Abstract
Fermented soybean products, including Soya sauce, Tempeh, Miso, and Natto have been consumed for decades, mainly in Asian countries. Beans are processed using either solid-state fermentation, submerged fermentation, or a sequential of both methods. Traditional ways are still used to conduct the fermentation processes, which, depending on the fermented products, might take a few days or even years to complete. Diverse microorganisms were detected during fermentation in various processes with Bacillus species or filamentous fungi being the two main dominant functional groups. Microbial activities were essential to increase the bean’s digestibility, nutritional value, and sensory quality, as well as lower its antinutritive factors. The scientific understanding of fermentation microbial communities, their enzymes, and their metabolic activities, however, still requires further development. The use of a starter culture is crucial, to control the fermentation process and ensure product consistency. A broad understanding of the spontaneous fermentation ecology, biochemistry, and the current starter culture technology is essential to facilitate further improvement and meet the needs of the current extending and sustainable economy. This review covers what is currently known about these aspects and reveals the limited available information, along with the possible directions for future starter culture design in soybean fermentation.
Introduction
The main food plants for humans include soybeans, wheat, maize, rice, sorghum, potato, oats, cassava, and sugar beets. Among them, soybeans (Glycine max (L.) Merr.) are characterized by their high protein and oil concentrations (Hymowitz Citation2008). The production of soybeans worldwide increased from 160 to 350 million tons in 1998 and 2018, respectively, due to advances in genetically modified technology such as climate and herbicide-resistant crops (FAOStat Citation2021; Salvagiotti et al. Citation2008). In the west, soybeans are mostly processed into two products: soybean meal for the feed sector and soybean seed oil for salad dressings, margarine, and mayonnaise. In Asia, several traditional foods are made from soybeans, such as Soy sauce, Tofu, Miso, Tempeh, and Natto (Hymowitz Citation2008; Xu, Guo, and Zhang Citation2019).
Soybeans were first known in China, where several fermented soy products were created. They spread to the nearby nations, where other process improvements were developed. These fermented soybean products are considered a valuable source of protein and are widely consumed for their high value in nutrition, functionality, and low cost. Soybean seeds contain about 36.5% protein, 30% carbohydrate, 19.9% lipids, and 9.3% fiber (Vagadia, Vanga, and Raghavan Citation2017). Soybean oil contains 57.7% polyunsaturated fatty acids, 22.8% monosaturated fatty acids, and 15.6% saturated fatty acids. Soybean protein contains high levels of lysine, leucine, valine, isoleucine, phenylalanine, and threonine but lacks tryptophan, cysteine, and methionine (Wolf Citation1970). However, due to their off-flavor and the presence of anti-nutritional factors (ANFs) such as phytic acid, oligosaccharides, and trypsin inhibitors, there is still limited adoption of soybeans as a major protein source in the diet (Rehms and Barz Citation1995; Yang et al. Citation2018).
The soybean fermentation process, as an example of food fermentation, was originally considered a preservation technique but has now evolved into a way to create traditional nutritive food products with distinctive flavors, aromas, and textures (Bessada, Barreira, and Oliveira Citation2019). Soybean fermentation is generally performed either in solid-state or submerged modes or a combination of both processes (Abass et al. Citation2017; M. Adams and Mitchell Citation2002; Garrido-Galand et al. Citation2021; He and Chung Citation2020; Saharan, Sadh, and Duhan Citation2017; Sarkar and Nout Citation2014; Thirunathan and Manickavasagan Citation2019). Traditionally, the fermentation of soybeans occurs spontaneously, with a variety of microorganisms originating from the seed surface or the surrounding environment. Elucidation of the roles of indigenous microorganisms enabled the rational control of the fermentation process through its application in starter culture technology (Geisen Citation1993; Liu, Chen, et al. Citation2021; Liu, Chen, et al. Citation2022; McCue et al. Citation2004; Park and Kim Citation2020; Shin and Jeong Citation2015; Singracha et al. Citation2017; Wang, Zhang, et al. Citation2021). These will be covered by this review, focusing mainly on the analysis of the soybean fermentation natural microflora, its contribution to final product quality, and the potential transformation process into the starter culture technology.
Physical and chemical factors limiting the consumption of soybeans
The primary component of a soybean is a dicotyledon seed enclosed by a roughly spherical shell or hull, the form of which varies greatly depending on cultivar and growth conditions (Perkins and D. R. B. T.-P. H. of S. P Citation1995). Despite the high nutritive value of soybeans, they contain anti-nutritive components that limit their consumption, including trypsin inhibitors, lipoxygenase, phytic acid, and oligosaccharides, . Studies, for instance, have linked the presence of trypsin inhibitors, a class of protease enzymes that block the function of proteolytic enzymes within the gastrointestinal system, to the poor nutritional value of soybeans (Embaby Citation2010). Several physical, chemical, biological and advanced techniques such as ohmic heating, microwave, ultrasound, high-pressure processing, radiofrequency, infrared, and gamma irradiation heating have been used to eliminate them or lower their amount. Although heat and chemical treatments are efficient and affordable inactivation methods, they also damage important nutrients like lysine and heat-labile vitamins (Huang, Kwok, and Liang Citation2004; Kaur et al. Citation2012; Kunitz Citation1947; Rouhana et al. Citation1996; Sessa, Haney, and Nelsen Citation1990; Yuan and Chang Citation2010). During seed germination, the endogenous proteases, which were shown to be more active at 35 °C, were primarily responsible for the decline in trypsin inhibitor levels (Avilés-Gaxiola, Chuck-Hernández, and Serna Saldívar Citation2018; V. Kumar et al. Citation2006; Nkhata et al. Citation2018). Trypsin inhibitors are also degraded by microbial isolates such as Rhizopus oligosporus, Actinomucor elegans, Lactiplantibacillus plantarum, and Bacillus subtilis during soybean fermentation (Adeyemo and Onilude Citation2013; Amadou et al. Citation2010; Dai et al. Citation2017; Huang et al. Citation2019).
Figure 1. Soybean consumption constraints and possible ways for their reduction/elimination. Photos of soybeans seeds dehull seeds and dicotyledon structures are included. This figure was created using BioRender (https://biorender.com/).
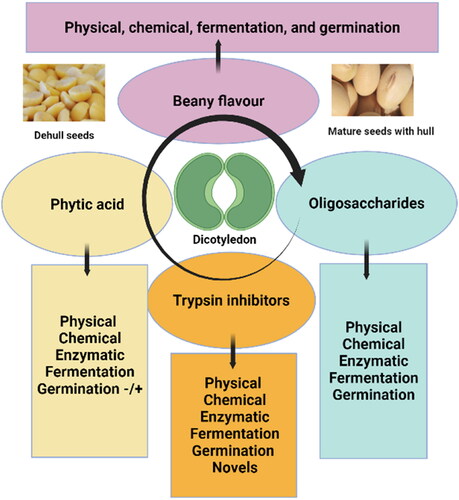
Similarly, several studies have highlighted the impact of other anti-nutritive components such as lipoxygenases, phytic acid, oligosaccharides, saponin, allergens, urease, lectin, and alkaloids (Aulitto et al. Citation2021; Dong and Saneoka Citation2020; Humer, Schwarz, and Schedle Citation2015; Karkle, Elisa Noemberg Lazzari and Beleia, Citation2010; Kumar et al. Citation2020; Shahidi and Oh Citation2020; Vagadia, Vanga, and Raghavan Citation2017; Wang, Zhang, et al. Citation2021; Wang et al. Citation1997). Various methods were applied to eliminate or minimize these factors including physical, chemical, and biological approaches (Chitra, Singh, and Rao Citation1996; Gao et al. Citation2013; Hu et al. Citation2019; Li et al. Citation2020; Shahidi and Oh Citation2020; Vagadia, Vanga, and Raghavan Citation2017; Villacrés et al. Citation2020). More information can be found elsewhere (Norozi, Rezaei, and Kazemifard Citation2022; Qin, Wang, and Luo Citation2022; Suprayogi et al. Citation2022). Overall, fermentation was able to degrade them while preserving the bioavailability of valuable and nutritive compounds, illustrating its potential application (Nkhata et al. Citation2018; Shirai et al. Citation1994).
Microbial ecology of traditional soybean fermentation
Each country produces its own artisan soybean products, using different soybean fermentation procedures. Several factors influence the distinct characteristics of flavor and texture, including the type and length of fermentation processes, salt concentration, and moisture content, as well as additional components (Devanthi and Gkatzionis Citation2019). The manufacturing-specific details, different ingredients, and names used in different countries are out of this review scope and can be found elsewhere (Erickson Citation2015; Garrido-Galand et al. Citation2021; Liu, Chen, et al. Citation2022; Luh Citation1995; Sarkar and Nout Citation2014). By understanding the microbial identities and their roles in different types of soybean fermentation, one can better control the fermentation processes and modulate the final product quality. Soybeans are generally subjected to either solid-state fermentation, submerged fermentation, or both. The microbial groups that lead the fermentation are mainly bacterial, fungal, or a mixture. shows the overall soybean process flow chart, the fermentation types, and the major microbial species that drive the processes. The basic concepts of these fermentation procedures are described in the following sections.
Figure 2. Process flow diagram of soybeans. This figure was created using BioRender (https://biorender.com/).
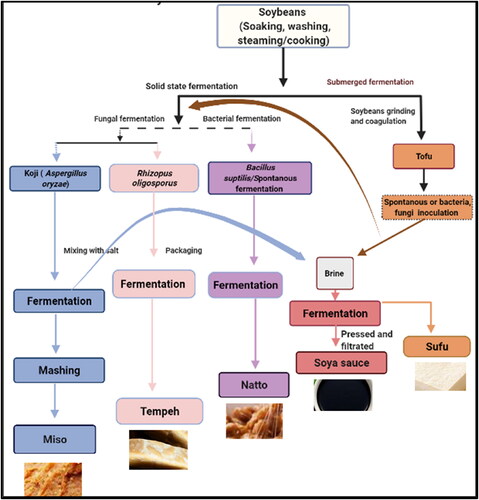
Soybean surface ecology
Limited knowledge was reported about the microbial endogenous community detected from the surface of the soybean seeds. An early report showed several fungal isolates including Fusarium spp. (including F. moniliforme, F. acuminatum, F. equiseti), Alternaria alternata and Diaporthe phaseolorum var. sojae were detected from the soybean surface (Miller and Roy Citation1982). For bacteria, the main predominant microorganisms were Pseudomonas whereas others belonging to Enterobacteriaceae and Proteobacteria were reported in low populations (Kuklinsky‐Sobral et al. Citation2004; Miller and Roy Citation1982; Wang, Zhang, et al. Citation2021). A study conducted by Mulyowidarso (Citation1991) showed that bean surface colonization contained between 2–5 log CFU/g of the total microbial count. Several bacteria have been reported in relevant research, such as Lactobacillus spp., Streptococcus facecium, Staphylococcus epidermidis, Klebsiella ozaenae, Citrobacter diversus, and Enterobacter cloacae which were predominant, as well as yeasts such as Pichia burtonii, Candida diddensiae and Rhodotorula rubra and mold species belonging to genera Trichosporon, Aspergillus and Rhizopus which were present in lower populations (Mulyowidarso Citation1991; Wei, Regenstein, and Zhou Citation2021). The microorganisms associated with the seeds play potentially crucial roles in the fermented product quality (Parkouda et al. Citation2009). The surrounding environment such as the soil, water, air, workers, and fermentation equipment may also introduce several microorganisms that are detected as the fermentation progresses, such as Escherichia coli, Salmonella, Staphylococcus, Proteobacteria, Fusarium, Geotrichum, Monascus, Aspergillus, and Mucor species (Natarajan et al. Citation2016; Xie et al. Citation2019). Further investigation is required to better understand the ecology of the soybean surface and the type of microbiota present in order to manage the subsequent processes. Knowledge about the soybean surface microbiota, mainly heat-resistant thermophilic spores, is essential to optimize the sterilizing or cooking steps that are necessary to eliminate or reduce the initial contamination and to not compete with the desired microorganisms and produce unpredictable fermented products. Furthermore, although starter cultures often have the ability to lead the fermentation process, the diversity and contamination of the survival indigenous population may make them more competitive than the inoculum strains and may therefore reduce the starter culture’s ability to dominate the fermentation to 100%.
Microbiology of the soaking process
Soybeans either heat-treated or untreated are mostly subjected to a soaking step before fermentation. Natural acid fermentation develops during non-heat-treated beans causing an increase in acidity to a final pH of 4.5. Several isolates were detected during the soaking process belonging to LAB, yeasts, Enterobacteriaceae, and Bacillus species (Chauhan et al. Citation2022; Mulyowidarso Citation1991; Nurdini, Nuraida, and Suwanto Citation2015). Lactcaseibacillus casei, Lactiplantibacillus plantarum, Leconostoc mesenteriodes, Pedicoccus sp., and Enterococcus faecium were the predominant LAB species during the whole soaking process. Enterobacteriaceae such as C. diversus and Klebsiella pneumoniae were also detected during the early stage of the soaking process and declined at the end. Yeasts such as P. burtonii, C. diddensiae, and R. rubra, Saccharomyces dairesnsis were also detected with a weak growth rate at the initial of the soaking process. Studies have shown that the ecology of soybeans is the main origin of the leading microorganisms of acid fermentation and not the tap water. In addition, it is believed that the microbial metabolites produced during this fermentation diffuse into the beans and affect their chemical profiles (Chauhan et al. Citation2022; Nurdini, Nuraida, and Suwanto Citation2015). This soybean acidification may control microbial pathogens and undesirable microbial growth, ensuring the safety and sensory quality of the final product (Chauhan et al. Citation2022; Nurdini, Nuraida, and Suwanto Citation2015). Weak acidification was linked to high Bacillus cereus levels in the final soybean product after heat treatment (Nout, Beernink, et al. Citation1987; Nout, De Dreu, et al. Citation1987). Endogenous species such as Citrobacter freundii, Pseudomonas fluorescens, K. pneumoniae, and Streptococcus sp. were found to produce high levels of vitamin B12 (Denter and Bisping Citation1994). Furthermore, the soaking process was reported to reduce the level of water-soluble anti-nutritive factors such as phytic acids, but also reduce other water-soluble important components such as vitamins, minerals, and phytochemicals (Garrido-Galand et al. Citation2021; Reddy and Pierson Citation1994; Rehman et al. Citation2014). Heat treatment of soybean before soaking was reported to eliminate most of the LAB, yeasts, and Enterobacteriaceae species, leaving behind heat resistance spore-forming bacteria such as Bacillus brevis causing acidification failure (Wickware Citation2015). This promoted undesirable microbial contaminant growth during the fermentation process and adding acetic and lactic acids into soaking water was recommended as a countermeasure (Nout, Beernink, et al. Citation1987; Steinkraus et al. Citation1965; Tunçel and Göktan Citation1990). To the best of the authors’ knowledge, limited information is available on the acidic fermentation changes that may occur in the beans during the soaking, as well as possible strategies (i.e., a biological strategy of using LAB or yeasts) that may control this process without interfering with the subsequent processes, as will be discussed later under Bacillus fermentation.
Microbiology of soybean fermentation
After preparation, soybeans are subjected to either solid-state, submerged fermentation processes, or a sequential of both processes. Solid-state fermentation is an old method of subjecting moist solid food particles to fermentation using bacteria, yeast, or filamentous fungi to produce fermented food or metabolites such as enzymes, flavors, acids, etc. Whole soybeans or their residues are subjected to bacterial or fungal solid-state fermentation to produce various fermented products including Natto, Tempeh, and the first stage of Miso, Soya sauce, and Sufu (Pandey Citation2003). Each product has its characteristic flavor and texture because of the unique microbial populations found in the raw materials that dominate the fermentation. The submerged fermentation is a process conducted in the presence of free excess water (Singhania Citation2011). Two main soybean-fermented products are produced through a submerged fermentation stage, as part of the process in brine: Soya sauce and Sufu.
Natto
Natto is produced by bacterial fermentation and characterized by a sweet, umami, and slightly bitter taste with the texture of a viscous polymer (Kanno and Takamatsu Citation1987). Traditionally, it was produced by wrapping boiled soybeans in rice straw pretreated with boiling water to kill vegetative microorganisms, leaving behind spore-forming Bacillus including B. subtilis subsp. subtilis (natto), and incubating it for about 3 days (Sarkar and Nout Citation2014). Rice straw is also a natural habitat of other spore-forming Bacillus species that are believed to have a crucial impact on the fermented product, such as B. amyloliquefaciens B. pumilus, Bacillus licheniformis, and Bacillus atrophaeus (Kubo et al. Citation2011; Meerak et al. Citation2008). Bacillus spores are known for their resistance to environmental stress, and their vegetative cells have high enzymatic activities to degrade protein, carbohydrates, and lipids (Ouoba et al. Citation2003). During fermentation, fructan and polypeptides such as glutamic acid are produced which cover Natto with a stringy mucous material of unique flavor and aroma (Reddy, Pierson, and Salunkhe Citation2009). Furthermore, microbial lipolytic activities in soybeans cause the production of fatty acids such as oleic, linoleic, and linolenic acids with a significant impact on the final product’s sensory quality (Hu et al. Citation2010; Sharma et al. Citation2020). The endogenous Bacillus species were reported to biosynthesize a wide range of alkyl pyrazines such as methyl pyrazine, dimethyl pyrazine, trimethyl pyrazine, and tetramethyl pyrazine with known pleasant aroma (Kłosowski, Mikulski, and Pielech-Przybylska Citation2021). They also produce inhibitory metabolites which suppress the growth of foodborne pathogens such as Escherichia coli, Bacillus cereus, Staphylococcus aureus, and Salmonella typhimurium and mycotoxin-producing fungi (Guo et al. Citation2006; Kaboré et al. Citation2013; N’Dir et al. Citation1994). Kinema is a subtype of Natto that is also produced by spontaneous Bacillus fermentation. It has a sticky texture and ammoniacal odor (Sharma et al. Citation2015). B. subtilis was the predominant microbial isolate found during the Kinema fermentation and was believed to be attached to the bean surface. Besides, yeasts such as Candida parapsilosis and Geotrichum candidum, as well as bacteria, mainly Enterococcus fuecium, were detected as originating from wooden mortar and pestle that were used to prepare soybeans (Sarkar et al. Citation1994; Tamang Citation2003). The isolated Bacillus showed higher enzymatic activities including peptidase, phosphatase, lipase, and esterase compared with Enterococcus and the detected yeasts made the authors link the physicochemical changes that occurred in the fermented beans to Bacillus strains (Tamang Citation2003). Premature arrest of alkaline fermentation (stuck fermentation) might be one of the challenging problems in soybean fermentation. The presence of organic acids, either naturally occurring during the soaking period from LAB fermentation or artificially introduced as previously described, may prevent this Bacillus fermentation (Hosoi and Kiuchi Citation2008). To remove the organic acids and eliminate their negative impact on Natto production, flowing tap water is often applied after soaking. However, excessive flowing may affect the taste of Natto (Hosoi and Kiuchi Citation2008). The mechanisms of such effects are largely unexplored and require further research, maybe by either optimizing the manufacturing process or selecting acid-tolerant Bacillus isolates to conduct the fermentation.
