Abstract
Seaweeds have garnered considerable attention due to their capacity to serve as exceptional reservoirs of numerous bioactive metabolites possessing substantial chemical and biological significance. .Phlorotannins constitute a significant class of natural polyphenols originating from brown seaweeds, featuring a broad spectrum of bioactive attributes and demonstrating potential applicability across various sectors. The potential health advantages associated with phlorotannins, particularly concerning the prevention of conditions linked to oxidative stress, such as inflammation, diabetes, and allergies, have generated substantial interest within the food and pharmaceutical industries. Nevertheless, current research remains insufficient in providing a comprehensive understanding of their absorption, as comparisons drawn with their terrestrial counterparts remain speculative. It is commonly presumed that phenolic compounds, including phlorotannins, face challenges due to their limited solubility, instability, and extensive metabolism, all of which restrict their bioavailability. In order to circumvent these limitations and amplify their utility as components of medicinal formulations or healthcare products, researchers have explored various strategies, including the encapsulation or integration of phlorotannins into nano-/micro-particles or advanced drug delivery systems. This review offers a thorough exploration of the structural and biological attributes of phlorotannins and furnishes insights into potential strategies showing promise for their effective utilization in preclinical and clinical applications.
Introduction
The marine environment, widely recognized as a vast and unique repository, houses a multitude of organisms, including macroalgae (seaweeds), microalgae and other diverse life forms such as tunicates, sponges, soft corals, and mollusks (Victor and Sharma Citation2015). These organisms play a vital role within the aquatic ecosystem by providing nourishment and shelter to fish and other marine life. Furthermore, they hold significant promise as resources for the exploration of novel bioactive compounds with potential utility for human health (Ghosh et al. Citation2022). These bioactive compounds derived from natural sources are highly prized for their favorable characteristics, including lower toxicity and enhanced effectiveness, making them valuable for a range of therapeutic purposes (Aung et al. Citation2017). In contrast to terrestrial environments, the distinctive environmental factors in the marine system, such as fluctuating temperature, pressure, and light conditions, likely contribute to the production of unique metabolites (including proteins, peptides, amino acids, fatty acids, sterols, polysaccharides, oligosaccharides, phenolic compounds, photosynthetic pigments, vitamins, and minerals) by certain marine organisms (Karthikeyan, Joseph, and Nair Citation2022).
Phlorotannins, a distinct class of secondary metabolites belonging to polyphenolic compounds, are particularly derived from brown seaweed (Phaeophyta), primarily from the orders Laminariales (Laminariaceae) and Fucales (Fucaceae and Sargassaceae) (Nazzaro et al. Citation2019). Although some reviews have suggested the presence of trace amounts of phlorotannins in red algae (Pradhan et al. Citation2022), these claims lack support from published research articles or specific algal species. Phlorotannins are oligomers or polymers composed of the monomeric unit “phloroglucinol” (1,3,5-trihydroxybenzene) and exhibit structural dissimilarity compared to terrestrial plant polyphenols like phenolic acids or flavonoids (Shrestha, Zhang, and Smid Citation2021). Due to variations in size, degree of polymerization, and types of subunits, phlorotannins display structural diversity with molecular weights ranging from 126 Da to 650 kDa (Catarino et al. Citation2022). Moreover, different extraction and analytical techniques used in research may yield different phlorotannin profiles, adding to the complexity of identifying and quantifying specific compounds. To date, approximately 150 phlorotannins have been reported from brown seaweeds, with research into these compounds continuing to grow exponentially (Rajan et al. Citation2021).
Reported biological properties of phlorotannins encompass antioxidant (Kord et al. Citation2021), anti-inflammatory (Raja et al. Citation2023), and anti-diabetic (Moheimanian et al. Citation2023) potentials. Nevertheless, despite the increasing research interest in the therapeutic applications of phlorotannins, their limited bioavailability in the digestive tract and circulatory system remains an area requiring improvements (Corona et al. Citation2016). Bioavailability is a critical concept within the realm of bio-efficacy, serving as a fundamental indicator for assessing the efficacy of ingested nutrients. It pertains to the degree to which absorbed nutrient components successfully enter the systemic circulation, thereby enabling the accomplishment of highly efficient biological activities (Shahidi and Peng Citation2018). Like other polyphenols, phlorotannins are commonly believed to possess low bioaccessibility, ranging from approximately 2%–15% (Zhao et al. Citation2023). These compounds readily form crosslinks with other macromolecules, such as carbohydrates or proteins. Additionally, high molecular weight phlorotannins (up to 100 kDa) have demonstrated a propensity to interact with dietary and endogenous proteins, resulting in poor absorption in the upper gastrointestinal tract (Corona et al. Citation2016). Furthermore, phlorotannins are highly unstable in the acid-base environment encountered during the transition from the small intestine to the gut, undergoing rapid transformation and/or metabolism by digestive enzymes (Tong, Liu, and Yu Citation2021).
Given these properties, further research is warranted to explore strategies that maximize the biological activity of phlorotannins and their incorporation into clinical treatments. In recent years, encapsulation techniques, which enclose targeted materials, have gained widespread application for delivery into the system. Various carriers have been utilized for encapsulation, including microspheres, hydrogels, and nanoparticle-based drug carriers such as liposomes, niosomes, polymeric nanoparticles, and solid lipid nanoparticles (Mirchandani, Patravale, and Brijesh Citation2021). These carriers have demonstrated remarkable capabilities in enhancing nutrient/drug entrapment, improving bioavailability, and optimizing the pharmacokinetic profiles. However, to the best of our knowledge, there is currently no comprehensive insight into the specific digestive process of phlorotannins or the strategies to improve their bioavailability. Therefore, this review aims to shed light on the possibilities of improving the delivery and therapeutic potential of phlorotannins by exploring various strategies and considering plausible perspectives. Additionally, it offers promising opportunities for maximizing their bioavailability and harnessing their beneficial properties for targeted and controlled drug release.
Overview of seaweeds and their global utilization
Importance of seaweeds
Seaweeds, also known as marine macroalgae, constitute a foundational botanical category within marine ecology, comprising an estimated ∼24,000 species, of which ∼12,000 have been described (Guiry Citation2012). Anchoring themselves to shallow-water rocky substrates or aggregating in shallow marine waters, seaweeds are taxonomically categorized into three groups based on pigmentation: Rhodophyta (red), Phaeophyta (brown) and Chlorophyta (green) macroalgae (Xie et al. Citation2023). Seaweeds are renowned for their ecological significance, giving rise to benthic seascape formations that, in terms of ecological importance, can be likened to terrestrial forests, such as primarily production construction, contribution to nitrogen fixation, and cementation of reef framework, facilitation of coral settlement and creation of habitats for other reef species (El-Manaway and Rashedy Citation2022). Within submerged ecosystems, numerous herbivorous organisms sustain themselves by relying on seaweeds, thus facilitating energy transfer to higher trophic levels. The central role played by seaweeds in the marine food web is highlighted by their ability to capture solar energy for autotrophic nutrition, simultaneously releasing oxygen through photosynthesis. This pivotal role is exemplified by their contribution to approximately half of the total primary production, despite their representation comprising less than 1% of Earth’s total photosynthetic biomass (Falkowski Citation2012). Beyond this, seaweeds fulfill a critical ecological function by serving as agents of nutrient and toxin regulation, actively sequestering substances deleterious to marine organisms. In this capacity, they function as a natural filtration system within the vast underwater environment, entrapping heavy metals and chemical agents (Znad, Awual, and Martini Citation2022).
Undoubtedly, seaweeds possess an inherent appeal due to their culinary and edible attributes. However, while over 200 species of seaweeds are globally cultivated or wild-harvested for various industries, only 21 of these have received official authorization for use as vegetables and condiments (Pereira Citation2016). Upon closer examination, seaweeds reveal a wealth of functional ingredients and bioactive compounds that can be harnessed for the development of food supplements and dietary health products. From a nutritional perspective, seaweeds excel in their capacity to accumulate saccharides, proteins, phenolic compounds, unsaturated fatty acids, vitamins, and minerals. Specifically, saccharides are the most prevalent constituents within seaweed species, constituting a range of 4%–76% of their dry mass (DW) (Shannon and Abu-Ghannam Citation2019). Primary polysaccharides found in brown algae include alginate, fucoidan, and laminarin, while red algae contain carrageenans and agarans, and green algae contain ulvans (Patel et al. Citation2023). Although protein, polyphenol, and lipid contents are generally modest across all species, they play indispensable roles, with proteins aiding in the synthesis of hormones, enzymes, and hemoglobin, lipids mitigating the risk of several chronic diseases, and polyphenols acting as potent antioxidants (Subbiah et al. Citation2023). Notably, the nutritional profiles of seaweeds exhibit multifaceted variability influenced by factors such as species, age, size, reproductive status, ecological context, and a constellation of environmental parameters, including seawater pH, depth, temperature, salinity, nutrient enrichment, oxygen levels, ultraviolet radiation exposure, light intensity, and the extent of herbivore activity (Lobine, Rengasamy, and Mahomoodally Citation2022; E. Park et al. Citation2023).
Global market of seaweeds
The global cultivation and production of seaweed have undergone significant growth in recent decades, witnessing a remarkable increase from 0.56 million tons in 1950 to 35.82 million tons in 2019 (Junning and Giulia Citation2021). According to the estimations by Food and Agriculture Organization (FAO), the global seaweed industry was valued at over USD $6 billion annually in 2018 (FAO Citation2018). This value is projected to continue its upward trajectory and reach USD $30.2 billion by 2025 (Deepika et al. Citation2022).
As documented by FAO (Citation2022), Asia overwhelmingly dominates global seaweed production, accounting for ∼99.54% of the total output. In contrast, Africa contributes 0.3%, America 0.07%, Europe 0.06% and Oceania 0.03% to a much lesser extent. Within Asian seaweed production, China stands as the foremost contributor, yielding over 20 million tons annually, constituting ∼59.46% of the global aggregate (FAO Citation2022). Notable contributors following China include Indonesia, South Korea, the Philippines, North Korea, and Japan. Japan, in particular, with its archipelagic geography, has a longstanding culinary tradition rooted in the utilization of various seaweed species for sustenance. The harvesting of Pyropia sp. from wild populations in Japan can be traced back to the seventeenth century. Additionally, additional seaweed species such as Undaria, Saccharina, Monostroma, Ulva, and Cladosiphon have also undergone significant cultivation and development (Tanaka, Ohno, and Largo Citation2020). Likewise, Australia, benefiting from its geographical location amidst the convergence of three distinct oceanic currents, showcases a rich tapestry of algal diversity, although it represents only 0.03% of the world market. For example, native seaweeds of the Chlorophyta phylum thrive in the pristine ecosystems of the Great Barrier Reef (Deepika et al. Citation2022).
In the broader context of global seaweed production, brown seaweeds hold a significant share, accounting for ∼62.37% in the year 2019. Within this category, noteworthy brown seaweed species such as Laminaria, Saccharina, and Lessonia emerge as preeminent contributors, collectively constituting ∼57.06% of the total brown seaweed production worldwide. Additional species, including Undaria, Sargassum, Macrocystis, and various miscellaneous varieties, make more modest contributions to this sector (Junning and Giulia Citation2021).
Utilizations of seaweeds
Seaweeds possess a rich history of utilization, extending into both culinary and medicinal domains. They have been integral to human diets, with particularly notable prominence in the Asian region, and have also been highly regarded for their ability to yield valuable compounds (Chopin and Tacon Citation2021). The global seaweed industry comprises 85% of food products for human consumption, and seaweed-derived extracts make up almost 40% of the world’s hydrocolloids market (Debbarma et al. Citation2022). For instance, agars and carrageenans extracted from red seaweeds, as well as alginates extracted from brown seaweeds, have found applications as thickening and gelling agents in various food products such as ice creams, jellies and shakes (Deepika et al. Citation2022). Furthermore, due to their macro- and micro-nutritional constituents, seaweeds are seen as a promising strategy to address the growing challenges associated with food supply stress resulting from the increasing global population (Duarte, Bruhn, and Krause-Jensen Citation2021). In addition to their culinary uses, some commercially significant seaweed species are also employed in the production of fertilizers, feed additives, biofuels, and various environmental applications, although to a lesser extent (Hessami, Rao, and Ravishankar Citation2019). For example, certain quaternary cations, such as NH4+ found in seaweed extracts from Laminaria sp., provide buffering capacity for the roots while acting as osmoprotectants, which are particularly beneficial in agriculture, especially in marshy lands (Blanz et al. Citation2019).
Brown seaweed-derived phlorotannins
Generalized structural diversity
Phlorotannins are found in various brown seaweed species, including but not limited to Ecklonia, Eisenia, and Ishige species (Erpel et al. Citation2020). They are known for their chain- and/or net-like structures with diverse molecular weights. Phlorotannins can be further classified into six subclasses based on their linkage polymorphism: phlorethols, fuhalols, fucols, fucophloroethols, eckols, and carmalols (as shown in ). These subclasses are characterized by various types of covalent bonds, including:
Aryl-ether bonds and ether bridges (para- or ortho-arranged).
Aryl-aryl bonds.
Phenyl bonds.
Dibenzo-1,4-dioxin bonds (Charoensiddhi et al. Citation2020; Kumar et al. Citation2022).
The presence of additional hydroxyl groups (–OH) accounts for the differences between fuhalols and phlorethols (Y. Li et al. Citation2017), as well as between eckols and carmalols (Catarino, Amarante, et al. Citation2021).
