Abstract
Tea flavonoids are widely recognized as critical flavor contributors and crucial health-promoting bioactive compounds, and have long been the focus of research worldwide in food science. The aim of this review paper is to summarize the major progress in tea flavonoid chemistry, their dynamics of constituents and concentrations during tea processing as well as storage, and their health functions studied between 2001 and 2021. Moreover, the utilization of tea flavonoids in the human body has also been discussed for a detailed understanding of their uptake, metabolism, and interaction with the gut microbiota. Many novel tea flavonoids have been identified, including novel A- and B-ring substituted flavan-3-ol derivatives, condensed and oxidized flavan-3-ol derivatives, and glycosylated and methylated flavonoids, and are found to be closely associated with the characteristic color, flavor, and health benefits of tea. Flavoalkaloids exist widely in various teas, particularly 8-C N-ethyl-2-pyrrolidinone-substituted flavan-3-ols. Tea flavonoids behave significantly difference in constituents and concentrations depending on tea cultivars, plantation conditions, multiple stresses, the tea-specified manufacturing steps, and even the long-term storage period. Tea flavonoids exhibit multiple health-promoting effects, particularly their anti-inflammatory in alleviating metabolic syndromes. Interaction of tea flavonoids with the gut microbiota plays vital roles in their health function.
Highlights
The newly identified tea flavonoids in last two decades are reviewed.
Various factors contribute to changes in tea flavonoids are explored.
The metabolism and interactions with gut microbiota of tea flavonoids are analyzed.
Introduction
As a perennial leafy crop, Camellia sinensis (L.) Kuntze, which is well known as tea, is commercially cultivated on more than 5.07 million hectares on a continent-wide scale, and 6.49 million metric ton tea is produced annually worldwide, which was worth more than USD 12.53 billion in 2019 according to the Food and Agriculture Organization of the United Nation (FAO) (http://www.fao.org/faostat/en/#data/QCL). Generally, these consumable tea products can be classified as green (non-fermented), white (slightly fermented), yellow (mild fermented), oolong (half fermented), black (fully fermented), and dark (post-fermented) tea based on their processing technologies (Ho, Lin, and Shahidi Citation2008) (). Among these, black and green teas are the two most consumed tea products worldwide, which accounting for about 75% and 15% global tea consumption, respectively.
Figure 1. Six traditional teas and their major chemical composition and major classification of flavonoids in tea and the chemistry structure.
Six typical traditional teas have been illustrated including green tea, white tea, yellow tea, oolong tea, black tea, and dark tea according to their unique processing procedures. Amino acids (theanine and GABA), alkaloids (caffein), lipids, soluble sugars, minerals, lipid soluble pigments, and others are major quality-related compounds in teas. Flavonoids which occupied around 25–35% behave typically different in six types of teas, that catechins are major flavonoids in green and white teas, proanthocyanidins are enriched in specialized green and white teas, theasinensins, theaflavins, and thearubigins are characteristics flavonoids in oolong teas and black teas, and theabrownines are particularly formed in dark teas. Besides, anthocyanins and novel flavoalkaloids are attracting attentions for their importance in teas.
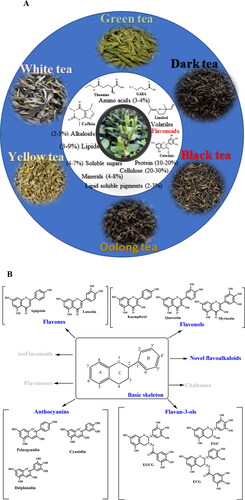
Tea plants biosynthesize various specialized metabolites related to the remarkable flavor and health functions (Liao, Zhou, and Zeng Citation2020). The popularity of tea consumption can be attributed to its unique color, flavor, and health properties, which is a result of the presence of thousands of metabolites (Preedy Citation2013). Among these metabolites, tea flavonoids are particularly specialized, exhibit hyperaccumulation, and account for 20-30% tea dry matter (Wang, Provan, and Helliwell Citation2000). Tea flavonoids have attracted much attention owing to their multiple roles in improving resistance to multiple stresses in fresh tea leaves, forming characteristic flavor and color, and most importantly, possessing many health benefits (He et al. Citation2021). Notably, considering the huge amount of tea consumed, it is an optimal source of dietary flavonoids that cannot be synthesized by the human body (Panche, Diwan, and Chandra Citation2016).
With the deepening of research and desirable demands from consumers, numerous tea flavonoids have been newly explored, and research on the chemistry, processing characteristics, and utilization of tea flavonoids have significantly progressed during the past two decades. It is necessary to comprehensively update the recognition of tea flavonoids which can greatly replenish the previous review of tea catechin chemistry, structure, and function by Wang, Provan, and Helliwell (Citation2000). Therefore, studies on tea flavonoids in the last two decades have been reviewed in this study: first, studies on flavonoid chemistry with emphasis on newly identified flavan-3-ol conjugates, expanding the exploration of anthocyanins and the structurally modified flavonols; second, studies on flavonoid constituents and concentrations during tea processing, as well as during tea storage; third, studies on the new insights of tea flavonoid utilization, including the potential mechanism of uptake and metabolism in the human body, and in vivo bioavailability enhancement by interaction with gut microbiota; and finally, studies proposing promising perspectives from desired tea flavonoid enrichment to their bioavailability enhancement. The present updated review will greatly contribute to a comprehensive and latest understanding of the chemistry and bioactivity of tea flavonoids, and offer important insights into novel strategies for their utilization.
Updates in tea flavonoids chemistry in the last 20 years
Favonoids have always been the hotspot and difficulty globally in food science (Halbwirth Citation2010; He et al. Citation2021). Over the past 20 years, several studies have focused on tea flavonoids, and research on their chemistry, mainly unraveling novel flavan-3-ol and its conjugates, structural identification of anthocyanins, and exploration of structurally modified flavonoids, have progressed significantly.
As the most representative secondary metabolites, flavonoids comprise a large group of structurally diverse analytes formed from a variety of basic skeletons and series derivatives that attracted research interest since long ago. Flavonoids are commonly divided into several groups depending on the carbon of the C-ring to which the B-ring is attached and the degree of unsaturation and oxidation of the C-ring, mainly including flavones, flavonols, flavanones, flavanols, and anthocyanins (). Major tea flavonoids include flavones, flavanols, and flavonols, as described previously (Wang, Provan, and Helliwell Citation2000), and the new aspects of flavan-3-ols conjugates, anthocyanins, and structurally modified flavonols are emphasized herein ().
Table 1. Major catechins, their oxidative and condensed conjugates, and other novel flavan-3-ol derivatives.
Flavan-3-ols and their conjugates
Flavan-3-ols and their conjugates in tea mainly include naturally occurring catechins, novel catechin conjugates and derivatives (such as A-ring and B-ring of substituted flavan-3-ols), and condensed and oxidized flavan-3-ol derivatives, including theaflavins (TFs), theasinensins (TSs), and proanthocyanidins (PAs) ().
Major naturally occurring flavan-3-ol individuals
Flavan-3-ols (mainly tea catechins) are the most abundant subclass of flavonoids in teas. The major constituents of catechins present in gallogated forms unique to tea plants include (-)-gallocatechin-3-gallate (GCG), (-)-epicatechin-3-gallate (ECG), and (-)-epigallocatechin gallate (EGCG); while non-gallogated forms include (-)-epigallocatechin (EGC), (-)-epicatechin (EC), (-)-gallocatechin (GC), (-)-catechin (C).
In addition to the major tea catechins, the naturally occurring catechin derivatives, such as hydroxylated catechin derivatives and methylated catechin derivatives have been well studied in recent years. For example, the extra hydroxyl located at the 4-position on C-ring of catechin derivatives (Engelhardt, Lakenbrink, and Pokorny Citation2003), including leucopelargonidin, leucocyanidin, and leucodelphinidin (), are also well known as leucoanthocyanidins. The concentration of these colorless leucoanthocyanins is around 2-3% in teas, which can successively be transformed to the oxidative products, pigmented anthocyanins (Neilson and Ferruzzi Citation2011). In addition, methylated EGCG (such as EGCG3″Me and EGCG4″Me), proven to be antiallergic and antihypertensive, have been studied comprehensively, particularly their levels in various tea cultivars and processing characteristics (Maeda-Yamamoto et al. Citation2012; Lv et al. Citation2014). Luo et al. (Citation2018) have reviewed O-methylated catechin biosynthesis in tea plants.
Novel A- and B-ring substituted flavan-3-ol derivatives
The A-ring and B-ring of flavan-3-ols can be structurally modified or changed, thereby forming many novel catechin conjugates and derivatives, such as A-ring-substituted and B-ring-substituted flavan-3-ols.
A-ring substituted flavan-3-ols
Different substituent groups generally conjugate with A-rings of flavan-3-ols at C8 or C6, producing many A-ring-substituted flavan-3-ols, including flavoalkaloids, acylated and caffeoylated flavan-3-ols, phenylpropanoidated flavan-3-ols, and carboxymethyl and carboxyl flavan-3-ols. Great progress has been made in research on the above-mentioned flavonoids, particularly flavoalkaloids.
(1) Flavoalkaloids
Flavoalkaloids generally refer to chemical compounds with a typical flavonoid skeleton linked with a nitrogen containing 5-membered ring substituted at C6 or/and C8 position of the A-ring of the flavonoid core structure (Cheng et al. Citation2018). Among them, 8-C N-ethyl-2-pyrrolidinone-substituted flavan-3-ols (EPSFs) are the most representative (). Till date, more than 28 EPSF compounds have been reported from different teas and are leading a new research aspect in recent years. Although the EPSF content in tea is very low (generally <1‰), they have attracted much attention for their potential use as marker compounds for some processed teas and in the quality control and authentication of white and dark teas. Moreover, they also exhibit some strong bioactivities, such as anti-inflammatory properties and protective effects in human microvascular endothelial cells (Dai et al. Citation2020).
Figure 2. Characteristics structural modification of tea flavonoids.
(A) Structure of major novel flavoalkaloids in teas; (B) Microbial biotransformation of the tea polyphenols during the pile-fermentation of dark teas; (C) Identified derivatives of polyphenol compounds formed in the pile-fermentation of dark teas; (D) Oxidation in tea catechins; (E) Structure of anthocyanins in tea; (F) Common O- and C-glycosylated tea flavonoids; (G) Common O- and C-methylated tea flavonoids. The conjugation of flavan-3-ols and other tea phytochemicals forming flavoalkaloids, particularly 8-C N-ethyl-2-pyrrolidinone-substituted flavan-3-ols (EPSFs). In addition to these conjugates, in dark teas, flavan-3-ol derivatives are commonly identified. Anthocyanins are particular hyperaccumulated in purple-leaf teas, including pelargonidin, cyanidin, delphinidin, and their 3-O-glycosides. Commonly tea flavonoids, particularly flavonols and several flavones are easily being structurally modified at 3, 6,7, or 8 positions producing O-/C-glycosylated flavonoids. Furthermore, structurally modified at 5 and 7 positions of A-ring, 3’, 4’, 5’ positions of B-ring, and 3 and 5 positions of C-ring are observed, that producing methylated flavonoids. In addition to the naturally occurring structural modification, theaflavins, theasinensins, thearubigins, theabrownines and other flavonoid derivatives are usually observed in fermented teas in details.
Thus far, flavoalkaloids have been identified in various types of tea. The first EPSF compound was identified in black tea in 2005 as a new black tea polyphenol having N-ethyl-2-pyrrolidinone moiety, namely ethylpyrrolidinonyl theasinensin A (Tanaka et al. Citation2005). Recently, some new flavoalkaloids have also been identified in Chinese Yunnan black tea ‘Jinya’, including (−)-6-(5″S)-N-ethyl-2-pyrrolidinone-epiafzelechin, (−)-8-(5″R)-N-ethyl-2-pyrrolidinone-epiafzelechin-3-O-gallate, and (−)-8-(5″S)-N-ethyl-2-pyrrolidinone-epiafzelechin-3-O-gallate (Li et al. Citation2020a). In addition, several flavoalkaloids have also been identified in different green teas; for instance, seven EPSFs are marker compounds for stored green tea, as their concentration increased the most significantly within 19 months among the 127 identified compounds (Dai et al. Citation2020). Furthermore, five new flavalkaloids, (−)-6-(5′′S)-N-ethyl-2-pyrrolidinone-epicatechin-3-O-gallate, (−)-6-(5′′R)-N-ethyl-2-pyrrolidinone-epicatechin-3-O-gallate, (−)-8-(5′′S)-N-ethyl-2-pyrrolidinone-epicatechin-3-O-gallate, (−)-8-(5′′S)-N-ethyl-2-pyrrolidinone-catechin-3-O-gallate, and (−)-8-(5′′R)-N-ethyl-2-pyrrolidinone-catechin-3-O-gallate, have also been identified in the ancient Chinese cultivated tea Xi-Gui (Cheng et al. Citation2018). Recently, two new dihydropyrrole-substituted epicatechins, (−)-6-(5″R)-2″-ethoxy-3″,4″-dihydro-2H-pyrrole-epicatechin-3-gallate and (−)-6-(5″R)-2″-ethoxy-3″,4″-dihydro-2H-pyrrole-epicatechin, have also been identified in sun-dried Dongting Biluochun tea, which is one of the most famous green tea products in China (Kang et al. Citation2021). Meanwhile, two novel flavoalkaloids, (−)-6-(5″-S)-N-ethyl-2-pyrrolidinone-epigallocatechin-O-gallate and (−)-6-(5″-R)-N-ethyl-2-pyrrolidinone-epigallocatechin-O-gallate, and new naturally occurring flavoalkaloid, (−)-8-N-ethyl-2-pyrrolidinone-epigallocatechin-O-gallate, have been isolated from white tea (C. sinensis) (Li et al. Citation2018). Yellow and dark teas also contain flavoalkaloids, particularly EPSFs. For example, substituted flavan-3-ols are used as marker compounds for the classification of all large-leaf yellow tea samples as their concentration rapidly increase with the increase in processing temperature (Zhou et al. Citation2019). Eight novel EPSFs (puerins I–VIII) have been found in Pu-erh teas (Wang et al. Citation2014), which are regarded as the marker compounds of Chinese dark teas.
It is worth noting that flavoalkaloids have also been identified in freash tea leaves. Recently, five novel cinnamoylated flavoalkaloids, including (−)-6-(5‴S)-N-ethyl-2-pyrrolidinone-3-O-cinnamoylepicatechin, (−)-6-(5‴R)-N-ethyl-2-pyrrolidinone-3-O-cinnamoylepicatechin, (−)-8-(5‴S)-N-ethyl-2-pyrrolidinone-3-O-cinnamoylepicatechin, and (−)-8-(5‴R)-N-ethyl-2-pyrrolidinone-3-O-cinnamoylepicatechin, have been detected in fresh Fuding-Dabai, Huangjingui, and Zimudan leaves (Gaur et al. Citation2020). In addition, several EPSFs have been identified in air-dried leaves of C. sinensis var. pubilimba leaves (Meng et al. Citation2018), and similarly, some flavoalkaloids have been detected in both fresh tea leaves and white teas (Li et al. Citation2018). These findings are reasonably suggesting that part of flavoalkaloids is possibly naturally originating from fresh tea leaves. Obviously, the foundation of these tea flavoalkaloids in fresh leaves urges further research that will uncover the origin of these flavoalkaloids, and their successively exaggeration during tea processing through nonenzymatic interaction are needed in the near future.