Tempeh
In Tempeh, the most common molds found are Rhizopus oryzae, R. oligosporus, and Aspergillus oryzae, with a lower population of other species such as T. pullulans, A. niger, A. alternata, Mucor javanicus, and Cladosporium oxysporiumand (Ahnan‐Winarno et al. Citation2021; Romulo and Surya Citation2021). Bacteria and yeasts were also isolated in Tempeh with a population ranging from 6 to 9 logs CFU/g. For bacteria, high populations of B. licheniformis, Lactobacillus delbreuckii, and Limosilactobacillus mucosae, and low populations of Enterobacteriaceae species such as K. pneumoniae, C. freundii, and E. cloacae were detected. Yeasts including species of Trichosporon, Candida, Rhodotorula, Pichia, and Yarrowia were also reported in low populations (Kustyawati Citation2017; Kustyawati et al. Citation2018; Nurdini, Nuraida, and Suwanto Citation2015; Rizal et al. Citation2021; Romulo and Surya Citation2021; Suwanto Citation2021). During Tempeh fermentation, the fungal mycelium is attached to the soybean surface, and studies report varying penetration capability from only a few cell layers in depth to penetrating the bean cell wall and growing inside (Mulyowidarso Citation1991; Paredes-Lopez et al., Citation1987; Sudarmadji and Markakis Citation1978). The growth of these endogenous microorganisms brings about chemical changes within the fermented beans. Several enzymes were detected during tempeh fermentation, including lipases, proteases, cellulases, pectinases, and amylases (Ruiz‐Terán and David Owens Citation1996). Such enzymes were reported to degrade the crude lipid, triglycerides, proteins, and carbohydrates, producing free ammonia, free amino acids, polypeptides, free fatty acids, reducing sugars, and gradually raising the pH (Handoyo and Morita Citation2006; Mulyowidarso Citation1991; Sarkar and Nout Citation2014). Various compounds were detected in fermented beans that have desirable flavor and aroma such as pyridine, diacetyl, butanediol, and other higher alcohols and organic acids (Mulyowidarso Citation1991). Tempeh fermentation was also reported to decrease the level of trypsin inhibitors and phytic acid and increase the bean digestibility and the levels of riboflavin, and niacin concentration (Owens, Astuti, and Kuswanto Citation2015; Romulo and Surya Citation2021). These physiochemical changes occurred with the soybeans converting unpalatable beans into more palatable ones with distinct clean and characteristic aromas. Besides the reported roles of filamentous fungi, knowledge about the other microbial groups including bacteria and yeasts might be essential. These groups were frequently reported during the fermentation process with various populations and their roles were scarily studied. For example, Tempeh was associated with a variety of sensory characteristics, including bitterness, that was believed to be linked to bacterial and yeast activities rather than mold growth, illustrating their essential impact (Barus et al. Citation2008; Suwanto Citation2021). Future research employing advanced technologies like metagenomic, and proteomic analysis may deepen our understanding of microbial populations, including the non-mold species, to better control Tempeh fermentation and its sensory quality.
Soya sauce
Soya sauce is produced using a traditional fermentation process by mold spores (Koji), under a non-sterile environment which leads to the growth of indigenous microorganisms. Koji is produced by subculturing A. oryzae or Aspergillus sojae on cereals including steamed rice, wheat bran, or soybean flour, and incubated for 3 days in a tray or small boxes in a warm room (25–35 °C), with good aeration and low moisture to prevent the growth of undesirable microbial growth. The mature koji culture has a yellow to yellowish-green color coating the surface of the whole mass, which contains several enzymes including proteases, α-amylases, cellulases, and lipases. Leucine aminopeptidases and two endopeptidases (alkaline protease and neutral) were found with A. oryzae during this koji solid-state fermentation, whereas acid carboxypeptidases were also found with A. sojae (Ito and Matsuyama Citation2021; Kusumoto et al. Citation2021; Yamashita Citation2021). These enzymes were reported to hydrolyze the soybeans protein into polypeptides, peptides, and amino acids (Kusumoto et al. Citation2021; Yamashita Citation2021). Similarly, starch and cellulose are enzymatically broken down into disaccharides and monosaccharides by the extracellular α-amylase and cellulase respectively. Soybean lipid breakdown was not reported at this stage of fermentation and mainly occurred in the subsequent brine fermentation process (Nout and Aidoo Citation2011). Solid-state fermentation supports fungal growth and optimal enzyme production that would not be possible during submerged fermentation (Chancharoonpong, Hsieh, and Sheu Citation2012). By ensuring fungal growth, the growth of undesirable microorganisms is prevented (Adams and Moss Citation2008) and enzyme degradation processes can take place to provide substrates for microorganisms in the subsequent fermentation (Zhu, Citation2013). The resulting mass is submerged in brine (moromi) to produce Soya sauce (Allwood, Wakeling, and Bean Citation2021).
During the moromi submerged fermentation, lactic acid and yeast fermentation take place spontaneously for about one year, giving the final product its distinct sensory quality (Yong and Wood Citation1974). An early study showed that lactic acid fermentation occurs in the early stage, followed by yeast alcoholic fermentation (Devanthi and Gkatzionis Citation2019), while fungal growth was mostly inhibited by the high sodium chloride concentration in brine. Salt-tolerant yeasts were detected and identified as Pichia guilliermondii, Z. rouxii, Candida glabrata, and Pyrococcus furinosa (Song, Jeong, and Baik Citation2015). Characteristic yeast flavor metabolites were detected during the fermentation including alkyl phenols such as 2-phenyl ethanol, 4-ethylphenol, and 4-ethyl guaiacol, and high levels of glycerol (Song, Jeong, and Baik Citation2015). For bacteria, homofermentative, salt-tolerant, tetrad-forming cocci isolate known as Tetracoccus soyae and Pediococcus acidihctici var. soya have been identified, as well as low levels of Lactobacillus sp. (Lockwood and Smith Citation1950; Yong and Wood Citation1974). In addition, Bacillus species including B. amyloliquefaciens, B. licheniformis, B. pumilus, and B. subtilis were also detected during brine fermentation (Devanthi and Gkatzionis Citation2019; Matsumoto and Tomoyasu Citation1925; Yang et al. Citation2017; Yong and Wood Citation1974). More recently, species such as W. paramesenteroides, P. acidilactici, Pediococcus pentosaceus, T. halophilus, and B. amyloliquefaciens were detected at high abundance during the fermentation process (Sun, Li, and Sun Citation2009). Lactic fermentation is essential to improve the stability of brine fermentation, preventing undesirable flavors and adding desirable metabolites, mainly organic acids, improving the Soy sauce sensory quality (Harada et al. Citation2017, Citation2018; Sun, Li, and Sun Citation2009). Continuing the acidification process might cause a decline in the neutral and alkaline proteinase activities, leading to a reduction of protein solubility and elongating the process completion (Hoang et al. Citation2016). For non-LAB species, Bacillus has been reported, with high antifungal and high enzymatic activities - protease, fibrinolytic, and α-amylase which are believed to contribute to the Soya sauce flavor profile and improve its stability (Baek et al. Citation2011; Cho et al. Citation2009; Sun, Li, and Sun Citation2009; Yoo et al. Citation1999). These findings showed the ecological complexity of the two fermentation steps, koji, and moromi. Despite the fact that during mormori maturation the majority of soy sauce flavors are created, this process has received little research attention. To the best of our knowledge, there is still much to learn about the mechanism of flavor formation and how the microorganisms present in moromi fermentation function. Such knowledge is necessary to control the fermentation process, which could speed up the brine maturation process and enhance the quality of the finished product.
Miso
A consortium of both fungi and bacteria can also produce artisan fermented soybean products, which is the case of Miso solid-state fermentation. The soybeans are subjected to fungal fermentation for 48 h, then mixed with other ingredients including salt, and left to ferment for up to 24 months (Allwood, Wakeling, and Bean Citation2021; Onda, Yanagida, Uchimura, et al. Citation2003). Traditionally, mashed cooked beans were shaped into balls, and wrapped in rice straw which was modified later using rice koji that was used in Soya sauce production (Kusumoto and Rai Citation2017). Many varieties of fungal isolates were detected during Miso fermentation. However the leading molds were mainly A. oryzae, A. sojae, besides, A. tamarii, A. awamori, A. saitoi, and A. karwachi (Ashu et al. Citation2016; Sanjukta and Rai Citation2016). The enzymes produced during fungal fermentation include alkaline, acid, and neutral proteases, lipases, amylase, glutaminase, acid phosphatase, metallopeptidase, and phytase (Sourabh et al. Citation2015). These enzymes were linked to the hydrolysis of protein, lipid, starch, glutamine, phytic acid, and formation of free fatty acids, reducing sugars, as well as glutamic acid, that provide Miso with a characteristic umami flavor (Allwood, Wakeling, and Bean Citation2021). The addition of salt selects halophilic LAB species such as Tetragenococcus halophilus which will predominate the fermentation and the subsequent ripening processes (Allwood, Wakeling, and Bean Citation2021; Onda, Yanagida, Uchimura, et al. Citation2003). These bacteria were reported to produce organic acids causing color brightening, enhanced product stability, and improved yeast growth. In contrast, other LAB species including Pediococcus acidilactici, L. plantarum, and Fructilactobacillus fructivorans were also detected and believed to have a negative impact on the product quality, namely over-acidification, swelling, and inhibition of other desirable microbial growth including that of yeasts and filamentous fungi (Allwood, Wakeling, and Bean Citation2021; Ohnishi Citation1994; Onda, Yanagida, Tsuji, et al. Citation2003; Onda, Yanagida, Uchimura, et al. Citation2003). Yeasts such as Wickerhamomyces anomalus, Zygosaccharomyces rouxii, Debaryomyces nepalensis, Pichia membranifaciens, and Candida prapsilosis were previously detected in Miso (Allwood, Wakeling, and Bean Citation2021; Kusumoto et al. Citation2021). These yeasts produce desirable metabolites including ethyl alcohol, higher alcohols, and succinic acid linked to the desirable aroma of Miso, while other yeasts and bacteria may produce a thick film coating the product surface and affecting its odor. These findings suggest that the typical Miso is produced by the metabolic activities of various microbial activities. It is challenging to identify the usual Miso microbial profile and identify which microorganisms are responsible for the related texture and flavor changes because of the presence of a variety of microbial groups. Comparative research employing specific inhibitors might shed more light on the microorganisms that make up a typical Miso. For example, the fermentation processes of coffee and cocoa revealed a rich microbial ecology of several fungi and bacteria species (De Vuyst and Weckx Citation2016; Elhalis, Cox, and Zhao Citation2023; Evangelista et al. Citation2014). Understanding the significance of each microbial group and its functions required a comparative study. The authors used selective agents such as Natamycin and Nisin to selectively suppress the fungal and bacteria, respectively, and compared the resulting beans to a spontaneous fermentation process (Elhalis, Cox, Frank, et al. Citation2020; Ho, Zhao, and Fleet Citation2014; Ho, Fleet, and Zhao Citation2018). More specifically, to illustrate the role of yeasts in coffee and cocoa fermentation, researchers suppressed yeast growth with Natamycin and then compared the resulting fermenting beans in the absence of endogenous yeasts to a spontaneous fermentation where yeasts were presented. The authors concluded that yeasts are essential to produce high-quality beans and are considered a typical microflora during both fermentation processes (Elhalis, Cox, Frank, et al. Citation2020; Ho, Zhao, and Fleet Citation2014). In contrast, a related study that used Nisin and lysozyme to restrict LAB growth during cocoa fermentation showed the unnecessary involvement of this microbial group to produce high-quality beans (Ho, Zhao, and Fleet Citation2015). Similar approaches might be followed to advance our understanding of the impact of different microbial groups on producing high-quality Miso.
Sufu
Sufu is produced from soymilk curd which is subjected firstly to fungal solid-state fermentation (soymilk curd overgrown with a mold known as pehtze). A study conducted by Han et al. (Citation2004) to monitor the endogenous microflora during this solid-state fermentation reported a wide range of microorganisms including LAB, spore-forming bacteria, and yeasts, in addition to filamentous fungi. Aerobic bacteria and spore-forming bacteria had an initial presence of about 4 log CFU/g which grew to a maximum population of 8–9 log CFU/g at the end. LAB presented with a similar initial count and grew steadily during the solid-state fermentation to reach a maximum count of log 7 CFU/g. Fungi had an initial count of less than 3 log CFU/g and increased to about 7 log CFU/g by the end. The inclusion of a salting process reported a significant reduction of the total population of aerobic bacteria, LAB, fungi, and a lower effect on the level of spore-forming Bacillus, similar to that reported with Miso as discussed above. The typical mold fermentation of Sufu is predominated by A. elegans, Mucor wutunkiao, Mucor racemosus, Rhizopus microsporus, R. oryzae, A. oryzae, G. candidum, and Trichosporon asahii (Moy, Lu, and Chou Citation2012; Wan et al. Citation2020; Xie et al. Citation2018). Several enzymatic activities including protease, α-amylase, lipase, α-galactosidase, esterase, and l-glutaminase were detected during the solid-state fermentation and associated with significant physiochemical changes in the fermented curd. These enzymes were reported to degrade the raw materials through a series of biochemical processes producing various fatty acids, amino acids, alcohols, organic acids, aldehydes, and esters, that impart a desirable impact on the product aroma and texture quality (He, Chen, and Chung Citation2022; Liu, Song, and Luo Citation2018; Xu et al. Citation2020). For example, Liu, Chen, et al. (Citation2022) detected basic proteases, neutral proteases, and amino acid decarboxylase during Sufu fermentation which was believed to increase the levels of free amino acids, peptides, and free ammonia. Similarly, different molecular mass proteases (73.1, 76.4, and 109.1 kDa) were detected during Sufu fermentation and were reported to degrade the curd protein into ammonia, amines, amino acids, and peptides (Han et al. Citation2003). The authors believed such enzymes belong to different microbes but not molds, i.e., A. elegans, which was commonly reported with proteases with molecular mass ranging from 30–40 kDa. By the end of the fermentation, high levels of long-chain fatty acid ethyl esters such as ethyl dodecanoate, ethyl octadecenoate, and ethyl octadecadienoate were detected. These are likely formed by the reaction between metabolic products such as ethanol and acids by fungal lipases. Similarly, sulfur-containing compounds such as methional increased with the extended maturation which might be formed by the indigenous microbial populations, amino acids enzymatic degradation, or Maillard reaction (He, Chen, and Chung Citation2022; Moy, Lu, and Chou Citation2012; Ouoba et al. Citation2003).
The mold-fermented soymilk curd is subjected to submerged fermentation in different dressing mixtures with various salt concentrations. The microbial diversity was potentially affected by the climate, locations, and raw materials. During fermentation, the level of dominant endogenous aerobic and spore-forming bacteria present at the earlier stage of solid-state fermentation declines, and significant reduction was observed with higher salt content dressings (Han, Rombouts, and Nout Citation2001, Han et al. Citation2004). The dominant genera found were Leuconostoc citreum, Weissella paramesenteroides, Lactococcus lactis, E. faecium, B. anthracis, B. cereus, and others belong to genera of Lactobacillus, Tetragenococcus, Acinetobacter, Pseudomonas, and Chryseobacterium (Fei et al. Citation2018; Wan et al. Citation2020; Zhen-Dong et al. Citation2021). The fungal communities that were presented at the early stage declined as the fermentation progressed. Yeasts such as Pichia cactophila, Kodamaea ohmerivoucher, Magnusiomyces capitatus, and Candida etchellsii were also detected initially and survived during the fermentation (He and Chung Citation2020; Wan et al. Citation2020; Wu et al. Citation2018). The endogenous species such as Tetragenococcus, Lactobacillus, Candida, Debaryomyces, and Geotrichum, Actinomucor were correlated to various desirable compounds such as free amino acids, fatty acids, esters, higher alcohols, and aldehydes that are known for their fruity, sweet, and nutty aroma, enhancing the final product quality (He and Chung Citation2020; Wan et al. Citation2020; Wang, Sun, et al. Citation2019). Most recently, Candida tropicalis and Rhodotorula sp. were reported to produce nonanoic acid that added milk flavor notes to the final products, most likely through their lipolytic activities (Kim et al. Citation2019; Li et al. Citation2022). On the contrary, endogenous isolates such as Bacillus, Weissella, and Alternaria were believed to negatively contribute to the final product quality (He and Chung Citation2020). Red Sufu is a variant of Sufu prepared using comparable methods, but the final maturation period is dominated by Monascus fermentation (Wang, Sun, et al. Citation2019). Monascus species including M. purpureus, M. anka, and M. ruber have been used to ferment substrates, mainly rice, and produce various secondary metabolites including pigments such as Ankaflavin and Monascin (yellow); Monascorubrin and Rubropunctatin (orange); Monascorubramine and Rubropunctamine (red) (Srianta et al. Citation2016). During red Sufu maturation, various aroma compounds were produced and esters and alcohols were abundant volatiles (Chung Citation2000; Wang, Sun, et al. Citation2019). Esters such as ethyl acetate, ethyl 2-methyl butanoate, ethyl 2-methyl propanoate, ethyl butanoate, and ethyl 3-phenylpropionate, are associated with adding fruity notes to the final products. The presence of these esters compounds was linked to yeast metabolic activities of lipids which produce free fatty acids that result in the synthesis of corresponding esters (Gao et al. Citation2010; Wang, Sun, et al. Citation2019). Alcohols, such as ethanol, phenylethyl alcohol, 1-octen-3-ol, 2-methylbutanol, hexanol, and 3-methylbutanol give flowery and sweet aroma to the final products (Devanthi et al. Citation2018; Zhang et al. Citation2019). Besides, other components including 2,3-butanedione, 3-(methylthio) propanal, and benzene acetaldehyde, were detected and believed to enhance the red Sufu characteristic aroma profile (Gao et al. Citation2010; Wang, Sun, et al. Citation2019). Overall, identifying the crucial flavor-producing microbial isolates among the endogenous microbiota of Sufu fermentation is still challenging because of the microbial community diversity, and their low culturability, as well as the long maturation duration (He, Chen, and Chung Citation2022; Huang et al. Citation2018; Li et al. Citation2022). Therefore, the intimate knowledge of the interactions between the detected microorganisms, their synergistic or antagonistic, fermentation environments, and product quality using the current advanced technology will help improve the comprehension of Sufu fermentation and the ability to control its production.