In addition to nonhalogenated phlorotannins, halogenated phlorotannins have also been reported. For instance, monobromo-/monoiodophloroglucinol and 4′-bromo/4′-idodoeckol were identified several decades ago by Glombitza and Gerstberger (Citation1985) from the brown alga Eisenia arborea. Subsequently, four other halogenated phlorotannins were discovered and reported in 1999 from another species, Carpophyllum angustifolium, after per-acetylation. These include 2-chlorophloroglucinol triacetate, 2[D’] iododiphlorethol pentaacetate, 3[A] chlorobifuhalol hexaacetate, and 3[A4] chlorodifucol hexaacetate (Glombitza and Schmidt Citation1999). In the same year, Sailler and Glombitza (Citation1999) isolated and characterized no fewer than 17 halogenated (brominated, chlorinated and iodinated) phlorotannins, along with 30 non-halogenated forms from the Sargassaceae Cystophora retroflexa.
Biosynthesis of phlorotannins in brown algae
The biosynthesis of phlorotannins involves multiple steps, and although consensus on the exact mechanisms varies among scientists, a general outline of the biosynthesis process can be summarized as follows: (A) phloroglucinol formulation, (B) oxidative coupling and enzymatic modification, and (C) subcellular localization. For a more detailed understanding of the potential biosynthetic pathway, refer to .
Figure 2. Potential biosynthesis pathway of phlorotannin in brown seaweed cells (Gómez and Huovinen Citation2020; Phang et al. Citation2023). PKS: polyketide synthase; CoA: coenzyme A.
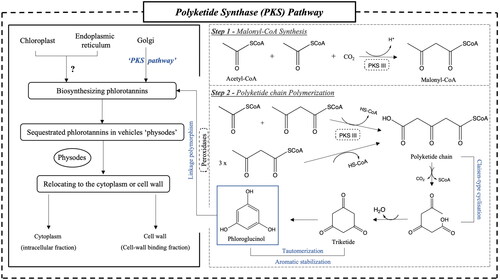
The biosynthesis of phlorotannins, which are considered the fundamental building blocks of this class of compounds, is thought to commence with the production of phloroglucinol. Three potential pathways for phloroglucinol production have been proposed, namely the shikimic acid pathway, the phenylpropanoid pathway, and/or a polyketide synthase pathway (PKS), based on existing knowledge (Pelletreau Citation2008). However, Siriwardhana, Lee, and Jeon (Citation2005) have suggested that the shikimic acid pathway is more likely to serve as the metabolic pathway for condensed tannins and hydrolyzable tannins within the polyphenol category, with limited evidence specifically addressing phlorotannins. Conversely, due to the involvement of type III polyketide synthase (PKS III), the PKS pathway has gained stronger support as a more plausible and widely accepted route for phlorotannin biosynthesis, as corroborated by research studies (Bertoni Citation2013; Subbiah et al. Citation2023).
This hypothesis received support from Meslet-Cladière et al. (Citation2013), whose genome investigation of the brown alga Ectocarpus siliculosus led to the discovery of a predicted key enzyme, PKS1, capable of facilitating the consecutive condensation of acetate and malonate units. In the first step, two molecules of acetyl coenzyme A (acetyl-CoA) are converted into malonyl-CoA in the presence of carbon dioxide, catalyzed by PKS III. The resulting polyketide chain undergoes Claisen-type cyclization, generating a hexacyclic triketide. Subsequently, tautomerization and aromatic stabilization processes occur, resulting in the production of thermodynamically stable phloroglucinols (Fernando, Lee, and Ahn Citation2022).
Once phloroglucinol is synthesized, it undergoes oxidative coupling reactions facilitated by peroxidases, notably vanadium-dependent haloperoxidases, or phenoloxidases, as well as heme-containing glycoproteins. These enzymes play a catalytic role in the formation of end-radicals within phloroglucinol oligomers, achieved through the dehydrogenation of hydrogen atoms on –OH bonds, utilizing hydrogen peroxide (H2O2) as a reactant (Berglin et al. Citation2004; Lemesheva et al. Citation2020). The coupled C–C and/or C–O–C linkages contribute to the structural diversity observed among the six classes of phlorotannins mentioned above.
Regarding the localization of phlorotannin biosynthesis, it has been proposed that they are predominantly found within the chloroplast or its membrane, with potential involvement of the endoplasmic reticulum and Golgi bodies (Schoenwaelder and Clayton Citation2000). In general, based on their interactions with algal cells, phlorotannins exist in two distinct sub-cellular fractions: intracellular (cytoplasm) and cell wall-binding fractions (Birkemeyer et al. Citation2020). Polar and/or reactive-formed phlorotannins are initially sequestered in physodes, specialized secretion vesicles, which have the ability to be secreted into the extracellular compartment to form insoluble cell-wall phlorotannins that become embedded in the alginate matrix and other components of the cell wall through oxidative cross-linking (Arnold and Targett Citation2003; Emeline et al. Citation2021). The second fraction of phlorotannins is predominantly associated with carbohydrates or proteins, such as alginates, and they exhibit characteristics akin to conventional tannins, contributing to the integrity of the cell wall (Birkemeyer et al. Citation2020).
Quantification of phlorotannins
Quantifying phlorotannins holds significant importance across various research and industrial purposes. Researchers, for instance, employ phlorotannin quantification to enhance their understanding of the ecological roles played by brown seaweeds in marine ecosystems or to assess potential applications in the food, pharmaceutical, or cosmetic industries, along with determining the nutritional values of seaweed-based products. Common methodologies utilized for the quantification of phlorotannins encompass spectrophotometry assays (e.g., Folin-Ciocalteu assay), high-performance liquid chromatography (HPLC), and nuclear magnetic resonance (NMR).
Two spectrophotometry assays have been commonly employed to quantitatively assess the phlorotannin contents in seaweeds, including the Folin-Ciocalteu assay and the 2,4-dimethoxybenzaldehyde (DMBA) assay, which have garnered recognition due to their applicability, stemming from their distinctive interactions with the target compounds (Erpel et al. Citation2023; Gümüş Yılmaz et al. Citation2019). However, reports indicate that the Folin-Ciocalteu method has limited sensitivity. There are also concerns about its accuracy due to potential interference from other constituents in the plant matrix. Soluble sugars and proteins, for instance, can react with the Folin-Ciocalteu reagents, leading to artificially elevated values (Castro-Alves and Cordenunsi Citation2015). Similarly, although the DMBA assay demonstrates greater specificity as it selectively reacts with 1,3- and 1,3,5-trihydroxybenzenes, making it a more discerning option for assessing the phlorotannin structures within seaweed, some phlorotannins may exhibit branching or feature aryl linkages and fuhalols with additional –OH groups at positions 2, 4, or 6. In cases where substitutions occur at these positions, the absence of a color change may impede the detection of these particular phlorotannins (Ford et al. Citation2019).
The Prussian blue assay (PBA), in addition, has seen limited application in a few studies and may exhibit somewhat lower specificity compared to the other two methods (Margraf et al. Citation2015; Subbiah et al. Citation2023). Given that phlorotannins are classified as tannins, the butanol-HCl-iron method may also represent a potential means of quantifying their content, although there is currently no confirmed research validating this approach.
Proton (1H) NMR spectroscopy indeed stands as another valuable approach for quantifying phlorotannin contents when utilizing phloroglucinol as an internal standard. However, it is essential to emphasize that NMR directly measures molar concentrations, and as such, the results obtained through NMR cannot be directly compared with those determined using spectrophotometric assays. Moreover, the quantification of phlorotannins using HPLC is impeded by the scarcity of commercially standardized reference compounds. In other words, the calibration of the sample content can only be achieved through quantification using known standards (Ford et al. Citation2019). presents the phlorotannin contents as reported in various scholarly sources through the aforementioned methodologies.
Table 1. Phlorotannin content among different seaweed species by different determination methods.
Indeed, the quantification of phlorotannin content can vary significantly based on the chosen extraction method, including the selection of organic solvents or solvents ratios. In the case of brown algae, specifically Sargassum angustifolium, researchers have employed three distinct ratios of ethanol-aqueous solvents (30%, 50% and 70%, v/v), resulting in a range of phlorotannin content from 0.4 to 3.4 mg phloroglucinol equivalents (PGE)/g (Hodhodi, Babakhani, and Rostamzad Citation2022). It is well-established that brown algae can adjust their production of these metabolites in response to environmental cues and stress conditions. Typically, phlorotannin levels in brown algae surpass 2% DW in temperate and tropical Atlantic areas, as well as temperate Pacific regions. As noted by Ank, da Gama, and Pereira (Citation2019), brown seaweeds from higher latitudes have demonstrated the capacity to produce phlorotannins in a broader range, often exceeding 4% DW, likely in response to environmental factors or stimuli, compared to algae found in lower latitudes, which generally contain less than 2% DW.
Furthermore, different seaweed parts and growth conditions also affect their phlorotannin contents. For instance, Iken et al. (Citation2007) reported the presence of 0.5% DW of phlorotannins in the pneumatocysts of Cystosphaera jacquinotii (Montagne) Skottsberg and 9% DW of phlorotannins in the branches of Desmarestia antárctica. Singh and Sidana (Citation2013) reported that phlorotannin concentrations can reach notable levels, sometimes as high as 25–30% DW in various algal thalli. However, it is a limitation that the specific seaweed species associated with these high phlorotannin concentrations are not specified in the provided information. This lack of information may suggest the possibility of referring to hypothetical or unidentified species, or it could indicate a potential misunderstanding or misinterpretation of the literature, thus warranting further research and investigation.
Biological activities and bioavailability of phlorotannins
Fascinating biological activities
Due to their diverse chemical structures and the presence of polyhydroxy groups, phlorotannins exhibit a wide range of biological activities. These activities, summarized in , highlight their promising potential in functional foods, nutraceuticals, and the pharmaceutical industry. These biological properties include antioxidative, anti-inflammatory, anti-allergic/histamine, anti-diabetic, and anti-bacterial/microbial effects, among others.
Table 2. A brief summary of phlorotannin biological activities.
Antioxidant
Phlorotannins have been affirmed as excellent exogenous antioxidants that exhibit their antioxidant properties through three main mechanisms, including direct reactive oxygen species (ROS) scavenging, nuclear factor erythroid 2-related factor 2 (Nrf2) signaling pathway and the upregulation of antioxidant enzyme activities.
The ROS are commonly generated as natural byproducts of various metabolic processes in living organisms. When overproduced, they can lead to a state of oxidative stress, which can, in turn, cause damage to lipids, proteins, and DNA, and is associated with various diseases. The ability of phlorotannins to defend against ROS is linked to their intricately branched molecular structure, where phenol rings donate their electrons to these oxidative agents, resulting in the formation of an intermediate phenoxyl radical species that stabilizes unpaired electrons and establishes hydrogen bonds with neighboring –OH groups or engages in dimerization (phenol coupling) to create C–O or C–C linkages (Phang et al. Citation2023). These mechanisms have been corroborated by numerous previous studies employing colorimetric assays focused on determining radical scavenging activity. These assays encompass the assessment of radical scavenging activity against various radicals, including the 2,2-diphenyl-1-picryl-hydrazine-hydrate (DPPH) and 2,2-azino-bis-3-ethylbenzothiazoline-6-sulfonic acid (ABTS) radicals. For example, phlorotannins derived from Fucus vesiculosus and Ascophyllum nodosum have exhibited comparable or even superior DPPH radical scavenging activity when compared to commercially available antioxidants such as butylated hydroxytoluene and ascorbic acid (Liu et al. Citation2017). Similarly, stronger ABTS inhibitory effects than those observed with ascorbic acid were found in the ethyl acetate fractions of Sargassum hemiphyllum and Sargassum pallidum, with the half maximal inhibitory concentration (IC50) values of 0.11 ± 0.01 mg/mL and 0.02 ± 0.00 mg/mL, respectively, in contrast to 0.27 ± 0.00 mg/mL (Chen et al. Citation2023). Additionally, they include assessments of reducing power via ferric ion reducing antioxidant power and phosphomolybdate assay, as well as metal chelating activity. The ferrous chelating properties of phlorotannins obtained from F. vesiculosus were further investigated, revealing up to 95% chelation in water extracts and 55% in acetone aqueous (v/v, 7:3) extracts (Wang, Jonsdottir, and Ólafsdóttir Citation2009).
Another mechanism modulated by phlorotannins involves the activation of Nrf2, a signaling pathway that leads to the downstream production of antioxidants. Phlorotannins have been found to form quinones that act as electrophiles, interacting with the thiol group in Kelch-like ECH-associated protein 1, a crucial sensor of oxidative and electrophilic stress. With further activating quinone reductase activity that led to the reduction and detoxification of electrophilic quinones and quinone derivatives, phlorotannins help result in the formation of Michael adducts and induce a conformational change that releases the Nrf2 protein, which, in turn, promotes the translocation of Nrf2 from the cytoplasm into the nucleus, upregulating the production of antioxidant enzymes such as the heme oxygenase-1 (Hmox-1) (Jun et al. Citation2014). Phlorotannins can also activate the Nrf2 pathway through other mechanisms, such as the phosphorylation of several downstream pathways. Notably, the differential activation of various signaling pathways in response to phlorotannin administration, such as the induction of phosphorylated c-Jun N-terminal kinase (JNK) and phosphorylated protein kinase B (PKB) on HepG2 hepatocytes and the activation of p-Akt and phosphorylated extracellular signal-regulated kinase (ERK) pathways on V79-4 lung fibroblasts by eckol (Jun et al. Citation2014; K. C. Kim et al. Citation2010), is contingent on several factors, including the specific types of phlorotannins, the cell types involved, and the nature of the oxidative stimuli applied.
In addition to its impact on Hmox-1, phlorotannins have also been observed to reduce the expression of NADPH oxidase 2 (NOX-2), a significant source of ROS production induced by lipopolysaccharide (LPS). This findings are supported with an increased expression of glycoprotein 91phox, a catalytic substrates of NOX complex (Cui et al. Citation2015). Meanwhile, A. D. Kim et al. (Citation2014) reported that eckol derived from Ecklonia cava could enhance the expression of manganese superoxide dismutase, which serves as the primary defense mechanism against mitochondrial ROS, safeguarding cells from oxidative stress.