(2) Other A-ring-substituted flavan-3-ols
In addition to flavoalkaloids, other A-ring-substituted flavan-3-ols, including acylated, caffeoylated, phenylpropanoidated, carboxymethyl and carboxyl flavan-3-ols, have also been identified in dark teas, particularly in Pu-erh tea (). Early reports have revealed the presence of two new 8-C substituted flavan-3-ols (puerins A and B) and two known cinchonain-type phenols (epicatechin-[7,8-bc]-4α-(4-hydroxyphenyl)-dihydro-2(3H)-pyranone and cinchonain lb) in Pu-erh tea (Zhou et al. Citation2005; Dong et al. Citation2008). Moreover, in 2014, four new phenylpropanoid-substituted flavan-3-ols, including (+)-gallocatechin-[8,7-e]-4α-(2,4,5-tri-hydroxyphenyl)-dihydro-2(3H)- pyranone, (-)-gallocatechin-[8,7-e]-4α-(2,4,5-trihydroxy-phenyl)-dihydro-2(3H)-pyranone, (-)-epigallocatechin-[8,7-e]-4β-(2,4,5-trihydroxyphenyl)-dihydro-2(3H)-pyranone, and (+)-epigallocatechin-[8,7-e]-4β-(2,4,5-trihydroxyphenyl)-dihydro-2(3H)-pyranone, and four new carboxymethyl- and carboxyl-substituted flavan-3-ols, including 8-carboxymethyl-(+)-catechin, 8-carboxymethyl-(+)-catechin methyl ester, 6-carboxymethyl-(+)-catechin, and 6-carboxyl-(−)-gallocatechin, have been identified in Pu-erh teas (Tao et al. Citation2014; Tian et al. Citation2014).
Furthermore, three monomeric acylated flavan-3-ols and four new acylated flavan-3-ols have been obtained from oolong tea (Hashimoto, Nonaka, and Nishioka Citation1987), and three hydroxycinnamoylated catechins, including (-)-epicatechin 3-O-caffeoate (a caffeoylated catechin), epigallocatechin3-O-p-coumaroate, and epigallocatechin-3-O-ferulate, with strong acetylcholinesterase (AChE) inhibitory activity, have been identified in Zijuan green tea (Wang et al. Citation2017). It is quite in blur about the chemical properties of these flavonoid derivatives, and studies on their formation mechanism are urgently needed.
B-Ring substituted flavan-3-ols
B-ring substituted flavan-3-ols are rarely reported than the A-ring substituted flavan-3-ols. The formation of these B-ring substituted flavan-3-ols shows a strong connection with the action of microorganisms involved in dark tea post-fermentation. Previously, Jiang et al. (Citation2011) isolated a new epicatechin oxidation product with a 3,6-dihydro-6-oxo-2H-pyran-2-carboxylic acid moiety from a commercially available post-fermented tea produced by microbial fermentation of green tea, and proposed a production mechanism that includes the oxygenative cleavage of the catechol B-ring of (-)-epicatechin. Recently, B-ring fusion lactones of flavan-3-ols have been systematically explored in Fuzhuan teas. Two new B-ring fission lactones of flavan-3-ols, namely fuzhuanins A and B, have been identified in Fuzhuan brick tea, and the former was indicated as one of the major characteristic constituents generated in the fungal fermentation process (Luo et al. Citation2013). Moreover, four new B-ring fission metabolites of flavan-3-ols, Fuzhuanins C-F, have also been identified in post-fermented Jing-Wei Fuzhuan brick tea (Zhu et al. Citation2015). In addition, teadenols A and B, which were isolated from tea leaves fermented with Aspergillus sp (Wulandari et al. Citation2011) and Pu-erh tea (Su et al. Citation2016), were identified as B-ring fission products originating from the autocleavage of EGCG and GCG respectively, by enzymatic reaction in the microbial fermentation process of tea leaves. Similarly, another two new phenolic compounds, namely teasperol which was identified in Chinese Liu-pao tea and teasperin which were from a Japanese tea product, seemed to be metabolites which come from tea catechins, such as EGCG, with the microbial fermentation of tea leaves (Kanegae et al. Citation2013). Thus, the B-ring substituted flavan-3-ols are only reported limited in these microbial fermented teas, suggesting that microbial fermentation is crucial for the formation of these chemical compounds.
Condensed & oxidized flavan-3-ol derivatives: TFs, TSs, and PAs
Flavan-3-ols are usually oxidized and polymerized into TFs, which are promising antioxidants, and the chemical reaction commonly occurs during the tea fermentation process under polyphenol oxidase (PPO) and peroxidase (POD) catalysis, particularly during black tea manufacturing (Sharma et al. Citation2020) (). Besides, multiple products, including iso- and neo-theavin, theavate A and B, isotheavin-3′-O-gallate, neotheavate-3-O-gallate, theavic acids, theagallins, and methylated theavins (Drynan et al. Citation2012), can also be formed during black tea fermentation in addition to TFs, according to the bimolecular reaction between a catechol and pyrogallol (Li et al. Citation2013). TFs can be further transformed into thearubigins (TRs) through long-term fermentation, which also greatly influence the color of tea soup and produced tea products. Although TRs are more condensed than TFs and individual catechins, they can still further condense into theabrownins (TBs), which are polysaccharides-, proteins-, lipids- or caffeine-conjugated complexes (Wang et al. Citation2016). As one of characteristic but unique highly oxidized and condensed catechin derivatives in dark teas, it is still difficult to identify their accurate chemical structures.
Individual catechins can directly undergo dimerization by C–C bonds between B-rings (at their B-rings), forming TSs. The TSs, which are also defined as biflavanols, are formed via the dimerization of two epigallocatechin-type derivatives that contain a 2′-2′ interflavonoid bond forming a biphenyl function (Stodt and Engelhardt Citation2013; Engelhardt Citation2020). TSs were first explored in green tea but widely found in black and oolong teas, accounting for around 1.0-1.5% (Xu et al. Citation2014; Ng et al. Citation2018). Currently, six TSs, TSA (EGCG dimer) and its isomer TSE, TSB (EGCG and EGC dimer), TSC (EGC dimer) and TSD (EGCG dimer), and TSF (EGCG and ECG dimer) are often discovered in fermented teas (Weerawatanakorn et al. Citation2015). As similar types of oxidation products originating from catechins, the formation competitions between TFs and TSs have been primarily studied (Xu et al. Citation2014). The findings that more individuals of TSs have been identified in partially fermented oolong tea are worthy further studied, which may promise a clear mechanism in explanation of their formation in the future. As confirmed, all the TSs are colorless, while other catechin oxidized products contributed to color of produced tea products and fermented tea soups. Furthermore, TSs have strong health-promoting activities, for instance, antioxidant, anticancer, anti-microbial, and lipid lowering activities, possess high bio-accessibility, and have recently been emphasized by researchers (Hou et al. Citation2010).
Due to the contribution of sharp astringency and mouth-drying feeling of tea infusions and their health-promoting effects, colorless PAs have obtained increasing attention in recent years. PAs are well known constituents of condensed flavan-3-ols, which commonly exist as B-types with C–C linkages between C4→C8 and C4→C6, with their average content in green and oolong teas being approximately 2-3% (Meng et al. Citation2019). As a structurally complex subclass of flavonoid compounds, PAs are considered as natural antioxidants and tyrosinase inhibitors (Song et al. Citation2017). It has been confirmed that the levels of tea PAs could be promoted by a Sucrose-Induced MYB transcription factor (Wang et al. Citation2019a), and furtherly uncovered their metabolism mechanism in Camellia sinensis (Wang et al. Citation2020). More than 20 PAs have been isolated and accurately identified, and commonly occurring polymers based on the monomer units catechin and epicatechin (procyanidins (PC)) and/or gallocatechin and epigallocatechin [prodelphinidins (PD)] are found in very low concentrations in teas (Fraser et al. Citation2012). The chemical structures of common PAs are shown in in detail.
Pigmented flavonoids: Anthocyanins
Anthocyanins are composed of an anthocyanidin (mainly malvidin, cyanidin, pelargonidin, petunidin, and delphinidin) conjugated with 1-3 sugar molecules, including glucose, galactose, xylose, arabinose, and rhamnose (Tang et al. Citation2021) (). They are major water-soluble pigments considered to be the most characteristic chemical compounds endowing fresh tea leaves with attractive colors, and play a critical role in determining the color intensity of purple tea. Anthocyanin content generally increases with increase in the intensity of the purple color of the buds (Liu et al. Citation2018; Hu et al. Citation2020). The total anthocyanin content in 9 freeze-dried purple-colored tea leaves is almost >16.6-fold higher than that in three freeze-dried ordinary green-colored tea leaves, and 33 anthocyanins have been identified in purple teas using widely targeted metabolomics in our newest results, with delphinidin 3-O-galactoside and cyanidin 3-O-galactoside being the most abundant (Shi et al. Citation2021a).
Anthocyanin-rich purple teas exhibit antioxidant, immunostimulatory, and anticancer activities, demonstrating their potential as a nutraceutical product (Lv et al. Citation2015). Anthocyanin-rich tea products have been attracting global interest (Wei et al. Citation2019), and great progress has been made in the studies of anthocyanin-rich teas in the last 20 years (Kilel et al. Citation2013). Green tea processing technology can effectively retain the vast majority of the anthocyanins originally present in the fresh leaves as compared to others processing methods (Lv et al. Citation2015), and therefore, purple tea leaves should be prepared into unfermented green tea instead of fermented black tea to preserve the high level of anthocyanins in the final tea products (Hu et al. Citation2020).
Flavonols and flavones
Except for tea catechins, teas are also rich in other subclasses of flavonoids, including flavonols and flavones. Flavonols and flavones are the vast majority of flavonoids distributed across plants, where they are predominantly conjugated with glucose and rhamnose as mono-, di- and tri-glycosides (). Flavonols, which occurs as the second abundant in all types of teas, have a hydroxyl group in position 3 of the C ring. Commonly, the major flavonols, including quercetin, kaempferol, myricetin, and their glycosides (mono-, di-, and tri-), make up approximately 0.5-2.5% (w/w) extract as aglycones in tea infusions (2.5 g dry tea brewed in 250 mL boiled water) (Panche, Diwan, and Chandra Citation2016). Compared to flavonols, flavones have a hydroxyl group at position 5 of the A-ring, while being hydroxylated at other positions, for the most part in position 7 of the A-ring or 3′ and 4′ of the B-ring. Luteolin and apigenin are the major constituents of flavones in tea (Ma et al. Citation2013). With the vast development of analysis technologies, several structurally modified flavonols and flavones have been identified in great numbers, which are mainly including glycosylated, prenylated, acetylated, and methylated modifications (Davies et al. Citation2018). It’s well known that the chemical features and biological activities of flavonoids are greatly influenced by the number and nature of the substituents affiliated to the basic structures (Halbwirth Citation2010). The consequence of these structural modifications is the alteration of bioactivities, performance, and even in vivo metabolism as compared to flavonoid aglycones. These glycosylated and methylated derivatives have been continuously explored, and vast progress have achieved along the decades.
Glycosylated flavonoids
Generally, natural flavonoids are the most abundant polyphenols in plant-derived foods and beverages, particularly in most case stably stored in their glycoside forms (Xiao Citation2017). Glycosidation can act as a better accumulation strategy in teas and greatly impact on tea flavor. Flavonoid glycosides, such as naringenin-7-O-glucoside, quercetin-3-O-galactoside, and kaemferol-3-O-(6″-O-p-courmaroyl)-glucoside, could be evaluated as the contributors of high bitterness and astringency in August teas (Zhu et al. Citation2020). Furthermore, the existence of various flavonoid glycosides can greatly stabilize and enhance the final color of tea products (Shi et al. Citation2021a).
Owing to the advantage of the current identification approach, many of C- and O-glycosylated flavonoids have been identified (Shi et al. Citation2021b), which are commonly found glycosylation at positions of 3 and 7. For example, in our newest results, myricetin, quercetin, luteolin, apigenin, and kaempferol, were identified by a widely targeted metabolomics strategy with various glycosylated derivatives, including 7 myricetin glycosides (all O-glycosylated forms), 25 quercetin glycosides (all O-glycosylated forms), 6 luteolin glycosides (including four C-glycosylated forms), 12 apigenin glycosides (including nine C-glycosylated forms), and 22 kaempferol glycosides (including one C-glycosylated forms) in dark teas.
Methylated flavonoids and other modified flavonoids
Although methylated flavonoids are less abundant than flavonoid aglycone and flavonoid glycosides, they are widely distributed in plants and tea leaves. They were reported as two common methylation patterns, which are C- and O- methylation of flavonoids, and many C- and O-methylated flavonoids have been identified (Wen et al. Citation2017) (). The O-methylation of flavonoids can enhance their antiallergic, anticancer, and cardioprotective effects (Ma et al. Citation2013), and the unraveling of methylated catechins, kaempferol, and quercetin, and anthocyanins were scheduled and progress were achieved (Lv et al. Citation2014; Shi et al. Citation2021b). In addition, we have attempted to explore the methylated flavonoid in dark teas which undergone microbial fermentation, and characteristic methylated derivatives, including 3′,4′,5-trihydroxy-7-methoxyflavone, 3′,4′,7-trihydroxy-5-methoxyflavone-7-O-β-D-glucopyranoside, and 3′,4′,7-trihydroxy-5-methoxyflavone, were identified in Pu-erh tea extract (Lv et al. Citation2010). Since flavonoids have drawn increasing attention due to their contribution to tea flavor and bioactivities, other modified derivatives have also been intensively studied in addition to the glycosylated and methylated forms. For example, novel acylated flavonol tetraglycosides, such as kaempferol 3-O-[(E)-p-coumaroyl-(1→2)][α-L-arabinopyranosyl-(1→3)][β-D-glucopyranosyl (1→3)-α-L-rhamnopyranosyl(1→6)]-β-D-glucopyranoside, have been isolated and structurally confirmed in Lu’an Guapian tea, a famous Chinese green tea (Bai et al. Citation2017), and two unique acylated flavonoid tetraglycosides named teaghrelin-1 and teaghrelin-2 in Chinese Chin-shin oolong tea (Lo et al. Citation2014).
A simplified flavonoid biosynthesis pathway map was self-organized by combining with others (Zhao et al. Citation2020) aimed at clearly demonstrating their origins, occurrence and metabolism, as shown in . To date, >7000 flavonoids have been identified through MS-based metabolomics strategy, however, because of the limited availability of authentic standards for peak identification in tandem MS or MSn, the comprehensive identification or annotation of flavonoids remains a great challenge (Akimoto et al. Citation2017). To expand the library information of plant flavonoids which also include specialized flavonoids in tea plants, the database of probable mass fragments for > 6800 known flavonoids (FsDatabase) can be a promising library in the future. Considering the demands of flavonoids and their numerous derivatives needed to be explored in detail, standards and external standard methods are widely used in the current analysis of metabolites with an efficient extraction method as an essential prerequisite. Based on the information of massive tea flavonoid standards, it can be scheduled to draw tea flavonoid fingerprint chromatography, which will be promising in providing a scientific basis for geographical traceability and genuine examination.
Figure 3. Simplified scheme of major tea flavonoids biosynthesis in fresh leaves. Flavonoid biosynthesis pathways are vital for tea plants. Owing to the unraveling of tea genomes, crucial genes and enzymes involved in tea flavonoid metabolism pathways have been studied in detail and well demonstrated.
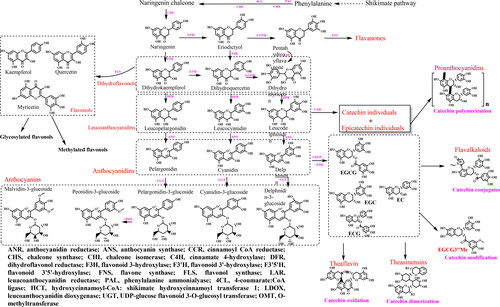
Dynamics of tea flavonoids during tea processing and during their storage period
Tea flavonoids behaved significantly difference in constituents and concentrations depending on their originating tea cultivars, plantation conditions, multiple stresses involving in preharvest, the tea-specified manufacturing steps involving in postharvest processing, and even the long-term storage period (). In this review, we tentatively concluded the dynamics of flavonoids during tea processing and storage with their detailed concentrations ().