Other products
Apart from these known fermented soybean products, other forms have also been subjected to fermentation to improve their nutrition and sensory quality. For instance, soybean paste (dajiang) was subjected to a spontaneous fermentation process to produce a high-value fermented product under a solid state (An et al. Citation2021; Wu et al. Citation2013). The microbiota present during the fermentation showed a high abundance of bacterial species, including T. halophilus, Enterococcus faecium, Leuconostoc mesenteroides, Leuconostoc gasicomitatum, Paenibacillus glycanilyticus, Staphylococcus epidermidis, and Bacillus firmus, which stayed predominant during the fermentation except for S. epidermidis. For fungi, several species including A. oryzae, A. elegans, Mucor wutongqiao, M. racemosus, R. oryzae, R. oligosporus, Zygosaccharomyces rouxii occurred in the early stage with a high population but mostly declined with the fermentation progression (An et al. Citation2021; Liu, Chen, et al. Citation2022; Wu et al. Citation2013; Yue et al. Citation2021). The correlation between the high abundance of the indigenous isolates including Tetragenococcus, Lactobacillus, and unclassified Enterocococcaceae, and the high levels of acetic acid, benzaldehyde, and esters linked to the desirable aroma and taste of soybean paste was observed by (An et al. Citation2021; Wu et al. Citation2013). Similarly, Soybean meal has been subjected to spontaneous solid-state fermentation processes to improve its sensory and nutritional quality. The studies showed reported a high abundance of Pseudomonas species during the early stage of fermentation which was replaced by Bacillus species including B. cereus, B. subtilis, and B. amyloliquefacien as fermentation progressed (Medeiros et al. Citation2018; Wang, Zhang, et al. Citation2021). Various enzymes were detected, including α-amylase, protease, and cellulase, which correlated to the increase in water content and water-soluble protein associated with the Bacillus growth. Relevant research also illustrated that the soybean meal supports the growth of R. oryzae and Ceratocystis fimbriata, producing several metabolites including acids, alcohols, ketones, esters, and aldehydes with strong fruity aroma (Bramorski, Christen, et al. Citation1998; Bramorski, Soccol, et al. Citation1998).
Comparison of microbial roles in spontaneously fermented soybean products
Based on the aforementioned discussions, similar microorganisms have been detected during multiple spontaneous fermentations of soybean products with a variety of functions. shows selected microorganisms and the reported main impact on different fermented soybean products. Three different fermentation types occur in soybeans. The first is alkaline fermentation, which is dominated by a wide range of Bacillus species including B. subtilis, B. amyloliquefaciens, B. licheniformis, B. pumilus, and B. velezensis. These species share hydrolytic capabilities, mainly their proteases, and polyglutamic acid production, in addition to their strain-specific properties (Sarkar, Cook, and Owens Citation1993; Shrestha, Dahal, and Ndungutse Citation2013). The resulting fermented products, such as Natto, Kinema, and Douchi, all mainly have a sticky appearance and powerful pungent odor. These findings might help to comprehend the typical roles of Bacillus species that might occur in soybean fermentation, considering the other changes that are highlighted in the earlier discussion for each Bacillus strain. The second type is fungal fermentation, which is dominated by filamentous fungi including Aspergillus, Rhizopus, and Mucor species. Examples of this type include Tempeh, Miso, Sufu, and Soya sauce. In Tempeh and Miso, the fungal growth causes the beans to fuze together by the fungal mycelium and its extracted enzymes that facilitate the mycelium attachment to the bean surface give these products their typical appearance as mycelial biomass with a softening texture (Wikandari et al. Citation2021). Additionally, several fungal enzymes were detected, including proteolytic, lipolytic, carbohydrate hydrolytic enzymes, and esterase, all of which play similar roles in increasing the bean digestibility and creating volatiles, mainly belonging to aldehydes and alcohols (Ghosh and Ray Citation2011; Mei Feng, Ostenfeld Larsen, and Schnürer Citation2007). Miso, Sufu, and Soya sauce are subsequently subjected to the third type of fermentation (maturation), which continues as either brine fermentation (submerged) in Sufu and Soya Sauce or continues as salt solid-state fermentation in the former product. Both types are dominant with LAB such as T. halophilus and yeasts such as Z. rouxiiin, which exhibit common osmophilic properties (Lee, Lee, and D. K. B. T.-A. M. Citation2002). During the maturation process, desirable volatiles, mainly esters, alcohols, and organic acids, are generated that give the final product its distinct fruity aroma profile, as well as several enzymes that continue the degradation process of lipid, fiber, and protein and soften their texture (Nout, Sarkar, and Beuchat Citation2007). Other subtypes of fermented soybeans, such as Manascus, which produces the characteristic red-colored Sufu, are included in the third fermentation (Wang, Sun, et al. Citation2019). These findings illustrate the crucial roles of endogenous microorganisms in soybean fermentation that can be manipulated to better control the fermentation process and produce high-quality products, as well as create novel fermented foods.
Table 1. Comparison of microbial roles in spontaneously fermented soybean products.
Starter culture technology for soybeans fermentation
Traditional spontaneous fermentations have long been used to produce fermented beverages and foods where the inoculum needed to kickstart the fermentation process consists of either the microorganisms found naturally in the product or the addition of a small amount of old fermented sample to a new batch (Caballero, Trugo, and Finglas Citation2003). Although these processes produce oriental and traditional sensory products, certain drawbacks such as contamination with undesirable microorganisms, and slow or failed fermentation can result in inconsistent fermentation products, and hence are not suitable for large-scale production facilities (Caballero, Trugo, and Finglas Citation2003). Nowadays with modern technology, starter cultures consisting of identified microorganisms are inoculated into food materials to produce fermented products, aiming to improve product stability, nutritional and sensory quality, consistency, and process economics (Hansen Citation2014). In soybean fermentation, several microorganisms including bacteria, yeasts, and filamentous fungi have been used as starter cultures and their roles have been studied.
Bacillus subtilis starter cultures
Soybeans fermented by B. subtilis inoculums produce Natto, as known in Japan, and similar products with different names in other countries, such as Douchi in China, Chung-kook-jong in Korea, and Kinema in Thailand. The fermented product is characterized by a slimy white appearance, soft texture, and powerful odor. Several conditions reported to affect Bacillus activity include inoculum size, water activity, salt concentration, pH, and temperature. Low inoculum size causes a delay in growth while higher levels cause exhaustion of the nutrients before bacterial enzyme production starts (Uyar and Baysal Citation2004). During fermentation, B. subtilis converts carbohydrates into organic acids causing a decline in pH at the beginning. Subsequently, soybean proteins are hydrolyzed by B. subtilis and this produces ammonia and amines that gradually increase pH to 8.34 in 48 h (Sarkar, Cook, and Owens Citation1993; Sarkar and Tamang Citation1995). The moisture content of the fermented beans is also increased through the Bacillus fermentation. The decline of soybean protein concentration is associated with the accumulation of microbial proteins, ending with a slight increase in the overall protein concentration at the end of the fermentation (Hu et al. Citation2010; Shrestha and Noomhorm Citation2001). Similarly, lipids and carbohydrates are hydrolyzed during fermentation, leading to degradation products such as free fatty acids and sugars (Sarkar, Cook, and Owens Citation1993; Sarkar and Tamang Citation1995). Further physiochemical changes also potentially impact the texture and sensory quality of the final products (Shrestha, Dahal, and Ndungutse Citation2013). Such changes are associated with improved bean digestibility, removal of beany odor, and reduction of indigestible oligosaccharides (Sarkar, Cook, and Owens Citation1993). Enzymatic activities have also been reported to produce several volatiles, including ketone, aldehydes, esters, and sulfur compounds that contribute significantly to the soybean sensory quality (Owens et al. Citation1997). The main volatiles found in fermented Natto were butanone, 2-methyl butanoic acid, trimethyl pyrazines, 2-pentrylfuran, 3-hydroxy butanone, and dimethyl disulfide that were believed to improve Natto sensory quality (Leejeerajumnean et al. Citation2001; Liu, Song, and Luo Citation2018). Furthermore, B. subtilis increases the total phenolic compounds, anthocyanins, and isoflavones, known for their beneficial health impact linked to glucosidase activities (Hu et al. Citation2010). Kinema was produced by subjecting soybeans to B. subtilis starter culture under different inoculum sizes, from about 2 to 7 log CFU/g, and monitoring under different incubation temperatures (18-52 °C) aiming to better control the fermentation process and improve the final product quality (Sharma et al. Citation2015). The authors found that optimum conditions were 3.0 log CFU/g, and fermentation at 37 °C for 48 h that produced a high-quality product with a nutty flavor, mild ammonia, and a highly sticky texture. It was also associated with a significant reduction in the concentrations of anti-nutritive factors including phytic acid, trypsin inhibitor activity, tannic, and total biogenic amines.
Other Bacillus species are also used to produce fermented soybeans, such as B. amyloliquefaciens which was isolated from a naturally fermented soybean process. Studies have shown that its high proteolytic and β-glucosidases activities decrease fermentation time, and lead to an increase in the total phenolic, flavonoid, and antioxidant levels associated with a reduction in the anti-nutritive factors (Cho et al. Citation2003; Jiang et al. Citation2019; Wang, Zhang, et al. Citation2021; Yang et al. Citation2019). Bacillus polyfermenticus was also isolated from Meju (a Korean soybean fermentation starter). It had high fibrinolytic and proteolytic enzymatic activities and higher poly-glutamate production, and the resultant fermented beans showed a different texture of lower viscosity than that produced with the traditional B. subtilis (Mo et al. Citation2010). Similarly, Bacillus velezensis was isolated from fermented soybeans ‘Douchi’ and was shown to eliminate glycinin and part of β- conglycinin (allergenic proteins) in soybean and hydrolysis soybean protein which significantly improves digestibility of the beans (Liu, Chen, et al. Citation2021). Furthermore, the authors reported their broad-spectrum antimicrobial effects on bacteria, yeast, and filamentous fungi which may suppress contaminant growth when fermentation is conducted under non-sterile conditions. shows the main Bacillus species and their characteristics and contributions to soybean fermentation.
Table 2. Bacterial and yeast starter cultures diversity in soybean fermentation.
LAB starter cultures
The most common products using LAB starter culture in soybean submerged fermentation are soy milk and fermented soya yogurt. L. plantarum is used to ferment soymilk and it improves the product texture through the production of exopolysaccharides. Similarly, Levilactobacillus brevis and L. plantarum inoculated into soybean milk produce a soy yogurt with significantly high concentrations of amino acids, aglycones, and high antioxidant capabilities (Hwang et al. Citation2021). Other LAB species such as Bifidobacterium breve, Lactobacillus rhamnosus, Lacticaseibacillus paracasei, Lc. casei, and Liquorilactobacillus mali have been reported to add pleasant flavors, aroma, and various bioactive compounds which improve human health (Marazza et al. Citation2012; Shimakawa et al. Citation2003; Sirilun et al. Citation2017). In Soya sauce, T. halophilus is inoculated into the submerged fermentation process (moromi, 12% NaCl), and its contribution to the finished product quality has been reported (Singracha et al. Citation2017). The microorganism is capable of reducing the level of biogenic amines and increasing desirable metabolite levels including acids, aldehydes, alcohols, ester, and phenol which improves the overall product safety and quality (). In detail, alcohols such as phenyl ethyl alcohol, 3-methyl-1-butanol, ethanol, and 2-methyl-1-butanol are among the highest alcohols (Zhao et al. Citation2018). For esters, ethyl acetate was the most common ester found, while 2-methyl butanal were aldehydes and ketones. These substances are correlated to fruity, sweet pleasing aroma characteristics. For phenols, guaiacol and 4-ethyl guaiacol were the most common flavor compounds detected that could contribute to the smoky aroma of Soya sauce (Zhao et al. Citation2018).
For solid-state fermentation, soybean paste has also been inoculated with T. halophilus, showing higher concentrations of lactic acid and acetic acid that enhance product stability, color, and prevention of rancidity (Cui et al. Citation2012). Various volatile compounds were also detected with higher concentrations compared to the spontaneously fermented paste, such as acetate, isoamyl alcohol, ethanol, and 4-ethyl phenol which improved the final product sensory quality and masked the undesirable soybean flavor. The authors also stated that the lactic acid produced during LAB activity created an optimal environment for yeast growth, performing a synergistic action between both microbial groups and enhancing the production of secondary desirable metabolites (Cui et al. Citation2012). Other LAB species such as L. plantarum, P. acidilactici, and Staphylococcus carnosus were applied to soybean paste and Miso fermentation processes and showed potential improvement in the fermented soybean product safety by reducing the concentration of biogenic amines (Lee et al. Citation2016; Nguyen et al. Citation2007; Zhao et al. Citation2020). In addition, higher levels of esters (isopentyl acetate), alcohols (phenyl ethyl alcohol and 2,3-butanediol), aldehydes (benzene acetaldehyde), aspartic acid, and glutamic acid were found in the inoculated beans compared to the non-treated beans, providing the fermented beans with fruity, nutty, sweet, and umami sensory quality (Zhao et al. Citation2020).
Yeast starter cultures
Yeasts are not the main fermenting microbial group in soybean fermentation, and their roles are not well-illustrated and sometimes controversial. Yeasts such as Saccharomyces, Klyveromyces, Debaryomyces, Hansenula, Candida, Pichia, Rhodoturola, and Zygosaccharomyces have been isolated during meju fermentation. They were reported to have high enzymatic activities such as protease, galactosidase, invertase, and inulinase that led to increased protein content, improved antioxidant activities, and reduced crude fiber levels in the resultant fermented beans (Lee et al. Citation1997; Rashad et al. Citation2011). On the other hand, kinema (a Nepalese fermented soybean product) produced by yeast fermentation such as C. parapsilosis and G. candidum had low quality and were associated with an undesirable rancid smell (Sarkar and Tamang Citation1994).
Overall, despite yeasts not being the main fermenting microorganism, recent attempts to inoculate yeasts in soybeans have still been reported, which shows their potential capability of improving the final product quality (). The organoleptic properties of Soya sauce were found to be improved using Z. rouxii individually or in co-culture with Meyerozyma guilliermondii and Candida variabilis after earlier LAB fermentation, producing desirable metabolites such as furanone, and higher alcohols with sweet and caramel flavor notes (Hayashida, Nishimura, and Slaughter Citation1997; Kim et al. Citation2011; Wah et al. Citation2013). A similar finding was also recorded using Z. rouxii individually or in co-culture with T. halophilus which showed potential flavor improvement during the Soya sauce maturation (Harada et al. Citation2017; Kwon and Kim Citation2005). Related studies showed Z. rouxii was found to produce a wide range of volatiles Among them, 4-hydroxy-5-methyl-3(3 H)-furanone was considered the crucial compounds that give Soya sauce and Miso their characteristic aroma (Ebine Citation2004; Hanya and Nakadai Citation2003). Similarly, the isolated C. versatilis was shown to produce various metabolites where higher alcohols, including isobutyl alcohol, isoamyl alcohol, and 2-phenyl ethanol, were essential aroma compounds of Soya sauce, but not with Miso (Aidoo, Nout, and Sarkar Citation2006; Ebine Citation2004).
For solid-state fermentation, Kluyveromyces marxianus was inoculated into Okara (the insoluble residues that are produced during soy milk and tofu manufacturing) and the fermented product showed higher levels of crude fat, soluble dietary fibers, and polysaccharides and a reduction in anti-nutritive factors such as phytic acid, trypsin inhibitors, and beany odor (Hu et al. Citation2019). A related study using Lindnera saturnus confirmed the hypothesis of metabolizing aldehydes such as 2-heptenal and hexanal which are responsible for the soybean green beany flavor, by bioconversion into the corresponding ester compounds, including ethyl hexanoate and ethyl heptanoate, which possess fruity and sweet aroma (Vong and Liu Citation2018).
Filamentous fungi starter culture
Among the filamentous fungi isolated during spontaneous soybeans fermentation, three main species belonging to Mucor, Aspergillus, and Rhizopus are commonly used in controlled solid-state fermentation. shows the most common filamentous fungi and their roles in soybean fermentation. Overall, the contribution of the filamentous fungi in soybean is mostly observed during solid-state fermentation type and if submerged fermentation is subsequently included their growth is potentially declined, however, the excreted enzymes during the former fermentation continue their functional activities under submerged fermentation (Liu, Chen, et al. Citation2021).
Table 3. Filamentous fungi starter cultures diversity in soybean fermentation.
Mucor species such as M. racemosus, M. Sufu, and A. elegans are the most common and characterized by narrow temperature tolerance between 20–30 °C and a slow growth rate out of this temperature range that limited their application during summer (Li et al. Citation2008; Yamasaki and Konno Citation1991). The inoculated beans were first covered with small white colonies and were later fully covered with white to grey mycelium in 2–3 days. A. elegans is used to ferment Okara and the resultant fermented products showed alkaline pH, higher levels of amino acids, more degraded fiber content, and masking the fishy smell of soybeans with a smooth mouthfeel that improves their sensory quality (Krishna Citation2005; Kronenberg and Hang Citation1984; Xu, Liu, and Zhou Citation2012). Sufu is mainly produced by using A. elegans and A. taiwanensis during the solid-state fermentation step (Pehtze) at a low temperature of 15–20 °C (Cheng et al. Citation2009). This low temperature is more favorable to Mucor spp. and not for bacteria, yeasts, and other filamentous fungi, and is characterized by high Mucor enzymatic activities of proteases, lipases, amylases, and galactosidases (Liu, Chen, et al. Citation2021). The fungal enzymes produced during the solid-state fermentation were responsible for the continuous degradation of the bean ingredients during subsequent processes liberating degraded compounds including free amino acids, fatty acids, and sugars in addition to secondary microbial metabolites. These physiochemical changes are crucial to producing Sufu with traditional organoleptic quality (Han, Rombouts, and Nout Citation2001; Liu, Chen, et al. Citation2021). Out of this temperature range, fermentation takes a long time or stuck making it difficult to produce fermented soybean products using these Mucor strains. Screening for alternative strains that have the capability to grow out of this temperature range received much attention recently. Mucor flavus spores were spread onto fresh tofu surfaces to produce Sufu at a low temperature (15 °C) and showed a significant growth rate with potential proteolytic enzyme activities that increased amino acid levels in the final product (Cheng et al. Citation2009). For high temperatures during summer, Rhizopus spp., such as R. arrhizus, R. oligosporus, and R. chinensis perform with better growth making them suitable for the summer season production (Cui, Li, and Liu Citation2016; Ruiz‐Terán and David Owens Citation1996).
R. oligosporus and R. oryzae were isolated and evaluated to be used as a starter culture to produce Tempeh (Baumann and Bisping Citation1995). The two strains showed high proteolytic activities releasing a significant amount of amino acids that are crucial for Tempeh quality regarding alkalinization, taste, and sliceability (Baumann and Bisping Citation1995; Sparringa and Owens Citation1999). R. oligosporus was also reported to increase the level of vitamins during Tempe production including riboflavin, nicotinamide nicotinic acid, and vitamin B6, but not B12 (Keuth and Bisping Citation1993). Furthermore, they reported with high capability to degrade most of the soybean polysaccharides such as raffinose, stachyose, sucrose, and melibiose reducing their negative nutritive impact on soybean consumption (Handoyo and Morita Citation2006; Rehms and Barz Citation1995). Related studies illustrated the antimicrobial activities of the resultant fermented soybeans of R. oligosporus (Kusumah et al. Citation2020; McCue et al. Citation2004). The authors believed fatty acids such as linoleic acid and α-linolenic acid 1-monolinolenin and 2-monolinolenin exhibited antimicrobial activity against gram-positive food pathogens including S. aureus and B. subtilis.