Anti-inflammatory
Inflammation, whether acute or chronic, can give rise to a range of health complications, including autoimmune diseases like rheumatoid arthritis (Shrivastava and Pandey Citation2013), cardiovascular diseases such as high blood pressure (Botelho et al. Citation2021), and gastrointestinal disorders like inflammatory bowel disease (Yao et al. Citation2019). Researchers have posited that phlorotannins exert significant effects on three pivotal mediators, including cytokines, enzymes, and signaling pathways, drawing from observations in cell cultures and animal studies.
Besides the previously mentioned antioxidant mechanisms, phlorotannins alleviate inflammation, often associated with oxidative stress, by modulating the production of inflammation-related cytokines. For instance, treatment with eckol isolated from E. cava at concentrations of 50 µg/mL and 100 µg/mL in a mouse model of anaphylaxis resulted in the reduction of mRNA expression levels of proinflammatory cytokines such as interleukin 1β (IL-1β), IL-6, and tumor necrosis factor-α (TNF-α) (Han et al. Citation2020). They also observed the downregulation of Th2-type cytokines, specifically IL-4, IL-5, and IL-13, which led to a reduction in immunoglobulin (Ig) E and eosinophilic responses. Another study reported an amelioration of endothelial dysfunction in mouse models through the use of pyrogallol-phloroglucinol-6,6-bieckol extracted from the same species, which was attributed to the reduced expression of TNF-α and IL-6 (Son et al. Citation2019). The observed enhancement of transforming growth factor beta can inhibit the activation and function of various immune cells, such as T cells and macrophages, reducing their capacity to produce pro-inflammatory cytokines and mediators. Simultaneously, it promotes the development and differentiation of regulatory T cells, which are essential in maintaining immune tolerance and preventing excessive inflammation (Bierie and Moses Citation2010).
Several enzymes implicated in the processes of inflammation are subject to regulation by phlorotannins. Notably, inducible nitric oxide synthase (iNOS) is among the enzymes responsible for the production of nitric oxide (NO), a pivotal signaling molecule associated with a range of physiological processes, including inflammation, immune responses, and tissue damage (Spiller et al. Citation2019). Recent investigations have revealed that trifuhalol A, extracted from Agarum cribrosum, exerts a significant downregulatory effect on iNOS expression in LPS-stimulated RAW264.7 cell models, resulting in a notable decrease in NO production, estimated at 36.6%–56.8% (Phasanasophon and Kim Citation2019). Lopes et al. (Citation2012) proposed that phlorotannins have the capacity to regulate NO levels not only by inhibiting related enzymes but also through direct scavenging and/or by reducing NO production via their influence on the inflammatory signaling cascade. Moreover, Kurihara, Konno, and Takahashi (Citation2015) reported that fucophlorethol C, isolated from the brown seaweed Colpomenia bullosa, effectively inhibited lipoxygenases (LOX) to a similar extent as the known inhibitor nordihydroguaiaretic acid. LOX plays a crucial role as a rate-limiting enzyme in the conversion of arachidonic acid into leukotrienes, known mediators of inflammation (Hu and Ma Citation2018); therefore, compounds that inhibit LOX have the potential to decelerate this process and exert anti-inflammatory effects.
In terms of signaling pathways, Catarino et al. (Citation2020) reported that phlorotannin derivatives from F. vesiculosus effectively inhibited the phosphorylation and degradation of inhibitory protein κBα, an intracellular protein that functions as a primary inhibitor of the proinflammatory transcription factor nuclear factor kappa B (NF-κB). This inhibition, in turn, prevents the activation of the NF-κB signaling pathway, thereby locking the inflammatory cascade at the transcriptional level. In line with the findings of S. Kim et al. (Citation2019), they observed that the addition of an ethanolic extract of E. cava, primarily containing dieckol, induced the translocation of NF-κB to the nucleus while preventing its binding to the cell DNA, along with a dose-dependent reduction in the levels of cytokines and various enzymes. Trifuhalol A was found to significantly inhibit the expression of JNK and ERK1/2 at concentrations ranging from 5 to 20 µg/mL (Phasanasophon and Kim Citation2019). This inhibition further attenuated mitogen-activated protein kinases (MAPKs), serving as crucial components of a series of vital signal transduction pathways regulating processes such as cell proliferation, cell differentiation, and cell death in eukaryotes (Morrison Citation2012).
Anti-diabetic
Diabetes is a global health issue affecting millions of individuals, with its incidence projected to increase in the coming years. This chronic condition is associated with severe complications, including cardiovascular diseases, kidney damage, and nerve damage (Dal Canto et al. Citation2019). Recent advances in the study of plant polyphenols have brought attention to phlorotannins as potential candidates for modulating key enzymes, specifically α-glucosidase and α-amylase (Lopes et al. Citation2019), which are responsible for the elevation of postprandial glycemia. Several phlorotannins have demonstrated lower IC50 values than acarbose, a clinically approved inhibitor for type 2 diabetes treatment, indicating greater inhibitory potential. For example, fucophloroethol structures obtained from Fucus distichus exhibited an IC50 of 0.89 µg/mL and 13.9 µg/mL against α-glucosidase and α-amylase, respectively. In contrast, acarbose, the pharmaceutical inhibitor, showed IC50 values of 112.0 µg/mL and 137.8 µg/mL for the same enzymes (Kellogg, Grace, and Lila Citation2014). Consistent with the findings of H.-A. Lee, Lee, and Han (Citation2017), oral administration of 2,7″-phloroglucinol-6,6′-bieckol led to a significant reduction in postprandial blood glucose levels in diabetic mouse models induced by streptozotocin, attributed to the delayed absorption of dietary carbohydrates through the inhibition of the related enzymes. The compound exhibited IC50 values of 23.35 µM against α-glucosidases and 6.94 µM against α-amylase, in contrast to acarbose at 130.04 and 165.12 µM, respectively. Lo Piparo et al. (Citation2008) hypothesized that the inhibitory activity of phlorotannins is influenced by the formation of hydrogen bonds between their hydroxyl groups and the carboxylate groups of Asp197 and Glu233, two active sites of α-amylase. This impedes the enzymatic breakdown of complex carbohydrates into simple sugars like glucose. Moreover, similar polyphenol categories, such as flavonoids and phenolic acids, offer insight into possible inhibitory mechanisms influenced by methylation and methoxylation processes on phlorotannin structures (Guan et al. Citation2022). The quantity, position, and substitution pattern of these hydroxyl and methoxy groups are presumed to participate in various chemical interactions with the active sites of α-glucosidase and α-amylase, including hydrogen bonding and electrostatic interactions, further influencing binding affinity and inhibitory potency (J. Zhang et al. Citation2022).
Hepatic gluconeogenesis represents another critical target for diabetes modulation, involving the regulation of insulin-related enzymes, such as glucose-6-phosphatase (G6Pase), phosphoenolpyruvate carboxykinase (PEPCK), and glucokinase. Octaphlorethol A, derived from Ishige foliacea, was identified as an inhibitor of hepatic gluconeogenesis in a study by S.-H. Lee et al. (Citation2016). It significantly suppressed the upregulation of hepatic mRNA expression levels of gluconeogenic enzymes, particularly PEPCK and G6Pase, leading to a reduced hepatic glucose production and potential benefits in diabetes management. Murray et al. (Citation2021) provided compelling evidence through a placebo-controlled randomized clinical trial, demonstrating that the administration of a polyphenol-rich seaweed extract containing 8,8′-bieckol, 6,6′-bieckol, and phlorofurofucoeckol A for 12 weeks resulted in a greater reduction in postprandial glucose levels compared to fasting glucose levels, with further support for the regulation of G6Pase and PEPCK. Conversely, S. Zhang et al. (Citation2020) indicated that certain phlorotannins, notably trifuhalol A, did not exhibit an inhibitory effect on G6Pase, even at increased concentrations. In a randomized crossover trial, the administration of polyphenols extracted from F. vesiculosus demonstrated the potential to moderate glycemic responses in females by reducing peak postprandial glucose concentration (Murray et al. Citation2019). However, this effect was not observed in males, suggesting a gender-specific response that requires further investigation to understand the underlying mechanisms and implications of these gender differences in response to polyphenol treatment.
Oxidative stress plays a pivotal role in the development of type 2 diabetes mellitus (T2DM), resulting from an imbalance between the production of ROS and antioxidant defense. In T2DM, the decreased levels of enzymatic antioxidants contribute to the development of diabetic complications. According to a meta-analysis of 22 studies conducted by Banik and Ghosh (Citation2021), the association between T2DM and biomarkers was emphasized, with eight studies focused on superoxide dismutase (SOD) levels and four on glutathione peroxidase levels. Administration of dieckol through intraperitoneal injections (10–20 mg/kg for 14 days) in diabetic mice resulted in a notable reduction in blood glucose and serum insulin levels, with a slight increase in the activity of the antioxidative enzyme, SOD, in the liver tissues (Kang et al. Citation2013). Similarly, eckol, 7-phloroeckol, and phlorofucofuroeckol-A have been identified as inhibitors of protein tyrosine phosphatase 1B (PTP1B) (Gunathilaka, Keertihirathna, and Peiris Citation2021), an enzyme critical for regulating insulin signaling. PTP1B overactivity can result in insulin resistance; thus, the inhibition of PTP1B is a potential therapeutic strategy to enhance efficient insulin signaling by preventing the dephosphorylation of tyrosine residues and promoting glucose uptake into cells (Koren and Fantus Citation2007).
Anti-allergy/histamine
Histamine, a bioactive amine released by immune cells, serves as a signaling molecule and neurotransmitter in response to allergic reactions (Mandola, Nozawa, and Eiwegger Citation2019). The prevalence and severity of allergic diseases have exhibited a significant rise over the past decade worldwide, particularly affecting approximately 20% of the population in developed countries (Y. Li et al. Citation2008). Two bioactive phloroglucinol derivatives, fucodiphloroethol G and phlorofucofuroeckol A, were initially isolated from E. cava by Y. Li et al. (Citation2008), who reported their notable inhibitory activity against histamine release. This inhibition was demonstrated in human basophilic leukemia (KU812) and rat basophilic leukemia (RBL-2H3) cell lines using a histamine release assay. To support their results, Le et al. (Citation2009) suggested that these active compounds may be useful in preventing allergen-IgE-induced allergic reactions. Likewise, G. Ahn et al. (Citation2015) highlighted the inhibitory effects of dieckol on IgE-mediated degranulation observed in LAD2 cells. An insightful hypothesis posits that phlorotannins have the capacity to stabilize the cell membrane by reducing intracellular Ca2+ levels, thereby inhibiting the binding activity between IgE and its high-affinity receptor. This inhibition, in turn, disrupts downstream signaling cascades, including the activation of protein tyrosine kinases (Syk, Lyn), calcium mobilization, and protein kinase C translocation, all of which are essential for degranulation (Shim, Lee, and Lee Citation2016; Yoshioka et al. Citation2014).
Sugiura et al. (Citation2021) discovered that the phlorotannin dieckol exhibited significant anti-allergic effects in an ear swelling test conducted on mice sensitized to arachidonic acid, 12-O-tetradecanoylphorbol-13-acetate and oxazolone. These effects were attributed to the suppression of degranulation, the release of chemical mediators, and the expression of mRNA, including cyclooxygenase-2 (COX-2), IL-6, and TNF-α, in rat basophilic leukemia-2H3 cells. In another study, 6,6′-bieckol and 6,8′-bieckol were found to inhibit COX-2 activity at a concentration of 500 µM, with inhibition ratios of 9.8% and 40.4%, respectively. Notably, 8,8′-bieckol (96.6%) and phlorofucofuroeckol A (96.8%) exhibited inhibitory effects comparable to that of epigallocatechin gallate (95.8%) (Sugiura et al. Citation2018). These findings align with the explanation that the bioactivity of phlorotannins is strongly influenced by their molecular size or the number of phenol groups. The structures of eckols and the bieckols, as presented by Sugiura et al. (Citation2018), suggest that the positioning of the hydroxyl group at either C-4 or C-4′ in these compounds may play a crucial role in their ability to suppress degranulation and COX-2 mRNA expression.
In addition, a recent study isolated three phlorotannins from Sargassum carpophyllum, including 2-[2-(3,5-dihydroxyphenoxy)-3,5-dihydroxyphenoxy]-1,3,5-benzenetriol, 2,2′-[[2-(3,5 dihydroxyphenoxy)-5-hydroxy-1,3-phenylene]bis(oxy)]bis(1,3,5-benzenetriol), and 2-[2-[4-[2-(3,5-dihydroxyphenoxy)-3,5-dihydroxyphenoxy]-3,5-dihydroxyphenoxy]-3,5 dihydroxyphenoxy]-1,3,5-benzenetriol (Matsui et al. Citation2022). These compounds were found to prevent immediate hypersensitivity by exhibiting a dose-dependent reduction in β-hexosaminidase secretion in vitro similar to other phlorotannins such as eckol (Han et al. Citation2020), with IC50 values of 50.7, 35.9, and 43.5 µM, respectively. Meanwhile, these phlorotannins exhibited a significant reduction of approximately 50% in prostaglandin D2 (PGD2) levels compared to the positive control group. PGD2 is a major prostanoid secreted by activated mast cells, and elevated PGD2 levels have been closely associated with medical conditions, including asthma and allergic reactions (Nakamura et al. Citation2015). However, their findings revealed no statistically significant effect on the expression of COX-2, the enzyme responsible for converting arachidonic acid to prostaglandin H2 (PGH2), the precursor of PGD2 synthesis. This contrasts with the conclusion previously reported by Sugiura et al. (Citation2018), and as a result, they were unable to establish a definitive relationship between COX-2 expression and the levels of PGD2. It is plausible that the activity of downstream enzymes involved in PGD2 synthesis or the presence of other mediators in the cellular environment may have influenced the observed results.