Figure 4. Tea flavonoids biosynthesis and transformation during tea manufacturing and their storage. Simplified scheme of transformation of major tea flavonoids during tea manufacturing and their storage have been illustrated. Tea flavonoids are biosynthesized and stored in tea fresh leaves, their constituents and concentrations are easily affected by multiple factors, particularly plantation environments, plunk conditions, and (a)biotic stress. In addition, tea processing and storing can also greatly changes tea flavonoids from their original constituents.
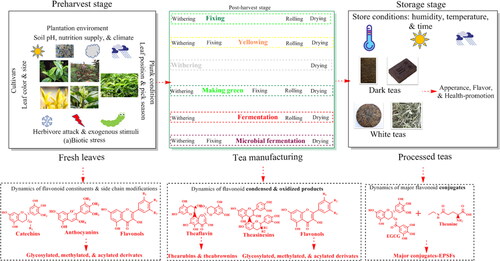
The distribution of tea flavonoids in fresh leaves
The concentration of tea flavonoids, particularly catechins, in fresh tea leaves differs greatly depending upon the tea cultivars (Fang et al. Citation2021), plantation environmental conditions (Wei et al. Citation2018), plucking seasons (Deka et al. Citation2021), and even the response to various exogenous stimuli (Shi et al. Citation2021c).
Difference of tea flavonoids in various cultivars
The tea cultivar is the most dominant factor determining the flavonoids, and significant differences in the constituents and concentrations of flavonoids from different cultivars are widely observed (Zhao et al. Citation2020; Wang et al. Citation2021). There are two major cultivated tea plant varieties: C. sinensis var. sinensis (CSS; Chinese type) and Camellia sinensis var. assamica (CSA; Assam type). The former is generally a small-leaf slower-growing shrub with adapted to extreme climates, while the latter is a large-leaf quick-growing shrub adapted to warm climates. Around 67% planted elite tea plant cultivars in China belong to CSS, which can produce quality green tea, while CSA is more suitable for black teas production (Wei et al. Citation2018). The genetic background of the cultivars can generally determine flavonoids accumulation patterns. Till date, the clear exploration of tea genomic has been done, and the unraveled flavonoid-related genes promised clear accumulation patterns of catechins and flavonol glycosides (Xia et al. Citation2017). ECG and flavonol di-glycosides particularly accumulated in CSA, while EGCG and flavonol tri-glycosides accumulated in CSS (Fang et al. Citation2021). ECG/EGCG and flavonol tri-glycosides/flavonol di-glycosides ratios have been used as potential indicators to distinguish the different varieties and cultivars.
Specialized tea cultivars rich in some desired naturally occurring catechin individuals have been widely screened in recent decades. The findings of these special tea cultivars laid the foundation for the development of potential tea products. The analysis of 403 accessions of representative tea germplasms collected from various locations in China revealed that the CSA had the highest levels of ECG and EC, whereas the Camellia sinensis var. pubilimba variety (CSP) had the highest levels of EGCG, GC, C, and GCG (Jin et al. Citation2014). EGCG is generally the dominant catechin and receives the most public attention, accounting for about 50% of tea catechins and possesses the most outstanding potential for antioxidant effects. Notably, five tea resources containing high EGCG (>15%) were screened among 109 tea germplasms, with the highest concentration being 16.24%. As an EGCG isomer, GCG possesses higher bioactivity than EGCG in some aspects, such as anti-allergy effect and Squalene Epoxidase inhibition, and our results showed that the GCG content in five tea cultivars among 200 tea germplasms was >1.5% (Lv et al. Citation2008). In particular, the GCG content of CSP is 6 − 9 times higher than that of CAS and CSS (Jin et al. Citation2014). Interestingly, Cocoa tea (Camellia ptilophylla Chang) can possess approximately 10% GCG (Wu et al. Citation2021a). Moreover, several hundred tea plant resources have been explored to identify cultivars with a high EGCG3″Me content. Naturally occurring EGCG3″Me and EGCG4″Me were previously detected in some special oolong tea germplasms (Chiu and Lin Citation2005), and four tea cultivars (EGCG3″Me >10 mg/g) among 71 major cultivars grown in China have been successively screened (Lv et al. Citation2014).
In addition to common green leaf tea cultivars, some specialized tea cultivars have also been studied in detail in the recent 20 years. Purple-leaf tea resources are continuously being paid attention. ‘Zijuan’ is the first and most representative anthocyanin-rich tea variety bred by Chinese scholars, which has been widely cultivated in China, and its anthocyanin constituents and accumulation mechanism have been reported extensively (Mei et al. Citation2021; Li et al. Citation2021b). The anthocyanin content in tea shoots is easily affected by different plucking leaf position and seasons. The anthocyanin content in the first and second leaves is 0.602 mg/g and 0.576 mg/g, respectively, which are significantly higher than that in the fourth and fifth leaves (0.176 mg/g and 0.099 mg/g, respectively) (Lv et al. Citation2015). Interestingly, many purple-leaf tea cultivars are potentially hyper-accumulated EGCG3″Me sources, suggesting that it is promising to find the hyperaccumulation of EGCG3″Me in purple-leaf tea cultivars (Shi et al. Citation2021a). In addition, Hongyacha, a novel wild tea plant discovered in the Fujian province of China, contains abundant cis-catechins, such as gallocatechin gallate, gallocatechin-(4→8)-gallocatechin gallate, and gallocatechin-3,5-di-O-gallate (Jin et al. Citation2018). CSP has a higher content of PA content than hundreds of tea germplasm resources (Wei et al. Citation2018).
Differentiation of tea flavonoids in fresh tea leaves under multiple environmental factors and exogenous stimuli
Tea plants prefer a warm and humid climate with plenty of rainfall, diffused light, weakly acidic and well-drained soil (Ho, Lin, and Shahidi Citation2008), and are often exposed to (a)biotic stress. Flavonoids are biosynthesized in the cytosol, stored in vacuoles, and exhibit flexibility in constituents and concentrations in teas responding to multiple environmental conditions and (a)biotic stress (Zeng, Xi, and Jiang Citation2019). The accumulation of flavonoids in fresh tea leaves can greatly function in various aspects related to interactions with the environment, such as protecting tea plants against ultraviolet radiation and intensive light (Lin et al. Citation2021).
Tea plantation conditions, harvest seasons, and other environmental factors play crucial roles in flavonoid accumulation patterns in tea (). In details, it has been clarified that environmental stress, particularly light, temperature, soil pH and nutrition, and plucking seasons can greatly influence primary and secondary metabolite accumulation in tea leaves, thereby affecting tea quality (Li et al. Citation2021b; Shao et al. Citation2021).
Table 2. Tea flavonoids interact with gut microbiota and metabolized products.
Among all environmental factors impacting tea flavonoids, light and soil nutrition are possibly the most important (Dong et al. Citation2019). Light, including intensity and quality, can greatly alter the constituents and concentrations of flavonoids in fresh tea leaves (Zhao et al. Citation2021). A detailed understanding of these light-induced improvements in flavonoid production mechanisms in tea plants is highly important, given the common application of shading to tea plants to mediate tea production quality (Zheng et al. Citation2019). Proofs confirm that both red and blue light promote tea catechin production; however, UV-A and UV-B can decrease flavonol glycosides, such as kaempferol-7-O-glucoside, myricetin-3-O-glucoside, and quercetin-7-O-glucoside, which are strongly related to the bitter and astringent flavor in tea, and promote non-galloylated catechins and anthocyanins production (Lin et al. Citation2021). Moreover, UV light treatments stimulate anthocyanin accumulation in ‘Ziyan’ leaves, which is another novel anthocyanin-rich tea cultivar with dark purple young shoots in China, and the anthocyanin content was the highest under UV-A treatment, which was approximately 66% higher than that in the control (Li et al. Citation2020b).
In addition, flavonoids accumulate after exposing tea plants to nitrogen depletion at 10 °C, particularly at high levels of anthocyanins and 3-rutinoside-7-rhamnosides, 3,7-di-rhamanosides, and 3-rutinosde-7-rhamnosides of kaempferol and quercetin (Huang et al. Citation2018). Soil nutrients, particularly nitrogen, have been studied in detail, and normal nitrogen levels promote flavonol glycoside biosynthesis through gene regulation and substrate carbohydrate accumulation, while abnormal nitrogen availability, particularly excess nitrogen, has inhibitory effects (Dong et al. Citation2019).
Despite tea plantation environmental factors, exogenous stimuli, including carbonyl volatiles (such as methyl jasmonate and indole) (Savvides et al. Citation2016; Ye et al. Citation2021), plant hormones (such as abscisic acid) (Gai et al. Citation2020), and plant-herbivore attack (Jing et al. Citation2021), play important roles in altering flavonoids constituents and concentrations in fresh tea leaves. Results from a recent study showed that abscisic acid (ABA) application on tea plants could alleviate drought stress and induce flavonoid metabolism (Gai et al. Citation2020). Flavonoid metabolism, for example, anthocyanin synthesis, is modulated by exogenous γ-amino butyric acid (GABA) at low temperatures (Zhu et al. Citation2019). Our previous results showed that exogenous methyl jasmonate (MeJA) application on tea shoots could prime secondary metabolism in tea, particularly promoting the expression of genes and enzymes involving in phenylpropanoid pathways, which are the origin of flavonoids (Shi et al. Citation2021c). The phytohormone brassinosteroids (BRs) can greatly improve the expression of phenylalanine ammonia-lyase (PAL), which plays a crucial role in the phenylpropanoid biosynthesis pathway, recognized as a major source of flavonoid production in teas. Li et al. (Citation2021b) applied melatonin to promote tea tolerance at sub-high temperatures, and found accumulation of catechins, including C, GC, and EGCG, and also confirmed melatonin-dedicated anthocyanin biosynthesis regulation in tea. Moreover, insect attacks play a role in tea quality-related nonvolatile specialized metabolites. The feeding of two major tea insects, tea green leafhoppers and tea geometrids, on tea increased catechin and TF levels by increasing PPO activity in tea (Liao et al. Citation2019).
Furthermore, the changes in global climate have also exhibited different impact on tea production and flavor quality; for instance, heat stress, low temperature, and drought stress directly affect tea flavonoids biosynthesis, resulting in a complex difference in their constituents and levels (Han, Li, and Ahammed Citation2018). Besides, plucking seasons, including spring, summer, and autumn, impact the regular flavan-3-ols, TSAs, procyanidins, glycosylated conjugates like quercetin-O-glycosides and apigenin-C-glycosides of nine varieties, and the complexity in their difference were confirmed (Dai et al. 2015). It was reported that the anthocyanin content was significantly higher in summer than that in spring and autumn (Maritim et al. Citation2021). Interestingly, Liu et al. (Citation2020) compared the flavonoid constituents in young and mature tea leaves and observed a decrease in flavanols and an increase in flavonols during the maturation of tea leaves.
Transformations of tea flavonoids during tea processing and during storage
Tea flavonoids change greatly during tea manufacturing from fresh tea leaves to processed teas, which contribute directly to the formation of flavor and health functions of different teas. Moreover, the continuously changing of tea flavonoids that commonly conjugated with other biochemicals during storage periods were observed and in-depth uncovered.
Changes in tea flavonoids during manufacturing
Differences in tea processing technologies entail different paths of biochemical changes within the tea leaves (Fang et al. Citation2021). Enzymatic and nonenzymatic reactions involving in tea manufacturing can lead to complicated transformations in tea flavonoids, which resulted in distinguish of tea flavonoids among the various tea types (Peterson et al. Citation2005). Generally, all tea manufacturing procedures, including withering, ‘killing-green’, ‘shaking-green’, yellowing, fermentation, microbial fermentation, and drying, have great influence on tea flavonoids. Catechins are the major tea flavonoids present in tea leaves; however, their levels in various types of teas are greatly different from each other. Various factors, such as temperature, system pH, oxygen availability, and moisture involved in tea manufacturing, contribute to changes in tea catechins (Ananingsih, Sharma, and Zhou Citation2013). Tea catechins can be affected significantly by changing the temperature, duration, and their interaction (Ye et al. Citation2018). For example, temperature is the key factor that influences the GCG content in tea, and high-temperature steam treatment can effectively increase the GCG content in green tea (Lv et al. Citation2008). In addition, it has been reported that the thermal stabilities of tea catechins are in the order ECG > EGC > EC > EGCG (Okumura et al. Citation2008).
Generally, green and white tea products contain the highest catechin content, followed by oolong and yellow tea, and it mostly decomposes in dark teas (Ho, Lin, and Shahidi Citation2008). Owing to the ‘killing-green’ processing procedures that deactivated enzymes in tea leaves, tea catechin constituents remain mostly in their primitive form as in withered tea leaves. As a unique processing step, the ‘shaking-green’ procedure greatly impacts tea flavonoids, and their contents are higher in light-shaking oolong tea than those in heavy-shaking tea, while the contents of TFs and TBs showed no significant difference. During the yellowing process, ester catechins are converted into simple catechins and produced TF at the same time (Zhang et al. Citation2019a). Fermentation is a crucial step in forming the characteristic flavor of black tea, and tea catechins are oxidized to form TFs, which can be further oxidized into TRs. In recent study, black tea was processed through oxygen-enriched fermentation, which significantly improved TF-3-G and TF-3,3′-DG content (Chen et al. Citation2021). Besides, fermentation was observed decreasing the contents of flavonoid glycosides that may lower bitterness flavor of summer green tea (Guo et al. Citation2021). As a unique processing step, microbial fermentation involved in dark tea processing produced diverse flavonoids in dark teas (Li et al. Citation2021a). These chemical compounds are formed by several reactions catalyzed by microbial enzymes or occur as a result of microbial metabolism, leading to the development of dark tea quality. For instance, it has been reported that flavonoids and their glycosides are formed during Fuzhuan tea manufacture, which are associated with a series of reactions, such as degradation, oxidation, condensation, structural modification, methylation, and glycosylation (Li et al. Citation2021a). In addition, it was reported that kaempferol and myricetin methyl derivatives accumulated in ripened Pu-erh tea, while a comparison of flavonoid composition in four typical Chinese dark teas prepared from the same batch of raw tea material showed an accumulation of flavonoid glycosides in Liupao tea and Fuzhuan tea (Shi et al. Citation2021b). In addition, the last tea manufacturing procedure, namely drying, also plays an important role in tea flavonoid constituents. Flavonoid glycosylation was observed and verified during the drying process, and it was reported that 20% flavonoids are decomposed during drying process of oolong tea production (Dou et al. Citation2007).
Transformation of tea flavonoids during storage
Storage is considered a crucial process to improve the typical sensory characteristics and health functions of white and dark teas. There is a belief that the longer dark tea is kept, the more flavor it gets (Lv et al. Citation2012). In addition, it is a deep belief in China that aging could improve the quality of white tea (Zhao et al. Citation2022). Owing to their influence on the chemical composition, the effects of storage on tea phytochemicals have been widely investigated in the recent years. The chemical interactions during the long-term tea storage have been well confirmed by the isolation and identification of flavoalkaloids, which are mainly conjugates of tea catechins, theanine, and other compounds (Gaur et al. Citation2020). Tea catechins and theanine have received special attention owing to their high levels and conjugation into EPSFs in processed teas during the extension of storage periods. The EPSFs were once considered to be formed through the conjugation of catechins and theanine as precursors during tea manufacturing, and were positively correlated with storage duration (Xie et al. Citation2019). EPSFs are significantly increased during the extension of storage periods, and their formation mechanism was well explained by the in vitro chemical reaction of tea catechins and theanine under special circumstances (Dai et al. Citation2018, Citation2020).