For Aspergillus, Koji is a common mold starter culture that mainly contains Aspergillus species such as A. oryzae, A. kawachii, A. sojae, A. awamori, and A. shirousamii used to produce Soya sauce, Miso, and other fermented soybean products (Kitamoto Citation2002; Matsushita et al. Citation2009). A. oryzae or A. sajae are the most popular and important among them being potential saccharification, and diastatic agents and positively contributing to the color, aroma, and sensory quality of the fermented beans, and neither of them produces mycotoxins (Kitamoto Citation2002). Generally, the soybeans with wheat flour are mixed with 0.05–0.3% w/w of the fungal spores and loaded into trays with layers depth of 3–5 cm (Devanthi and Gkatzionis Citation2019; Yan et al. Citation2013). During the fermentation, the inoculated mold showed high enzymatic hydrolysis of soybean components including proteins into small peptides and amino acids by proteinases, and starch degraded into simple sugars by α-amylases. These enzymatic hydrolyzes decrease the acidity from pH 6.5 to 7.3 and increase the internal temperature, in addition, the fungal mycelia grow and sporulate on the soybean surface producing a greenish mass within three days (Devanthi and Gkatzionis Citation2019; Luh Citation1995). The resultant fermented beans were reported with several desirable flavors and aroma compounds including esters and higher alcohols similar to that reported with fungal fermented soybean products mentioned above. Moreover, glutamic acid, which is a key compound produced by glutaminase during koji fermentation, is a crucial component of the umami flavor (Ohata et al. Citation2007; Zhu, 2013). The degraded compounds of protein and polysaccharides by the koji enzymes were also suggested to contribute to several flavor compounds created after fermentation during the ripening process such as the Maillard reaction giving the final products their characteristic sensory quality (Ogasawara, Yamada, and Egi Citation2006). Relevant research used other isolates such as Aspergillus awamori and A. fumigatus showed their high enzymatic activities that were correlated to a significant increase in the levels of amino acids, desired flavor metabolites, and antioxidant activities of the fermented products (Ghanem et al. Citation2020; Wardhani, Vázquez, and Pandiella Citation2009).
Comparative studies were conducted to investigate the impact of different mold species inoculated individually or combined into a soybean fermentation process. The data showed that the fermented sample by A. elegans and R. arrhizus at a ratio of 5:1 showed a high level of key desirable volatiles, especially ethyl caproate which has a low threshold of fruity and pineapple odor notes (Cui, Li, and Liu Citation2016). R. oryzae by itself was associated with high levels of degraded compounds including fatty acids, and furans sulfur-containing volatiles. A. oryzae was strongly related to degraded sugars such as arabinose, mannose, and mannitol, as well as free amino acids, methionine, serine, and isoleucine while ketoglutaric acid-derived amino acids were detected with Penicillium glabrum (Park and Kim Citation2020; Sun et al. Citation2019). These studies illustrated that the quality of the fermented product is strongly correlated to the type of the microorganism present and its metabolic activities (Lee et al. Citation2017; Z. Li et al. Citation2017; Park and Kim Citation2020). Overall, the filamentous fungi strains that were applied to the fungal fermented soybean products mostly declined during the long subsequent brine fermentation process. Further research may be considered to identify novel strains and strategies for overcoming the long fermentation time, high salt and acid contents, and producing high-quality final products.
Criteria for selecting and developing starter cultures
According to the literature data, the microflora of spontaneous soybean fermentation is complex and its contribution to the final product quality is varied. To convert the spontaneous fermentation process into a controlled one using starter culture technology, three main areas need to be fulfilled (Vinícius de Melo Pereira et al. Citation2017). The first one is the ability of the selected microorganisms to carry out the desired fermentation biotransformation. The second is the final product quality that can be achieved and the last is the feasibility of a commercially developed process (see ).
Figure 3. Starter culture selective criteria in soybean fermentation. This figure was created using BioRender (https://biorender.com/).
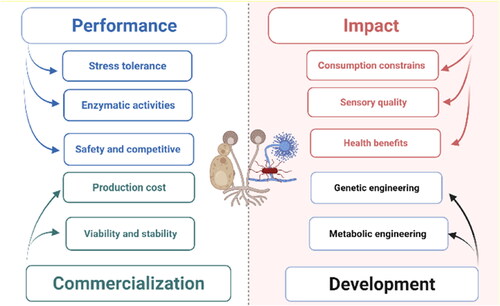
As illustrated earlier, soybean ecology harbors a wide range of indigenous microorganisms, and these isolates might compete with the starter culture inoculum. Furthermore, foodborne pathogens, mycotoxin-producing fungi, and other contaminants that produce undesirable metabolites were also detected during the spontaneous fermentation process (Anal et al. Citation2020; Jeong et al. Citation2016; Woo et al. Citation2019; Yoon, Kim, and Hwang Citation2008). This type of complicated ecology must be considered during selecting and evaluating the microorganisms for starter culture applications. Firstly, the selected strains should be characterized by high adaptation to the fermentation environment and are potentially able to compete with the natural microflora. Such criteria might be studied by subjecting the selected isolates to grow under several stressors such as high temperature, wide pH values, organic acids, osmotic, and alcohols as a common feature of the fermentation environment and monitoring their tolerance capabilities. Successful growth under these harsh associated stressors is a potential indicator of the high fermentation performance of the isolates as starter cultures, considering that the performance is mostly strain specific. Similar approaches were applied for the early evaluation of the selected isolates before application in starter culture technology for other foods (dos Santos Cruxen et al., Citation2019; Elhalis et al. Citation2021a; Pereira et al. Citation2012). The inoculum density and timing of the inoculation need to be taken into consideration. Studies indicate that a high inoculum size increases the likelihood of successful fermentation and avoids the risk of stuck and sluggish fermentation under some circumstances (Lleixà et al. Citation2016; Papagianni and Mattey Citation2006). Timing is also crucial for fermentation success, especially with cocultures (Henick-Kling and Park Citation1994). With the increase interesting in novel fermented products, cocultures of fungi and bacteria can work. However, evaluation studies should be conducted to ensure whether they are more efficient if they are working together or inoculated sequentially. Under some circumstances, especially long-time fermentation such as cocoa and cheese, microorganisms are required to initiate the activity of another microbial group in balance throughout the fermentation process (Giraffa Citation2004; Ho, Zhao, and Fleet Citation2014; Schwan and Wheals Citation2004). This pattern should be considered during designing the starter cultures for similar soybean fermentation types, i.e., Soya sauce, ensuring the right inoculation timing for each microbial strain. Secondly, data from the literature show a high concentration of protein, lipid, and oligosaccharides in soybeans. These compounds require high microbial enzymatic activities including proteases, lipases, and amylases to be degraded. Various methods have been applied to screen these enzymes and measure their activities that were involved in the selection process of promising starter cultures in similar food products (Kasana, Salwan, and Yadav Citation2011; Li et al. Citation2021; Thomson, Delaquis, and Mazza Citation1999). The development of a novel procedure to monitor enzyme activity on a real plant matrix, like soybeans, is equally important, but challenging. Finally, the selected strain safety and its possible productivity of the antagonist compound against the natural microflora contaminate should be considered and evaluated (Abass et al. Citation2017; Cintas et al. Citation1998; dos Santos Cruxen et al., 2019; Elhalis et al. Citation2021a; Koutinas et al. Citation2009; Russo, Spano, and Capozzi Citation2017; Thumu and Halami Citation2020). For instance, literature data showed a wide range of LAB naturally presented with several fermented soybean products including Lv. brevis, L. plantarum, P. acidilactici, T. halophilus, and E. faecium (Liu et al. Citation2012; Ouoba et al. Citation2005; Sarkar et al. Citation1994). Studying these species showed that most of them are halophilic and can grow at high pH values; from pH 5–9, making them suitable to be used in soybean starter culture technology (Chen, Yanagida, and Hsu Citation2006; Holzapfel et al. Citation2006). In addition, they produce various secondary metabolites that were reported to impact fermented product sensory quality. Furthermore, they were potential producers of various organic acids and other antimicrobial metabolites that inhibit food contaminants and foodborne pathogens (Chen, Yanagida, and Hsu Citation2006; Holzapfel et al. Citation2006; Sarkar and Nout Citation2014). Such features make them suitable candidates and might be considered for starter culture trials.
One of the major soybean constraints for human consumption is the presence of anti-nutritive factors, in addition to their low digestibility and palatability (Handoyo and Morita Citation2006; Li et al. Citation2019; Mukherjee, Chakraborty, and Dutta Citation2015; Vagadia, Vanga, and Raghavan Citation2017). The roles of main microbial isolates involved in spontaneous soybean fermentation processes were elucidated through this literature review to facilitate the selection to overcome these limitations. For example, A. sojae and A. ficuum were reported to degrade phytic acid and oligosaccharides (Olukomaiya et al. Citation2020). Similarly yeasts such as Saccharomyces cerevisiae and C. utilis and LAB such as L. plantarum reduced oligosaccharide, trypsin inhibitors, and phytate (Adeyemo and Onilude Citation2013; Kasprowicz‐Potocka et al. Citation2018). So far, the possibility of reducing most of the anti-nutritive factors by fermentation is illustrated, thus, attention should be paid to the promising candidates with positive attributes of reducing or eliminating the anti-nutritive factors. Several traditional and advanced methods have been applied to monitoring the soy consumption constraints such as trypsin inhibitors (Hamerstrand, Black, and Glover Citation1981; Liu Citation2019), phytic acid (Haug and Lantzsch Citation1983; Skoglund, Carlsson, and Sandberg Citation1998), beany flavor (Lu et al. Citation2013; Surrey Citation1964). The microbial contribution to final product sensory quality is another important impact that should be investigated during the evaluation study. As explained in this literature review, during soybean fermentation microorganisms create a wide range of metabolites such as higher alcohols, ketones, aldehydes, and esters that potentially modulate the product taste. Each microorganism has been shown to produce distinct volatile and nonvolatile profiles associated with characteristics of sensory quality that are different from each product to another. Sensory analysis is usually conducted by determining the aroma profile of each isolate was performed using mainly headspace solid-phase micro-extraction (HS-SPME) gas chromatograph equipped mass spectrometer GCMS method (Elhalis et al. Citation2021b; Jacobsen and Hinrichsen Citation1997; Motlhanka et al. Citation2022; Sørensen et al. Citation2011; Wang et al. Citation1997). For nonvolatiles, high-performance liquid chromatography HPLC and ultra-high-performance liquid chromatography were commonly applied (Elhalis, Cox, and Zhao Citation2020; Yi and Hong Citation2021), while the electronic tongue and trained panel are involved at the end (Da Silva et al. Citation2012; Tuitemwong et al. Citation1993).
For commercial purposes, two main factors relating to the design of starter cultures should be fulfilled. First, the selected starter culture strains should be suitable to be cultivated on cheap substrates to decrease the overall production costs. Secondly, selected starter culture strains should tolerate the downstream processing including drying, packaging, freeze-drying, and rehydration, and have high stability during storage without losing their fermentation performance and properties (Fleet Citation2008; Soubeyrand, Julien, and Sablayrolles Citation2006). Such development of commercialization may be affected by the Nagoya Protocol on benefit sharing (Johansen Citation2017). Before starting any research and development work on biological resources, according to the concept of this protocol, prior informed consent by the provider country is needed. This can be completed based on mutually agreed terms describing access to the materials and benefit shares that may be monetary or non-monetary (Cooper Citation2023).
Further development in starter culture technology might also be achieved by genetic improvement using current molecular techniques. High-throughput screening for specific genes and metabolic pathways has been widely applied to achieve starter cultures strains with high capability to metabolize a wide range of inexpensive substrates such as lignocellulosic agricultural and forest residues (Hatti-Kaul et al. Citation2018). Furthermore, genes may be introduced to increase the productivity of desirable metabolites or deleted to avoid undesirable metabolic pathways, such as with LAB in dairy fermentations (Weckbecker and Hummel Citation2006; Xiao et al. Citation2010; Yu et al. Citation2008; Zhang et al. Citation2012). Such technology may enhance the positive contribution of starter cultures to food, decrease the production cost and improve stability, which is essential in today’s economic and sustainability goals, but the genetically modified organisms (GMOs) policy may raise some concerns.
Conclusion and future prospects
Soybeans are rich protein sources with a balanced amino acid content but lack sulfur-containing amino acids, combined with several consumption constraints such as beany flavor, trypsin inhibitors, phytic acid, and oligosaccharides. In Asia, they have been subjected to traditional fermentation processes and their microbial ecology is highly complicated and varied from one product to another. This literature review demonstrated the crucial roles of the fermentation process and the potential improvement of applying starter culture technology. Overall, more diverse fungi and bacteria were detected during the fermentation of Miso, Sufu, and Soya sauce while other products such as Tempeh and Natto are either mainly harbored by filamentous fungi and Bacillus ssp., respectively. The quality of the resultant fermented products varies depending on the natural microflora, the processing type of either solid-state, submerged state, and the processed ingredients. Several studies have confirmed the microbial capability to degrade soybean anti-nutritive factors, remove unpleasant beany flavors, and improve its digestibility. Starter culture applications have been an alternative way to control the fermentation process, improving the sensory quality, and enhancing its safety, nutritional, and health effects. Such strains should have high fermentation performance and enzymatic activities to tolerate the harsh fermentation process. They also should create the desired flavor, aroma, and texture and positively address various soybean constraints including oligosaccharides, trypsin inhibitors, phytates, and beany flavor. Furthermore, the ability to be commercialized and their viability during storage should be considered. Advanced understanding of spontaneous fermentation processes and starter culture technology could open a new way in directing future researchers to overcome the soybean consumption constraints, improving the final product safety, quality, nutritional and health benefits in more economic and sustainable aspects, as well as creating novel products. This understanding can be further improved using synthetic biology and strain engineering molecular techniques, as well as omics technologies, including metabolomics, which is not well applied in soybean fermentation. Further improvement that has not been well studied, to the best of the authors’ knowledge, is the application of bioreactors in soybean fermentation processes. Compared with other food fermentations such as yogurt and wines, bioreactors may offer potential capabilities to improve the overall process by reducing the cost, increasing safety, accelerating the process, and improving product consistency. Currently, most soybean-submerged fermentations are conducted in wood, concrete, epoxy cement, or steel tanks, while solid-state fermentations are conducted on individual trays and handled manually. Further investigation is required to improve the overall quality and increase the production scale in response to the current demand for sustainable protein sources.
Authors’ contributions
H.E.: compiled data from literature review and drafted the manuscript; C.X.H.: revised the manuscript; Y.C.: Supervision, wrote, Reviewed and Edited. All authors have read and agreed to the published version of the manuscript.
Acknowledgments
The authors gratefully acknowledge financial support from the Agency for Science, Technology and Research (A*STAR).
Disclosure statement
No potential competing interest was reported by the authors.
Additional information
Funding
References
- Abass, A. B., W. Awoyale, M. Sulyok, and E. O. Alamu. 2017. Occurrence of regulated mycotoxins and other microbial metabolites in dried cassava products from nigeria. Toxins 9 (7):207. doi: 10.3390/toxins9070207.
- Adams, M., and M. Moss. 2008. Food microbiology. Third Edition, 463. Cambridge, UK: The Royal Society of Chemistry. https://www.academia.edu/36453321/Food_Microbiology_Third_Edition.
- Adams, M., and R. Mitchell. 2002. Fermentation and pathogen control: A risk assessment approach. International Journal of Food Microbiology 79 (1–2):75–83. doi: 10.1016/S0168-1605(02)00181-2.
- Adeyemo, S. M., and A. A. Onilude. 2013. Enzymatic reduction of anti-nutritional factors in fermenting soybeans by Lactobacillus plantarum isolates from fermenting cereals. Nigerian Food Journal 31 (2):84–90. doi: 10.1016/S0189-7241(15)30080-1.
- Ahnan‐Winarno, A. D., L. Cordeiro, F. G. Winarno, J. Gibbons, and H. Xiao. 2021. Tempeh: A semicentennial review on its health benefits, fermentation, safety, processing, sustainability, and affordability. Comprehensive Reviews in Food Science and Food Safety 20 (2):1717–67. doi: 10.1111/1541-4337.12710.
- Aidoo, K. E., M. J. Rob Nout, and Sarkar, P. K. 2006. Occurrence and function of yeasts in Asian indigenous fermented foods. FEMS Yeast Research 6 (1):30–9. doi: 10.1111/j.1567-1364.2005.00015.x.
- Allwood, J. G., L. T. Wakeling, and D. C. Bean. 2021. Fermentation and the microbial community of Japanese koji and miso: A review. Journal of Food Science 86 (6):2194–207. doi: 10.1111/1750-3841.15773.
- Amadou, I., T. Amza, M. B. K. Foh, M. T. Kamara, and G.-W. Le. 2010. Influence of Lactobacillus plantarum Lp6 fermentation on the functional properties of soybean protein meal. Emirates Journal of Food and Agriculture 22 (6):456–65. doi: 10.9755/ejfa.v22i6.4663.
- An, F., M. Li, Y. Zhao, Y. Zhang, D. Mu, X. Hu, S. You, J. Wu, and R. Wu. 2021. Metatranscriptome-based investigation of flavor-producing core microbiota in different fermentation stages of dajiang, a traditional fermented soybean paste of Northeast China. Food Chemistry 343:128509. doi: 10.1016/j.foodchem.2020.128509.
- Anal, A. K., G. Perpetuini, A. Petchkongkaew, R. Tan, S. Avallone, R. Tofalo, H. V. Nguyen, S. Chu-Ky, P. H. Ho, T. T. Phan, et al. 2020. Food safety risks in traditional fermented food from South-East Asia. Food Control. 109:106922. doi: 10.1016/j.foodcont.2019.106922.
- Ashu, E., A. Forsythe, A. Vogan, and J. Xu. 2016. Filamentous fungi in fermented foods. Fermented Foods, Part I: Biochemistry and Biotechnology; Didier, M ., Ramesh, R., Eds, 60–90. Boca Raton: Taylor & Francis Group. doi: 10.1201/b19872-5.
- Aulitto, M., A. Strazzulli, F. Sansone, F. Cozzolino, M. Monti, M. Moracci, G. Fiorentino, D. Limauro, S. Bartolucci, and P. Contursi. 2021. Prebiotic properties of Bacillus coagulans MA-13: Production of galactoside hydrolyzing enzymes and characterization of the transglycosylation properties of a GH42 β-galactosidase. Microbial Cell Factories 20 (1):1–17. doi: 10.1186/s12934-021-01553-y.
- Avilés-Gaxiola, S., C. Chuck-Hernández, and S. O. Serna Saldívar. 2018. Inactivation Methods of Trypsin Inhibitor in Legumes: A Review. Journal of Food Science 83 (1):17–29. doi: 10.1111/1750-3841.13985.