Anti-microbial/viral
The hypothesis suggests that phlorotannins may exert antiviral effects through two primary mechanisms: by preventing the virus from entering host cells and by inhibiting viral replication once it has entered the host cell. J.-Y. Park et al. (Citation2013) observed that the dieckol derived from E. cava competitively inhibits 3CLpro, a cysteine proteinase with similarities to chymotrypsin, which is crucial for viral replication. Other enzymes, such as reverse transcriptase and protease of human immunodeficiency virus type 1 (HIV-1), were reported to be potently inhibited by 8,4′′′-dieckol and 8,8′-bieckol (M.-J. Ahn et al. Citation2004). Likewise, it was observed that phlorotannins extracted from E. cava suppressed the production of surface proteins, such as hemagglutinin and neuraminidase, which are responsible for the initial attachment of the virus to host cells and the release of newly formed virions, respectively (Cho et al. Citation2019). Meanwhile, phlorotannins were found to increase the activity of mitochondrial dehydrogenases, causing membrane depolarization, as well as reducing the ergosterol content of the fungal cell wall, thus demonstrating efficacy against yeast and several dermatophytes (Lopes et al. Citation2013). A synergistic effect was reported when using phlorotannins in conjunction with antibiotics against the Listeria genus (H.-J. Kim et al. Citation2018).
Bioavailability of phlorotannins
The concept of bioavailability refers to the proportion of an administrated dose of nutrients that enters the systemic circulation (Price and Patel Citation2021), which is influenced by factors such as biological response (bioactivity), metabolism, absorption (bioaccessibility). While numerous biological activities of phlorotannins have been determined, research on their bioavailability is still ongoing. Scientists have hypothesized that they exhibit similar behaviors to other polyphenols, such as phenolic acids and flavonoids. In this regard, the overall bioavailability of phlorotannins is anticipated to be limited, as Catarino, Marçal, et al. (Citation2021) reported only a small fraction (14.1%) of phlorotannins from F. vesiculosus is likely to undergo absorption in the upper gastrointestinal tract. This finding aligns with the results of Corona et al. (Citation2016), who observed that, following the ingestion of a phlorotannin-rich capsule by healthy volunteers, most of the phlorotannin metabolites were subsequently detected in their urine and plasma, indicating a low rate of absorption in the small intestine.
In detail, the low absorption rate could probably be explained by the polymeric nature of phlorotannins, as well as their molecular weight and interactions with other dietary components (Meng et al. Citation2021). The initial challenge in the absorption of phlorotannins is to liberate them from the seaweed matrices, because they are typically embedded or bound within the cellular and structural components that hinder their release, especially polysaccharides and proteins (Ford et al. Citation2020). Phlorotannins, with higher molecular weights, have a greater capacity to form bonds with proteins, which reduces their solubility and makes it more challenging for the digestive system to break them down and absorb them in the small intestine (Cáceres-Jiménez et al. Citation2023). Also, it is hypothesized that enzymes responsible for metabolizing them may have reduced access to the bound phlorotannins, slowing down their transformation into low molecular weight derivatives. Catarino, Marçal, et al. (Citation2021) observed a shielding effects between bound phlorotannins and gastric enzymes that prevent their degradation during the upper gastrointestinal and therefore forcing their transportation to the colon. On the other hand, some interactions between phlorotannins and cell wall polysaccharides, such as fucoidans and alginates, would also affects their release. For example, alginates can form a three-dimensional gel-like network that captures phlorotannins, similar to the patterns seen with condensed tannins-proanthocyanidins (Liu and Bourvellec Citation2023).
Moreover, phlorotannins undergo various metabolic processes. Similar to terrestrial polyphenols, these metabolism processes can be categorized into three phases. Phase I reactions primarily entail intramolecular modifications, including processes such as oxidation, reduction, and hydrolysis. Meanwhile, Phase II reactions involve the conjugation of endogenous substances with ingested compounds, transforming them into water-soluble substances that can be easily excreted from the body. This process is often referred to as detoxification and is achieved through main processes like acetylation, methylation, glucuronidation, and sulfation (also known as sulfonation) (Schaffenburg, Lockshin, and DeKlotz Citation2021). It is worth noting that recent emphasis has been placed on a term “Phase III reaction” in the context of drug or nutrient metabolism, which is responsible for the final elimination of toxins and metabolic products from cells (Phang-Lyn and Llerena Citation2019). Together, these processes influence their stability during transit. In their pioneering work, Corona et al. (Citation2016) investigated the possibility of the relationship between ingested seaweed and their colonic biotransformation by gut microbiota. This study successfully identified a variety of unconjugated and conjugated metabolites of phlorotannins, including glucuronides and/or sulfates, in urine and plasma samples. During Phase II detoxification, phlorotannins undergo transformations that cause them to form larger and more polar conjugates, which hampers their passive diffusion across biological membranes, ultimately diminishing their bioavailability and facilitating their elimination from the body (Corona et al. Citation2017). This possibility found further support in a clinical experiment conducted on obese individuals, where the analysis of their urine revealed the presence of various phlorotannin conjugates, including phloroglucinol sulfate, hydroxytrifurahol A-sulfate, dioxinodehydroeckol, fucophloroethol glucuronide, diphlorethol sulfates, and dioxinodehydroeckol glucuronide (Baldrick et al. Citation2018).
Safety and stability of phlorotannins
When considering phlorotannins for medical or health-promoting purposes, addressing their safety concerns is imperative. However, the majority of the available studies are conducted in vitro based on cell models, with limited in vivo animal or human research. Indeed, phlorotannins have been shown to be nontoxic or not significantly toxic to several cell lines in most of the studies.
For example, diphlorethohydroxycarmalol from Ishige okamurae was reported to increase cell viability against H2O2-induced oxidative stress in murine hippocampal neuronal cells. The highest cell survival rate reached 77.21% at a dose of 50 µM, compared to the blank control, which only had a survival rate of 17.18% (Heo et al. Citation2012). This assessment was conducted using the 2,5-diphenyl-2H-tetrazolium bromide (MTT) assay, a widely used test in cell biology and toxicology for evaluating cell viability and proliferation, enabling the indirect assessment of substance toxicity. However, there were some controversial results, such as dieckol (20–100 µg/mL) decreasing cell viability and increasing prostaglandin E2 (PGE2) release on HaCaT cells exposed to particulate matter (PM10) (Ha et al. Citation2019), which could potentially lead to cardiovascular conditions, respiratory symptoms, chronic inflammation and tissue damage. These results suggest that the response patterns may vary depending on the cell line models and the specific doses applied.
In terms of animal studies, the safety and toxicity of phlorotannins have been extensively investigated using zebrafish, mice, rats and dogs. These studies have generally shown a 100% survival rate while exhibiting efficient biological activities. However, some slight symptoms have been reported, such as soft stool and diarrhea in Beagle dogs when given high-purity phlorotannin fractions at a dose of 750 mg/kg on days 3 and 13 (Yang et al. Citation2014). It is worth noting that these effects were only observed at high oral doses, and no adverse effects were found at doses of 250 and 500 mg/kg. Similar safety has also been demonstrated in human trials with phlorotannin-rich capsules, especially eckol from E. cava at a dose of 500 mg/day and treatment for one week (Um et al. Citation2018).
The European Food Safety Authority (EFSA) Panel on Dietetic Products, Nutrition and Allergies (NDA) delivered a scientific report on the safety of E. cava products (SeaPolynolTM) (Patent NO.: US10016471B2) as a phlorotannin-rich food supplements (Turck et al. Citation2017). They reported that there is no evidence of genotoxicity for the product and identified a low risk of allergic reactions. They suggested an intake of 163 mg/day for adolescents aged 12 to 14 years (mean body weight of 43.4 kg), 230 mg/day for adolescents above 14 years of age (mean body weight of 61.3 kg), and 263 mg/day for adults (default body weight of 70 kg).
Investigating strategies to improve bioavailability and bioactivities of phlorotannins
Research on phlorotannins is still in its early stages, and one commonly employed approach to overcome their inherent limitations involves the micro-/nano-encapsulation of phlorotannins within a carrier (Tong, Liu, and Yu Citation2021). Various carrier systems, such as nano-emulsions, liposomes, edible films, nanoparticles, nanogels, have been utilized for delivering terrestrial polyphenols (J. Zhang et al. Citation2023). In the case of phlorotannins, specific examples of targeted carriers can be observed.
Polymeric nanoparticles
To enhance the therapeutic effectiveness of phlorotannins in the human body, Bai et al. (Citation2020) conducted a study on the encapsulation efficiency of polyvinylpyrrolidone (PVP) nanoparticles, employing various ratios in combination with phlorotannins. Among these ratios, the nanoparticles with a 1:8 ratio exhibited superior stability in water, remaining without precipitation for 5, 10, and 15 days at room temperature compared to free phlorotannins. Furthermore, the application of PVP nanoparticles at concentrations of 6.25 and 12.5 µg/mL in a HaCaT keratinocyte model resulted in a significant reduction of ROS production by 12% and 18%, respectively, without detectable irreversible cytotoxic effects. However, after discovering the carcinogenic nature of PVP, its use in health supplements and oral administration became limited. Consequently, Bai, Chen, and Qi (Citation2022) developed an alternative approach by formulating improved protein-polysaccharide nanocomplexes using whey protein isolate and chitosan oligosaccharides, which are edible, cost-effective, and commercially available. In their experiment, Caco-2 cells exposed to hydrogen peroxide exhibited a decrease in cell viability to 68.79 ± 3.78%. However, treatment with phlorotannin-loaded nanoparticles at varying concentrations revealed a dose-dependent increase in cell viability. Notably, at a concentration of 40 µg/mL of phlorotannin-loaded nanoparticles, the cell viability significantly rose to 84.83 ± 1.92% (p < .05). Additionally, the administration of the nanoparticles at the aforementioned concentration effectively restrained ROS generation in H2O2-treated cells and NO production in LPS-induced cells, bringing them to levels comparable to the negative control group, which further validates the enhanced anti-inflammatory and antioxidant capacity achieved through nano-encapsulation.
Another investigation employed electrospinning to prepare zein nanofiber membranes loaded with ε-polylysine-polyphenol-polyaspartic acid for the controlled delivery of polyphenols, including phlorotannins (Yingying et al. Citation2022). In terms of their in vitro release profile, approximately 19.65% of the phlorotannins were released within the initial 2 h under acidic conditions (pH 1.2), thereby illustrating the protective effect of zein nanofiber membranes against the acidic gastric pH environment. Moreover, a higher release of 62.35% occurred over an 8-h period at pH 6.8, which was facilitated by the electrostatic interaction between ɛ-polylysine and polyaspartic acid, effectively enhancing system stability and preventing burst release. Consistent with the findings reported by Amjadi et al. (Citation2022), the investigation revealed that zein nanofiber membranes effectively preserved the activity of polyphenols and displayed favorable biocompatibility, as well as induced a higher degree of stress and apoptosis in HT-29 cells, a human colorectal adenocarcinoma cell line with epithelial morphology. Consequently, electrospinning has been validated as a highly effective method for fabricating sub-micron or nano-scale polymer fibers, which can be tailored to serve as safe and nontoxic drug delivery systems (DDSs). Moreover, Surendhiran, Cui, and Lin (Citation2019) suggested using various biodegradable polymer-based nanofibers for food preservation purposes. In their study, they fabricated sodium alginate and poly(ethylene oxide) (PEO) blended nanofibers encapsulated with phlorotannin (50:50:10, v/v/v) via electrospinning process, which exhibited temperature-dependent antimicrobial activity. The preservation capacity of active nanofibers was assessed against Salmonella enteritidis on chicken at 4 and 25 °C, with cell count drastically decreasing from 6.20 to 3.28 Log CFU/g at 4 °C and from 8.80 to 2.53 Log CFU/g at 25 °C. Due to the presence of 8 interconnected rings in marine algal polyphenols, the phlorotannin encapsulated nanofibers showed higher overall acceptability scores in sensory assessment, with food preservability almost equal to sodium nitrite, a widely used synthetic preservative in meat industries (Sathya et al. Citation2017). Similarly, a multifunctional wound dressing using gelatin/chitosan hybrid fibers embedded with a phlorotannin-enriched extract derived from the seaweed Undaria pinnatifida was prepared (Ferreira et al. Citation2021). Electrospun meshes were created by incorporating 1 or 2 weight (wt)% of the extract, resulting in enhanced structural stability over time, as indicated by reduced in vitro degradation rates under enzymatic conditions. Initial antimicrobial assessments demonstrated the effectiveness of mesh against Pseudomonas aeruginosa and Staphylococcus aureus, confirming its antimicrobial activity. Regarding biological characterization, none of the tested conditions showed cytotoxic effects on hDNF cells, allowing for the adhesion and proliferation of the cells over a span of 14 days, with the exception of the 2 wt% condition after 7 days exhibited some cytotoxicity effects.