A conversion of phenolic component in the white tea aging process has taken place, and it was found that total flavonoids increased, while total polyphenols decreased. Non-targeted metabolomics was previously conducted on the 1-, 7-, and 13-year stored white teas to profile their conversion, which showed that most metabolites were related to flavonol glycosides and flavone glycosides, and glycosyl flavonols were dynamically converted to flavonols during aging (Zhao et al. Citation2022). Similarly, it was found that the chemical profiles of Raw Pu-erh teas stored in wet-hot or dry-cold environment for 1–9 years were similar at an early stage but started to differentiate from each other at the 5th and the 7th year, and the discriminating features including flavoalkaloids, flavan-3-ols, and flavonol-O-glycosides were discovered (Xu et al. Citation2019). In addition, 47 compounds were identified as critical metabolites responsible for the variation of quality in aged Qingzhuan tea, with catechin methylation, flavonoid glycosylation, flavoalkaloid degradation, and theabrownine formation (Cheng et al. Citation2020). Furthermore, it has been reported that galloylated catechins and TF-3-G are responsible for distinguishing between short and long-time preserved Keemun black tea (Huang, Jiang, et al. Citation2021).
Current research and trends on health functions of tea flavonoids and derivatives
During the two decades, scientific research workers have systemic summarized the health functions of tea, including preventing cancer, cardiovascular disease, hypertension, hyperlipidemia, hyperglycemia, kidney disease, neurodegenerative diseases, alleviating metabolic syndrome disease, as well as anti-radiation, anti-allergy, antibacterial and anti-virus effects (Chen and Zhen Citation2014; Huang, Jiang, et al. Citation2021). As the most studied functional components, tea flavonoids have been proven to play key roles in many aspects relating to health promotion, particularly anti-inflammatory, antioxidant, antimicrobial, neuroprotective, antihypertensive, and anti-carcinogenic properties () (Xiao Citation2017; Sanlier, Gokcen, and Altuğ Citation2018; Pei, Liu, and Bolling Citation2020). It has been reported that one cup tea (2 g of tea leaves brewed in hot water for 3 min) can approximately provide 150-200 mg of flavonoids, which may play a role in preventing and managing modern chronic diseases (Zeng, Xi, and Jiang Citation2019). In the past two decades, the uptake, absorption, transportation, and interaction with the gut microbiota of tea flavonoids have been widely studied. In addition, the extensive variation between flavonoid individuals and different structural modifications of flavonoids can greatly affect their bioactivities. Based on these facts, we have summarized the updates that offer clear insights into the in vivo bioactivities of tea flavonoids.
Figure 5. Major bioactivities of tea flavonoids and mechanism of their human body utilization. (A) General bioactivities of tea flavonoids reported and general absorption and metabolism of tea flavonoids in vivo; (B) Biological fate of tea flavonoids in human body. CsF, represents tea flavonoids; CsF-aglycone represents the aglycone forms of CsF. Tea flavonoids are reported with various bioactivities. They are commonly recognized as digested with the help of organs, but in fact the largest portion of tea flavonoids are bio-transformed to small molecules under gut microbiota which are more easily absorbed.
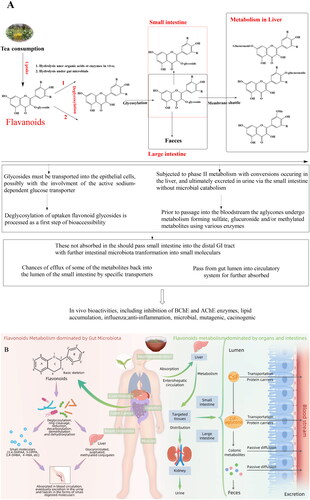
Progress in metabolic syndrome alleviation of tea flavonoids
Accumulating evidence suggests that flavonoids have various biological properties, and particularly the anti-inflammatory effects of flavan-3-ols and metabolic syndrome alleviation effects of flavonols are being explored intensively (Murota, Nakamura, and Uehara Citation2018; Zhang et al. Citation2019b; Wu et al. Citation2021).
Metabolic syndrome alleviation by inhibiting inflammatory of flavan-3-ols
Inflammatory processes play a central role in the development and progression of numerous pathological conditions, such as inflammatory bowel disease (IBD) and metabolic syndromes (Salaritabar et al. Citation2017). Monomeric flavan-3-ols and their oxidized or condensed products show many promising pharmacological effects, particularly anti-inflammatory activity (Xing et al. Citation2019; Huang et al. Citation2019). Principally, it is initiated by the interaction of tea flavonoids with toll-like receptors expressed on the surface of immune cells. They are then internalized into the cytosol and transferred into the nucleus, where they attach to the aryl hydrocarbon receptor (Ah-R). Subsequently, Ah-R easily binds to the Ah-R nuclear translocator, and induces beneficial protective enzymes and cytokines via the Ah response element, upregulating the anti-inflammatory system (Etxeberria et al. Citation2013; Samynathan et al. Citation2021).
Monomeric flavan-3-ols (particularly EGCG) can effectively inhibit inflammation. EGCG has been extensively investigated as an anti-inflammatory agent in IBD, which can inhibit IL-8, PGE2, and MIP-3α gene expression and release in TNF-α-stimulated human colon adenocarcinoma cell lines (Xing et al. Citation2019). In addition to flavan-3-ol monomers, their condensed products, particularly TFs, TRs, and TBs, are highly effective in attenuating metabolic syndrom (Huang et al. Citation2019; Khairudin, Mhd Jalil, and Hussin Citation2021). Very limited evidence has directly supported these flavan-3-ol condensed product anti-inflammatory bioactivities in vivo; TSs actually possesses significant anti-inflammatory properties, which have been mostly confirmed in vitro (Peluso et al. Citation2015; Koch Citation2020).
Metabolic syndromes alleviation of flavonols and their structurally modified side-chain derivatives
As major flavonols in teas, quercetin, kaempferol, and myricetin and their derivatives positively alleviate metabolic syndromes. The consumption of tea flavonoids inhibits inflammation and improves insulin resistance (IR) by inhibiting hepatic IKK/NF-κB signaling and enhancing glucose catabolism, thereby alleviating metabolic syndromes (Ren et al. Citation2019). Kaempferol and quercetin have been studied as valuable compounds that could improve insulin-stimulated glucose uptake in mature 3T3-L1 adipocytes and significantly inhibit NO production through cell models. Furthermore, myricetin has also studied in detail and confirmed to improve insulin sensitivity, decrease serum glucose and insulin levels, and reverse the defective expression of phosphoinositide 3-kinase (PI3K) regulatory subunit, which is effective in alleviating hypertriglyceridemia and hypercholesterolemia and reducing serum proinflammatory cytokine (TNF-α and IL-6) levels.
Studies in the last 20 years have provided evidence that flavonoid aglycones and their corresponding glycosides can prevent dietary fat absorption, inhibit lipid absorption, accelerate lipid metabolism, upregulate energy usage, and prevent lipid accumulation (Mulvihill and Huff Citation2012). Glycosylated products (including C- and O-glycosylation) possess many unique properties, particularly the enhancement of hydrophilicity and absorption in vivo (Wen et al. Citation2017). As summarized by Xiao (Citation2017), C-glycosylated flavonoids tend to enhance the inhibitory potential against the formation of advanced glycation end products (AGE), which are strongly associated with inflammation, aging, and particularly diabetes, while the O-glycosylated flavonoids behave in the opposite manner. Due to their large molecular size and high polarity, these glycosylated flavonoids have a low inhibitory effect against α-amylase, which is commonly diagnosed as an important marker for diabetes and hyperlipidemia in the human body. However, myricetin 3-O-rhamnoside has a strong inhibition (Manaharan et al. Citation2012). Effective α-glucosidase inhibition prevents excess glucose absorption during carbohydrate digestion in the small intestine, which results in diabetes and metabolic syndromes. Compared to flavonoid aglycones, their glycosylated forms (quercetin glycosides, hydroxyl apigenin glycosides, vitexin, etc.) greatly lower α-glucosidase inhibition depending on the conjugation position and side-chain sugar class (Kawabata et al. Citation2003). However, glycosylation at the C-3 position of kaempferol, quercetin, and myricetin significantly diminishes sorbitol accumulation in the presence of diabetes by enhancing aldose reductase (AR) inhibition (Mok and Lee Citation2013).
Wen and Walle (Citation2006a, Citation2006b) have reported that methylation could improve the membrane-crossing ability of flavonoids, prevent degradation, and increase their chemo-preventive properties compared to those of their aglycones, which could be a reasonable exploration of their enhanced antioxidant, anticancer, and immunomodulatory activities. Flavonoids commonly undergo phase I and II metabolism in the liver and small intestine, leading to flavonoid sulfate, glucuronide, and methylate formation (Gonzales et al. Citation2015; Xiao Citation2017). Methylation increases the affinity of the cell membrane for flavonoids; however, it decreases their solubility. After methylated flavonoids enter the human digestive system, they are demethylated in a process different from the common flavonoid aglycone uptake and absorption pathway. Successively, the demethylated products are readily conjugated by glucuronidation and sulfation, but not through in vivo methyltransferase-mediate methylation. Methylation of flavonoids greatly protects against rapid hepatic metabolism and improves their bioavailability and metabolic stability (Wen and Walle 2006b), which increases safety in vivo (Tsuji, Winn, and Walle Citation2006). According to Lin et al. (Citation2020), anthocyanin-accumulated purple-leaf tea can greatly ameliorate high-fat diet-induced obesity and metabolic disorders by modulating the gut microbiota in mice. Defining the importance of the abovementioned mechanisms in human chronic diseases will offer suggestions for dietary recommendations for tea flavonoid consumption.
Although structural modification could positively impact flavonoid bioactivities, tea catechin individuals are proven to have a high bioavailability (Wang, Provan, and Helliwell Citation2000). However, the concentration in human plasma is approximately 5-50 times lower than the concentrations usually used, and only 0.48% ingested catechin reach circulation within 3 h after tea consumption (Hodgson et al. Citation2014). It has been confirmed in human and animal studies that EC and (+)-C have higher bioavailability than EGCG (Mw, 458) possessing 8 -OH on rings, while the molecular weight of the formers was 290 with 3 less -OH. Chen and Yang (Citation2020) have summarized that the recovery of galloyl catechins and nongalloyl catechins were 11% and 28%, respectively. These results have also been proven by the oral administration of green tea extracts or catechins in rat models (Chow and Hakim Citation2011). However, EGCG have been tested with higher bioavailability in mice models by the confirmation of its peak concentration in human and rat plasma could reach 7.5 μmol L−1 and 2 ∼ 9 μmol L−1, respectively when orally administrating with high dose (Lambert et al. Citation2006; Chow et al. 2005). In addition, TF (9 -OH, Mw 564) and TF-3,3′-DG (14 -OH, Mw 868) with extremely low bioavailability (nearly zero in vivo; Murota, Nakamura, and Uehara Citation2018) were tested. The low tea flavonoid utilization efficiency directly by human organs greatly urges the exploration of gut microbiota to improve their utilization. Due to the low bioavailability of dietary flavonoid compounds in the small intestine (Thilakarathna and Rupasinghe Citation2013), their metabolism by gut microbiota is gaining increasing attention.
Potential mechanisms of in vivo uptake, absorption, and improved bioavailability of tea flavonoids cooperated with gut microbiota
Since the unraveling of the human gastrointestinal (GI) tract, it has been confirmed that the gut microbiota plays a crucial role in human health (de Vos and de Vos Citation2012; Zmora et al. Citation2018). Among trillions of microorganisms identified in humans, Firmicutes, Bacteroidetes, Proteobacteria, Actinobacteria, Verrucomicrobia, and Fusobacteria are the dominant species (Sender, Fuchs, and Milo Citation2016). Alterations in the gut microbiota are linked to the development of chronic diseases such as obesity, IBD, and metabolic syndromes (Loo et al. Citation2020). Diet plays an important role in initiating, shaping, and modulating gut microbiota, which can play a prominent role in human health. It is critical to comprehensively understand the interactions and modulation between tea flavonoids and gut microbiota, successfully promoting human health.
These flavonoids usually undergo two main types of biotransformation in the liver and intestine, including phase I, which mainly involves functionalization hydrolysis or oxidation reactions, and phase II, which involves detoxifying conjugation reactions, such as glucuronidation or sulfation, successively leads to hydroxylation, methylation, and reduction in the liver (Tomás-Barberán and Espín Citation2019) (). Flavonoids and their glycoside are poorly absorbed in the small intestine, but it can be effectively metabolized by the colonic microflora into aglycone in the large intestine (Oesterle et al. Citation2021). The colonic microbiota plays an important role in the metabolism of flavonoids into smaller and easily absorbed phenolic acids that contribute to immune function and host metabolism modulation (Rothenberg, Zhou, and Zhang Citation2018) ().
Interaction of flavan-3-ols with gut microbiota
Recently, Chen and Yang (Citation2020) have comprehensively demonstrated the biological fates of tea polyphenols which laid emphasis on flavan-3-ols and their interactions with gut microbiota resulting in prophyclatic health effects. Flavan-3-ols are mainly absorbed in the upper small intestine by the transportation of the monocarboxylic acid transporter, and the remaining may passively diffuse directly (Neilson and Ferruzzi Citation2011). After consuming green tea, the digestive release, absorption, metabolism, and elimination of flavan-3-ol are major factors that influence its bioavailability (Jin et al. Citation2012). Pathways have been proposed for green tea flavan-3-ol degradation in the colon and the catabolism of phenolic compounds passing into the circulatory system from the large intestine. The metabolism of individual flavan-3-ols is exhibited differentially, generating a diverse plasma profile of metabolites and native forms (). Generally, once taken up by intestinal absorptive cells, flavan-3-ols are first glucuronidated by uridine diphosphate glucuronyltransferase (UDPGT) at C5, C7, and C3’ positions, and sulfation at different sites is carried out by sulfotransferase (SULT) or phenol-sulfotransferase (PST) involved in phase II metabolism (). Glucuronide, sulfate, and methyl product concentrations peak approximately 2 h after intake, and decrease after 8 h (Clifford, van der Hooft, and Crozier Citation2013; Li and de Wiele Citation2021). Approximately 1.68% ingested flavan-3-ol are present in the plasma, urine, and feces. The bioavailability of the nongallated catechins was higher than that of the gallated forms. In the latest published results, authors have uncovered in depth that oral EGCG ameliorated the colonic inflammation in a gut microbiota-dependent manner (Wu et al. Citation2021b). These findings will offer better insights into developing EGCG as a promising modulator for gut microbiota to prevent and alleviate IBD.
Although catechins possess higher absorption than TFs and TRs, their protective effects were evidenced as they ameliorated colonic architecture disruption and inflammation by modulating gut microbiota (Fuchs et al. Citation2014). PAs, the most regular condensed flavonols in teas, were also investigated as it significantly decreased proinflammatory mediators and increased the synthesis of anti-inflammatory cytokines IL-2 and IL-4. However, they were degraded into small molecules in vivo, which had nearly zero absorption in the small intestine. Intriguingly, it was found that the majority of procyanidins with a degree of polymerization ≥2 could reach the colon and were extensively metabolized by the colonic microbiota (Liu et al. Citation2018). Procyanidin B2 can be absorbed to a very limited extent. Furthermore, Liu et al. (Citation2021) reported that TSs exhibited recalcitrance to degradation with the help of human gut microbiota. Besides green tea, oolong tea, and black tea, TBs extracted from dark tea are verified in playing vital roles in protection attenuating hypercholesterolemia via modulation of gut microbiota and bile acid metabolism (Huang et al. Citation2019). Daily supplementation of 200-400 mg Fuzhuan tea extracts for eight weeks reversed the altered dominant bacterial phyla in the gut and increased the levels of Lactobacillus and Bifidobacteriaceae. Recently, health-promoting effects, such as modulating the gut microbiota and improving the abundance of Bifidobacterium, Lactobacillus, and Lactococcus, has increased the popularity of the combination of Pu-erh tea and ‘Chenpi’ (Zheng et al. Citation2020).