- Baek, S.-Y., H.-J. Yun, H.-D. Park, and S.-H. Yeo. 2011. Isolation and optimized culture conditions of fibrinolytic enzyme producing strain isolated from Korean traditional soybean sauce. Microbiology and Biotechnology Letters 39 (4):330–6.
- Barus, T., A. Suwanto, A. T. Wahyudi, and H. Wijaya. 2008. Role of bacteria in tempe bitter taste formation: Microbiological and molecular biological analysis based on 16S rRNA gene. Microbiology Indonesia 2 (1):17–21. doi: 10.5454/mi.2.1.4.
- Baumann, U., and B. Bisping. 1995. Proteolysis during Tempe fermentation. Food Microbiology 12:39–47. doi: 10.1016/S0740-0020(95)80077-8.
- Bessada, S. M. F., J. C. M. Barreira, and M. B. P. P. Oliveira. 2019. Pulses and food security: Dietary protein, digestibility, bioactive and functional properties. Trends in Food Science & Technology 93:53–68. doi: 10.3389/fsufs.2021.681662.
- Bramorski, A., P. Christen, M. Ramirez, C. R. Soccol, and S. Revah. 1998. Production of volatile compounds by the edible fungus Rhizopus oryzae during solid state cultivation on tropical agro-industrial substrates. Biotechnology Letters 20 (4):359–62. doi: 10.1023/A:1005323229125.
- Bramorski, A., C. R. Soccol, P. Christen, and S. Revah. 1998. Fruity aroma production by Ceratocystis fimbriata in solid cultures from agro-industrial wastes. Revista de Microbiologia 29 (3):208–12. doi: 10.1590/S0001-37141998000300012.
- Caballero, B., L. C. Trugo, and P. M. Finglas. 2003. Encyclopedia of food sciences and nutrition. San Diego, CA: Academic.
- Chancharoonpong, C., P.-C. Hsieh, and S.-C. Sheu. 2012. Enzyme production and growth of Aspergillus oryzae S. on soybean koji fermentation. APCBEE Procedia 2:57–61. doi: 10.1016/j.apcbee.2012.06.011.
- Chauhan, D., K. Kumar, N. Ahmed, P. Thakur, Q. U. E. H. Rizvi, S. Jan, and A. N. Yadav. 2022. Impact of soaking, germination, fermentation, and roasting treatments on nutritional, anti-nutritional, and bioactive composition of black soybean (Glycine max L.). Journal of Applied Biology & Biotechnology 10 (5):186–92. doi: 10.7324/JABB.2022.100523.
- Chen, Y., F. Yanagida, and J. Hsu. 2006. Isolation and characterization of lactic acid bacteria from suan‐tsai (fermented mustard), a traditional fermented food in Taiwan. Journal of Applied Microbiology 101 (1):125–30. doi: 10.1111/j.1365-2672.2006.02900.x.
- Cheng, Y.-Q., Q. Hu, L.-T. Li, M. Saito, and L.-J. Yin. 2009. Production of sufu, a traditional Chinese fermented soybean food, by fermentation with Mucor flavus at low temperature. Food Science and Technology Research 15 (4):347–52. doi: 10.3136/fstr.15.347.
- Chitra, U., U. Singh, and P. V. Rao. 1996. Phytic acid, in vitro protein digestibility, dietary fiber, and minerals of pulses as influenced by processing methods. Plant Foods for Human Nutrition (Dordrecht, Netherlands) 49 (4):307–16. doi: 10.1007/BF01091980.
- Cho, K. M., R. K. Math, S. Y. Hong, S. M. A. Islam, D. K. Mandanna, J. J. Cho, M. G. Yun, J. M. Kim, and H. D. Yun. 2009. Iturin produced by Bacillus pumilus HY1 from Korean soybean sauce (kanjang) inhibits growth of aflatoxin producing fungi. Food Control. 20 (4):402–6. doi: 10.1016/j.foodcont.2008.07.010.
- Cho, S.-J., S.-H. Oh, R. D. Pridmore, M. A. Juillerat, and C.-H. Lee. 2003. Purification and characterization of proteases from Bacillus amyloliquefaciens isolated from traditional soybean fermentation starter. Journal of Agricultural and Food Chemistry 51 (26):7664–70. doi: 10.1021/jf0259314.
- Chung, H. Y. 2000. Volatile Flavor Components in Red Fermented Soybean (Glycine max) Curds. Journal of Agricultural and Food Chemistry 48 (5):1803–9. doi: 10.1021/jf991272s.
- Cintas, L. M., P. Casaus, M. F. Fernández, and P. E. Hernández. 1998. Comparative antimicrobial activity of enterocin L50, pediocin PA-1, nisin A and lactocin S against spoilage and foodborne pathogenic bacteria. Food Microbiology 15 (3):289–98. doi: 10.1006/fmic.1997.0160.
- Cooper, D. 2023. Convention on Biological Diversity. https://www.cbd.int/
- Cui, X. H., M. A. Li, and P. Liu. 2016. The effect of different strains on the quality of fermented bean curd. China Condiment 41 (11):32–6. doi: 10.1111/1750-3841.14412.
- Cui, Y., X. Qu, H. Li, S. He, H. Liang, H. Zhang, and Y. Ma. 2012. Isolation of halophilic lactic acid bacteria from traditional Chinese fermented soybean paste and assessment of the isolates for industrial potential. European Food Research and Technology 234 (5):797–806. doi: 10.1007/s00217-012-1689-8.
- Da Silva, J., S. Prudencio, M. Carrão‐Panizzi, C. Gregorut, F. Fonseca, and L. Mattoso. 2012. Study on the flavour of soybean cultivars by sensory analysis and electronic tongue. International Journal of Food Science & Technology 47 (8):1630–8. doi: 10.1111/j.1365-2621.2012.03013.x.
- Dai, C., H. Ma, R. He, L. Huang, S. Zhu, Q. Ding, and L. Luo. 2017. Improvement of nutritional value and bioactivity of soybean meal by solid-state fermentation with Bacillus subtilis. LWT 86:1–7. doi: 10.1016/j.lwt.2017.07.041.
- De Vuyst, L., and S. Weckx. 2016. The cocoa bean fermentation process: From ecosystem analysis to starter culture development. Journal of Applied Microbiology 121 (1):5–17. doi: 10.1111/jam.13045.
- Denter, J., and B. Bisping. 1994. Formation of B-vitamins by bacteria during the soaking process of soybeans for tempe fermentation. International Journal of Food Microbiology 22 (1):23–31. doi: 10.1016/0168-1605(94)90004-3.
- Devanthi, P. V. P., and K. Gkatzionis. 2019. Soy sauce fermentation: Microorganisms, aroma formation, and process modification. Food Research International (Ottawa, Ont.) 120:364–74. doi: 10.1016/j.foodres.2019.03.010.
- Devanthi, P. V. P., R. Linforth, H. Onyeaka, and K. Gkatzionis. 2018. Effects of co-inoculation and sequential inoculation of Tetragenococcus halophilus and Zygosaccharomyces rouxii on soy sauce fermentation. Food Chemistry 240:1–8. doi: 10.1016/j.foodchem.2017.07.094.
- Dong, Q., and H. Saneoka. 2020. Physiological characteristics, phytase activity, and mineral bioavailability of a low-phytate soybean line during germination. Plant Foods for Human Nutrition (Dordrecht, Netherlands) 75 (3):383–9. doi: 10.1007/s11130-020-00827-x.
- dos Santos Cruxen, C. E., G. D. Funck, L. Haubert, G. da Silva Dannenberg, J. de Lima Marques, F. C. Chaves, W. P. da Silva, Â. M. Fiorentini, C. E. d S. Cruxen, G. D. Funck, et al. 2019. Selection of native bacterial starter culture in the production of fermented meat sausages: Application potential, safety aspects, and emerging technologies. Food Research International (Ottawa, Ont.) 122:371–82. doi: 10.1016/j.foodres.2019.04.018.
- Ebine, H. 2004. Industrialization of Japanese miso fermentation. In Food Science and Technology-New York-Marcel Dekker (99–142). Boca Raton: MARCEL DEKKER AG.
- Elhalis, H., J. Cox, D. Frank, and J. Zhao. 2020. The crucial role of yeasts in the wet fermentation of coffee beans and quality. International Journal of Food Microbiology 333:108796. doi: 10.1016/j.ijfoodmicro.2020.108796.
- Elhalis, H., J. Cox, D. Frank, and J. Zhao. 2021a. Microbiological and biochemical performances of six yeast species as potential starter cultures for wet fermentation of coffee beans. LWT 137:110430. a. doi: 10.1016/j.lwt.2020.110430.
- Elhalis, H., J. Cox, D. Frank, and J. Zhao. 2021b. The role of wet fermentation in enhancing coffee flavor, aroma and sensory quality. European Food Research and Technology 247 (2):485–98. doi: 10.1007/s00217-020-03641-6.
- Elhalis, H., J. Cox, and J. Zhao. 2020. Ecological diversity, evolution and metabolism of microbial communities in the wet fermentation of Australian coffee beans. International Journal of Food Microbiology 321:108544. a doi: 10.1016/j.ijfoodmicro.2020.108544.
- Elhalis, H., J. Cox, and J. Zhao. 2023. Coffee fermentation: Expedition from traditional to controlled process and perspectives for industrialization. Applied Food Research 3 (1):100253. doi: 10.1016/j.afres.2022.100253.
- Embaby, H. E.-S. 2010. Effect of heat treatments on certain antinutrients and in vitro protein digestibility of peanut and sesame seeds. Food Science and Technology Research 17 (1):31–8. doi: 10.3136/fstr.17.31.
- Erickson, D. R. 2015. Practical handbook of soybean processing and utilization. Champaign, IL: AOCS Press; St. Louis, Mo.: United Soybean Board.
- Evangelista, S. R., C. F. Silva, M. G. P. da Cruz Miguel, C. de Souza Cordeiro, A. C. M. Pinheiro, W. F. Duarte, and R. F. Schwan. 2014. Improvement of coffee beverage quality by using selected yeasts strains during the fermentation in dry process. Food Research International 61:183–95. doi: 10.1016/j.foodres.2013.11.033.
- FAOStat 2021. Statistical Yearbook 2021 - World Food and Agriculture. http://www.fao.org/faostat/en/#data.
- Fei, Y., L. Li, L. Chen, Y. Zheng, and B. Yu. 2018. High-throughput sequencing and culture-based approaches to analyze microbial diversity associated with chemical changes in naturally fermented tofu whey, a traditional Chinese tofu-coagulant. Food Microbiology 76:69–77. doi: 10.1016/j.fm.2018.04.004.
- Fleet, G. H. 2008. Wine yeasts for the future. FEMS Yeast Research 8 (7):979–95. doi: 10.1111/j.1567-1364.2008.00427.x.
- Gao, X.-L., C. Cui, H.-F. Zhao, M.-M. Zhao, L. Yang, and J.-Y. Ren. 2010. Changes in volatile aroma compounds of traditional Chinese-type soy sauce during moromi fermentation and heat treatment. Food Science and Biotechnology 19 (4):889–98. doi: 10.1007/s10068-010-0126-7.
- Gao, Y., C. Wang, Q. Zhu, and G. Qian. 2013. Optimization of solid-state fermentation with Lactobacillus brevis and Aspergillus oryzae for trypsin inhibitor degradation in soybean meal. Journal of Integrative Agriculture 12 (5):869–76. doi: 10.1016/S2095-3119(13)60305-6.
- Garrido-Galand, S., A. Asensio-Grau, J. Calvo-Lerma, A. Heredia, and A. Andrés. 2021. The potential of fermentation on nutritional and technological improvement of cereal and legume flours: A review. Food Research International (Ottawa, Ont.) 145:110398. doi: 10.1016/j.foodres.2021.110398.
- Geisen, R. 1993. Fungal starter cultures for fermented foods: Molecular aspects. Trends in Food Science & Technology 4 (8):251–6. doi: 10.1016/0924-2244(93)90140-6.
- Ghanem, K. Z., M. Z. Mahran, M. M. Ramadan, H. Z. Ghanem, M. Fadel, and M. H. Mahmoud. 2020. A comparative study on flavour components and therapeutic properties of unfermented and fermented defatted soybean meal extract. Scientific Reports 10 (1):1–7. doi: 10.1038/s41598-020-62907-x.
- Ghosh, B., and R. R. Ray. 2011. Current commercial perspective of Rhizopus oryzae: A review. Journal of Applied Sciences 11 (14):2470–86. doi: 10.3923/jas.2011.2470.2486.
- Giraffa, G. 2004. Studying the dynamics of microbial populations during food fermentation. FEMS Microbiology Reviews 28 (2):251–60. doi: 10.1016/j.femsre.2003.10.005.
- Guo, X., D. Li, W. Lu, X. Piao, and X. Chen. 2006. Screening of Bacillus strains as potential probiotics and subsequent confirmation of the in vivo effectiveness of Bacillus subtilis MA139 in pigs. Antonie Van Leeuwenhoek 90 (2):139–46. doi: 10.1007/s10482-006-9067-9.
- Hamerstrand, G. E., L. T. Black, and J. D. Glover. 1981. Trypsin inhibitors in soy products: modification of the standard analytical procedure
- Han, B.-Z., C.-F. Cao, F. M. Rombouts, and M. J. R. Nout. 2004. Microbial changes during the production of Sufu—a Chinese fermented soybean food. Food Control. 15 (4):265–70. doi: 10.1016/S0956-7135(03)00066-5.
- Han, B.-Z., F. M. Rombouts, and M. J. R. Nout. 2001. A Chinese fermented soybean food. International Journal of Food Microbiology 65 (1-2):1–10. doi: 10.1016/S0168-1605(00)00523-7.
- Han, B., J. Wang, F. M. Rombouts, and M. J. R. Nout. 2003. Effect of NaCl on textural changes and protein and lipid degradation during the ripening stage of sufu, a Chinese fermented soybean food. Journal of the Science of Food and Agriculture 83 (9):899–904. doi: 10.1002/jsfa.1425.
- Handoyo, T., and N. Morita. 2006. Structural and functional properties of fermented soybean (tempeh) by using Rhizopus oligosporus. International Journal of Food Properties 9 (2):347–55. doi: 10.1080/10942910500224746.
- Hansen, E. B. 2014. Starter Cultures: Uses in the Food Industry. In Encyclopedia of Food Microbiology. (529–34). London, Amsterdam: Academic Press. doi: 10.1016/B978-0-12-384730-0.00320-7.
- Hanya, Y., and T. Nakadai. 2003. Yeasts and soy products. In Yeasts in Food (413–28). Oxford, Reino Unido: Woodhead Publishing, Elsevier.
- Harada, R., M. Yuzuki, K. Ito, K. Shiga, T. Bamba, and E. Fukusaki. 2017. Influence of yeast and lactic acid bacterium on the constituent profile of soy sauce during fermentation. Journal of Bioscience and Bioengineering 123 (2):203–8. doi: 10.1016/j.jbiosc.2016.08.010.
- Harada, R., M. Yuzuki, K. Ito, K. Shiga, T. Bamba, and E. Fukusaki. 2018. Microbe participation in aroma production during soy sauce fermentation. Journal of Bioscience and Bioengineering 125 (6):688–94. doi: 10.1016/j.jbiosc.2017.12.004.
- Hatti-Kaul, R., L. Chen, T. Dishisha, and H. E. Enshasy. 2018. Lactic acid bacteria: From starter cultures to producers of chemicals. FEMS Microbiology Letters 365 (20). doi:10.1093/femsle/fny213.
- Haug, W., and H. Lantzsch. 1983. Sensitive method for the rapid determination of phytate in cereals and cereal products. Journal of the Science of Food and Agriculture 34 (12):1423–6. doi: 10.1002/jsfa.2740341217.
- Hayashida, Y., K. Nishimura, and J. C. Slaughter. 1997. The influence of mash pre‐aging on the development of the flavour‐active compound, 4‐hydroxy‐2 (or5)‐ethyl‐5 (or2)‐methyl‐3 (2H)‐furanone (HEMF), during soy sauce fermentation. International Journal of Food Science & Technology 32 (1):11–4. doi: 10.1046/j.1365-2621.1997.00378.x.
- He, W., Z. Chen, and H. Y. Chung. 2022. Dynamic correlations between major enzymatic activities, physicochemical properties and targeted volatile compounds in naturally fermented plain sufu during production. Food Chemistry 378:131988. doi: 10.1016/j.foodchem.2021.131988.
- He, W., and H. Y. Chung. 2020. Exploring core functional microbiota related with flavor compounds involved in the fermentation of a natural fermented plain sufu (Chinese fermented soybean curd). Food Microbiology 90:103408. doi: 10.1016/j.fm.2019.103408.
- Henick-Kling, T., and Y. H. Park. 1994. Considerations for the use of yeast and bacterial starter cultures: SO2 and timing of inoculation. American Journal of Enology and Viticulture 45 (4):464–9. doi: 10.5344/ajev.1994.45.4.464.
- Ho, V. T. T., G. H. Fleet, and J. Zhao. 2018. Unravelling the contribution of lactic acid bacteria and acetic acid bacteria to cocoa fermentation using inoculated organisms. International Journal of Food Microbiology 279:43–56. doi: 10.1016/j.ijfoodmicro.2018.04.040.
- Ho, V. T. T., J. Zhao, and G. Fleet. 2015. The effect of lactic acid bacteria on cocoa bean fermentation. International Journal of Food Microbiology 205:54–67. doi: 10.1016/j.ijfoodmicro.2015.03.031.
- Ho, V., J. Zhao, and G. Fleet. 2014. Yeasts are essential for cocoa bean fermentation. International Journal of Food Microbiology 174:72–87. doi: 10.1016/j.ijfoodmicro.2013.12.014.
- Hoang, N. X., S. Ferng, C.-H. Ting, W.-H. Huang, R. Y.-Y. Chiou, and C.-K. Hsu. 2016. Optimizing the initial moromi fermentation conditions to improve the quality of soy sauce. LWT 74:242–50. doi: 10.1016/j.lwt.2016.07.049.
- Holzapfel, W. H., C. Franz, W. Ludwig, W. Back, and L. M. T. Dicks. 2006. The genera pediococcus and tetragenococcus. Prokaryotes 4:229–66. doi: 10.1007/0-387-30744-3_8.
- Hosoi, T., and K. Kiuchi. 2008. Natto–a food made by fermenting cooked soybeans with Bacillus subtilis (natto). In Handbook of fermented functional foods (227–45). CRC Press Boca Raton, FL. doi: 10.1201/9781420053289.
- Hu, Y., C. Ge, W. Yuan, R. Zhu, W. Zhang, L. Du, and J. Xue. 2010. Characterization of fermented black soybean natto inoculated with Bacillus natto during fermentation. Journal of the Science of Food and Agriculture 90 (7):1194–202. doi: 10.1002/jsfa.3947.