Liposomes
Nano-liposomes, also known as submicron bilayer lipid vesicles, represent a novel technology for the efficient encapsulation and targeted delivery of bioactive agents, which enable the incorporation of a wide range of bioactive materials, including pharmaceuticals, cosmetics, and nutraceuticals (Mozafari Citation2010). This technology has opened up diverse opportunities for the development of advanced delivery systems in various fields, allowing for improved efficacy and controlled release of bioactive compounds. Savaghebi, Ghaderi-Ghahfarokhi, and Barzegar (Citation2021) explored the potential of nano-liposomes encapsulated with brown algae extract (Sargassum boveanum) for their antioxidant and antimicrobial properties. Their research indicated that the ethyl acetate fraction demonstrated significant activity against gram-positive bacteria (Bacillus cereus), with minimum inhibitory concentration (MIC) values of 0.937 and 0.469 mg/mL before and after encapsulation in nano-liposomes. This finding was supported by the speculation made by Hierholtzer et al. (Citation2013) that phlorotannins, present in the brown algae extract, exert antimicrobial effects by disrupting cell membranes and eliciting substantial extra- and intra-cellular responses. On the contrary, certain bacterial strains have developed resistance to a vast array of antibiotics, particularly among gram-negative species. This resistance can be attributed to the presence of an additional outer membrane within their cell wall structure, serving as a protective barrier that restricts the entry of numerous antibiotics into the bacterial cell (Breijyeh, Jubeh, and Karaman Citation2020). Phlorotannin extracts and their encapsulated forms exhibited MIC values of 1.875 and 0.937 mg/mL, respectively, against P. aeruginosa and Escherichia coli (Savaghebi, Ghaderi-Ghahfarokhi, and Barzegar Citation2021). Contrary to expectations, a previous study conducted by Savaghebi, Barzegar, and Mozafari (Citation2020) on the same seaweed species revealed that nano-liposomes exhibited a lower antioxidant capacity compared to the free algal extract. The investigation specifically highlighted a reduction in both the radical scavenging ability and the reducing antioxidant power of the nano-liposomes when compared to the unencapsulated algal extract. Notably, the nano-liposomes displayed a notably diminished scavenging capacity on ABTS radicals, with half maximal effective concentration (EC50) values measured at 453.9 and 219.5 ppm, respectively. Zou et al. (Citation2014) proposed that the observed phenomenon can be plausibly attributed to the structural interactions between the liposome matrix and the loaded compounds. In addition to the localization of phenolic compounds within the core of nano-liposomes, the interactions between the compounds and the lipid bilayers also play a significant role in enhancing their retention time and impeding the kinetics of their release (Karonen Citation2022). The balance between the biological activities of the encapsulated compounds and their release patterns within nano-liposomes introduces new challenges for their diverse applications. Undoubtedly, the attainment of an optimal release profile, which guarantees the desired biological effects while preserving stability and controlled release, constitutes a complex undertaking. Addressing these challenges holds the key to unlocking the successful utilization of nano-liposomes as efficacious delivery systems within a wide array of industries.
Composite scaffolds
The incorporation of specific compounds within a range of composite scaffolds, achieved through various mechanisms such as encapsulation, absorption, or entrapment, holds promise for advantageous utilization in osteoinductive applications within the realm of bone tissue engineering (Romagnoli, D’Asta, and Brandi Citation2013). Im et al. (Citation2017) have introduced a biocomposite scaffold that incorporates collagen extracted from fish skin along with phlorotannin sourced from brown algae E. cava. Significantly, this composite scaffold exhibited a substantial increase in both proliferation and mineralization (p < .05) of osteoblast-like cells, in stark contrast to the control group lacking phlorotannins, as well as the group that was only exposed to phlorotannins. This notable enhancement can likely be attributed to the delayed and sustained release of phlorotannin facilitated by the coated components. Supported by Yeo, Jung, and Kim (Citation2012), the utilization of phlorotannins as supplemental bioactive agents in conjunction with scaffolds has been shown to be advantageous for promoting bone tissue growth, attributed to the ability of phlorotannin to create pronounced hydrophilic sites on the composite materials, leading to enhanced wettability.
Future perspectives and possibilities
Apart from using different materials to embed phlorotannins, various chemical/physical modifications to the vehicle can achieve more targeted delivery and controlled release (Priya, Desai, and Singhvi Citation2023). Some of the most widely employed approaches can be summarized as follows: PEGylation (attachment or amalgamation of polyethylene glycol), surface modifications (grafting ligands, peptides and/or proteins), as well as the addition of co-solvents or co-surfactants. Moreover, significant attention has been devoted to the manipulation of microenvironments through the incorporation of magnetic fields (Hari et al. Citation2023; Sanadgol and Wackerlig Citation2020) and/or ultrasonic fields (Wei, Cornel, and Du Citation2021). These modifications alter the drug delivery environment and enhance the permeability of nutrients, ultimately leading to the desired outcomes of targeted or controlled release effects. However, it should be noted that although these strategies have been applied to several terrestrial polyphenols, no research has been conducted specifically on phlorotannins.
PEGylation
The term “PEGylation” was coined to describe the covalent and non-covalent processes that attach or merge PEG polymer chains with molecules and macrostructures (Gupta et al. Citation2019). PEGylation offers various benefits over non-PEGylated products in enhancing the drug circulation time and improving drug stability by reducing their immunogenicity and antigenicity (Yadav and Dewangan Citation2021). It has also been confirmed that PEGylation enhances the permeability and retention effects due to its unrecognizable property by the reticuloendothelial system, providing a unique opportunity to target tumors following systemic administration (Kalyane et al. Citation2019). Regarding specific applications of polyphenols, multifunctional PEG-mesoporous silica nanoparticles (MSNPs) loaded with curcumin (CUR), a polyphenol with a long history of use in Ayurveda medicines to treat various diseases, exhibited strong fluorescence in HepG2 and HeLa cells compared to non-PEGylated MSPNs, reflecting a high accumulation of nanocarriers within cancerous cells and an enhancement in the bioavailability of CUR (Elbialy et al. Citation2020). Likewise, liposomal formulations of luteolin, a naturally derived polyphenol, with or without PEG modification, were studied (Sinha and PK Citation2019). PEGylated liposomes carrying luteolin were the most effective in inducing caspase-3 and caspase-14 protein expressions, two proteins that modulate the cell death program of human keratinocytes. Similar results were observed with liposome containing polyphenols from the red seaweed Kappaphycus alvarezii, which not only increased cellular uptake efficiency but also offered site-specific targeted delivery to overexpressed folate receptors in human adenoma MCF-7 breast cancer cells (Baskararaj et al. Citation2020), suggesting a promising future for phlorotannins.
Recent scrutiny has raised concerns about PEGylation, making it a topic of controversy. While PEGylation reduces interactions with the mononuclear phagocyte system, it is also expected to impede interactions with tumor cells (e.g., via a receptor), potentially affecting uptake by the target tissue (Verhoef and Anchordoquy Citation2013). One critical step in DDSs is the internalization of nanoparticles by cells through endocytosis. Interestingly, evidence suggests that certain PEGylated nanocarriers may not undergo effective receptor-mediated endocytosis in cancer cells within carcinoma tissue (Magarkar et al. Citation2012). Through free energy analysis, Shen et al. (Citation2018) discovered that the aggregation of PEG polymers during the membrane wrapping process of PEGylated carriers introduces a significant energy penalty of approximately 800kBT. The presence of such a substantial energy barrier and the formation of a ligand-free region on the surface of PEGylated nanoparticles can hinder the membrane-wrapping process and reduce the likelihood of internalization by tumor cells. This physically-based theory of free energy transformation was also emphasized by Y. Li, Kröger, and Liu (Citation2014), who suggested that the grafting of PEG polymers before and after the endocytosis process had an effect comparable to or even greater than the bending energy of the membrane during endocytosis. Neglecting an investigation into this free energy gap, the delivery of PEGylated nanoparticles to diseased cells cannot be predicted. Apart from that, it has been observed that linear PEGylated nanocarriers can stimulate the production of anti-PEG IgM, resulting in significant alterations in the pharmacokinetics and biological distribution after several repeated injections, a phenomenon known as “accelerated blood clearance” (Kierstead et al. Citation2015).
Peptide-functionalization
Peptide-functionalization, as the name suggests, involves attaching specific peptides to the surface of co-polymer nanoparticles or micelles. This approach is commonly used in DDSs, particularly in gene therapy, to enhance various desirable characteristics such as superior colloidal stability, enhanced cellular uptake efficiency, effective escape from endo/lysosomes, efficient nuclear import, and successful unpacking of DNA (Muhammad et al. Citation2019). By designing peptides that can recognize specific receptors or biomarkers on target cells or tissues, these modified copolymer carriers can improve drug delivery efficiency and minimize off-target effects. Sun et al. (Citation2017) demonstrated the effectiveness of this strategy by coating MSPNs encapsulating CUR and doxorubicin (DOX) with an azido-peptide sequence composed of glycine (G), phenylalanine (F), leucine (L), histidine (H-H-H), arginine (R-R), aspartic acid (D), and serine (S). These MSPNs exhibited high loading efficiency and content of CUR and DOX, and they were selectively taken up by DOX-resistant MCF-7/ADR cells due to specific recognition triggered by the attached tetrapeptide R-G-D-S, allowing them to enter lysosomes. Additionally, another part of this sequence, H-H-H-R, was found to induce effective lysosomal membrane permeability, which is beneficial for drug escape from lysosomes, CUR-mediated inhibition of P-glycoprotein 1 (P-gp), and nuclear import of DOX into cells. P-gp is a type of membrane transporter primarily responsible for pumping foreign substances out of cells, which can limit the entry of drugs, reduce their bioavailability, and contribute to multidrug resistance. Another example is the TAT peptide, a short sequence derived from HIV, which can facilitate the delivery of various cargoes, including drugs, proteins, and genetic material, into cells. Sancini et al. (Citation2013) reported that CUR-decorated nanoliposomes gained the ability to cross the blood-brain barrier (BBB), a selective barrier that restricts the entry of various molecules (e.g., drugs, toxins, and pathogens), after functionalization with a modified cell-penetrating TAT peptide. They observed a three-fold increase in the uptake of intact nanoliposomes by human brain capillary endothelial cells, with no adverse effects on cell viability.
Magnetic drug targeting
Superparamagnetic iron oxide nanoparticles (SPIONs), like maghemite (γ-Fe2O3), magnetite (Fe3O4) or hematite (α-Fe2O3) particles, offer the potential to encapsulate drugs or therapeutic agents for controlled release, hyperthermia therapy and BBB crossing, and targeted delivery when subjected to an external magnetic field (Mittal, Roy, and Gandhi Citation2022). Amanzadeh et al. (Citation2019) demonstrated the benefits of quercetin-SPIONs, which significantly enhanced learning and memory in rats compared to free quercetin. This enhancement is likely due to the prolonged circulation of quercetin in the bloodstream and its increased concentration in the brain, facilitated by the incorporation of SPIONs. In addition to these improvements, Ramya et al. (Citation2021) proposed a pH-responsive release behavior with silibinin-loaded Fe2O3 nanoparticles. They observed that less than 25 ± 2.2% of silibinin was released within 48 h at pH 7.4 in phosphate-buffered saline. However, more than 54 ± 1.6% of entrapped silibinin was released after a 48-h incubation at pH 4.5. This behavior is attributed to the deformation of the linkage between silibinin and Fe2O3 nanoparticles induced by the acidic pH, facilitating their efficient delivery to the tumor environment (pH 4.0–5.0). Furthermore, C. Li et al. (Citation2020) optimized and prepared tea polyphenol-alginate/chitosan magnetic microcapsules by incorporating nano-Fe3O4 particles and magnetofluid. These microcapsules demonstrated a good slow-release effect lasting up to 100 h and exhibited superparamagnetism with extremely low magnetic retention and coercivity, which means that there is no remanent magnetization when an external magnetic field is removed, making them excellent carriers for delivering tea polyphenols in tumor endovascular embolization therapy.
In the field of seaweed bioactive compounds, magnetic MSPNs coated with a Fe3O4 network have been synthesized for the delivery of fucoxanthin, a carotenoid pigment derived from brown seaweeds (Feng et al. Citation2022), and they can be effectively guided to the tumor site with no adverse effects. Indeed, while specific research on phlorotannins may be limited, the applications and strategies discussed in the context of other compounds provide a valuable foundation for exploring the potential delivery of phlorotannins through various nanocarriers and modifications.
Conclusion
Seaweeds exhibit remarkable versatility, holding significant importance across various sectors of the global market. They function as a valuable resource for environmental conservation, economic growth, and numerous industries. Capitalizing on their rich array of macro- and micro-nutrients, seaweeds present diverse and sustainable opportunities to enhance soil fertility, serve as constituents in animal feed, act as a renewable source of bioenergy, and contribute to the improvement of water quality. Moreover, beyond their pivotal role in environmental ecosystems, seaweeds merge as highly nutritious components, offering a broad spectrum of health benefits attributable to their distinctive composition of metabolites. This review has highlighted the generalized chemical properties of phlorotannins, a group of polyphenols derived from brown seaweeds. It covers their classification, biosynthesis pathway, and quantification among different seaweed species. Phlorotannins are promising candidates in the biomedical and nutraceutical sectors that provide safe and effective alternatives to synthetic counterparts, due to their remarkable biological activities, such as antioxidant, anti-inflammatory, anti-diabetic, anti-allergy/histamine, and anti-microbial/viral, which can be the basic mechanisms of further therapeutic applications. Despite their importance, few studies have focused on the absorption of phlorotannins. Current evidence suggests they have a bioavailability similar to terrestrial polyphenols, which hinders a comprehensive understanding of their interactions and underlying mechanisms within the human gastrointestinal tract and presenting a significant challenge for their prospective applications. To address these challenges, several innovative strategies, particularly encapsulation techniques, have been proposed to protect phlorotannins against gastrointestinal tract biotransformation and degradation. Additionally, phlorotannins can be released in a controlled and targeted manner through additional modifications, with the goal of maximizing their therapeutic potential. However, further in-depth research and exploration of these strategies are crucial to unlock the full potential of phlorotannins in various applications, including pharmaceuticals, functional foods, and nutraceuticals.