Interactions of flavonols, flavanones, and anthocyanins with gut microbiota
Although flavonoids possess anticancer, antimicrobial, antioxidant, and anti-inflammatory bioactivities, their variable bioavailability is <10% (Huang et al. Citation2016; Huang, Jiang, et al. Citation2021). Due to their low bioavailability in human organs, gut microbiota (such as Bacteroides fragilis, Clostridium perfringen s, Eubacterium ramulus, Streptococcus S-2, Lactobacillus L-2, Bifidobacterium B-9, and Bacteroides JY-6) have significantly promoted bioavailability through the transformation of flavonols (quercetin), flavanone (apigenin and luteolin), and anthocyanins into small biological molecules, such as 3,4-dihydroxyphenylacetic acid, 3-(3-hydroxyphenyl)propionic acid, 3,4-dihydroxybenzoic acid, and 4-hydroxybenzoic acid (Carrasco-Pozo et al. Citation2015; Santangelo, Silvestrini, and Mancuso Citation2019; Pei, Liu, and Bolling Citation2020) ().
As a naturally occurring flavone in teas, apigenin possesses both antioxidative and anti-inflammatory effects, which may be beneficial for treating IBD. Apigenin was partially excreted in the feces, and apigenin aglycone was detected in the cecum luminal content; thus, the bacterial community in the colon was exposed to dietary apigenin. Once apigenin enters the colon, it is degraded by various enzymes produced by human gut microbiota. E. ramulus, a strictly anaerobic human gut bacterium, metabolizes apigenin, quercetin, and naringenin to 3-(4-hydroxyphenyl) propionic acid. The degradation product 3-(4-hydroxyphenyl) propionic acid, also known as desaminotyrosine (DAT), is beneficial during influenza by triggering type I interferon signaling, which in turn augments antiviral responses by phagocytic cells, thereby preventing inflammation and severe disease (Wang et al. Citation2019b).
Anthocyanins and PAs are poorly absorbed in the small intestine in their intact forms (Appeldoorn et al. Citation2009). In most cases, anthocyanin absorption is dependent on the structure of the aglycone moiety. Anthocyanins are relatively unstable in the gastrointestinal tract, even under normal conditions of the small intestine, and phenolic products may be produced through anthocyanin degradation. Anthocyanins that survive the small intestine environment are rapidly transformed into low molecular weight compounds by the colonic microbiota.
A clear understanding of tea flavonoid chemistry will contribute to the understanding of their utilization. To fulfill the demand for future accurate usage of tea flavonoids with high bio-accessibility, further detailed studies are required to uncover their intake, bioavailability, and metabolism in the human body.
Conclusion and perspectives
Advanced analytical instruments and approaches can effectively promote further exploration of tea flavonoids, and sophisticated modeling of animals and humans can promote precise utilization of tea flavonoids in the near future. The continuous uncovering of tea flavonoids in chemistry, acknowledgement of the dynamics of tea flavonoids pre, during, and post manufacturing all focused on achieving an accurate and efficient utilization in the human body. This review is an update that combines the progress of research on tea flavonoids, which will greatly contribute to a comprehensive understanding and rethinking of their potential utilization in the human body. Perspective sights aimed at the purpose are as follows.
Further screening of specialized resources rich in naturally hypperaccumulated tea flavonoids
Specialized tea cultivars with high content of naturally occurring flavonoids should be screened and further cultivated; exogenous inducement and special cultivation in the preharvest stage should be applied to increase the accumulation of desired flavonoids; and tea manufacturing technologies should be promoted and storage conditions should be optimized to maintain high content of tea flavonoids in the near future. Moreover, it is meaningful to apply some convenient and effective method, such as the on-line HPLC-DPPH method (Zhang et al. Citation2013), to evaluate the preliminary antioxidant activities of newly identified tea flavonoids and their derivatives, which will aid screening the potential tea flavonoid in teas with high antioxidant activities.
Massive scale application of metabolic engineering promoting structurally modified tea flavonoids
A better understanding of tea flavonoids by the release of tea genome and secondary metabolite database will greatly promise the possibility of structurally modified tea flavonoids by applying massive scale enzymatic engineering. Currently, EGCG3’’Me and anthocyanins are widely investigated worldwide because of their health functions. The uncovering of accurate information about the related upstream genes and enzymes dominating ECGC3’’Me and anthocyanins biosynthesis can greatly ensure an achievable metabolic engineering. It is necessary and realistic to design accurate gene clustering to enrich the desired tea flavonoids for medical usage through metabolic engineering. With the promise of accurate identification of novel tea flavonoids, it is potential to prepare more structurally modified tea flavonoids with precise metabolic engineering approaches, and successively increasing their yields.
Innovate nanoparticle system promoting tea flavonoid bioavailability
Human intervention studies have shown that it is urgently need to improve the bioavailability of tea flavonoids in the human body and creating a new nanoparticle system to load tea flavonoids is essential and promising for this purpose. Nanotechnology has been widely used for its potential to improve human health and well-being, and has attracted considerable attention in the agriculture and food sectors. Considering consumer demand for healthy and natural carrier materials, there is growing interest in developing natural, renewable, and sustainable plant-based environment friendly nanomaterials, such as nanocelluloses, and edible oil-based lipid nanoparticles, which has significantly improved the bioavailability of flavonoids. These nanomaterials are particularly suitable for altering the physicochemical, sensory, and nutritional properties of food-derived compounds. Hence, designing new nanoparticle system creation and optimizing existing systems that can load targeted bioactive tea compounds, including flavonoids, needs to be scheduled.
CRediT authorship contribution statement
Jiang Shi: Investigation, Writing – original draft. Gaozhong Yang: Investigation, Writing – original draft. Qiushuang You: Investigation, Writing – original draft. Shili Sun: Writing – review & editing. Ruohong Chen: Writing – review & editing. Zhi Lin: Writing – review & editing. Jesus Simal-Gandara: Conceptualization, Writing – review & editing. Haipeng Lv: Conceptualization, Writing – review & editing. All authors reviewed the manuscript. Jiang Shi and Gaozhong Yang contributed equally to this work.
Supplemental Material
Download ()Disclosure statement
No potential conflict of interest was reported by the authors.
Additional information
Funding
References
- Akimoto, N., T. Ara, D. Nakajima, K. Suda, C. Ikeda, S. Takahashi, R. Muneto, M. Yamada, H. Suzuki, D. Shibata, et al. 2017. FlavonoidSearch: A system for comprehensive flavonoid annotation by mass spectrometry. Scientific Reports 7 (1):1–9. doi: 10.1038/s41598-017-01390-3.
- Ananingsih, V. K., A. Sharma, and W. Zhou. 2013. Green tea catechins during food processing and storage: A review on stability and detection. Food Research International 50 (2):469–79. doi: 10.1016/j.foodres.2011.03.004.
- Appeldoorn, M. M., J. P. Vincken, A. M. Aura, P. C. H. Hollman, and H. Gruppen. 2009. Procyanidin dimers are metabolized by human microbiota with 2-(3,4-dihydroxyphenyl)acetic acid and 5-(3,4-dihydroxyphenyl)-gamma-valerolactone as the major metabolites. Journal of Agricultural and Food Chemistry 57 (3):1084–92. doi: 10.1021/jf803059z. doi: 10.1021/jf803059z.
- Bai, W. X., C. Wang, Y. J. Wang, W. J. Zheng, W. Wang, X. C. Wan, and G. H. Bao. 2017. Novel acylated flavonol tetraglycoside with inhibitory effect on lipid accumulation in 3t3-l1 cells from lu’an guapian tea and quantification of flavonoid glycosides in six major processing types of tea. Journal of Agricultural and Food Chemistry 65 (14):2999–3005. doi: 10.1021/acs.jafc.7b00239.
- Carrasco-Pozo, C., M. Gotteland, R. L. Castillo, and C. Chen. 2015. 3,4-Dihydroxyphenylacetic acid, a microbiota-derived metabolite of quercetin, protects against pancreatic β-cells dysfunction induced by high cholesterol. Experimental Cell Research 334 (2):270–82. doi: 10.1016/j.yexcr.2015.03.021.
- Cheng, J., F. H. Wu, P. Wang, J. P. Ke, X. C. Wan, M. H. Qiu, and G. H. Bao. 2018. Flavoalkaloids with a pyrrolidinone ring from Chinese ancient cultivated tea Xi-Gui. Journal of Agricultural and Food Chemistry 66 (30):7948–57. doi: 10.1021/acs.jafc.8b02266.
- Cheng, L., Q. Yang, Z. Chen, J. Zhang, Q. Chen, Y. Wang, and X. Wei. 2020. Distinct changes of metabolic profile and sensory quality during Qingzhuan tea processing revealed by LC-MS-based metabolomics. Journal of Agricultural and Food Chemistry 68 (17):4955–65. doi: 10.1021/acs.jafc.0c00581.
- Chen, L., F. Liu, Y. Yang, Z. Tu, J. Lin, Y. Ye, and P. Xu. 2021. Oxygen-enriched fermentation improves the taste of black tea by reducing the bitter and astringent metabolites. Food Research International (Ottawa, ON) 148:110613. doi: 10.1016/j.foodres.2021.110613.
- Chen, T. T., and C. S. Yang. 2020. Biological fates of tea polyphenols and their interactions with microbiota in the gastrointestinal tract: Implications on health effects. Critical Reviews in Food Science and Nutrition 60 (16):2691–709. doi: 10.1080/10408398.2019.1654430.
- Chen, Z. M., and Y. S. Zhen. 2014. Health functions of tea. 1st ed. Beijing, China. Science Press. (In Chinese).
- Chiu, F. L., and J. K. Lin. 2005. HPLC analysis of naturally occurring methylated catechins, 3’ ‘- and 4’ ‘-methyl-epigallocatechin gallate, in various fresh tea leaves and commercial teas and their potent inhibitory effects on inducible nitric oxide synthase in macrophages. Journal of Agricultural and Food Chemistry 53 (18):7035–42. doi: 10.1021/jf0507442.
- Chow, H. H. S., and I. A. Hakim. 2011. Pharmacokinetic and chemoprevention studies on tea in humans. Pharmacological Research 64 (2):105–12. doi: 10.1016/j.phrs.2011.05.007. doi: 10.1016/j.phrs.2011.05.007.
- Chow, H. H. S., I. A. Hakim, D. R. Vining, J. A. Crowell, J. Ranger-Moore, W. M. Chew, C. A. Celaya, S. R. Rodney, Y. Hara, and D. S. Alberts. 2005. Effects of dosing condition on the oral bioavailability of green tea catechins after single-dose administration of polyphenon E in healthy individuals. Clinical Cancer Research: An Official Journal of the American Association for Cancer Research 11 (12):4627–33. doi: 10.1158/1078-0432.CCR-04-2549.
- Clifford, M. N., J. J. van der Hooft, and A. Crozier. 2013. Human studies on the absorption, distribution, metabolism, and excretion of tea polyphenols. The American Journal of Clinical Nutrition 98 (6 Suppl):1619S–30S. doi: 10.3945/ajcn.113.058958.
- Dai, W. D., N. Lou, D. C. Xie, Z. Y. Hu, H. Y. Song, M. L. Lu, D. Shang, W. L. Wu, J. k Peng, P. Y. Yin, et al. 2020. N-Ethyl-2-pyrrolidinone-substituted flavan-3-ols with anti-inflammatory activity in lipopolysaccharide-stimulated macrophages are storage-related marker compounds for green tea. Journal of Agricultural and Food Chemistry 68 (43):12164–72. doi: 10.1021/acs.jafc.0c03952.
- Dai, W. D., D. D. Qi, T. Yang, H. P. Lv, L. Guo, Y. Zhang, Y. Zhu, Q. H. Peng, D. C. Xie, J. F. Tan, et al. 2015. Nontargeted analysis using ultraperformance liquid chromatography-quadrupole time-of-flight mass spectrometry uncovers the effects of harvest season on the metabolites and taste quality of tea (Camellia sinensis L.). Journal of Agricultural and Food Chemistry 63 (44):9869–78. doi: 10.1021/acs.jafc.5b03967.
- Dai, W. D., J. F. Tan, M. L. Lu, Y. Zhu, P. L. Li, Q. H. Peng, L. Guo, Y. Zhang, D. C. Xie, Z. Y. Hu, et al. 2018. Metabolomics investigation reveals that 8-C N-ethyl-2-pyrrolidinone-substituted flavan-3-ols are potential marker compounds of stored white teas. Journal of Agricultural and Food Chemistry 66 (27):7209–18. doi: 10.1021/acs.jafc.8b02038.
- Davies, K. M., N. W. Albert, Y. F. Zhou, and K. E. Schwinn. 2018. Functions of flavonoid and betalain pigments in abiotic stress tolerance in plants. Annual Plant Reviews Online 1 (1):21–62. doi: 10.1002/9781119312994.apr0604.
- de Vos, W. M., and E. A. de Vos. 2012. Role of the intestinal microbiome in health and disease: From correlation to causation. Nutrition Reviews 70 (suppl_1):S45–S56. doi: 10.1111/j.1753-4887.2012.00505.x.
- Deka, H., T. Barman, J. Dutta, A. Devi, P. Tamuly, R. K. Paul, and T. Karak. 2021. Catechin and caffeine content of tea (Camellia sinensis L.) leaf significantly differ with seasonal variation: A study on popular cultivars in North East India. Journal of Food Composition and Analysis 96:103684. doi: 10.1016/j.jfca.2020.103684.
- Dong, F., J. H. Hu, Y. Z. Shi, M. Y. Liu, Q. F. Zhang, and J. Y. Ruan. 2019. Effects of nitrogen supply on flavonol glycoside biosynthesis and accumulation in tea leaves (Camellia sinensis). Plant Physiology and Biochemistry: PPB 138:48–57. doi: 10.1016/j.plaphy.2019.02.017.
- Dong, F., Z. Y. Yang, P. M. He, and Z. Lin. 2008. Liquid chromatographic-mass spectrometric analysis of antioxidant compounds from Pu-erh tea. Journal of Chinese Institute of Food Science and Technology 8 (2):133–41. doi: 10.16429/j.1009-7848.2008.02.003.
- Dou, J., V. S. Lee, J. T. Tzen, and M. R. Lee. 2007. Identification and comparison of phenolic compounds in the preparation of oolong tea manufactured by semifermentation and drying processes. Journal of Agricultural and Food Chemistry 55 (18):7462–8. doi: 10.1021/jf0718603.
- Drynan, J. W., M. N. Clifford, J. Obuchowicz, and N. Kuhnert. 2012. MALDI-TOF mass spectrometry: Avoidance of artifacts and analysis of caffeine-precipitated SII thearubigins from 15 commercial black teas. Journal of Agricultural and Food Chemistry 60 (18):4514–25. doi: 10.1021/jf205125y.
- Engelhardt, U. H. 2020. Tea chemistry - What do and what don’t we know? - A micro review. Food Research International (Ottawa, ON) 132:109120. doi: 10.1016/j.foodres.2020.109120.
- Engelhardt, U. H., C. Lakenbrink, and O. Pokorny. 2003. Proanthocyanidins, bisflavanols, and hydrolyzable tannins in green and black teas. In Nutraceutical beverages, ed. F. Shahidi, and D. K. Weerasinghe, 254–64. Washington, DC: American Chemical Society. https://pubs.acs.org/doi/abs/10.1021/bk-2004-0871.ch019.