- Hu, Y., C. Piao, Y. Chen, Y. Zhou, D. Wang, H. Yu, and B. Xu. 2019. Soybean residue (okara) fermentation with the yeast Kluyveromyces marxianus. Food Bioscience 31:100439. doi: 10.1016/j.fbio.2019.100439.
- Huang, H., K. Kwok, and H. Liang. 2004. Effects of tea polyphenols on the activities of soybean trypsin inhibitors and trypsin. Journal of the Science of Food and Agriculture 84 (2):121–6. doi: 10.1002/jsfa.1610.
- Huang, L., C. Wang, Y. Zhang, X. Chen, Z. Huang, G. Xing, and M. Dong. 2019. Degradation of anti‐nutritional factors and reduction of immunoreactivity of tempeh by co‐fermentation with Rhizopus oligosporus RT‐3 and Actinomucor elegans DCY‐1. International Journal of Food Science & Technology 54 (5):1836–48. doi: 10.1111/ijfs.14085.
- Huang, X., S. Yu, B. Han, and J. Chen. 2018. Bacterial community succession and metabolite changes during sufu fermentation. LWT 97:537–45. doi: 10.1016/j.lwt.2018.07.041.
- Humer, E., C. Schwarz, and K. Schedle. 2015. Phytate in pig and poultry nutrition. Journal of Animal Physiology and Animal Nutrition 99 (4):605–25. doi: 10.1111/jpn.12258.
- Hwang, C. E., S. C. Kim, D. H. Kim, H. Y. Lee, H. K. Suh, K. M. Cho, and J. H. Lee. 2021. Enhancement of isoflavone aglycone, amino acid, and CLA contents in fermented soybean yogurts using different strains: Screening of antioxidant and digestive enzyme inhibition properties. Food Chemistry 340:128199. doi: 10.1016/j.foodchem.2020.128199.
- Hymowitz, T. 2008. The history of the soybean. In Soybeans (1–31). Urbana, Illinois, USA: AOCS Press, Elsevier. doi: 10.1016/B978-1-893997-64-6.50004-4.
- Ito, K., and A. Matsuyama. 2021. Koji molds for Japanese soy sauce brewing: Characteristics and key enzymes. Journal of Fungi 7 (8):658. doi: 10.3390/jof7080658.
- Jacobsen, T., and L. Hinrichsen. 1997. Bioformation of flavour by Penicillium candidum, Penicillium nalgiovense and Geotrichum candidum on glucose, peptone, maize oil and meat extract. Food Chemistry 60 (3):409–16. doi: 10.1016/S0308-8146(96)00361-5.
- Jeong, D.-W., B. Lee, J.-Y. Her, K.-G. Lee, and J.-H. Lee. 2016. Safety and technological characterization of coagulase-negative staphylococci isolates from traditional Korean fermented soybean foods for starter development. International Journal of Food Microbiology 236:9–16. doi: 10.1016/j.ijfoodmicro.2016.07.011.
- Jiang, K., B. Tang, Q. Wang, Z. Xu, L. Sun, J. Ma, S. Li, H. Xu, and P. Lei. 2019. The bio-processing of soybean dregs by solid state fermentation using a poly γ-glutamic acid producing strain and its effect as feed additive. Bioresource Technology 291:121841. doi: 10.1016/j.biortech.2019.121841.
- Johansen, E. 2017. Future access and improvement of industrial lactic acid bacteria cultures. Microbial Cell Factories 16 (1):230. doi: 10.1186/s12934-017-0851-1.
- Kaboré, D., D. S. Nielsen, H. Sawadogo-Lingani, B. Diawara, M. H. Dicko, M. Jakobsen, and L. Thorsen. 2013. Inhibition of Bacillus cereus growth by bacteriocin producing Bacillus subtilis isolated from fermented baobab seeds (maari) is substrate dependent. International Journal of Food Microbiology 162 (1):114–9. doi: 10.1016/j.ijfoodmicro.2012.12.027.
- Kanno, A., and H. Takamatsu. 1987. Changes in the Volatile Components of “Natto” during Manufacturing and Storage Studies on “Natto”, Part IV. Nippon Shokuhin Kogyo GAKKAISHI 34 (5):330–5. doi: 10.3136/nskkk1962.34.5_330.
- Karkle, E. N Lazzari, and Beleia, A. 2010. Effect of soaking and cooking on phytate concentration, minerals, and texture of food-type soybeans. Ciência e Tecnologia de Alimentos 30 (4):1056–60. doi: 10.1590/S0101-20612010000400034.
- Kasana, R. C., R. Salwan, and S. K. Yadav. 2011. Microbial proteases: Detection, production, and genetic improvement. Critical Reviews in Microbiology 37 (3):262–76. doi: 10.3109/1040841X.2011.577029.
- Kasprowicz‐Potocka, M., A. Zaworska, P. Gulewicz, P. Nowak, and A. Frankiewicz. 2018. The effect of fermentation of high alkaloid seeds of Lupinus angustifolius var. Karo by Saccharomyces cerevisieae, Kluyveromyces lactis, and Candida utilis on the chemical and microbial composition of products. Journal of Food Processing and Preservation 42 (2):e13487. doi: 10.1111/jfpp.13487.
- Kaur, S., S. Sharma, B. N. Dar, and B. Singh. 2012. Optimization of process for reduction of antinutritional factors in edible cereal brans. Food Science and Technology International 18 (5):445–54. doi: 10.1177/1082013211428236.
- Keuth, S., and B. Bisping. 1993. Formation of vitamins by pure cultures of tempe moulds and bacteria during the tempe solid substrate fermentation. Journal of Applied Bacteriology 75 (5):427–34. doi: 10.1111/j.1365-2672.1993.tb02798.x.
- Kim, J.-Y., M.-W. Jun, Y.-J. Seong, H. Park, J. Ahn, and Y.-C. Park. 2019. Direct Biotransformation of Nonanoic Acid and Its Esters to Azelaic Acid by Whole Cell Biocatalyst of Candida tropicalis. ACS Sustainable Chemistry & Engineering 7 (21):17958–66. doi: 10.1021/acssuschemeng.9b04723.
- Kim, J.-Y., S.-H. Yeo, S.-Y. Baek, and H.-S. Choi. 2011. Molecular and morphological identification of fungal species isolated from bealmijang meju. Journal of Microbiology and Biotechnology 21 (12):1270–9. doi: 10.4014/jmb.1105.05013.
- Kitamoto, K. 2002. Molecular biology of the Koji molds. Advances in Applied Microbiology 51:129–53. doi: 10.1016/S0065-2164(02)51004-2.
- Kłosowski, G., D. Mikulski, and K. Pielech-Przybylska. 2021. Pyrazines Biosynthesis by Bacillus Strains Isolated from Natto Fermented Soybean. Biomolecules 11 (11):1736. doi: 10.3390/biom11111736.
- Koutinas, A. A., H. Papapostolou, D. Dimitrellou, N. Kopsahelis, E. Katechaki, A. Bekatorou, and L. A. Bosnea. 2009. Whey valorisation: A complete and novel technology development for dairy industry starter culture production. Bioresource Technology 100 (15):3734–9. doi: 10.1016/j.biortech.2009.01.058.
- Krishna, C. 2005. Solid-state fermentation systems—an overview. Critical Reviews in Biotechnology 25 (1-2):1–30. doi: 10.1080/07388550590925383.
- Kronenberg, H. J., and Y. D. Hang. 1984. Biochemical changes in Okara during metauza fermentation
- Kubo, Y., A. P. Rooney, Y. Tsukakoshi, R. Nakagawa, H. Hasegawa, and K. Kimura. 2011. Phylogenetic analysis of Bacillus subtilis strains applicable to natto (fermented soybean) production. Applied and Environmental Microbiology 77 (18):6463–9. doi: 10.1128/AEM.00448-11.
- Kuklinsky‐Sobral, J., W. L. Araújo, R. Mendes, I. O. Geraldi, A. A. Pizzirani‐Kleiner, and J. L. Azevedo. 2004. Isolation and characterization of soybean‐associated bacteria and their potential for plant growth promotion. Environmental Microbiology 6 (12):1244–51. doi: 10.1111/j.1462-2920.2004.00658.x.
- Kumar, A., C. Sahu, P. A. Panda, M. Biswal, R. P. Sah, M. K. Lal, M. J. Baig, P. Swain, L. Behera, K. Chattopadhyay, et al. 2020. Phytic acid content may affect starch digestibility and glycemic index value of rice (Oryza sativa L.). Journal of the Science of Food and Agriculture 100 (4):1598–607. doi: 10.1111/j.1365-2621.1985.tb13325.x.
- Kumar, V., A. Rani, V. Pandey, and G. S. Chauhan. 2006. Changes in lipoxygenase isozymes and trypsin inhibitor activity in soybean during germination at different temperatures. Food Chemistry 99 (3):563–8. doi: 10.1016/j.foodchem.2005.08.024.
- Kunitz, M. 1947. Crystalline soybean trypsin inhibitor: II. General properties. Journal of General Physiology 30 (4):291–310. doi: 10.1085/jgp.30.4.291.
- Kustyawati, M. E. 2017. April 2017), 24(2. Profile of aroma compounds and acceptability of modified tempeh. International Food Research Journal 24 (2):734–40. 734–740. doi: 10.1155/2021/6676042.
- Kustyawati, M. E., F. Pratama, D. Saputra, and A. Wijaya. 2018. 2018. Viability of molds and bacteria in tempeh processed with supercritical carbon dioxides during storage. International Journal of Food Science 2018:1–7. doi: 10.1155/2018/8591015.
- Kusumah, D., M. Wakui, M. Murakami, X. Xie, K. Yukihito, and I. Maeda. 2020. Linoleic acid, α-linolenic acid, and monolinolenins as antibacterial substances in the heat-processed soybean fermented with Rhizopus oligosporus. Bioscience, Biotechnology, and Biochemistry 84 (6):1285–90. doi: 10.1080/09168451.2020.1731299.
- Kusumoto, K.-I, and A. K. Rai. 2017. Miso, the traditional fermented soybean paste of Japan. In Fermented Foods (122–34). Boca Raton: CRC Press.
- Kusumoto, K.-I., Y. Yamagata, R. Tazawa, M. Kitagawa, T. Kato, K. Isobe, and Y. Kashiwagi. 2021. Japanese traditional Miso and Koji making. Journal of Fungi 7 (7):579. doi: 10.3390/jof7070579.
- Kwon, D.-J., and W.-J. Kim. 2005. Isolation of higher alcohol-producing yeast as the flavor components and determination of optimal culture conditions. Food Science and Biotechnology 14 (5):576–80. https://koreascience.kr/article/JAKO200509905833821.pdf.
- Lee, C.-H, and S. S. Lee, D. K. B. T.-A. M. 2002. Cereal fermentation by fungi. In G. G. Khachatourians & and B. Arora (Eds.), Agriculture and Food Production (Vol. 2, 151–70). London: Elsevier. doi: 10.1016/S1874-5334(02)80009-0.
- Lee, S., S. Lee, D. Singh, J. Y. Oh, E. J. Jeon, H. S. Ryu, D. W. Lee, B. S. Kim, and C. H. Lee. 2017. Comparative evaluation of microbial diversity and metabolite profiles in doenjang, a fermented soybean paste, during the two different industrial manufacturing processes. Food Chemistry 221:1578–86. doi: 10.1016/j.foodchem.2016.10.135.
- Lee, Y.-C., H.-F. Kung, Y.-L. Huang, C.-H. Wu, Y.-R. Huang, and Y.-H. Tsai. 2016. Reduction of biogenic amines during miso fermentation by Lactobacillus plantarum as a starter culture. Journal of Food Protection 79 (9):1556–61. doi: 10.4315/0362-028X.JFP-16-060.
- Leejeerajumnean, A., S. C. Duckham, J. D. Owens, and J. M. Ames. 2001. Volatile compounds in Bacillus‐fermented soybeans. Journal of the Science of Food and Agriculture 81 (5):525–9. doi: 10.1002/jsfa.843.
- Li, H., H. Zhou, J. Zhang, X. Fu, Z. Ying, and X. Liu. 2021. Proteinaceous α-amylase inhibitors: Purification, detection methods, types and mechanisms. International Journal of Food Properties 24 (1):277–90. doi: 10.1080/10942912.2021.1876087.
- Li, J., R. Zhou, Z. Ren, Y. Fan, S. Hu, C. Zhuo, and Z. Deng. 2019. Improvement of protein quality and degradation of allergen in soybean meal fermented by Neurospora crassa. LWT 101:220–8. doi: 10.1016/j.lwt.2018.10.089.
- Li, K., J. Tang, Z. Zhang, Z. Wu, A. Zhong, Z. Li, and Y. Wang. 2022. Correlation between flavor compounds and microorganisms of Chaling natural fermented red sufu. LWT 154:112873. doi: 10.1016/j.lwt.2021.112873.
- Li, L., Z.-Y. Yang, X.-Q. Yang, G.-H. Zhang, S.-Z. Tang, and F. Chen. 2008. Debittering effect of Actinomucor elegans peptidases on soybean protein hydrolysates. Journal of Industrial Microbiology & Biotechnology 35 (1):41–7. doi: 10.1007/s10295-007-0264-y.
- Li, S., Z. Jin, D. Hu, W. Yang, Y. Yan, X. Nie, J. Lin, Q. Zhang, D. Gai, Y. Ji, et al. 2020. Effect of solid-state fermentation with Lactobacillus casei on the nutritional value, isoflavones, phenolic acids and antioxidant activity of whole soybean flour. LWT 125:109264. doi: 10.1016/j.lwt.2020.109264.
- Li, Z., J. Rui, X. Li, J. Li, L. Dong, Q. Huang, C. Huang, Z. Wang, L. Li, P. Xuan, et al. 2017. Bacterial community succession and metabolite changes during doubanjiang-meju fermentation, a Chinese traditional fermented broad bean (Vicia faba L.) paste. Food Chemistry 218:534–42. doi: 10.1016/j.foodchem.2016.09.104.
- Liu, C., F. Gong, X. Li, H. Li, Z. Zhang, Y. Feng, and H. Nagano. 2012. Natural populations of lactic acid bacteria in douchi from Yunnan Province, China. Journal of Zhejiang University SCIENCE B 13 (4):298–306. doi: 10.1631/jzus.B1100221.
- Liu, J., J. Chen, s Li, w Tian, H. Wu, and B. Han. 2021. Comparison of volatile and non-volatile metabolites in sufu produced with bacillus licheniformis by rapid fermentation. International Journal of Food Properties 24 (1):553–63. doi: 10.1080/10942912.2021.1901733.
- Liu, K. 2019. Soybean trypsin inhibitor assay: Further improvement of the standard method approved and reapproved by American Oil Chemists’ Society and American Association of Cereal Chemists International. Journal of the American Oil Chemists’ Society 96 (6):635–45. doi: 10.1002/aocs.12205.
- Liu, L., X. Chen, L. Hao, G. Zhang, Z. Jin, C. Li, Y. Yang, J. Rao, and B. Chen. 2022. Traditional fermented soybean products: Processing, flavor formation, nutritional and biological activities. Critical Reviews in Food Science and Nutrition 62 (7):1971–89. doi: 10.1080/10408398.2020.1848792.
- Liu, X., J. Liang, Y. Ma, J. Sun, Y. Liu, X. Gu, and Y. Wang. 2022. The impact of protein hydrolysis on biogenic amines production during sufu fermentation. Food Control 140, 109105. doi: 10.1016/j.foodcont.2022.109105.
- Liu, Y., H. Song, and H. Luo. 2018. Correlation between the key aroma compounds and gDNA copies of Bacillus during fermentation and maturation of natto. Food Research International 112:175–83. doi: 10.1016/j.foodres.2018.06.033.
- Liu, Z., X. Guan, X. Zhong, X. Zhou, and F. Yang. 2021. Bacillus velezensis DP‐2 isolated from Douchi and its application in soybean meal fermentation. Journal of the Science of Food and Agriculture 101 (5):1861–8. doi: 10.1002/jsfa.10801.
- Lleixà, J., M. Manzano, A. Mas, M. Portillo, and C. del. 2016. Saccharomyces and non-Saccharomyces Competition during Microvinification under Different Sugar and Nitrogen Conditions. Frontiers in Microbiology, 7. doi: 10.3389/fmicb.2016.01959.
- Lockwood, L. B., and A. K. Smith. 1950. Fermented soy foods and sauce. Yearbook of Agriculture 51:357–61. https://naldc.nal.usda.gov/download/IND43894083/PDF.
- Lu, W., X. Zhao, Z. Xu, N. Dong, S. Zou, X. Shen, and J. Huang. 2013. Development of a new colorimetric assay for lipoxygenase activity. Analytical Biochemistry 441 (2):162–8. doi: 10.1016/j.ab.2013.06.007.
- Luh, B. S. 1995. Industrial production of soy sauce. Journal of Industrial Microbiology 14 (6):467–71. doi: 10.1007/BF01573959.
- Marazza, J. A., M. A. Nazareno, G. S. de Giori, and M. S. Garro. 2012. Enhancement of the antioxidant capacity of soymilk by fermentation with Lactobacillus rhamnosus. Journal of Functional Foods 4 (3):594–601. doi: 10.1016/j.jff.2012.03.005.
- Matsumoto, T., and R. Tomoyasu. 1925. Studies on purple speck of soybean seed. Japanese Journal of Phytopathology 1 (6):1–14_1. _1. doi: 10.3186/jjphytopath.1.6_1.
- Matsushita, M., S. Tada, S. Suzuki, K.-I. Kusumoto, and Y. Kashiwagi. 2009. Deletion analysis of the promoter of Aspergillus oryzae gene encoding heat shock protein 30. Journal of Bioscience and Bioengineering 107 (4):345–51. doi: 10.1016/j.jbiosc.2008.11.024.
- McCue, P., Y.-T. Lin, R. G. Labbe, and K. Shetty. 2004. Sprouting and solid-state bioprocessing by Rhizopus oligosporus increase the in vitro antibacterial activity of aqueous soybean extracts against Helicobacter pylori. Food Biotechnology 18 (2):229–49. doi: 10.1081/FBT-200025669.
- Medeiros, S., J. Xie, P. W. Dyce, H. Y. Cai, K. DeLange, H. Zhang, and J. Li. 2018. Isolation of bacteria from fermented food and grass carp intestine and their efficiencies in improving nutrient value of soybean meal in solid state fermentation. Journal of Animal Science and Biotechnology 9 (1):29. doi: 10.1186/s40104-018-0245-1.
- Meerak, J., P. Yukphan, M. Miyashita, H. Sato, Y. Nakagawa, and Y. Tahara. 2008. Phylogeny of γ-polyglutamic acid-producing Bacillus strains isolated from a fermented locust bean product manufactured in West Africa. The Journal of General and Applied Microbiology 54 (3):159–66. doi: 10.2323/jgam.54.159.
- Mei Feng, X., T. Ostenfeld Larsen, and J. Schnürer. 2007. Production of volatile compounds by Rhizopus oligosporus during soybean and barley tempeh fermentation. International Journal of Food Microbiology 113 (2):133–41. doi: 10.1016/j.ijfoodmicro.2006.06.025.
