. Dervisoglu, and T. Kahyaoglu. 2016. Influence of an ultrasonic nozzle in spray-drying and storage on the properties of blueberry powder and microcapsules. Journal of the Science of Food and Agriculture 96 (12):4062–76. doi: 10.1002/jsfa.7605.
- Tylewicz, U., M. Nowacka, B. Martín-García, A. Wiktor, and A. M. Gómez Caravaca. 2018. 5 – Target sources of polyphenols in different food products and their processing by-products. In Polyphenols: Properties, recovery, and applications, ed. C. M. Galanakis, 135–75. Cambridge: Woodhead Publishing. doi: 10.1016/B978-0-12-813572-3.00005-1.
- USDA National Agricultural Statistics Service. 2021. Quick Stats. United States Department of Agriculture. Accessed June 22, 2021. https://quickstats.nass.usda.gov/.
- Vega-Galvez, A., E. Lara, V. Flores, K. D. Scala, and R. Lemus-Mondaca. 2012. Effect of selected pretreatments on convective drying process of blueberries (Var. O’neil). Food and Bioprocess Technology 5 (7):2797–8. doi: 10.1007/s11947-011-0656-x.
- Viljakainen, S., A. Visti, and S. Laakso. 2002. Concentrations of organic acids and soluble sugars in juices from Nordic berries. Acta Agriculturae Scandinavica, Section B – Soil & Plant Science 52 (2):101–9. doi: 10.1080/090647102321089846.
- Wilkowska, A., W. Ambroziak, J. Adamiec, and A. Czyżowska. 2017. Preservation of antioxidant activity and polyphenols in chokeberry juice and wine with the use of microencapsulation. Journal of Food Processing and Preservation 41 (3):e12924. doi: 10.1111/jfpp.12924.
- Wilkowska, A., W. Ambroziak, A. Czyżowska, and J. Adamiec. 2016. Effect of microencapsulation by spray drying and freeze drying technique on the antioxidant properties of blueberry (Vaccinium myrtillus) juice polyphenolic compounds. Polish Journal of Food and Nutrition Sciences 66 (1):11–6. doi: 10.1515/pjfns-2015-0015.
- Wray, D., and H. S. Ramaswamy. 2015. Microwave-osmotic/microwave-vacuum drying of whole cranberries: Comparison with other methods. Journal of Food Science 80 (12):E2792–2802. doi: 10.1111/1750-3841.13132.
- Yadav, A. K., and S. V. Singh. 2014. Osmotic dehydration of fruits and vegetables: A review. Journal of Food Science and Technology 51 (9):1654–73. doi: 10.1007/s13197-012-0659-2.
- Yamamoto, C., T. L. Neoh, H. Honbou, T. Furuta, S. Kimura, and H. Yoshii. 2012. Formation of a polymer-coated inclusion complex of D-limonene and β-cyclodextrin by spray drying. Drying Technology 30 (15):1714–9. doi: 10.1080/07373937.2012.699487.
- Yu, Y., T. Z. Jin, X. Fan, and Y. Xu. 2017. osmotic dehydration of blueberries pretreated with pulsed electric fields: Effects on dehydration kinetics, and microbiological and nutritional qualities. Drying Technology 35 (13):1543–51. doi: 10.1080/07373937.2016.1260583.
- Yu, Y., T. Z. Jin, and G. Xiao. 2017. Effects of pulsed electric fields pretreatment and drying method on drying characteristics and nutritive quality of blueberries. Journal of Food Processing and Preservation 41 (6):e13303. doi: 10.1111/jfpp.13303.
- Yuan, W., L. Zhou, G. Deng, P. Wang, D. Creech, and S. Li. 2011. Anthocyanins, phenolics, and antioxidant capacity of Vaccinium L. in Texas, USA. Pharmaceutical Crops 2 (1):11–23. doi: 10.2174/2210290601102010011.
- Zhao, Y. 2007. Berry fruit: Value-added products for health promotion. Berry fruit: Value-added products for health promotion. Boca Raton, FL: CRC Press.
- Zielinska, M., and M. Markowski. 2016. The influence of microwave-assisted drying techniques on the rehydration behavior of blueberries (Vaccinium corymbosum L.). Food Chemistry 196:1188–96. doi: 10.1016/j.foodchem.2015.10.054.
- Zielinska, M., and A. Michalska. 2016. Microwave-assisted drying of blueberry (Vaccinium corymbosum L.) fruits: Drying kinetics, polyphenols, anthocyanins, antioxidant capacity, colour and texture. Food Chemistry 212:671–80. doi: 10.1016/j.foodchem.2016.06.003.
- Zielinska, M., and A. Michalska. 2018. The influence of convective, microwave vacuum and microwave-assisted drying on blueberry pomace physicochemical properties. International Journal of Food Engineering 14 (3):1–14. doi: 10.1515/ijfe-2017-0332.
- Zielinska, M. P. and Sadowski, W. Błaszczak. 2016. Combined hot air convective drying and microwave-vacuum drying of blueberries (Vaccinium corymbosum L.): Drying kinetics and quality characteristics. Drying Technology 34 (6):665–84. doi: 10.1080/07373937.2015.1070358.