- Etxeberria, U., A. Fernández-Quintela, F. I. Milagro, L. Aguirre, J. A. Martínez, and M. P. Portillo. 2013. Impact of polyphenols and polyphenol-rich dietary sources on gut microbiota composition. Journal of Agricultural and Food Chemistry 61 (40):9517–33. doi: 10.1021/jf402506c.
- Fang, Z. T., W. T. Yang, C. Y. Li, D. Li, J. J. Dong, D. Zhao, H. R. Xu, J. H. Ye, X. Q. Zheng, Y. R. Liang, et al. 2021. Accumulation pattern of catechins and flavonol glycosides in different varieties and cultivars of tea plant in China. Journal of Food Composition and Analysis 97:103772. doi: 10.1016/j.jfca.2020.103772.
- Fraser, K., S. J. Harrison, G. A. Lane, D. E. Otter, Y. Hemar, S.-Y. Quek, and S. Rasmussen. 2012. HPLC–MS/MS profiling of proanthocyanidins in teas: A comparative study. Journal of Food Composition and Analysis 26 (1–2):43–51. doi: 10.1016/j.jfca.2012.01.004.
- Fuchs, D., Y. De Graaf, R. Van Kerckhoven, and R. Draijer. 2014. Effect of tea theaflavins and catechins on microvascular function. Nutrients 6 (12):5772–85. doi: 10.3390/nu6125772.
- Gai, Z., Y. Wang, Y. Ding, W. Qian, C. Qiu, H. Xie, L. Sun, Z. Jiang, Q. Ma, L. Wang, et al. 2020. Exogenous abscisic acid induces the lipid and flavonoid metabolism of tea plants under drought stress. Scientific Reports 10 (1):12275. doi: 10.1038/s41598-020-69080-1.
- Gaur, R., J. P. Ke, P. Zhang, Z. Yang, and G. H. Bao. 2020. Novel cinnamoylated flavoalkaloids identified in tea with acetylcholinesterase inhibition effect. Journal of Agricultural and Food Chemistry 68 (10):3140–8. doi: 10.1021/acs.jafc.9b08285.
- Gonzales, G. B., G. Smagghe, C. Grootaert, M. Zotti, K. Raes, and J. Van Camp. 2015. Flavonoid interactions during digestion, absorption, distribution and metabolism: A sequential structure-activity/property relationship-based approach in the study of bioavailability and bioactivity. Drug Metabolism Reviews 47 (2):175–90. doi: 10.3109/03602532.2014.1003649.
- Guo, X.-Y., Y.-Q. Lv, Y. Ye, Z.-Y. Liu, X.-Q. Zheng, J.-L. Lu, Y.-R. Liang, and J.-H. Ye. 2021. Polyphenol oxidase dominates the conversions of flavonol glycosides in tea leaves. Food Chemistry 339:128088. doi: 10.1016/j.foodchem.2020.128088.
- Halbwirth, H. 2010. The creation and physiological relevance of divergent hydroxylation patterns in the flavonoid pathway. International Journal of Molecular Sciences 11 (2):595–621. doi: 10.3390/ijms11020595.
- Han, W. Y., X. Li, and G. J. Ahammed. 2018. Stress physiology of tea in the face of climate change. 1st ed. Singapore: Springer. doi: 10.1007/978-981-13-2140-5.
- Hashimoto, F., G. I. Nonaka, and I. Nishioka. 1987. Tannins and related compounds. lvi. isolation of four new acylated flavan-3-ols from oolong tea. Chemical and Pharmaceutical Bulletin 35 (2):611–6. doi: 10.1248/cpb.35.611.
- He, H. F., K. Wei, J. F. Yin, and Y. Ye. 2021. Insight into tea flavonoids: Composition and chemistry. Food Reviews International 37 (8):812–23. doi: 10.1080/87559129.2020.1721530.
- Ho, C. T., J. K. Lin, and F. Shahidi. 2008. Tea and tea products: Chemistry and health-promoting properties. 1st ed. Boca Raton: CRC press. doi: 10.1201/9781420008036.
- Hodgson, A. B., R. K. Randell, K. Mahabir-Jagessar-T, S. Lotito, T. Mulder, D. J. Mela, A. E. Jeukendrup, and D. M. Jacobs. 2014. Acute effects of green tea extract intake on exogenous and endogenous metabolites in human plasma. Journal of Agricultural and Food Chemistry 62 (5):1198–208. doi: 10.1021/jf404872y.
- Hou, D. X., S. Masuzaki, S. Tanigawa, F. Hashimoto, J. H. Chen, T. Sogo, and M. Fujii. 2010. Oolong tea theasinensins attenuate cyclooxygenase-2 expression in lipopolysaccharide (LPS)-activated mouse macrophages: Structure-activity relationship and molecular mechanisms. Journal of Agricultural and Food Chemistry 58 (24):12735–43. doi: 10.1021/jf103605j.
- Hu, J. G., L. J. Zhang, Y. Y. Sheng, K. R. Wang, Y. L. Shi, Y. R. Liang, and X. Q. Zheng. 2020. Screening tea hybrid with abundant anthocyanins and investigating the effect of tea processing on foliar anthocyanins intea. Folia Horticulturae 0 (0):279–90. doi: 10.2478/fhort-2020-0025.
- Huang, J. C., L. Chen, B. Xue, Q. Y. Liu, S. Y. Ou, Y. Wang, and X. C. Peng. 2016. Different flavonoids can shape unique gut microbiota profile in vitro. Journal of Food Science 81 (9):H2273–H2279. doi: 10.1111/1750-3841.13411.
- Huang, A., Z. D. Jiang, M. Tao, M. C. Wen, Z. P. Xiao, L. Zhang, M. Y. Zha, J. Y. Chen, Z. Q. Liu, and L. Zhang. 2021. Targeted and nontargeted metabolomics analysis for determining the effect of storage time on the metabolites and taste quality of keemun black tea. Food Chemistry 359:129950. doi: 10.1016/j.foodchem.2021.129950.
- Huang, Y. N., K. Y. Xing, L. Qiu, Q. L. Wu, and H. Wei. 2021. Therapeutic. implications of functional tea ingredients for ameliorating inflammatory bowel disease: A focused review. Critical Reviews in Food Science and Nutrition:1–15. doi: 10.1080/10408398.2021.1884532.
- Huang, H., Q. Y. Yao, E. H. Xia, and L. Z. Gao. 2018. Metabolomics and transcriptomics analyses reveal nitrogen influences on the accumulation of flavonoids and amino acids in young shoots of tea plant (Camellia sinensis L.) Associated with Tea Flavor. Journal of Agricultural and Food Chemistry 66 (37):9828–38. doi: 10.1021/acs.jafc.8b01995.
- Huang, F., X. Zheng, X. Ma, R. Jiang, W. Zhou, S. Zhou, Y. Zhang, S. Lei, S. Wang, J. Kuang, et al. 2019. Theabrownin from Pu-erh tea attenuates hypercholesterolemia via modulation of gut microbiota and bile acid metabolism. Nature Communications 10 (1):4971. doi: 10.1038/s41467-019-12896-x.
- Jiang, H. Y., T. Shii, Y. Matsuo, T. Tanaka, Z. H. Jiang, and I. Kouno. 2011. A new catechin oxidation product and polymeric polyphenols of post-fermented tea. Food Chemistry 129 (3):830–6. doi: 10.1016/j.foodchem.2011.05.031.
- Jin, J. Q., Y. F. Chai, Y. F. Liu, J. Zhang, M. Z. Yao, and L. Chen. 2018. Hongyacha, a naturally caffeine-free tea plant from Fujian, China. Journal of Agricultural and Food Chemistry 66 (43):11311–9. doi: 10.1021/acs.jafc.8b03433.
- Jing, T. T., W. K. Du, T. Gao, Y. Wu, N. Zhang, M. Y. Zhao, J. Y. Jin, J. M. Wang, W. Schwab, X. C. Wan, et al. 2021. Herbivore-induced DMNT catalyzed by CYP82D47 plays an important role in the induction of JA-dependent herbivore resistance of neighboring tea plants. Plant, Cell & Environment 44 (4):1178–91. doi: 10.1111/pce.13861.
- Jin, J. Q., J. Q. Ma, C. L. Ma, M. Z. Yao, and L. Chen. 2014. Determination of catechin content in representative Chinese tea germplasms. Journal of Agricultural and Food Chemistry 62 (39):9436–41. doi: 10.1021/jf5024559.
- Jin, J. S., M. Touyama, T. Hisada, and Y. Benno. 2012. Effects of green tea consumption on human fecal microbiota with special reference to Bifidobacterium species. Microbiology and Immunology 56 (11):729–39. doi: 10.1111/j.1348-0421.2012.00502.x.
- Kanegae, A., A. Sakamoto, H. Nakayama, Y. Nakazono, I. Yakashiro, Y. Matsuo, T. Tanaka, and K. Ishimaru. 2013. New phenolic compounds from Camellia sinensis L. fermented leaves. Journal of Natural Medicines 67 (3):652–6. doi: 10.1007/s11418-012-0704-5.
- Kang, N. X., J. P. Zhao, Y. Zhou, Z. R. Peng, Y. L. Liu, Q. M. Xu, I. A. Khan, and S. L. Yang. 2021. New dihydropyrrole-substituted epicatechins from sun-dried Dongting-Biluochun tea leaves and their inhibitory activities on acetylcholinesterase. ACS Food Science & Technology 1 (3):310–5. doi: 10.1021/acsfoodscitech.1c00057.
- Kawabata, J., K. Mizuhata, E. Sato, T. Nishioka, Y. Aoyama, and T. Kasai. 2003. 6-Hydroxyflavonoids as alpha-glucosidase inhibitors from marjoram (Origanum majorana) leaves . Bioscience, Biotechnology, and Biochemistry 67 (2):445–7. doi: 10.1271/bbb.67.445.
- Khairudin, M. A. S., A. M. Mhd Jalil, and N. Hussin. 2021. Effects of polyphenols in tea (Camellia sinensis sp.) on the modulation of gut microbiota in human trials and animal studies. Gastroenterology Insights 12 (2):202–16. doi: 10.3390/gastroent12020018.
- Kilel, E. C., A. K. Faraj, J. K. Wanyoko, F. N. Wachira, and V. Mwingirwa. 2013. Green tea from purple leaf coloured tea clones in kenya- their quality characteristics. Food Chemistry 141 (2):769–75. doi: 10.1016/j.foodchem.2013.03.051.
- Koch, W. 2020. Theaflavins, thearubigins, and theasinensins. In Handbook of dietary phytochemicals, ed. J. B. Xiao, S. D. Sarker, and Y. Asakawa, 1–29. Singapore: Springer. doi: 10.1007/978-981-13-1745-3_20-1.
- Lambert, J. D., M. J. Lee, L. Diamond, J. Ju, J. Hong, M. Bose, H. L. Newmark, and C. S. Yang. 2006. Dose-dependent levels of epigallocatechin-3-gallate in human colon cancer cells and mouse plasma and tissues. Drug Metabolism and Disposition: The Biological Fate of Chemicals 34 (1):8–11. doi: 10.1124/dmd.104.003434. doi: 10.1124/dmd.104.003434.
- Li, X., G. J. Ahammed, X. N. Zhang, L. Zhang, P. Yan, L. P. Zhang, J. Y. Fu, and W. Y. Han. 2021b. Melatonin-mediated regulation of anthocyanin biosynthesis and antioxidant defense confer tolerance to arsenic stress in Camellia sinensis L. Journal of Hazardous Materials 403:123922. doi: 10.1016/j.jhazmat.2020.123922.
- Liao, Y. Y., Z. M. Yu, X. Y. Liu, L. T. Zeng, S. H. Cheng, J. L. Li, J. C. Tang, and Z. Y. Yang. 2019. Effect of major tea insect attack on formation of quality-related nonvolatile specialized metabolites in tea (Camellia sinensis) Leaves. Journal of Agricultural and Food Chemistry 67 (24):6716–24. doi: 10.1021/acs.jafc.9b01854.
- Liao, Y. Y., X. C. Zhou, and L. T. Zeng. 2020. How does tea (Camellia sinensis) produce specialized metabolites which determine its unique quality and function: A review. Critical Reviews in Food Science and Nutrition:1–17. doi: 10.1080/10408398.2020.1868970.
- Li, Q. Q., and T. V. de Wiele. 2021. Gut microbiota as a driver of the. interindividual variability of cardiometabolic effects from tea polyphenols. Critical Reviews in Food Science and Nutrition:1–27. doi: 10.1080/10408398.2021.1965536.
- Li, Q., Y. L. Jin, R. G. Jiang, Y. Q. Xu, Y. Y. Zhang, Y. Luo, J. N. Huang, K. B. Wang, and Z. H. Liu. 2021a. Dynamic changes in the metabolite profile and taste characteristics of Fu brick tea during the manufacturing process. Food Chemistry 344:128576. doi: 10.1016/j.foodchem.2020.128576.
- Li, X., G. J. Liu, W. Zhang, Y. L. Zhou, T. J. Ling, X. C. Wan, and G. H. Bao. 2018. Novel flavoalkaloids from white tea with inhibitory activity against the formation of advanced glycation end products. Journal of Agricultural and Food Chemistry 66 (18):4621–9. doi: 10.1021/acs.jafc.8b00650.
- Li, S. M., C. Y. Lo, M. H. Pan, C. S. Lai, and C. T. Ho. 2013. Black tea: Chemical analysis and stability. Food and Function 4 (1):10–8. doi: 10.1039/c2fo30093a.
- Lin, N., X. Y. Liu, W. F. Zhu, X. Cheng, X. H. Wang, X. C. Wan, and L. L. Liu. 2021. Ambient ultraviolet B signal modulates tea flavor characteristics via shifting a metabolic flux in flavonoid biosynthesis. Journal of Agricultural and Food Chemistry 69 (11):3401–14. doi: 10.1021/acs.jafc.0c07009.
- Lin, Y. C., H. F. Lu, J. C. Chen, H. C. Huang, Y. H. Chen, Y. S. Su, C. Y. Tung, and C. Huang. 2020. Purple-leaf tea (Camellia sinensis L.) ameliorates high-fat diet induced obesity and metabolic disorder through the modulation of the gut microbiota in mice. BMC Complementary Medicine and Therapies 20 (1):376. doi: 10.1186/s12906-020-03171-4.
- Li, W., L. Tan, Y. Zou, X. Tan, J. Huang, W. Chen, and Q. Tang. 2020b. The effects of ultraviolet A/B treatments on anthocyanin accumulation and gene expression in dark-purple tea cultivar ‘Ziyan’ (Camellia sinensis). Molecules 25 (2):354. doi: 10.3390/molecules25+020354.
- Liu, Z. B., M. E. Bruins, W. J. C. de Bruijn, and J. P. Vincken. 2020. A comparison of the phenolic composition of old and young tea leaves reveals a decrease in flavanols and phenolic acids and an increase in flavonols upon tea leaf maturation. Journal of Food Composition and Analysis 86:103385. doi: 10.1016/j.jfca.2019.103385.
- Liu, Z. B., W. J. C. de Bruijn, M. G. Sanders, S. S. Wang, M. E. Bruins, and J. P. Vincken. 2021. Insights in the recalcitrance of theasinensin A to human gut microbial degradation. Journal of Agricultural and Food Chemistry 69 (8):2477–84. doi: 10.1021/acs.jafc.1c00727.
- Liu, L. F., X. Yi, A. Liu, W. J. Xiao, and Z. H. Gong. 2018. Research progress of tea anthocyanin. Journal of Tea Communication 45 (1):3–8. (In Chinese). doi: 10.3969/j.issn.1009-525X.2018.01.001.