- Miller, W. A., and K. W. Roy. 1982. Mycoflora of soybean leaves, pods, and seeds in Mississippi. Canadian Journal of Botany 60 (12):2716–23. doi: 10.1139/b82-331.
- Mo, A.-Y., B. Kwon, S. Kamala-Kannan, K.-J. Lee, B.-T. Oh, D.-H. Kim, M.-S. Yang, J.-H. Kim, and S.-M. Park. 2010. Isolation and characterization of Bacillus polyfermenticus isolated from Meju, Korean soybean fermentation starter. World Journal of Microbiology and Biotechnology 26 (6):1099–105. doi: 10.1007/s11274-009-0276-z.
- Molnárová, J.,R. Vadkertiová,and E. Stratilová. 2013. Extracellular enzymatic activities and physiological profiles of yeasts colonizing fruit trees. Journal of Basic Microbiology 54 (S1):S74–84. doi:10.1002/jobm.201300072
- Motlhanka, K., K. Lebani, M. Garcia-Aloy, and N. Zhou. 2022. Functional characterization of khadi yeasts isolates for selection of starter cultures. Journal of Microbiology and Biotechnology 32 (3):307–16. doi: 10.4014/jmb.2109.09003.
- Moy, Y.-S., T.-J. Lu, and C.-C. Chou. 2012. Volatile components of the enzyme-ripened sufu, a Chinese traditional fermented product of soy bean. Journal of Bioscience and Bioengineering 113 (2):196–201. doi: 10.1016/j.jbiosc.2011.09.021.
- Mukherjee, R., R. Chakraborty, and A. Dutta. 2015. Role of fermentation in improving nutritional quality of soybean meal—a review. Asian-Australasian Journal of Animal Sciences 29 (11):1523–9. doi: 10.5713/ajas.15.0627.
- Mulyowidarso, R. K. 1991. The microbiology and biochemistry of soybean soaking for tempe fermentation. [ University of New South Wales (Australia)]. doi: 10.1016/0168-1605(89)90078-0.
- N’Dir, B., C. Hbid, C. Cornélius, D. Roblain, P. Jacques, F. Vanhentenryck, M. Diop, and P. Thonart. 1994. Propriétés antifongiques de la microflore sporulée du” nététu. Cahiers Agricultures 3 (1):23–30.
- Natarajan, S., F. Khan, Q. Song, S. Lakshman, P. Cregan, R. Scott, E. Shipe, and W. Garrett. 2016. Characterization of soybean storage and allergen proteins affected by environmental and genetic factors. Journal of Agricultural and Food Chemistry 64 (6):1433–45. doi: 10.1021/acs.jafc.5b05172.
- Nguyen, T. T. T., G. Loiseau, C. Icard-Vernière, I. Rochette, S. Trèche, and J.-P. Guyot. 2007. Effect of fermentation by amylolytic lactic acid bacteria, in process combinations, on characteristics of rice/soybean slurries: A new method for preparing high energy density complementary foods for young children. Food Chemistry 100 (2):623–31. doi: 10.1016/j.foodchem.2005.09.080.
- Nkhata, S. G., E. Ayua, E. H. Kamau, and J. Shingiro. 2018. Fermentation and germination improve nutritional value of cereals and legumes through activation of endogenous enzymes. Food Science & Nutrition 6 (8):2446–58. doi: 10.1002/fsn3.846.
- Norozi, M., M. Rezaei, and M. Kazemifard. 2022. Effect of different acid processing methodologies on the nutritional value and reduction of anti‐nutrients in soybean meal. Journal of Food Processing and Preservation 46 (1):e16205. doi: 10.1111/jfpp.16205.
- Nout, M. J., and K. E. Aidoo. 2011. Asian fungal fermented food. In Industrial applications (29–58). New York, NY: Springer. doi: 10.1007/978-3-642-11458-8_2.
- Nout, M. J. R., G. Beernink, and T. M. G. Bonants-van Laarhoven. 1987. Growth of Bacillus cereus in soyabean tempeh. International Journal of Food Microbiology 4 (4):293–301. doi: 10.1016/0168-1605(87)90004-3.
- Nout, M. J. R., M. A. De Dreu, A. M. Zuurbier, and T. M. G. Bonants-Van Laarhoven. 1987. Ecology of controlled soyabean acidification for tempe manufacture. Food Microbiology 4 (2):165–72. doi: 10.1016/0740-0020(87)90032-3.
- Nout, M. J. R., P. K. Sarkar, and L. R. Beuchat. 2007. Indigenous fermented foods. In Food microbiology: fundamentals and frontiers, 3rd edition (817–35). Washington: ASM Press.
- Nurdini, A. L., L. Nuraida, and A. Suwanto. 2015. Microbial growth dynamics during tempe fermentation in two different home industries. International Food Research Journal 22 (4):1668. doi: 10.1016/j.ijgfs.2021.100413.
- Ogasawara, M., Y. Yamada, and M. Egi. 2006. Taste enhancer from the long-term ripening of miso (soybean paste). Food Chemistry 99 (4):736–41. doi: 10.1016/j.foodchem.2005.08.051.
- Ohata, M., K. Kohama, Y. Morimitsu, K. Kubota, and E. Sugawara. 2007. The formation mechanism by yeast of 4-hydroxy-2 (or 5)-ethyl-5 (or 2)-methyl-3 (2H)-furanone in miso. Bioscience, Biotechnology, and Biochemistry 71 (2):407–13. doi: 10.1271/bbb.60466.
- Ohnishi, K. 1994. Practical application of new-type halophilic lactic acid bacteria for Miso-manufacturing. Nippon Jyozo Kyokaishi 89:269–75. doi: 10.6013/jbrewsocjapan1988.89.269.
- Olukomaiya, O. O., O. Q. Adiamo, W. C. Fernando, R. Mereddy, X. Li, and Y. Sultanbawa. 2020. Effect of solid-state fermentation on proximate composition, anti-nutritional factor, microbiological and functional properties of lupin flour. Food Chemistry 315:126238. doi: 10.1016/j.foodchem.2020.126238.
- Onda, T., F. Yanagida, M. Tsuji, T. Shinohara, and K. Yokotsuka. 2003. Production and purification of a bacteriocin peptide produced by Lactococcus sp. strain GM005, isolated from Miso-paste. International Journal of Food Microbiology 87 (1-2):153–9. doi: 10.1016/S0168-1605(03)00063-1.
- Onda, T., F. Yanagida, T. Uchimura, M. Tsuji, S. Ogino, T. Shinohara, and K. Yokotsuka. 2003. Analysis of lactic acid bacterial flora during miso fermentation. Food Science and Technology Research 9 (1):17–24. doi: 10.3136/fstr.9.17.
- Ouoba, L. I. I., M. D. Cantor, B. Diawara, A. S. Traore, and M. Jakobsen. 2003. Degradation of African locust bean oil by Bacillus subtilis and Bacillus pumilus isolated from soumbala, a fermented African locust bean condiment. Journal of Applied Microbiology 95 (4):868–73. doi: 10.1046/j.1365-2672.2003.02063.x.
- Ouoba, L. I. I., B. Diawara, N. T. Annan, L. Poll, and M. Jakobsen. 2005. Volatile compounds of Soumbala, a fermented African locust bean (Parkia biglobosa) food condiment. Journal of Applied Microbiology 99 (6):1413–21. doi: 10.1111/j.1365-2672.2005.02722.x.
- Owens, J. D., N. Allagheny, G. Kipping, and J. M. Ames. 1997. Formation of volatile compounds during Bacillus subtilis fermentation of soya beans. Journal of the Science of Food and Agriculture 74 (1):132–40. doi: 10.1002/(SICI)1097-0010(199705)74:1 < 132::AID-JSFA779 > 3.0.CO;2-8.
- Owens, J. D., M. Astuti, and K. R. Kuswanto. 2015. Tempe and related products. In Indigenous fermented foods of southeast Asia (1–108. CRC Press Taylor and Francis Group FL, USA.
- Pandey, A. 2003. Solid-state fermentation. Biochemical Engineering Journal 13 (2-3):81–4. doi: 10.1016/S1369-703X(02)00121-3.
- Papagianni, M., and M. Mattey. 2006. Morphological development of Aspergillus niger in submerged citric acid fermentation as a function of the spore inoculum level. Application of Neural Network and Cluster Analysis for Characterization of Mycelial Morphology. Microbial Cell Factories 5 (1):3. doi: 10.1186/1475-2859-5-3.
- Paredes-Lopez, O., G. I. Harry, and R. Montes-Rivera. 1987. Development of a fermentation procedure to produce a tempe-related food using common beans as substrate. Biotechnology Letters 9 (5):333–8. doi: 10.1007/BF01025799.
- Park, M. K., and Y.-S. Kim. 2020. Comparative metabolic expressions of fermented soybeans according to different microbial starters. Food Chemistry 305:125461. doi: 10.1016/j.foodchem.2019.125461.
- Parkouda, C., D. S. Nielsen, P. Azokpota, L. Ivette Irène Ouoba, W. K. Amoa-Awua, L. Thorsen, J. D. Hounhouigan, J. S. Jensen, K. Tano-Debrah, B. Diawara, et al. 2009. The microbiology of alkaline-fermentation of indigenous seeds used as food condiments in Africa and Asia. Critical Reviews in Microbiology 35 (2):139–56. doi: 10.1080/10408410902793056.
- Pereira, G. V., M. de, M. G. Miguel, C. P. da, C. L. Ramos, and R. F. Schwan. 2012. Microbiological and physicochemical characterization of small-scale cocoa fermentations and screening of yeast and bacterial strains to develop a defined starter culture. Applied and Environmental Microbiology 78 (15):5395–405. doi: 10.1128/AEM.01144-12.
- Perkins, E. G, D. R. B. T.-P. H. of S. P. 1995. Chapter 2 - Composition of Soybeans and Soybean Products (and U. Erickson (ed.); 9–28. New York, NY: AOCS Press. doi: 10.1016/B978-0-935315-63-9.50006-1.
- Qin, P., T. Wang, and Y. Luo. 2022. A review on plant-based proteins from soybean: Health benefits and soy product development. Journal of Agriculture and Food Research 7:100265. doi: 10.1016/j.jafr.2021.100265.
- Rashad, M. M., A. E. Mahmoud, H. M. Abdou, and M. U. Nooman. 2011. Improvement of nutritional quality and antioxidant activities of yeast fermented soybean curd residue. African Journal of Biotechnology 10 (28):5504–13. doi: 10.5897/AJB10.1658.
- Reddy, N. R., and M. D. Pierson. 1994. Reduction in antinutritional and toxic components in plant foods by fermentation. Food Research International 27 (3):281–90. doi: 10.1016/0963-9969(94)90096-5.
- Reddy, N. R., M. D. Pierson, and D. K. Salunkhe. 2009. Legume-based fermented foods: their preparation and nutritional quality. Boca Raton: CRC Press. doi: 10.1080/10408398209527353.
- Rehman, S., J. A. Awan, F. M. Anjum, and M. Randhawa. 2014. Antinutrients and Toxicity in Plant‐based Foods: Cereals and Pulses. Practical Food Safety: Contemporary Issues and Future Directions :311–39. doi: 10.1002/9781118474563.ch16.
- Rehms, H., and W. Barz. 1995. Degradation of stachyose, raffinose, melibiose and sucrose by different tempe-producing Rhizopus fungi. Applied Microbiology and Biotechnology 44 (1-2):47–52. doi: 10.1007/BF00164479.
- Rizal S., M. E. Kustyawati, and U. Hasanudin. 2021. The growth of yeast and fungi, the formation of β-glucan, and the antibacterial activities during soybean fermentation in producing tempeh. International Journal of Food Science 2021:6676042. doi: 10.1155/2021/6676042.
- Romulo, A., and R. Surya. 2021. Tempe: A traditional fermented food of Indonesia and its health benefits. International Journal of Gastronomy and Food Science 26:100413. doi: 10.1016/j.ijgfs.2021.100413.
- Rouhana, A., J. Adler‐Nissen, U. R. I. Cogan, and H. Frøkiær. 1996. Heat inactivation kinetics of trypsin inhibitors during high temperature‐short time processing of soymilk. Journal of Food Science 61 (2):265–9. doi: 10.1111/j.1365-2621.1996.tb14173.x.
- Ruiz‐Terán, F., and J. David Owens. 1996. Chemical and enzymic changes during the fermentation of bacteria‐free soya bean tempe. Journal of the Science of Food and Agriculture 71 (4):523–30. doi: 10.1002/(SICI)1097-0010(199608)71:4 < 523::AID-JSFA613 > 3.0.CO;2-R.
- Russo, P., G. Spano, and V. Capozzi. 2017. Safety evaluation of starter cultures. Starter Cultures in Food Production :101–28. doi: 10.1002/9781118933794.ch6.
- Saharan, P., P. K. Sadh, and J. S. Duhan. 2017. Comparative assessment of effect of fermentation on phenolics, flavanoids and free radical scavenging activity of commonly used cereals. Biocatalysis and Agricultural Biotechnology 12:236–40. doi: 10.1016/j.bcab.2017.10.013.
- Salvagiotti, F., K. G. Cassman, J. E. Specht, D. T. Walters, A. Weiss, and A. Dobermann. 2008. Nitrogen uptake, fixation and response to fertilizer N in soybeans: A review. Field Crops Research 108 (1):1–13. doi: 10.1016/j.fcr.2008.03.001.
- Sanjukta, S., and A. K. Rai. 2016. Production of bioactive peptides during soybean fermentation and their potential health benefits. Trends in Food Science & Technology 50:1–10. doi: 10.1016/j.tifs.2016.01.010.
- Sarkar, P. K., P. E. Cook, and J. D. Owens. 1993. Bacillus fermentation of soybeans. World Journal of Microbiology & Biotechnology 9 (3):295–9. doi: 10.1007/BF00383066.
- Sarkar, P. K., and M. J. R. Nout. 2014. Handbook of indigenous foods involving alkaline fermentation. CRC Press. https://books.google.com.sg/books?id=PVfSBQAAQBAJ&lpg=PP1&pg=PR7#v=onepage&q&f=false
- Sarkar, P. K., and J. P. Tamang. 1994. The influence of process variables and inoculum composition on the sensory quality of kinema. Food Microbiology 11 (4):317–25. doi: 10.1006/fmic.1994.1036.
- Sarkar, P. K., and J. P. Tamang. 1995. Changes in the microbial profile and proximate composition during natural and controlled fermentations of soybeans to produce kinema. Food Microbiology 12:317–25. doi: 10.1016/S0740-0020(95)80112-X.
- Sarkar, P. K., J. P. Tamang, P. E. Cook, and J. D. Owens. 1994. Kinema—a traditional soybean fermented food: Proximate composition and microflora. Food Microbiology 11 (1):47–55. doi: 10.1006/fmic.1994.1007.
- Schwan, R. F., and A. E. Wheals. 2004. The microbiology of cocoa fermentation and its role in chocolate quality. Critical Reviews in Food Science and Nutrition 44 (4):205–21. doi: 10.1080/10408690490464104.
- Sessa, D. J., J. K. Haney, and T. C. Nelsen. 1990. Inactivation of soybean trypsin inhibitors with ascorbic acid plus copper. Journal of Agricultural and Food Chemistry 38 (7):1469–74. doi: 10.1021/jf00097a008.
- Shahidi, F., and W. Y. Oh. 2020. Lipid-derived flavor and off-flavor of traditional and functional foods: An overview. Journal of Food Bioactives (10). doi: 10.31665/JFB.2020.10224.
- Sharma, A., S. Kumari, P. Wongputtisin, M. J. R. Nout, and P. K. Sarkar. 2015. Optimization of soybean processing into kinema, a Bacillus‐fermented alkaline food, with respect to a minimum level of antinutrients. Journal of Applied Microbiology 119 (1):162–76. doi: 10.1111/jam.12826.
- Sharma, R., P. Garg, P. Kumar, S. K. Bhatia, and S. Kulshrestha. 2020. Microbial fermentation and its role in quality improvement of fermented foods. Fermentation 6 (4):106. doi: 10.3390/fermentation6040106.
- Shimakawa, Y., S. Matsubara, N. Yuki, M. Ikeda, and F. Ishikawa. 2003. Evaluation of Bifidobacterium breve strain Yakult-fermented soymilk as a probiotic food. International Journal of Food Microbiology 81 (2):131–6. doi: 10.1016/S0168-1605(02)00224-6.
- Shin, D., and D. Jeong. 2015. Korean traditional fermented soybean products: Jang. Journal of Ethnic Foods 2 (1):2–7. doi: 10.1016/j.jef.2015.02.002.
- Shirai, K., S. Revah‐Moiseev, M. García‐Garibay, and V. M. Marshall. 1994. Ability of some strains of lactic acid bacteria to degrade phytic acid. Letters in Applied Microbiology 19 (5):366–9. doi: 10.1111/j.1472-765X.1994.tb00477.x.
- Shrestha, A. K., N. R. Dahal, and V. Ndungutse. 2013. Bacillus fermentation of soybean: A review. Journal of Food Science and Technology Nepal 6:1–9. doi: 10.3126/jfstn.v6i0.8252.
- Shrestha, A. K., and A. Noomhorm. 2001. Composition and functional properties of fermented soybean flour (kinema). Journal of Food Science and Technology-Mysore 38 (5):467–70.
- Singhania, R. R. 2011. Production of celluloytic enzymes for the hydrolysis of lignocellulosic biomass. In Biofuels (177–201). San Diego, United States of America: Elsevier. doi: 10.1016/B978-0-12-385099-7.00008-5.
- Singracha, P., N. Niamsiri, W. Visessanguan, S. Lertsiri, and A. Assavanig. 2017. Application of lactic acid bacteria and yeasts as starter cultures for reduced-salt soy sauce (moromi) fermentation. LWT 78:181–8. doi: 10.1016/j.lwt.2016.12.019.
- Sirilun, S., B. S. Sivamaruthi, P. Kesika, S. Peerajan, and C. Chaiyasut. 2017. Lactic acid bacteria mediated fermented soybean as a potent nutraceutical candidate. Asian Pacific Journal of Tropical Biomedicine 7 (10):930–6. doi: 10.1016/j.apjtb.2017.09.007.
- Skoglund, E., N.-G. Carlsson, and A.-S. Sandberg. 1998. High-performance chromatographic separation of inositol phosphate isomers on strong anion exchange columns. Journal of Agricultural and Food Chemistry 46 (5):1877–82. doi: 10.1021/jf9709257.
- Song, Y., D. Jeong, and S. Baik. 2015. Monitoring of yeast communities and volatile flavor changes during traditional Korean soy sauce fermentation. Journal of Food Science 80 (9):M2005–M2014. doi: 10.1111/1750-3841.12995.