Abbreviations | ||
ABTS | = | 2,2-azino-bis-3-ethylbenzothiazoline-6-sulfonic acid |
Acetyl-CoA | = | acetyl coenzyme A |
AMPK | = | AMP-activated protein kinase |
BBB | = | blood-brain barrier |
CAT | = | catalase |
COX-2 | = | cycloocygenase-2 |
CUR | = | curcumin |
DDS | = | drug delivery system |
DMBA | = | 2,4-dimethoxybenzaldehyde assay |
DOX | = | doxorubicin |
DPPH | = | 2,2-diphenyl1-picryl-hydrazine-hydrate |
DW | = | dry mass |
EC50 | = | half maximal effective concentration |
EFSA | = | the European Food Safety Authority |
ERK | = | extracellular signal-regulated kinase |
FAO | = | Food and Agriculture Organization |
G6Pase | = | glucosde-6-phosphatase |
HAE | = | hydrothermal-assisted extraction |
HIV-1 | = | human immunodeficiency virus type 1 |
Hmox-1 | = | heme oxygenase-1 |
HPAE | = | high-pressure-assisted extraction |
HPLC | = | high-performance liquid chromatography |
H2O2 | = | hydrogen peroxide |
IC50 | = | half maximal inhibitory concentration |
Ig | = | immunoglobulin |
IL | = | interleukin |
iNOS | = | inducible nitric oxide synthase |
JNK | = | c-Jun N-terminal kinase |
LOX | = | lipoxygenases |
LPS | = | lipopolysaccharide |
MAE | = | microwave-assisted extraction |
MIC | = | minimum inhibitory concentration |
MDA | = | malondialdehyde |
MSPN | = | mesoporous silica nanoparticle |
MTT | = | 2,5-diphenyl-2H-tetrazolium bromide |
NADES | = | natural deep eutectic solvents |
NDA | = | the Panel on Dietetic Products, Nutrition and Allergies |
NF-κB | = | nuclear factor kappa B |
NMR | = | nuclear magnetic resonance |
NO | = | nitric oxide |
NOX | = | NADPH oxidase |
Nrf2 | = | nuclear factor erythroid 2-related factor 2 |
–OH | = | hydroxyl group |
PBA | = | Prussian blue assay |
PEG | = | polyethylene glycol |
PEO | = | poly(ethylene oxide) |
PEPCK | = | phosphoenolpyruvate carboxykinase |
PGD2 | = | prostaglandin D2 |
PGE2 | = | prostaglandin E2 |
PGH2 | = | prostaglandin H2 |
PGE | = | phloroglucinol equivalents |
P-gp | = | P-glycoprotein 1 |
PKB | = | protein kinase B |
PKS | = | polyketide synthase |
PTP1B | = | protein tyrosine phosphatase 1B |
PVP | = | polyvinylpyrrolidone |
ROS | = | reactive oxygen species |
SOD | = | superoxide dismutase |
SPION | = | superparamagnetic iron oxide nanoparticle |
T2DM | = | type 2 diabetes mellitus |
TNF-α | = | tumor necrosis factor alpha |
UAE | = | ultrasound-assisted extraction |
UMAE | = | ultrasound–microwave-assisted extraction |
Acknowledgments
We would like to express our gratitude to “The Future Food Hallmark Research Initiative” at the University of Melbourne, Australia. Furthermore, we extend our appreciation to the Honours, Master’s, PhD, and Postdoctoral researchers of Dr. Hafiz Suleria’s group from the School of Agriculture, Food and Ecosystem Sciences within the Faculty of Science at the University of Melbourne for their invaluable support.
Disclosure statement
No potential conflict of interest was reported by the authors.
Additional information
Funding
References
- Ahn, G., Y. Amagai, A. Matsuda, S.-M. Kang, W. Lee, K. Jung, K. Oida, H. Jang, S. Ishizaka, K. Matsuda, et al. 2015. Dieckol, a phlorotannin of Ecklonia cava, suppresses IgE-mediated mast cell activation and passive cutaneous anaphylactic reaction. Experimental Dermatology 24 (12):968–70. doi:10.1111/exd.12814.
- Ahn, M.-J., K.-D. Yoon, S.-Y. Min, J. S. Lee, J. H. Kim, T. G. Kim, S. H. Kim, N.-G. Kim, H. Huh, and J. Kim. 2004. Inhibition of HIV-1 reverse transcriptase and protease by phlorotannins from the brown alga Ecklonia cava. Biological & Pharmaceutical Bulletin 27 (4):544–7. doi:10.1248/bpb.27.544.
- Amanzadeh, E., A. Esmaeili, R. E. N. Abadi, N. Kazemipour, Z. Pahlevanneshan, and S. Beheshti. 2019. Quercetin conjugated with superparamagnetic iron oxide nanoparticles improves learning and memory better than free quercetin via interacting with proteins involved in LTP. Scientific Reports 9 (1):6876. doi:10.1038/s41598-019-43345-w.
- Amjadi, S., S. Gholizadeh, A. Ebrahimi, H. Almasi, H. Hamishehkar, and R. A. Taheri. 2022. Development and characterization of the carvone-loaded zein/pullulan hybrid electrospun nanofibers for food and medical applications. Industrial Crops and Products 183:114964. doi:10.1016/j.indcrop.2022.114964.
- Ank, G., B. A. P. da Gama, and R. C. Pereira. 2019. Latitudinal variation in phlorotannin contents from Southwestern Atlantic brown seaweeds. PeerJ. 7: E 7379. doi:10.7717/peerj.7379.
- Arnold, T., and N. Targett. 2003. To grow and defend: Lack of tradeoffs for brown algal phlorotannins. Oikos 100 (2):406–8. doi:10.1034/j.1600-0706.2003.11680.x.
- Aung, T. N., Z. Qu, R. D. Kortschak, and D. L. Adelson. 2017. Understanding the effectiveness of natural compound mixtures in cancer through their molecular mode of action. International Journal of Molecular Sciences 18 (3):656. doi:10.3390/ijms18030656.
- Bai, Y., X. Chen, and H. Qi. 2022. Characterization and bioactivity of phlorotannin loaded protein-polysaccharide nanocomplexes. Lwt 155:112998. doi:10.1016/j.lwt.2021.112998.
- Bai, Y., Y. Sun, Y. Gu, J. Zheng, C. Yu, and H. Qi. 2020. Preparation, characterization and antioxidant activities of kelp phlorotannin nanoparticles. Molecules 25 (19):4550. doi:10.3390/molecules25194550.
- Baldrick, F. R., K. McFadden, M. Ibars, C. Sung, T. Moffatt, K. Megarry, K. Thomas, P. Mitchell, J. M. W. Wallace, L. K. Pourshahidi, et al. 2018. Impact of a (poly) phenol-rich extract from the brown algae Ascophyllum nodosum on DNA damage and antioxidant activity in an overweight or obese population: A randomized controlled trial. The American Journal of Clinical Nutrition 108 (4):688–700. doi:10.1093/ajcn/nqy147.
- Banik, S., and A. Ghosh. 2021. The association of oxidative stress biomarkers with type 2 diabetes mellitus: A systematic review and meta-analysis. Health Science Reports 4 (4):e389. doi:10.1002/hsr2.389.
- Baskararaj, S., T. Panneerselvam, S. Govindaraj, S. Arunachalam, P. Parasuraman, S. R. K. Pandian, M. Sankaranarayanan, U. P. Mohan, P. Palanisamy, V. Ravishankar, et al. 2020. Formulation and characterization of folate receptor-targeted PEGylated liposome encapsulating bioactive compounds from Kappaphycus alvarezii for cancer therapy. 3 Biotech 10 (3):136. doi:10.1007/s13205-020-2132-7.
- Berglin, M., L. Delage, P. Potin, H. Vilter, and H. Elwing. 2004. Enzymatic cross-linking of a phenolic polymer extracted from the marine alga Fucus serratus. Biomacromolecules 5 (6):2376–83. doi:10.1021/bm0496864.
- Bertoni, G. 2013. A key step in phlorotannin biosynthesis revealed. The Plant Cell 25 (8):2770. doi:10.1105/tpc.113.250813.
- Bierie, B., and H. L. Moses. 2010. Transforming growth factor beta (TGF-β) and inflammation in cancer. Cytokine & Growth Factor Reviews 21 (1):49–59. doi:10.1016/j.cytogfr.2009.11.008.
- Birkemeyer, C., V. Lemesheva, S. Billig, and E. Tarakhovskaya. 2020. Composition of intracellular and cell wall-bound phlorotannin fractions in fucoid algae indicates specific functions of these metabolites dependent on the chemical structure. Metabolites 10 (9):369. doi:10.3390/metabo10090369.
- Blanz, M., P. Ascough, I. Mainland, P. Martin, M. A. Taggart, B. Dieterich, J. Wishart, K. L. Sayle, A. Raab, and J. Feldmann. 2019. Seaweed fertilisation impacts the chemical and isotopic composition of barley: Implications for analyses of archaeological skeletal remains. Journal of Archaeological Science 104:34–44. doi:10.1016/j.jas.2019.02.003.
- Botelho, J., V. Machado, Y. Leira, L. Proença, and J. J. Mendes. 2021. Periodontal inflamed surface area mediates the link between homocysteine and high blood pressure. Biomolecules 11 (6):875. doi:10.3390/biom11060875.
- Breijyeh, Z., B. Jubeh, and R. Karaman. 2020. Resistance of gram-negative bacteria to current antibacterial agents and approaches to resolve it. Molecules 25 (6):1340. doi:10.3390/molecules25061340.
- Cáceres-Jiménez, S., J. L. Ordóñez-Díaz, J. M. Moreno-Rojas, and G. Pereira-Caro. 2023. 9 - Bioaccesibility and bioavailability of marine polyphenols. In Marine phenolic compounds, ed. J. R. Pérez Correa, R. Mateos, and H. Domínguez, 265–98. Netherlands: Elsevier.
- Castro-Alves, V. C., and B. R. Cordenunsi. 2015. Total soluble phenolic compounds quantification is not as simple as it seems. Food Analytical Methods 8 (4):873–84. doi:10.1007/s12161-014-9961-0.
- Catarino, M. D., S. J. Amarante, N. Mateus, A. M. Silva, and S. M. Cardoso. 2021. Brown algae phlorotannins: A marine alternative to break the oxidative stress, inflammation and cancer network. Foods 10 (7):1478. doi:10.3390/foods10071478.
- Catarino, M. D., C. Marçal, T. Bonifácio-Lopes, D. Campos, N. Mateus, A. M. Silva, M. M. Pintado, and S. M. Cardoso. 2021. Impact of phlorotannin extracts from Fucus vesiculosus on human gut microbiota. Marine Drugs 19 (7):375. doi:10.3390/md19070375.
- Catarino, M. D., S. M. Pires, S. Silva, F. Costa, S. S. Braga, D. C. Pinto, A. M. Silva, and S. M. Cardoso. 2022. Overview of phlorotannins’ constituents in fucales. Marine Drugs 20 (12):754. doi:10.3390/md20120754.
- Catarino, M. D., A. Silva, M. T. Cruz, N. Mateus, A. M. Silva, and S. M. Cardoso. 2020. Phlorotannins from Fucus vesiculosus: Modulation of inflammatory response by blocking NF-κB signaling pathway. International Journal of Molecular Sciences 21 (18):6897. doi:10.3390/ijms21186897.
- Charoensiddhi, S., R. E. Abraham, P. Su, and W. Zhang. 2020. Seaweed and seaweed-derived metabolites as prebiotics. Advances in Food and Nutrition Research 91:97–156.
- Chen, J., Z. Zhou, P. Li, S. Ye, W. Li, M. Li, L. Zhu, and Y. Ding. 2023. Investigation of the potential phlorotannins and mechanism of six brown algae in treating type II diabetes mellitus based on biological activity, UPLC-QE-MS/MS, and network pharmacology. Foods 12 (16):3000. doi:10.3390/foods12163000.
- Cho, H. M., T. P. Doan, T. K. Q. Ha, H. W. Kim, B. W. Lee, H. T. T. Pham, T. O. Cho, and W. K. Oh. 2019. Dereplication by high-performance liquid chromatography (HPLC) with quadrupole-time-of-flight mass spectroscopy (qTOF-MS) and antiviral activities of phlorotannins from Ecklonia cava. Marine Drugs 17 (3):149. doi:10.3390/md17030149.
- Chopin, T., and A. G. Tacon. 2021. Importance of seaweeds and extractive species in global aquaculture production. Reviews in Fisheries Science & Aquaculture 29 (2):139–48. doi:10.1080/23308249.2020.1810626.
- Chouh, A., T. Nouadri, M. D. Catarino, A. M. Silva, and S. M. Cardoso. 2022. Phlorotannins of the Brown algae Sargassum vulgare from the Mediterranean Sea coast. Antioxidants 11 (6):1055. doi:10.3390/antiox11061055.
- Chowdhury, M. T. H., I. Bangoura, J.-Y. Kang, N.-G. Park, D.-H. Ahn, and Y.-K. Hong. 2011. Distribution of phlorotannins in the brown alga Ecklonia cava and comparison of pretreatments for extraction. Fisheries and Aquatic Sciences 14 (3):198–204. doi:10.5657/FAS.2011.0198.
- Corona, G., M. M. Coman, Y. Guo, S. Hotchkiss, C. Gill, P. Yaqoob, J. P. Spencer, and I. Rowland. 2017. Effect of simulated gastrointestinal digestion and fermentation on polyphenolic content and bioactivity of brown seaweed phlorotannin-rich extracts. Molecular Nutrition & Food Research 61 (11):1700223. doi:10.1002/mnfr.201700223.
- Corona, G., Y. Ji, P. Anegboonlap, S. Hotchkiss, C. Gill, P. Yaqoob, J. P. Spencer, and I. Rowland. 2016. Gastrointestinal modifications and bioavailability of brown seaweed phlorotannins and effects on inflammatory markers. The British Journal of Nutrition 115 (7):1240–53. doi:10.1017/S0007114516000210.
- Cui, Y., J.-Y. Park, J. Wu, J. H. Lee, Y.-S. Yang, M.-S. Kang, S.-C. Jung, J. M. Park, E.-S. Yoo, S.-H. Kim, et al. 2015. Dieckol attenuates microglia-mediated neuronal cell death via ERK, Akt and NADPH oxidase-mediated pathways. The Korean Journal of Physiology & Pharmacology: Official Journal of the Korean Physiological Society and the Korean Society of Pharmacology 19 (3):219–28. doi:10.4196/kjpp.2015.19.3.219.