- Li, N., H. T. Zhu, D. Wang, M. Zhang, C. R. Yang, and Y. J. Zhang. 2020a. New flavoalkaloids with potent α-glucosidase and acetylcholinesterase inhibitory activities from Yunnan black tea ‘Jin-Ya’. Journal of Agricultural and Food Chemistry 68 (30):7955–63. doi: 10.1021/acs.jafc.0c02401.
- Lo, Y.-H., Y.-J. Chen, C.-I. Chang, Y.-W. Lin, C.-Y. Chen, M.-R. Lee, V. S. Y. Lee, and J. T. C. Tzen. 2014. Teaghrelins, unique acylated flavonoid tetraglycosides in chin-shin oolong tea, are putative oral agonists of the ghrelin receptor. Journal of Agricultural and Food Chemistry 62 (22):5085–91. doi: 10.1021/jf501425m.
- Loo, Y. T., K. Howell, M. Chan, P. Z. Zhang, and K. Ng. 2020. Modulation of the human gut microbiota by phenolics and phenolic fiber-rich foods. Comprehensive Reviews in Food Science and Food Safety 19 (4):1268–9. doi: 10.1111/1541-4337.12563.
- Luo, Z. M., H. X. Du, L. X. Li, M. Q. An, Z. Z. Zhang, X. C. Wan, G. H. Bao, L. Zhang, and T. J. Ling. 2013. Fuzhuanins A and B: The B-ring fission lactones of flavan-3-ols from Fuzhuan Brick-Tea. Journal of Agricultural and Food Chemistry 61 (28):6982–90. doi: 10.1021/jf401724w.
- Luo, Y., S. S. Yu, J. Li, Q. Li, K. B. Wang, J. N. Huang, and Z. H. Liu. 2018. Molecular characterization of WRKY transcription factors that act as negative regulators of O-methylated catechin biosynthesis in tea plants (Camellia sinensis L.). Journal of Agricultural and Food Chemistry 66 (43):11234–43. doi: 10.1021/acs.jafc.8b02175.
- Lv, H. P., W. D. Dai, J. F. Tan, L. Guo, Y. Zhu, and Z. Lin. 2015. Identification of the anthocyanins from the purple leaf coloured tea cultivar Zijuan (Camellia sinensis var. assamica) and characterization of their antioxidant activities. Journal of Functional Foods 17:449–58. doi: 10.1016/j.jff.2015.05.043.
- Lv, H. P., Z. Lin, Q. S. Zhong, and L. Wang. 2010. Study on the chemical component of E8 fraction from pu-erh tea. Journal of Tea Science 30 (6):423–8. (In Chinese). doi: 10.13305/j.cnki.jts.2010.06.014.
- Lv, H. P., J. F. Tan, L. Guo, J. P. Gu, and Z. Lin. 2008. Study on the GCG in green tea. Journal of Tea Science 28 (2):79–82. (In Chinese).
- Lv, H. P., T. Yang, C. Y. Ma, C. P. Wang, J. Shi, Y. Zhang, Q. H. Peng, J. F. Tan, L. Guo, and Z. Lin. 2014. Analysis of naturally occurring 3″-Methyl-epigallocatechin gallate in 71 major tea cultivars grown in China and its processing characteristics. Journal of Functional Foods 7:727–36. doi: 10.1016/j.jff.2013.12.009.
- Lv, H. P., Q. S. Zhong, Z. Lin, L. Wang, J. F. Tan, and L. Guo. 2012. Aroma characterisation of Pu-erh tea using headspace-solid phase microextraction combined with GC/MS and GC–olfactometry. Food Chemistry 130 (4):1074–81. doi: 10.1016/j.foodchem.2011.07.135.
- Ma, C. Y., H. P. Lv, X. Z. Zhang, Z. M. Chen, J. Shi, M. L. Lu, and Z. Lin. 2013. Identification of regioisomers of methylated kaempferol and quercetin by ultra high performance liquid chromatography quadrupole time-of-flight (UHPLC-QTOF) tandem mass spectrometry combined with diagnostic fragmentation pattern analysis. Analytica Chimica Acta 795:15–24. doi: 10.1016/j.aca.2013.07.038.
- Maeda-Yamamoto, M., K. Ema, M. Monobe, Y. Tokuda, and H. Tachibana. 2012. Epicatechin-3-O-(3″-O-methyl)-gallate content in various tea cultivars (Camellia sinensis L.) and its in vitro inhibitory effect on histamine release. Journal of Agricultural and Food Chemistry 60 (9):2165–70. doi: 10.1021/jf204497b.
- Manaharan, T., D. Appleton, H. M. Cheng, and U. D. Palanisamy. 2012. Flavonoids isolated from Syzygiumaqueum leaf extract as potential antihyperglycaemicagents. Food Chemistry 132 (4):1802–7. doi: 10.1016/j.foodchem.2011.11.147.
- Maritim, T. K., M. Masand, R. Seth, and R. K. Sharma. 2021. Transcriptional analysis reveals key insights into seasonal induced anthocyanin degradation and leaf color transition in purple tea (Camellia sinensis (L.) O. Kuntze). Scientific Reports 11 (1):1–14. doi: 10.1038/s41598-020-80437-4.
- Mei, Y., H. Xie, S. R. Liu, J. Y. Zhu, S. Q. Zhao, and C. L. Wei. 2021. Metabolites and transcriptional profiling analysis reveal the molecular mechanisms of the anthocyanin metabolism in the “Zijuan” tea plant (Camellia sinensis var. assamica). Journal of Agricultural and Food Chemistry 69 (1):414–27. doi: 10.1021/acs.jafc.0c06439.
- Meng, X. H., N. Li, H. T. Zhu, D. Wang, C. R. Yang, and Y. J. Zhang. 2019. Plant resources, chemical constituents, and bioactivities of tea plants from the genus Camellia section Thea. Journal of Agricultural and Food Chemistry 67 (19):5318–49. doi: 10.1021/acs.jafc.8b05037.
- Meng, X. H., H. T. Zhu, H. Yan, D. Wang, C. R. Yang, and Y. J. Zhang. 2018. C-8 N-Ethyl-2-pyrrolidinone-substituted flavan-3-ols from the leaves of Camellia sinensis var. pubilimba. Journal of Agricultural and Food Chemistry 66 (27):7150–5. doi: 10.1021/acs.jafc.8b02066.
- Mok, S. Y., and S. Lee. 2013. Identification of flavonoids and flavonoid rhamnosides from Rhododendron mucronulatum for. albiflorum and their inhibitory activities against aldose reductase. Food Chemistry 136 (2):969–74. doi: 10.1016/j.foodchem.2012.08.091.
- Mulvihill, E. E., and M. W. Huff. 2012. Protection from metabolic dysregulation, obesity, and atherosclerosis by citrus flavonoids: Activation of hepatic PGC1α-mediated fatty acid oxidation. PPAR Research 2012:857142. doi: 10.1155/2012/857142.
- Murota, K., Y. Nakamura, and M. Uehara. 2018. Flavonoid metabolism: The interaction of metabolites and gut microbiota. Bioscience, Biotechnology, and Biochemistry 82 (4):600–10. doi: 10.1080/09168451.2018.1444467.
- Neilson, A. P., and M. G. Ferruzzi. 2011. Influence of formulation and processing on absorption and metabolism of flavan-3-ols from tea and cocoa. Annual Review of Food Science and Technology 2:125–51. doi: 10.1146/annurev-food-022510-133725.
- Ng, K. W., Z. J. Cao, H. B. Chen, Z. Z. Zhao, L. Zhu, and T. Yi. 2018. Oolong tea: A critical review of processing methods, chemical composition, health effects, and risk. Critical Reviews in Food Science and Nutrition 58 (17):2957–80. doi: 10.1080/10408398.2017.1347556.
- Oesterle, I., D. Braun, D. Berry, L. Wisgrill, A. Rompel, and B. Warth. 2021. Polyphenol exposure, metabolism, and analysis: A global exposomics perspective. Annual Review of Food Science and Technology 12:461–84. doi: 10.1146/annurev-food-062220-090807.
- Okumura, H., M. Ichitani, T. Takihara, and K. K. Kunimoto. 2008. Effect of cyclodextrins on the thermal epimerization of tea catechins. Food Science and Technology Research 14 (1):83–8. doi: 10.3136/fstr.14.83.
- Panche, A. N., A. D. Diwan, and S. R. Chandra. 2016. Flavonoids: An overview. Journal of Nutritional Science 5:e47. doi: 10.1017/jns.2016.41.
- Pei, R. S., X. C. Liu, and B. Bolling. 2020. Flavonoids and gut health. Current Opinion in Biotechnology 61:153–9. doi: 10.1016/j.copbio.2019.12.018.
- Peluso, I., C. Miglio, G. Morabito, F. Ioannone, and M. Serafini. 2015. Flavonoids and immune function in human: A systematic review. Critical Reviews in Food Science and Nutrition 55 (3):383–95. doi: 10.1080/10408398.2012.656770.
- Peterson, J., J. Dwyer, S. Bhagwat, D. Haytowitz, J. Holden, A. L. Eldridge, G. Beecher, and J. Aladesanmi. 2005. Major flavonoids in dry tea. Journal of Food Composition and Analysis 18 (6):487–501. doi: 10.1016/j.jfca.2004.05.006.
- Preedy, V. R. 2013. Tea in health and disease prevention. London: Academic Press. doi: 10.1016/C2010-0-64948-0.
- Ren, N., E. Kim, B. Li, H. B. Pan, T. T. Tong, C. S. Yang, and Y. Y. Tu. 2019. Flavonoids alleviating insulin resistance through inhibition of inflammatory signaling. Journal of Agricultural and Food Chemistry 67 (19):5361–73. doi: 10.1021/acs.jafc.8b05348.
- Rothenberg, D. O. N., C. B. Zhou, and L. Y. Zhang. 2018. A review on the weight-loss effects of oxidized tea polyphenols. Molecules 23 (5):1176. doi: 10.3390/molecules23051176.
- Salaritabar, A., B. Darvishi, F. Hadjiakhoondi, A. Manayi, A. Sureda, S. F. Nabavi, L. R. Fitzpatrick, S. M. Nabavi, and A. Bishayee. 2017. Therapeutic potential of flavonoids in inflammatory bowel disease: A comprehensive review. World Journal of Gastroenterology 23 (28):5097–114. doi: 10.3748/wjg.v23.i28.5097.
- Samynathan, R., M. Thiruvengadam, S. H. Nile, M. A. Shariati, M. Rebezov, R. K. Mishra, B. Venkidasamy, S. Periyasamy, I. Chung, and M. Paterio. 2021. Recent insights on tea metabolites, their biosynthesis and chemo-preventing effects: A review. Critical Reviews in Food Science and Nutrition:1–20. doi: 10.1080/10408398.2021.1984871.
- Sanlier, N., B. B. Gokcen, and M. Altuğ. 2018. Tea consumption and disease correlations. Trends in Food Science & Technology 78:95–106. doi: 10.1016/j.tifs.2018.05.026.
- Santangelo, R., A. Silvestrini, and C. Mancuso. 2019. Ginsenosides, catechins, quercetin and gut microbiota: Current evidence of challenging interactions. Food and Chemical Toxicology: An International Journal Published for the British Industrial Biological Research Association 123:42–9. doi: 10.1016/j.fct.2018.10.042.
- Savvides, A., S. Ali, M. Tester, and V. Fotopoulos. 2016. Chemical priming of plants against multiple abiotic stresses: Mission possible? Trends in Plant Science 21 (4):329–40. doi: 10.1016/j.tplants.2015.11.003.
- Sender, R., S. Fuchs, and R. Milo. 2016. Revised estimates for the number of human and bacteria cells in the body. PLoS Biology 14 (8):e1002533. doi: 10.1371/journal.pbio.1002533.
- Shao, C. Y., C. Y. Zhang, Z. D. Lv, and C. W. Shen. 2021. Pre-and post-harvest exposure to stress influence quality-related metabolites in fresh tea leaves (Camellia sinensis). Scientia Horticulturae 281:109984. doi: 10.1016/j.scienta.2021.109984.
- Sharma, N., H. T. Phan, M. Chikae, Y. Takamura, A. F. Azo‐Oussou, and M. D. C. Vestergaard. 2020. Black tea polyphenol theaflavin as promising antioxidant and potential copper chelator. Journal of the Science of Food and Agriculture 100 (7):3126–35. doi: 10.1002/jsfa.10347.
- Shi, J., W. J. Ma, C. P. Wang, W. L. Wu, J. Tian, Y. Zhang, Y. L. Shi, J. T. Wang, Q. H. Peng, Z. Lin, et al. 2021b. Impact of various microbial-fermented methods on the chemical profile of dark tea using a single raw tea material. Journal of Agricultural and Food Chemistry 69 (14):4210–22. doi: 10.1021/acs.jafc.1c00598.
- Shi, J., J. Simal-Gandara, J. F. Mei, W. J. Ma, Q. H. Peng, Y. L. Shi, Q. Xu, Z. Lin, and H. P. Lv. 2021a. Insight into the pigmented anthocyanins and the major potential co-pigmented flavonoids in purple-coloured leaf teas. Food Chemistry 363:130278. doi: 10.1016/j.foodchem.2021.130278.
- Shi, J., J. T. Wang, H. P. Lv, Q. H. Peng, M. Schreiner, S. Baldermann, and Z. Lin. 2021c. Integrated proteomic and metabolomic analyses reveal the importance of aroma precursor accumulation and storage in methyl jasmonate-primed tea leaves. Horticulture Research 8 (1):1–14. doi: 10.1038/s41438-021-00528-9.
- Song, W., X. F. Zhu, X. D. Ding, H. B. Yang, S. T. Qin, H. Chen, and S. D. Wei. 2017. Structural features, antioxidant and tyrosinase inhibitory activities of proanthocyanidins in leaves of two tea cultivars. International Journal of Food Properties 20 (6):1348–58. doi: 10.1080/10942912.2016.1209682.
- Stodt, U., and U. H. Engelhardt. 2013. Progress in the analysis of selected tea constituents over the past 20 years. Food Research International 53 (2):636–48. doi: 10.1016/j.foodres.2012.12.052.
- Su, X. Q., G. J. Zhang, Y. Ma, M. Chen, S. H. Chen, S. M. Duan, J. Q. Wan, F. Hashimoto, H. P. Lv, J. H. Li, et al. 2016. Isolation, identification, and biotransformation of teadenol a from solid state fermentation of pu-erh tea and in vitro antioxidant activity. Applied Sciences 6 (6):161. doi: 10.3390/app6060161.
- Tanaka, T., S. Watarumi, M. Fujieda, and I. Kouno. 2005. New black tea polyphenol having N-ethyl-2-pyrrolidinone moiety derived from tea amino acid theanine: Isolation, characterization and partial synthesis. Food Chemistry 93 (1):81–7. doi: 10.1016/j.foodchem.2004.09.013.
- Tang, H., J. C. Tang, J. Y. Liu, B. Zhou, and Y. Y. Chen. 2021. Metabolomics analyses reveal anthocyanins-rich accumulation in naturally mutated purple-leaf tea (Camellia sinensis L.). All Life 14 (1):744–55. doi:10.1080/26895293.2021.1968509.
- Tao, M. K., M. Xu, H. T. Zhu, R. R. Cheng, D. Wang, C. R. Yang, and Y. J. Zhang. 2014. New phenylpropanoid-substituted flavan-3-ols from Pu-er ripe tea. Natural Product Communications 9 (8):1167–70. doi: 10.1177/1934578X1400900827.