- Sørensen, L. M., K. Gori, M. A. Petersen, L. Jespersen, and N. Arneborg. 2011. Flavour compound production by Yarrowia lipolytica, Saccharomyces cerevisiae and Debaryomyces hansenii in a cheese-surface model. International Dairy Journal 21 (12):970–8. doi: 10.1016/j.idairyj.2011.06.005.
- Soubeyrand, V., A. Julien, and J.-M. Sablayrolles. 2006. Rehydration protocols for active dry wine yeasts and the search for early indicators of yeast activity. American Journal of Enology and Viticulture 57 (4):474–80. doi: 10.5344/ajev.2006.57.4.474.
- Sourabh, A., A. K. Rai, A. Chauhan, K. Jeyaram, M. Taweechotipatr, P. S. Panesar, R. Sharma, R. Panesar, S. S. Kanwar, and S. Walia. 2015. Health related issues and indigenous fermented products. In Indigenous fermented foods of South Asia (Vol. 7). CRC Press, London.
- Sparringa, R. A., and J. D. Owens. 1999. Causes of alkalinization in tempe solid substrate fermentation. Enzyme and Microbial Technology 25 (8-9):677–81. doi: 10.1016/S0141-0229(99)00097-6.
- Srianta I., E. Zubaidah, T. Estiasih, M. Yamada, and Harijono. 2016. Comparison of Monascus purpureus growth, pigment production and composition on different cereal substrates with solid state fermentation. Biocatalysis and Agricultural Biotechnology 7:181–6. doi: 10.1016/j.bcab.2016.05.011.
- Steinkraus, K. H., J. P. VanBuren, L. R. Hackler, and D. B. Hand. 1965. A pilot-plant process for production of dehydrated tempeh. Food Technology 19 (1):63. +.
- Sudarmadji, S., and P. Markakis. 1978. Lipid and other changes occurring during the fermentation and frying of tempeh. Food Chemistry 3 (3):165–70. doi: 10.1016/0308-8146(78)90015-8.
- Sun, C. Y., D. H. Li, and L. J. Sun. 2009. The function of enzymes in naturally fermented soybean paste and soybean sauce products. China Food Addit 3:164–9.
- Sun, X., G. Lyu, Y. Luan, H. Yang, and Z. Zhao. 2019. Metabolomic study of the soybean pastes fermented by the single species Penicillium glabrum GQ1-3 and Aspergillus oryzae HGPA20. Food Chemistry 295:622–9. doi: 10.1016/j.foodchem.2019.05.162.
- Suprayogi, W. P. S., A. Ratriyanto, N. Akhirini, R. F. Hadi, W. Setyono, and A. Irawan. 2022. Changes in nutritional and antinutritional aspects of soybean meals by mechanical and solid-state fermentation treatments with Bacillus subtilis and Aspergillus oryzae. Bioresource Technology Reports 17:100925. doi: 10.1016/j.biteb.2021.100925.
- Surrey, K. 1964. Spectrophotometric method for determination of lipoxidase activity. Plant Physiology 39 (1):65–70. doi: 10.1104/pp.39.1.65.
- Suwanto, A. 2021. Dynamics of microbial community during tempeh fermentation. BIOTROPIA-The Southeast Asian Journal of Tropical Biology, 28 (1) doi: 10.11598/btb.0.0.0.820.
- Tamang, J. P. 2003. Native microorganisms in the fermentation of kinema. Indian Journal of Microbiology 43 (2)June 2003), :127–30.
- Thirunathan, P., and A. Manickavasagan. 2019. Processing methods for reducing alpha-galactosides in pulses. Critical Reviews in Food Science and Nutrition 59 (20):3334–48. doi: 10.1080/10408398.2018.1490886.
- Thomson, C. A., P. J. Delaquis, and G. Mazza. 1999. Detection and measurement of microbial lipase activity: A review. Critical Reviews in Food Science and Nutrition 39 (2):165–87. doi: 10.1080/10408399908500492.
- Thumu, S. C. R., and P. M. Halami. 2020. In vivo safety assessment of Lactobacillus fermentum strains, evaluation of their cholesterol‐lowering ability and intestinal microbial modulation. Journal of the Science of Food and Agriculture 100 (2):705–13. doi: 10.1002/jsfa.10071.
- Tuitemwong, P., L. E. Erickson, D. Y. C. Fung, C. S. Setser, and S. K. Perng. 1993. Sensory analysis of soy yogurt and frozen soy yogurt produced from rapid hydration hydrothermal cooked soy milk. Journal of Food Quality 16 (3):223–39. doi: 10.1111/j.1745-4557.1993.tb00108.x.
- Tunçel, G., and D. Göktan. 1990. Effect of different methods of soaking soya beans on the growth of Bacillus cereus, Klebsiella pneumoniae and Staphylococcus aureus in tempeh. Journal of the Science of Food and Agriculture 53 (3):287–96. doi: 10.1002/jsfa.2740530302.
- Uyar, F., and Z. Baysal. 2004. Production and optimization of process parameters for alkaline protease production by a newly isolated Bacillus sp. under solid state fermentation. Process Biochemistry 39 (12):1893–8. doi: 10.1016/j.procbio.2003.09.016.
- Vagadia, B. H., S. K. Vanga, and V. Raghavan. 2017. Inactivation methods of soybean trypsin inhibitor–A review. Trends in Food Science & Technology 64:115–25. doi: 10.1016/j.tifs.2017.02.003.
- Villacrés, E., M. B. Quelal, E. Fernández, G. Garcìa, G. Cueva, and C. M. Rosell. 2020. Impact of debittering and fermentation processes on the antinutritional and antioxidant compounds in Lupinus mutabilis sweet. LWT 131:109745. doi: 10.1016/j.lwt.2020.109745.
- Vinícius de Melo Pereira, G., V. T. Soccol, S. K. Brar, E. Neto, and C. R. Soccol. 2017. Microbial ecology and starter culture technology in coffee processing. Critical Reviews in Food Science and Nutrition 57 (13):2775–88. doi: 10.1080/10408398.2015.1067759.
- Vong, W. C., and S.-Q. Liu. 2018. Bioconversion of green volatiles in okara (soybean residue) into esters by coupling enzyme catalysis and yeast (Lindnera saturnus) fermentation. Applied Microbiology and Biotechnology 102 (23):10017–26. doi: 10.1007/s00253-018-9396-4.
- Wah, T. T., S. Walaisri, A. Assavanig, N. Niamsiri, and S. Lertsiri. 2013. Co-culturing of Pichia guilliermondii enhanced volatile flavor compound formation by Zygosaccharomyces rouxii in the model system of Thai soy sauce fermentation. International Journal of Food Microbiology 160 (3):282–9. doi: 10.1016/j.ijfoodmicro.2012.10.022.
- Wan, H., T. Liu, C. Su, X. Ji, L. Wang, Y. Zhao, and Z. Wang. 2020. Evaluation of bacterial and fungal communities during the fermentation of Baixi sufu, a traditional spicy fermented bean curd. Journal of the Science of Food and Agriculture 100 (4):1448–57. doi: 10.1002/jsfa.10151.
- Wang, B., Q. Zhang, N. Zhang, K. H. Bak, O. P. Soladoye, R. E. Aluko, Y. Fu, and Y. Zhang. 2021. Insights into formation, detection and removal of the beany flavor in soybean protein. Trends in Food Science & Technology 112:336–47. doi: 10.1016/j.tifs.2021.04.018.
- Wang, C., J. Sun, B. Lassabliere, B. Yu, F. Zhao, F. Zhao, Y. Chen, and S. Q. Liu. 2019. Potential of lactic acid bacteria to modulate coffee volatiles and effect of glucose supplementation: Fermentation of green coffee beans and impact of coffee roasting. Journal of the Science of Food and Agriculture 99 (1):409–20.
- Wang, J., M. Zhao, N. Xie, M. Huang, and Y. Feng. 2022. Community structure of yeast in fermented soy sauce and screening of functional yeast with potential to enhance the soy sauce flavor. International Journal of Food Microbiology 370:109652. doi: 10.1016/j.ijfoodmicro.2022.109652.
- Wang, P., X. Ma, W. Wang, D. Xu, X. Zhang, J. Zhang, and Y. Sun. 2019. Characterization of flavor fingerprinting of red sufu during fermentation and the comparison of volatiles of typical products. Food Science and Human Wellness 8 (4):375–84. doi: 10.1016/j.fshw.2019.11.004.
- Wang, R., P. Dong, Y. Zhu, M. Yan, W. Liu, Y. Zhao, L. Huang, D. Zhang, and H. Guo. 2021. Bacterial community dynamics reveal its key bacterium, Bacillus amyloliquefaciens ZB, involved in soybean meal fermentation for efficient water-soluble protein production. LWT 135:110068. doi: 10.1016/j.lwt.2020.110068.
- Wang, Z. H., J. Dou, D. Macura, T. D. Durance, and S. Nakai. 1997. Solid phase extraction for GC analysis of beany flavours in soymilk. Food Research International 30 (7):503–11. doi: 10.1016/S0963-9969(98)00006-4.
- Wardhani, D. H., J. A. Vázquez, and S. S. Pandiella. 2009. Mathematical modeling of the development of antioxidant activity in soybeans fermented with Aspergillus oryzae and Aspergillus awamori in the solid state. Journal of Agricultural and Food Chemistry 57 (2):540–4. doi: 10.1021/jf802492s.
- Weckbecker, A., and W. Hummel. 2006. Cloning, expression, and characterization of an (R)-specific alcohol dehydrogenase from Lactobacillus kefir. Biocatalysis and Biotransformation 24 (5):380–9. doi: 10.1080/10242420600893827.
- Wei, G., J. M. Regenstein, and P. Zhou. 2021. The aroma profile and microbiota structure in oil furu, a Chinese fermented soybean curd. Food Research International (Ottawa, Ont.) 147:110473. doi: 10.1016/j.foodres.2021.110473.
- Wickware, C. 2015. Critical Parameters For The Production Of Safe Soybean Tempeh [Thesis, Cornell University Graduate School]. Cornell University Library. https://hdl.handle.net/1813/40612.
- Wikandari R., D. A. Kinanti, R. D. Permatasari, N. L. Rahmaningtyas, N. R. Chairunisa, Sardjono C. Hellwig, and M. J. Taherzadeh. 2021. Correlations between the Chemical, Microbiological Characteristics and Sensory Profile of Fungal Fermented Food. In Fermentation 7 (4):261., (Issue doi: 10.3390/fermentation7040261.
- Wolf, W. J. 1970. Soybean proteins. Their functional, chemical, and physical properties. Journal of Agricultural and Food Chemistry 18 (6):969–76. doi: 10.1021/jf60172a025.
- Woo, S. Y., S. Y. Ryu, F. Tian, S. Y. Lee, S. B. Park, and H. S. Chun. 2019. Simultaneous determination of twenty mycotoxins in the Korean soybean paste doenjang by LC-MS/MS with immunoaffinity cleanup. Toxins 11 (10):594. doi: 10.3390/toxins11100594.
- Wu, J. R., J. C. Zhang, P. U. Shi, R. Wu, X. Q. Yue, and H. P. Zhang. 2013. Bacterial community involved in traditional fermented soybean paste dajiang made in northeast China. Annals of Microbiology 63 (4):1417–21. doi: 10.1007/s13213-013-0604-2.
- Wu, J., T. Tian, Y. Liu, Y. Shi, D. Tao, R. Wu, and X. Yue. 2018. The dynamic changes of chemical components and microbiota during the natural fermentation process in Da-Jiang, a Chinese popular traditional fermented condiment. Food Research International (Ottawa, Ont.) 112:457–67. doi: 10.1016/j.foodres.2018.06.021.
- Xiao, Z., C. Lv, C. Gao, J. Qin, C. Ma, Z. Liu, P. Liu, L. Li, and P. Xu. 2010. A novel whole-cell biocatalyst with NAD + regeneration for production of chiral chemicals. PloS One 5 (1):e8860. doi: 10.1371/journal.pone.0008860.
- Xie, C., H. Zeng, C. Wang, Z. Xu, and L. Qin. 2018. Volatile flavour components, microbiota and their correlations in different sufu, a Chinese fermented soybean food. Journal of Applied Microbiology 125 (6):1761–73. doi: 10.1111/jam.14078.
- Xie, M., J. Wu, F. An, X. Yue, D. Tao, R. Wu, and Y. Lee. 2019. An integrated metagenomic/metaproteomic investigation of microbiota in dajiang-meju, a traditional fermented soybean product in Northeast China. Food Research International (Ottawa, Ont.) 115:414–24. doi: 10.1016/j.foodres.2018.10.076.
- Xu, D., P. Wang, X. Zhang, J. Zhang, Y. Sun, L. Gao, and W. Wang. 2020. High-throughput sequencing approach to characterize dynamic changes of the fungal and bacterial communities during the production of sufu, a traditional Chinese fermented soybean food. Food Microbiology 86:103340. doi: 10.1016/j.fm.2019.103340.
- Xu, L. N., S. Guo, and S. W. Zhang. 2019. Effects of solid-state fermentation on the nutritional components and antioxidant properties from quinoa. Emirates Journal of Food and Agriculture 31 (1): 39–45. doi: 10.9755/ejfa.2019.v31.i1.1898.
- Xu, X., H. Liu, and Y. Zhou. 2012. Study on the meitauza production from okara by Actinomucor elegans and Zymomonas mobilis. In Information Technology and Agricultural Engineering (329–36). Berlin, Heidelberg: Springer. doi: 10.1007/978-3-642-27537-1_41.
- Yamasaki, Y., and H. Konno. 1991. Purification and Properties of Glucoamylase from Mucor javanicus. Agricultural and Biological Chemistry 55 (9):2429–30. doi: 10.1080/00021369.1991.10870962.
- Yamashita, H. 2021. Koji starter and Koji world in Japan. Journal of Fungi 7 (7):569. doi: 10.3390/jof7070569.
- Yan, Y-z., Y-l Qian, F-d Ji, J-y Chen, and B-z Han. 2013. Microbial composition during Chinese soy sauce koji-making based on culture dependent and independent methods. Food Microbiology 34 (1):189–95. doi: 10.1016/j.fm.2012.12.009.
- Yang, A., L. Zuo, Y. Cheng, Z. Wu, X. Li, P. Tong, and H. Chen. 2018. Degradation of major allergens and allergenicity reduction of soybean meal through solid-state fermentation with microorganisms. Food & Function 9 (3):1899–909. doi: 10.1039/C7FO01824J.
- Yang, J., X. Wu, H. Chen, D. Sun-Waterhouse, H. Zhong, and C. Cui. 2019. A value-added approach to improve the nutritional quality of soybean meal byproduct: Enhancing its antioxidant activity through fermentation by Bacillus amyloliquefaciens SWJS22. Food Chemistry 272:396–403. doi: 10.1016/j.foodchem.2018.08.037.
- Yang, Y., Y. Deng, Y. Jin, Y. Liu, B. Xia, and Q. Sun. 2017. Dynamics of microbial community during the extremely long‐term fermentation process of a traditional soy sauce. Journal of the Science of Food and Agriculture 97 (10):3220–7. doi: 10.1002/jsfa.8169.
- Yi, S.-H., and S.-P. Hong. 2021. Characteristics of Bacterial Strains with Desirable Flavor Compounds from Korean Traditional Fermented Soybean Paste (Doenjang). Molecules 26 (16):5067. doi: 10.3390/molecules26165067.
- Yong, F. M., and B. J. B. Wood. 1974. Microbiology and Biochemistry of Soy Sauce Fermentation. Advances in Applied Microbiology, 17: 157–94. doi: 10.1016/S0065-2164(08)70558-6.
- Yoo, S. K., W. H. Cho, S. M. Kang, and S. H. Lee. 1999. Isolation and identification of microorganisms in Korean traditional soybean paste and soybean sauce. Microbiology and Biotechnology Letters 27 (2):113–7.
- Yoon, M. Y., Y. J. Kim, and H.-J. Hwang. 2008. Properties and safety aspects of Enterococcus faecium strains isolated from Chungkukjang, a fermented soy product. LWT - Food Science and Technology 41 (5):925–33. doi: 10.1016/j.lwt.2007.05.024.
- Yu, L., X. Pei, T. Lei, Y. Wang, and Y. Feng. 2008. Genome shuffling enhanced L-lactic acid production by improving glucose tolerance of Lactobacillus rhamnosus. Journal of Biotechnology 134 (1-2):154–9. doi: 10.1016/j.jbiotec.2008.01.008.
- Yuan, S. H., and S. K. C. Chang. 2010. Trypsin inhibitor activity in laboratory-produced and commercial soymilk. In Chemistry, texture, and flavor of soy (23–43). Washington: American Chemical Society. doi: 10.1021/bk-2010-1059.ch002.
- Yue, X., M. Li, Y. Liu, X. Zhang, and Y. Zheng. 2021. Microbial diversity and function of soybean paste in East Asia: What we know and what we don’t. Current Opinion in Food Science 37:145–52. doi: 10.1016/j.cofs.2020.10.012.
- Zhang, J., C. Wu, G. Du, and J. Chen. 2012. Enhanced acid tolerance in Lactobacillus casei by adaptive evolution and compared stress response during acid stress. Biotechnology and Bioprocess Engineering 17 (2):283–9. doi: 10.1007/s12257-011-0346-6.
- Zhang, X., P. Wang, D. Xu, W. Wang, and Y. Zhao. 2019. Aroma patterns of Beijing rice vinegar and their potential biomarker for traditional Chinese cereal vinegars. Food Research International (Ottawa, Ont.) 119:398–410. doi: 10.1016/j.foodres.2019.02.008.
- Zhao, G., L.-L. Ding, Y. Yao, Y. Cao, Z.-H. Pan, and D.-H. Kong. 2018. Extracellular Proteome Analysis and Flavor Formation During Soy Sauce Fermentation. Frontiers in Microbiology 9:1872. doi: 10.3389/fmicb.2018.01872.
- Zhao, H., J. Xu, R. Wang, X. Liu, X. Peng, and S. Guo. 2023. Succession and Diversity of Microbial Flora during the Fermentation of Douchi and Their Effects on the Formation of Characteristic Aroma. Foods 12 (2):329. doi: 10.3390/foods12020329.
- Zhao, J., C. Niu, S. Du, C. Liu, F. Zheng, J. Wang, and Q. Li. 2020. Reduction of biogenic amines formation during soybean paste fermentation by using Staphylococcus carnosus M43 and Pediococcus acidilactici M28 as starter culture. LWT 133:109917. doi: 10.1016/j.lwt.2020.109917.
- Zhen-Dong, Z., W. Yu-Rong, X. Fan-Shu, H. Qiang-Chuan, and G. Zhuang. 2021. Distinct bacterial community of a solid-state fermented Chinese traditional food huase sufu revealed by high-throughput sequencing. Food Science and Biotechnology 30 (9):1233–41. doi: 10.1007/s10068-021-00963-3.
- Zhu, Y., and J. Tramper. 2013. Tramper J. Koji-where East meets West in fermentation. Biotechnology Advances. 31 (8):1448–57. doi: 10.1016/j.biotechadv.2013.07.001.