- Dal Canto, E., A. Ceriello, L. Rydén, M. Ferrini, T. B. Hansen, O. Schnell, E. Standl, and J. W. Beulens. 2019. Diabetes as a cardiovascular risk factor: An overview of global trends of macro and micro vascular complications. European Journal of Preventive Cardiology 26 (2_suppl):25–32. doi:10.1177/2047487319878371.
- Debbarma, J., P. Viji, B. M. Rao, and C. Ravishankar. 2022. Seaweeds: Potential applications of the aquatic vegetables to augment nutritional composition, texture, and health benefits of food and food products. In Sustainable global resources of seaweeds volume 2: Food, pharmaceutical and health applications, 3– 54. Ranga Rao, A., & Ravishankar, G.A., Eds., Springer, Cham, Switzerland.
- Deepika, C., J. Wolf, N. Moheimani, B. Hankamer, B. von Herzen, and A. R. Rao. 2022. Utilisation of seaweeds in the Australian market–commercialisation strategies: Current trends and future prospects. In Sustainable global resources of seaweeds volume 1: Bioresources, cultivation, trade and multifarious applications, 265–94. Ranga Rao, A., & Ravishankar, G.A., Ed., Springer, Cham, Switzerland.
- Dong, X., Y. Bai, Z. Xu, Y. Shi, Y. Sun, S. Janaswamy, C. Yu, and H. Qi. 2019. Phlorotannins from Undaria pinnatifida sporophyll: Extraction, antioxidant, and anti-inflammatory activities. Marine Drugs 17 (8):434. doi:10.3390/md17080434.
- Duarte, C. M., A. Bruhn, and D. Krause-Jensen. 2021. A seaweed aquaculture imperative to meet global sustainability targets. Nature Sustainability 5 (3):185–93. doi:10.1038/s41893-021-00773-9.
- Dutot, M., R. Fagon, M. Hemon, and P. Rat. 2012. Antioxidant, anti-inflammatory, and anti-senescence activities of a phlorotannin-rich natural extract from brown seaweed Ascophyllum nodosum. Applied Biochemistry and Biotechnology 167 (8):2234–40. doi:10.1007/s12010-012-9761-1.
- Elbialy, N. S., S. F. Aboushoushah, B. F. Sofi, and A. Noorwali. 2020. Multifunctional curcumin-loaded mesoporous silica nanoparticles for cancer chemoprevention and therapy. Microporous and Mesoporous Materials 291:109540. doi:10.1016/j.micromeso.2019.06.002.
- El-Manaway, I. M., and S. H. Rashedy. 2022. The ecology and physiology of seaweeds: An overview. In Sustainable global resources of seaweeds volume 1: Bioresources, cultivation, trade and multifarious applications, 3–16. Ranga Rao, A., & Ravishankar, G.A., Eds., Springer, Cham, Switzerland.
- Emeline, C. B., D. Ludovic, V. Laurent, L. Catherine, I. Kruse, A. G. Erwan, W. Florian, and P. Philippe. 2021. Induction of phlorotannins and gene expression in the brown macroalga Fucus vesiculosus in response to the herbivore Littorina littorea. Marine Drugs 19 (4):185. doi:10.3390/md19040185.
- Erpel, F., C. Camilo, R. Mateos, and J. R. Pérez-Correa. 2023. A macroporous resin purification process to obtain food-grade phlorotannin-rich extracts with α-glucosidase inhibitory activity from Chilean brown seaweeds: An UHPLC-MSn profiling. Food Chemistry 402:134472. doi:10.1016/j.foodchem.2022.134472.
- Erpel, F., R. Mateos, J. Pérez-Jiménez, and J. R. Pérez-Correa. 2020. Phlorotannins: From isolation and structural characterization, to the evaluation of their antidiabetic and anticancer potential. Food Research International 137:109589. doi:10.1016/j.foodres.2020.109589.
- Falkowski, P. 2012. Ocean science: The power of plankton. Nature 483 (7387):S17–S20. doi:10.1038/483S17a.
- FAO. 2018. The global status of seaweed production, trade and utilization. Globefish Research Programme 124:120. FAO: Rome.
- FAO (Food and Agriculture Organization). 2022. The state of world fisheries and aquaculture. Towards blue transformation. Rome: FAO.
- Feng, H., M. Li, Z. Xing, X-k Ouyang, and J. Ling. 2022. Efficient delivery of fucoxanthin using metal–polyphenol network-coated magnetic mesoporous silica. Journal of Drug Delivery Science and Technology 77:103842. doi:10.1016/j.jddst.2022.103842.
- Fernando, I. P. S., W. Lee, and G. Ahn. 2022. Marine algal flavonoids and phlorotannins; an intriguing frontier of biofunctional secondary metabolites. Critical Reviews in Biotechnology 42 (1):23–45. doi:10.1080/07388551.2021.1922351.
- Ferreira, C. A., A. P. Januário, R. Félix, N. Alves, M. F. Lemos, and J. R. Dias. 2021. Multifunctional gelatin/chitosan electrospun wound dressing dopped with Undaria pinnatifida phlorotannin-enriched extract for skin regeneration. Pharmaceutics 13 (12):2152. doi:10.3390/pharmaceutics13122152.
- Ford, L., C. Curry, M. Campbell, K. Theodoridou, G. Sheldrake, J. Dick, L. Stella, and P. J. Walsh. 2020. Effect of phlorotannins from brown seaweeds on the in vitro digestibility of pig feed. Animals 10 (11):2193. doi:10.3390/ani10112193.
- Ford, L., K. Theodoridou, G. N. Sheldrake, and P. J. Walsh. 2019. A critical review of analytical methods used for the chemical characterisation and quantification of phlorotannin compounds in brown seaweeds. Phytochemical Analysis: PCA 30 (6):587–99. doi:10.1002/pca.2851.
- Garcia-Vaquero, M., R. Ravindran, O. Walsh, J. O’Doherty, A. K. Jaiswal, B. K. Tiwari, and G. Rajauria. 2021. Evaluation of ultrasound, microwave, ultrasound–microwave, hydrothermal and high pressure assisted extraction technologies for the recovery of phytochemicals and antioxidants from brown macroalgae. Marine Drugs 19 (6):309. doi:10.3390/md19060309.
- Gheda, S., R. A. Hamouda, M. A. Naby, T. M. Mohamed, T. M. Al-Shaikh, and A. Khamis. 2023. Potent effect of phlorotannins derived from Sargassum linifolium as antioxidant and antidiabetic in a streptozotocin-induced diabetic rats model. Applied Sciences 13 (8):4711. doi:10.3390/app13084711.
- Gheda, S., M. A. Naby, T. Mohamed, L. Pereira, and A. Khamis. 2021. Antidiabetic and antioxidant activity of phlorotannins extracted from the brown seaweed Cystoseira compressa in streptozotocin-induced diabetic rats. Environmental Science and Pollution Research 28 (18):22886–901. doi:10.1007/s11356-021-12347-5.
- Ghosh, S., T. Sarkar, S. Pati, Z. A. Kari, H. A. Edinur, and R. Chakraborty. 2022. Novel bioactive compounds from marine sources as a tool for functional food development. Frontiers in Marine Science 9:76. doi:10.3389/fmars.2022.832957.
- Glombitza, K.-W., and G. Gerstberger. 1985. Phlorotannins with dibenzodioxin structural elements from the brown alga Eisenia arborea. Phytochemistry 24 (3):543–51. doi:10.1016/S0031-9422(00)80764-5.
- Glombitza, K.-W., and A. Schmidt. 1999. Nonhalogenated and halogenated phlorotannins from the brown alga Carpophyllum angustifolium. Journal of Natural Products 62 (9):1238–40. doi:10.1021/np990076c.
- Gómez, I., and P. Huovinen. 2020. Brown algal phlorotannins: An overview of their functional roles. In Antarctic seaweeds: Diversity, adaptation and ecosystem services, 365–88. Gómez, I., & Huovinen, P., Eds., Springer, Cham, Switzerland.
- Guan, L., H. Long, F. Ren, Y. Li, and H. Zhang. 2022. A structure—Activity relationship study of the inhibition of α-amylase by benzoic acid and its derivatives. Nutrients 14 (9):1931. doi:10.3390/nu14091931.
- Guiry, M. D. 2012. How many species of algae are there? Journal of Phycology 48 (5):1057–63. doi:10.1111/j.1529-8817.2012.01222.x.
- Gümüş Yılmaz, G., J. L. Gómez Pinchetti, A. Cifuentes, M. Herrero, and E. Ibáñez. 2019. Comparison of extraction techniques and surfactants for the isolation of total polyphenols and phlorotannins from the brown algae Lobophora variegata. Analytical Letters 52 (17):2724–40. doi:10.1080/00032719.2019.1597878.
- Gunathilaka, T., L. R. Keertihirathna, and D. Peiris. 2021. Advanced pharmacological uses of marine algae as an anti-diabetic therapy. In Natural Medicinal Plants, 79–95. El-Shemy, H., IntechOpen, London, UK.
- Gupta, V., S. Bhavanasi, M. Quadir, K. Singh, G. Ghosh, K. Vasamreddy, A. Ghosh, T. J. Siahaan, S. Banerjee, and S. K. Banerjee. 2019. Protein PEGylation for cancer therapy: Bench to bedside. Journal of Cell Communication and Signaling 13 (3):319–30. doi:10.1007/s12079-018-0492-0.
- Ha, J. W., H. Song, S. S. Hong, and Y. C. Boo. 2019. Marine alga Ecklonia cava extract and dieckol attenuate prostaglandin E2 production in HaCaT keratinocytes exposed to airborne particulate matter. Antioxidants 8 (6):190. doi:10.3390/antiox8060190.
- Han, E. J., H.-S. Kim, K. Sanjeewa, K. Herath, Y.-J. Jeon, Y. Jee, J. Lee, T. Kim, S.-Y. Shim, and G. Ahn. 2020. Eckol from Ecklonia cava suppresses immunoglobulin E-mediated mast cell activation and passive cutaneous anaphylaxis in mice. Nutrients 12 (5):1361. doi:10.3390/nu12051361.
- Hari, S. K., A. Gauba, N. Shrivastava, R. M. Tripathi, S. K. Jain, and A. K. Pandey. 2023. Polymeric micelles and cancer therapy: An ingenious multimodal tumor-targeted drug delivery system. Drug Delivery and Translational Research 13 (1):135–63. doi:10.1007/s13346-022-01197-4.
- Heo, S.-J., S.-H. Cha, K.-N. Kim, S.-H. Lee, G. Ahn, D.-H. Kang, C. Oh, Y.-U. Choi, A. Affan, D. Kim, et al. 2012. Neuroprotective effect of phlorotannin isolated from Ishige okamurae against H 2 O 2-induced oxidative stress in murine hippocampal neuronal cells, HT22. Applied Biochemistry and Biotechnology 166 (6):1520–32. doi:10.1007/s12010-012-9545-7.
- Hermund, D. B., H. Torsteinsen, J. Vega, F. L. Figueroa, and C. Jacobsen. 2022. Screening for new cosmeceuticals from brown algae Fucus vesiculosus with antioxidant and photo-protecting properties. Marine Drugs 20 (11):687. doi:10.3390/md20110687.
- Hessami, M. J., A. R. Rao, and G. A. Ravishankar. 2019. Opportunities and challenges in seaweeds as feed stock for biofuel production. In Handbook of algal technologies and phytochemicals, 39–50. Ravishankar, G. A, & Ambati, R.R., Eds., CRC Press, USA.
- Hierholtzer, A., L. Chatellard, M. Kierans, J. C. Akunna, and P. J. Collier. 2013. The impact and mode of action of phenolic compounds extracted from brown seaweed on mixed anaerobic microbial cultures. Journal of Applied Microbiology 114 (4):964–73. doi:10.1111/jam.12114.
- Hodhodi, A., A. Babakhani, and H. Rostamzad. 2022. Effect of different extraction conditions on phlorotannin content and antioxidant activity of extract from brown algae (Sargassum angustifolium). Journal of Food Processing and Preservation 46 (3):e16307. doi:10.1111/jfpp.16307.
- Hu, C., and S. Ma. 2018. Recent development of lipoxygenase inhibitors as anti-inflammatory agents. MedChemComm 9 (2):212–25. doi:10.1039/c7md00390k.
- Iken, K., C. D. Amsler, J. M. Hubbard, J. B. McClintock, and B. J. Baker. 2007. Allocation patterns of phlorotannins in Antarctic brown algae. Phycologia 46 (4):386–95. doi:10.2216/06-67.1.
- Im, J., C. H. Choi, F. Mun, J. Lee, H. Kim, W.-K. Jung, C. H. Jang, and G. Kim. 2017. A polycaprolactone/fish collagen/alginate biocomposite supplemented with phlorotannin for hard tissue regeneration. RSC Advances 7 (4):2009–18. doi:10.1039/C6RA25182J.
- Ismail, M. M., G. M. El Zokm, A. M. El Sikaily, A. I. Selim, and G. A. Ismail. 2023. Chemodiversity and bioactivity assessment of phlorotannins from some Phaeophyta species from the Red Sea. Journal of Applied Phycology 35 (4):1769–88. doi:10.1007/s10811-023-03000-4.
- Jégou, C., N. Kervarec, S. Cérantola, I. Bihannic, and V. Stiger-Pouvreau. 2015. NMR use to quantify phlorotannins: The case of Cystoseira tamariscifolia, a phloroglucinol-producing brown macroalga in Brittany (France). Talanta 135:1–6. doi:10.1016/j.talanta.2014.11.059.