- Thilakarathna, S. H., and H. P. V. Rupasinghe. 2013. Flavonoid bioavailability and attempts for bioavailability enhancement. Nutrients 5 (9):3367–87. doi: 10.3390/nu5093367.
- Tian, L. W., M. K. Tao, M. Xu, J. Hu, H. T. Zhu, W. Y. Xiong, D. Wang, C. R. Yang, and Y. J. Zhang. 2014. Carboxymethyl- and carboxyl-catechins from ripe pu-er tea. Journal of Agricultural and Food Chemistry 62 (50):12229–34. doi: 10.1021/jf5036959.
- Tomás-Barberán, F. A., and J. C. Espín. 2019. Effect of food structure and processing on (poly)phenol-gut microbiota interactions and the effects on human health. Annual Review of Food Science and Technology 10:221–38. doi: 10.1146/annurev-food-032818-121615.
- Tsuji, P. A., R. N. Winn, and T. Walle. 2006. Accumulation and metabolism of the anticancer flavonoid 5,7-dimethoxyflavone compared to its unmethylated analog chrysin in the Atlantic killifish. Chemico-Biological Interactions 164 (1–2):85–92. doi: 10.1016/j.cbi.2006.08.023.
- Wang, M. Q., J. Firrman, L. S. Liu, and K. Yam. 2019b. A review on flavonoid apigenin: Dietary intake, ADME, antimicrobial effects, and interactions with human gut microbiota. BioMed Research International 2019:7010467. doi: 10.1155/2019/7010467.
- Wang, W., X. W. Fu, X. L. Dai, F. Hua, G. X. Chu, M. J. Chu, F. L. Hu, T. J. Ling, L. P. Gao, Z. W. Xie, et al. 2017. Novel acetylcholinesterase inhibitors from Zijuan tea and biosynthetic pathway of caffeoylated catechin in tea plant. Food Chemistry 237:1172–8. doi: 10.1016/j.foodchem.2017.06.011. doi: 10.1016/j.foodchem.2017.06.011.
- Wang, Q. P., J. S. Gong, Y. Chisti, and S. Sirisansaneeyakul. 2016. Production of theabrownins using a crude fungal enzyme concentrate. Journal of Biotechnology 231:250–9. doi: 10.1016/j.jbiotec.2016.06.010.
- Wang, P. Q., Y. J. Liu, L. J. Zhang, W. Z. Wang, H. Hou, Y. Zhao, X. L. Jiang, J. Yu, H. R. Tan, Y. S. Wang, et al. 2020. Functional demonstration of plant flavonoid carbocations proposed to be involved in the biosynthesis of proanthocyanidins. The Plant Journal: For Cell and Molecular Biology 101 (1):18–36. doi: 10.1111/tpj.14515.
- Wang, P. Q., G. L. Ma, L. J. Zhang, Y. Li, Z. P. Fu, X. Y. Kan, Y. H. Han, H. Y. Wang, X. L. Jiang, Y. J. Liu, et al. 2019a. A sucrose-induced MYB (SIMYB) transcription factor promoting proanthocyanidin accumulation in the tea plant (Camellia sinensis). Journal of Agricultural and Food Chemistry 67 (5):1418–28. doi: 10.1021/acs.jafc.8b06207.
- Wang, H. F., G. J. Provan, and K. Helliwell. 2000. Tea flavonoids: Their functions, utilisation and analysis. Trends in Food Science & Technology 11 (4–5):152–60. doi: 10.1016/S0924-2244(00)00061-3.
- Wang, P., J. Yu, S. Jin, S. Chen, C. Yue, W. Wang, S. Gao, H. Cao, Y. Zheng, M. Gu, et al. 2021. Genetic basis of high aroma and stress tolerance in the oolong tea cultivar genome. Horticulture Research 8 (1):1–15. doi: 10.1038/s41438-021-00542-x.
- Wang, W. N., L. Zhang, S. Wang, S. P. Shi, Y. Jiang, N. Li, and P. F. Tu. 2014. 8-C N-ethyl-2-pyrrolidinone substituted flavan-3-ols as the marker compounds of Chinese dark teas formed in the post-fermentation process provide significant antioxidative activity . Food Chemistry 152:539–45. doi: 10.1016/j.foodchem.2013.10.117.
- Weerawatanakorn, M., W. L. Hung, M. H. Pan, S. M. Li, D. X. Li, X. C. Wan, and C. T. Ho. 2015. Chemistry and health beneficial effects of oolong tea and theasinensins. Food Science and Human Wellness 4 (4):133–46. doi: 10.1016/j.fshw.2015.10.002.
- Wei, K., L. Y. Wang, Y. Z. Zhang, L. Ruan, H. L. Li, L. Y. Wu, L. Y. Xu, C. C. Zhang, X. G. Zhou, H. Cheng, et al. 2019. A coupled role for CsMYB75 and CSGSTF1 in anthocyanin hyperaccumulation in purple tea. The Plant Journal: For Cell and Molecular Biology 97 (5):825–40. doi: 10.1111/tpj.14161.
- Wei, C. L., H. Yang, S. B. Wang, J. Zhao, C. Liu, L. P. Gao, E. H. Xia, Y. Lu, Y. L. Tai, G. B. She, et al. 2018. Draft genome sequence of Camellia sinensis var. sinensis provides insights into the evolution of the tea genome and tea quality. Proceedings of the National Academy of Sciences of the United States of America 115 (18):E4151–E4158. doi: 10.1073/pnas.1719622115.
- Wen, L. R., Y. M. Jiang, J. L. Yang, Y. P. Zhao, M. M. Tian, and B. Yang. 2017. Structure, bioactivity, and synthesis of methylated flavonoids. Annals of the New York Academy of Sciences 1398 (1):120–9. doi: 10.1111/nyas.13350.
- Wen, X., and T. Walle. 2006a. Methylated flavonoids have greatly improved intestinal absorption and metabolic stability. Drug Metabolism and Disposition: The Biological Fate of Chemicals 34 (10):1786–92. doi: 10.1124/dmd.106.011122.
- Wen, X., and T. Walle. 2006b. Methylation protects dietary flavonoids from rapid hepatic metabolism. Xenobiotica; the Fate of Foreign Compounds in Biological Systems 36 (5):387–97. doi: 10.1080/00498250600630636.
- Wu, Z. H., S. M. Huang, T. T. Li, N. Li, D. D. Han, B. Zhang, Z. Z. Xu, S. Y. Zhang, J. M. Pang, S. L. Zhang, et al. 2021b. Gut microbiota from green tea polyphenol-dosed mice improves intestinal epithelial homeostasis and ameliorates experimental colitis. Microbiome 9 (1):184. doi: 10.1186/s40168-021-01115-9.
- Wulandari, R. A., M. Amano, T. Yanagita, T. Tanaka, I. Kouno, D. Kawamura, and K. Ishimaru. 2011. New phenolic compounds from Camellia sinensis L. leaves fermented with Aspergillus sp. Journal of Natural Medicines 65 (3–4):594–7. doi: 10.1007/s11418-011-0515-0.
- Wu, M., Q. Y. Luo, R. X. Nie, X. P. Yang, Z. Z. Tang, and H. Chen. 2021. Potential implications of polyphenols on aging considering oxidative stress, inflammation, autophagy, and gut microbiota. Critical Reviews in Food Science and Nutrition 61 (13):2175–93. doi: 10.1080/10408398.2020.1773390.
- Wu, W. L., T. Tong, Y. Hu, H. Zhou, X. Yin, and S. G. Zhang. 2021a. Camellia ptilophylla and specific chemical components, their health beneficial effects. Journal of Tea Science 41 (5):593–607. (In Chinese). doi: 10.3969/j.issn.1000-369X.2021.05.001.
- Xia, E. H., H. B. Zhang, J. Sheng, K. Li, Q. J. Zhang, C. Kim, Y. Zhang, Y. Liu, T. Zhu, W. Li, et al. 2017. The tea tree genome provides insights into tea flavor and independent evolution of caffeine biosynthesis. Molecular Plant 10 (6):866–77. doi: 10.1016/j.molp.2017.04.002.
- Xiao, J. B. 2017. Dietary flavonoid aglycones and their glycosides: Which show better biological significance? Critical Reviews in Food Science and Nutrition 57 (9):1874–905. doi: 10.1080/10408398.2015.1032400.
- Xie, D. C., W. D. Dai, M. L. Lu, J. F. Tan, Y. Zhang, M. Chen, and Z. Lin. 2019. Nontargeted metabolomics predicts the storage duration of white teas with 8-C N-ethyl-2-pyrrolidinone-substituted flavan-3-ols as marker compounds. Food Research International 125:108635. doi: 10.1016/j.foodres.2019.108635.
- Xing, L. J., H. Zhang, R. L. Qi, R. Tsao, and Y. Mine. 2019. Recent advances in the understanding of the health benefits and molecular mechanisms associated with green tea polyphenols. Journal of Agricultural and Food Chemistry 67 (4):1029–43. doi: 10.1021/acs.jafc.8b06146.
- Xu, B., H. Y. Jiang, J. J. Xue, Q. L. Liu, J. Y. Zhang, and Y. Wang. 2014. Forming mechanism of TSs and TFs in tea fresh leaf suspension fermentation. Chinese Agricultural Science Bulletin 30 (22):140–7. (In Chinese).
- Xu, S. S., J. J. Wang, Y. M. Wei, W. W. Deng, X. C. Wan, G. H. Bao, Z. W. Xie, T. J. Ling, and J. M. Ning. 2019. Metabolomics based on UHPLC-Orbitrap-MS and global natural product social molecular networking reveals effects of time scale and environment of storage on the metabolites and taste quality of raw pu-erh tea. Journal of Agricultural and Food Chemistry 67 (43):12084–93. doi: 10.1021/acs.jafc.9b05314.
- Ye, M., M. M. Liu, M. Erb, G. Glauser, J. Zhang, X. W. Li, and X. L. Sun. 2021. Indole primes defence signalling and increases herbivore resistance in tea plants. Plant, Cell & Environment 44 (4):1165–77. doi: 10.1111/pce.13897.
- Ye, Y. L., J. N. Yan, J. L. Cui, S. H. Mao, M. F. Li, X. L. Liao, and H. R. Tong. 2018. Dynamic changes in amino acids, catechins, caffeine and gallic acid in green tea during withering. Journal of Food Composition and Analysis 66:98–108. doi: 10.1016/j.jfca.2017.12.008.
- Zeng, X. Q., Y. Xi, and W. B. Jiang. 2019. Protective roles of flavonoids and flavonoid-rich plant extracts against urolithiasis: A review. Critical Reviews in Food Science and Nutrition 59 (13):2125–35. doi: 10.1080/10408398.2018.1439880.
- Zhang, L., C. Ho, J. Zhou, J. S. Santos, L. Armstrong, and D. Granato. 2019b. Chemistry and biological activities of processed Camellia sinensis teas: A comprehensive review. Comprehensive Reviews in Food Science and Food Safety 18 (5):1474–95. doi: 10.1111/1541-4337.12479.
- Zhang, J., Z. X. Liang, T. Zhang, Z. Q. Li, J. J. Zhu, P. C. Zhang, and W. J. Xiao. 2019a. Dynamic changes of main quality components during yellow tea processing. Food Science 40 (16):200–5. doi: 10.7506/spkx1002-6630-20181004-015.
- Zhang, Y. T., Q. Li, H. Xing, X. F. Lu, L. S. Zhao, K. K. Qu, and K. S. Bi. 2013. Evaluation of antioxidant activity of ten compounds in different tea samples by means of an on-line HPLC–DPPH assay. Food Research International 53 (2):847–56. doi: 10.1016/j.foodres.2013.03.026.
- Zhao, F., M. J. Chen, S. Jin, S. Y. Wang, W. J. Yue, L. X. Zhang, and N. X. Ye. 2022. Macro-composition quantification combined with metabolomics analysis uncovered key dynamic chemical changes of aging white tea. Food Chemistry 366:130593. doi: 10.1016/j.foodchem.2021.130593.
- Zhao, J., P. H. Li, T. Xia, and X. C. Wan. 2020. Exploring plant metabolic genomics: Chemical diversity, metabolic complexity in the biosynthesis and transport of specialized metabolites with the tea plant as a model. Critical Reviews in Biotechnology 40 (5):667–88. doi: 10.1080/07388551.2020.1752617.
- Zhao, X. C., X. S. Zeng, N. Lin, S. W. Yu, A. R. Fernie, and J. Zhao. 2021. CsbZIP1-CsMYB12 mediates the production of bitter-tasting flavonols in tea plants (Camellia sinensis) through a coordinated activator–repressor network. Horticulture Research 8 (1):1–18. doi: 10.1038/s41438-021-00545-8.
- Zheng, C., J. Q. Ma, C. L. Ma, S. Y. Shen, Y. F. Liu, and L. Chen. 2019. Regulation of growth and flavonoid formation of tea plants (Camellia sinensis) by Blue and Green Light. Journal of Agricultural and Food Chemistry 67 (8):2408–19. doi: 10.1021/acs.jafc.8b07050.
- Zheng, Y. Y., X. Zeng, T. T. Chen, W. Peng, and W. W. Su. 2020. Chemical profile, antioxidative, and gut microbiota modulatory properties of ganpu tea: A derivative of pu-erh tea. Nutrients 12 (1):224. doi: 10.3390/nu12010224.
- Zhou, J., Y. Wu, P. Long, C.-T. Ho, Y. Wang, Z. Kan, L. Cao, L. Zhang, and X. Wan. 2019. LC-MS-based metabolomics reveals the chemical changes of polyphenols during high-temperature roasting of large-leaf yellow tea. Journal of Agricultural and Food Chemistry 67 (19):5405–12. doi: 10.1021/acs.jafc.8b05062.
- Zhou, Z. H., Y. J. Zhang, M. Xu, and C. R. Yang. 2005. Puerins A and B, two new 8-C substituted flavan-3-ols from Pu-er tea . Journal of Agricultural and Food Chemistry 53 (22):8614–7. doi: 10.1021/jf051390h.
- Zhu, Y. F., J. J. Chen, X. M. Ji, X. Hu, T. J. Ling, Z. Z. Zhang, G. H. Bao, and X. C. Wan. 2015. Changes of major tea polyphenols and production of four new B-ring fission metabolites of catechins from post-fermented Jing-Wei Fu brick tea. Food Chemistry 170:110–7. doi: 10.1016/j.foodchem.2014.08.075.
- Zhu, X. J., J. R. Liao, X. L. Xia, F. Xiong, Y. Li, J. Z. Shen, B. Wen, Y. C. Ma, Y. H. Wang, and W. P. Fang. 2019. Physiological and iTRAQ-based proteomic analyses reveal the function of exogenous γ-aminobutyric acid (GABA) in improving tea plant (Camellia sinensis L.) tolerance at cold temperature. BMC Plant Biology 19 (1):43. doi: 10.1186/s12870-019-1646-9.
- Zhu, J. Y., Q. S. Xu, S. Q. Zhao, X. B. Xia, X. M. Yan, Y. L. An, X. Z. Mi, L. X. Guo, L. Samarina, and C. L. Wei. 2020. Comprehensive co-expression analysis provides novel insights into temporal variation of flavonoids in fresh leaves of the tea plant (Camellia sinensis). Plant Science: An International Journal of Experimental Plant Biology 290:110306. doi: 10.1016/j.plantsci.2019.110306.
- Zmora, N., G. Zilberman-Schapira, J. Suez, U. Mor, M. Dori-Bachash, S. Bashiardes, E. Kotler, M. Zur, D. Regev-Lehavi, R. B.-Z. Brik, et al. 2018. Personalized gut mucosal colonization resistance to empiric probiotics is associated with unique host and microbiome features. Cell 174 (6):1388–405. doi: 10.1016/j.cell.2018.08.041.