- Jun, Y.-J., M. Lee, T. Shin, N. Yoon, J.-H. Kim, and H.-R. Kim. 2014. Eckol enhances heme oxygenase-1 expression through activation of Nrf2/JNK pathway in HepG2 cells. Molecules 19 (10):15638–52. doi:10.3390/molecules191015638.
- Junning, C., and Giulia, G. 2021. Global seaweeds and microalgae production, 1950–2019. Food and Agriculture Organization of the United Nations, Rome.
- Kalyane, D., N. Raval, R. Maheshwari, V. Tambe, K. Kalia, and R. K. Tekade. 2019. Employment of enhanced permeability and retention effect (EPR): Nanoparticle-based precision tools for targeting of therapeutic and diagnostic agent in cancer. Materials Science & Engineering. C, Materials for Biological Applications 98:1252–76. doi:10.1016/j.msec.2019.01.066.
- Kang, M.-C., W. A. J. P. Wijesinghe, S.-H. Lee, S.-M. Kang, S.-C. Ko, X. Yang, N. Kang, B.-T. Jeon, J. Kim, D.-H. Lee, et al. 2013. Dieckol isolated from brown seaweed Ecklonia cava attenuates type ІІ diabetes in db/db mouse model. Food and Chemical Toxicology 53:294–8. doi:10.1016/j.fct.2012.12.012.
- Karonen, M. 2022. Insights into polyphenol–lipid interactions: Chemical methods, molecular aspects and their effects on membrane structures. Plants 11 (14):1809. doi:10.3390/plants11141809.
- Karthikeyan, A., A. Joseph, and B. G. Nair. 2022. Promising bioactive compounds from the marine environment and their potential effects on various diseases. Journal of Genetic Engineering and Biotechnology 20 (1):1–38. doi:10.1186/s43141-021-00290-4.
- Kellogg, J., M. H. Grace, and M. A. Lila. 2014. Phlorotannins from Alaskan seaweed inhibit carbolytic enzyme activity. Marine Drugs 12 (10):5277–94. doi:10.3390/md12105277.
- Kierstead, P. H., H. Okochi, V. J. Venditto, T. C. Chuong, S. Kivimae, J. M. Fréchet, and F. C. Szoka. 2015. The effect of polymer backbone chemistry on the induction of the accelerated blood clearance in polymer modified liposomes. Journal of Controlled Release 213:1–9. doi:10.1016/j.jconrel.2015.06.023.
- Kim, A. D., K. A. Kang, M. J. Piao, K. C. Kim, J. Zheng, C. W. Yao, J. W. Cha, C. L. Hyun, H. K. Kang, N. H. Lee, et al. 2014. Cytoprotective effect of Eckol against oxidative stress-induced mitochondrial dysfunction: Involvement of the Foxo3a/Ampk pathway. Journal of Cellular Biochemistry 115 (8):1403–11. doi:10.1002/jcb.24790.
- Kim, H.-J., C. Dasagrandhi, S.-H. Kim, B.-G. Kim, S.-H. Eom, and Y.-M. Kim. 2018. In vitro antibacterial activity of phlorotannins from edible brown algae, Eisenia bicyclis against streptomycin-resistant Listeria monocytogenes. Indian Journal of Microbiology 58 (1):105–8. doi:10.1007/s12088-017-0693-x.
- Kim, K. C., K. A. Kang, R. Zhang, M. J. Piao, G. Y. Kim, M. Y. Kang, S. J. Lee, N. H. Lee, Y.-J. Surh, and J. W. Hyun. 2010. Up-regulation of Nrf2-mediated heme oxygenase-1 expression by eckol, a phlorotannin compound, through activation of Erk and PI3K/Akt. The International Journal of Biochemistry & Cell Biology 42 (2):297–305. doi:10.1016/j.biocel.2009.11.009.
- Kim, S., S.-S. Kang, S.-I. Choi, G.-H. Kim, and J.-Y. Imm. 2019. Ecklonia cava extract containing dieckol suppresses RANKL-induced osteoclastogenesis via MAP kinase/NF-κB pathway inhibition and heme oxygenase-1 induction. Journal of Microbiology and Biotechnology 29 (1):11–20.
- Koivikko, R., J. Eränen, J. Loponen, and V. Jormalainen. 2008. Variation of phlorotannins among three populations of Fucus vesiculosus as revealed by HPLC and colorimetric quantification. Journal of Chemical Ecology 34 (1):57–64. doi:10.1007/s10886-007-9410-2.
- Kord, A., Y. Foudil-Cherif, M. Amiali, A. Boumechhour, and R. Benfares. 2021. Phlorotannins composition, radical scavenging capacity and reducing power of phenolics from the brown alga Cystoseira sauvageauana. Journal of Aquatic Food Product Technology 30 (4):426–38. doi:10.1080/10498850.2021.1895392.
- Koren, S., and I. G. Fantus. 2007. Inhibition of the protein tyrosine phosphatase PTP1B: Potential therapy for obesity, insulin resistance and type-2 diabetes mellitus. Best Practice & Research. Clinical Endocrinology & Metabolism 21 (4):621–40. doi:10.1016/j.beem.2007.08.004.
- Kumar, L. R., P. T. Paul, K. Anas, C. Tejpal, N. Chatterjee, T. Anupama, S. Mathew, and C. Ravishankar. 2022. Phlorotannins–Bioactivity and extraction perspectives. Journal of Applied Phycology 34 (4):2173–85. doi:10.1007/s10811-022-02749-4.
- Kurihara, H., R. Konno, and K. Takahashi. 2015. Fucophlorethol C, a phlorotannin as a lipoxygenase inhibitor. Bioscience, Biotechnology, and Biochemistry 79 (12):1954–6. doi:10.1080/09168451.2015.1062716.
- Le, Q.-T., Y. Li, Z.-J. Qian, M.-M. Kim, and S.-K. Kim. 2009. Inhibitory effects of polyphenols isolated from marine alga Ecklonia cava on histamine release. Process Biochemistry 44 (2):168–76. doi:10.1016/j.procbio.2008.10.002.
- Lee, H.-A., J.-H. Lee, and J.-S. Han. 2017. A phlorotannin constituent of Ecklonia cava alleviates postprandial hyperglycemia in diabetic mice. Pharmaceutical Biology 55 (1):1149–54. doi:10.1080/13880209.2017.1291693.
- Lee, S.-H., S.-C. Ko, M.-C. Kang, D. H. Lee, and Y.-J. Jeon. 2016. Octaphlorethol A, a marine algae product, exhibits antidiabetic effects in type 2 diabetic mice by activating AMP-activated protein kinase and upregulating the expression of glucose transporter 4. Food and Chemical Toxicology 91:58–64. doi:10.1016/j.fct.2016.02.022.
- Le Lann, K., G. Surget, C. Couteau, L. Coiffard, S. Cérantola, F. Gaillard, M. Larnicol, M. Zubia, F. Guérard, N. Poupart, et al. 2016. Sunscreen, antioxidant, and bactericide capacities of phlorotannins from the brown macroalga Halidrys siliquosa. Journal of Applied Phycology 28 (6):3547–59. doi:10.1007/s10811-016-0853-0.
- Lemesheva, V., C. Birkemeyer, D. Garbary, and E. Tarakhovskaya. 2020. Vanadium-dependent haloperoxidase activity and phlorotannin incorporation into the cell wall during early embryogenesis of Fucus vesiculosus (Phaeophyceae). European Journal of Phycology 55 (3):275–84. doi:10.1080/09670262.2019.1709131.
- Li, C., B. Li, C. Zhu, and X. Meng. 2020. Modeling and optimization of tea polyphenol-alginate/chitosan magnetic microcapsules. Journal of Molecular Structure 1208:127827. doi:10.1016/j.molstruc.2020.127827.
- Li, Y., X. Fu, D. Duan, X. Liu, J. Xu, and X. Gao. 2017. Extraction and identification of phlorotannins from the brown alga, Sargassum fusiforme (Harvey) Setchell. Marine Drugs 15 (2):49. doi:10.3390/md15020049.
- Li, Y., M. Kröger, and W. K. Liu. 2014. Endocytosis of PEGylated nanoparticles accompanied by structural and free energy changes of the grafted polyethylene glycol. Biomaterials 35 (30):8467–78. doi:10.1016/j.biomaterials.2014.06.032.
- Li, Y., S.-H. Lee, Q.-T. Le, M.-M. Kim, and S.-K. Kim. 2008. Anti-allergic effects of phlorotannins on histamine release via binding inhibition between IgE and FcεRI. Journal of Agricultural and Food Chemistry 56 (24):12073–80. doi:10.1021/jf802732n.
- Liu, X., and C. L. Bourvellec. 2023. 7 - Interactions with other macromolecules. In Marine phenolic compounds, ed. J. R. Pérez-Correa, R. Mateos, and H. Domínguez, 219–38. Elsevier, Netherlands.
- Liu, X., W. Yuan, R. Sharma-Shivappa, and J. van Zanten. 2017. Antioxidant activity of phlorotannins from brown algae. International Journal of Agricultural and Biological Engineering 10 (6):184–91. doi:10.25165/j.ijabe.20171006.2854.
- Lobine, D., K. R. Rengasamy, and M. F. Mahomoodally. 2022. Functional foods and bioactive ingredients harnessed from the ocean: Current status and future perspectives. Critical Reviews in Food Science and Nutrition 62 (21):5794–823. doi:10.1080/10408398.2021.1893643.
- Lopes, G., M. Barbosa, P. B. Andrade, and P. Valentão. 2019. Phlorotannins from Fucales: Potential to control hyperglycemia and diabetes-related vascular complications. Journal of Applied Phycology 31 (5):3143–52. doi:10.1007/s10811-019-01816-7.
- Lopes, G., E. Pinto, P. B. Andrade, and P. Valentao. 2013. Antifungal activity of phlorotannins against dermatophytes and yeasts: Approaches to the mechanism of action and influence on Candida albicans virulence factor. PLoS One 8 (8):e72203. doi:10.1371/journal.pone.0072203.
- Lopes, G., C. Sousa, L. R. Silva, E. Pinto, P. B. Andrade, J. Bernardo, T. Mouga, and P. Valentão. 2012. Can phlorotannins purified extracts constitute a novel pharmacological alternative for microbial infections with associated inflammatory conditions? PLoS One 7 (2):e31145. doi:10.1371/journal.pone.0031145.
- Lo Piparo, E., H. Scheib, N. Frei, G. Williamson, M. Grigorov, and C. J. Chou. 2008. Flavonoids for controlling starch digestion: Structural requirements for inhibiting human α-amylase. Journal of Medicinal Chemistry 51 (12):3555–61. doi:10.1021/jm800115x.
- Magarkar, A., E. Karakas, M. Stepniewski, T. Rog, and A. Bunker. 2012. Molecular dynamics simulation of PEGylated bilayer interacting with salt ions: A model of the liposome surface in the bloodstream. The Journal of Physical Chemistry. B 116 (14):4212–9. doi:10.1021/jp300184z.
- Mandola, A., A. Nozawa, and T. Eiwegger. 2019. Histamine, histamine receptors, and anti-histamines in the context of allergic responses. LymphoSign Journal 6 (2):35–51. doi:10.14785/lymphosign-2018-0016.
- Margraf, T., A. R. Karnopp, N. D. Rosso, and D. Granato. 2015. Comparison between Folin-Ciocalteu and Prussian Blue assays to estimate the total phenolic content of juices and teas using 96-well microplates. Journal of Food Science 80 (11):C2397–C2403. doi:10.1111/1750-3841.13077.
- Matsui, T., C. Ito, M. Itoigawa, and T. Shibata. 2022. Three phlorotannins from Sargassum carpophyllum are effective against the secretion of allergic mediators from antigen-stimulated rat basophilic leukemia cells. Food Chemistry 377:131992. doi:10.1016/j.foodchem.2021.131992.
- Meng, W., T. Mu, H. Sun, and M. Garcia-Vaquero. 2021. Phlorotannins: A review of extraction methods, structural characteristics, bioactivities, bioavailability, and future trends. Algal Research 60:102484. doi:10.1016/j.algal.2021.102484.
- Meshalkina, D., E. Tsvetkova, A. Orlova, R. Islamova, M. Grashina, D. Gorbach, V. Babakov, A. Francioso, C. Birkemeyer, L. Mosca, et al. 2023. First insight into the neuroprotective and antibacterial effects of phlorotannins isolated from the cell walls of brown algae Fucus vesiculosus and Pelvetia canaliculata. Antioxidants 12 (3):696. doi:10.3390/antiox12030696.
- Meslet-Cladière, L., L. Delage, C. J.-J. Leroux, S. Goulitquer, C. Leblanc, E. Creis, E. A. Gall, V. Stiger-Pouvreau, M. Czjzek, and P. Potin. 2013. Structure/function analysis of a type III polyketide synthase in the brown alga Ectocarpus siliculosus reveals a biochemical pathway in phlorotannin monomer biosynthesis. The Plant Cell 25 (8):3089–103. doi:10.1105/tpc.113.111336.
- Mirchandani, Y., V. B. Patravale, and S. Brijesh. 2021. Solid lipid nanoparticles for hydrophilic drugs. Journal of Controlled Release 335:457–64. doi:10.1016/j.jconrel.2021.05.032.
- Mittal, A., I. Roy, and S. Gandhi. 2022. Magnetic nanoparticles: An overview for biomedical applications. Magnetochemistry 8 (9):107. doi:10.3390/magnetochemistry8090107.
- Moheimanian, N., H. Mirkhani, A. Purkhosrow, J. Sohrabipour, and A. R. Jassbi. 2023. In vitro and in vivo antidiabetic, α-glucosidase inhibition and antibacterial activities of three brown algae, Polycladia myrica, Padina antillarum, and Sargassum boveanum, and a red alga, Palisada perforata from the Persian Gulf. Innovative Journal of Pharmaceutical Research 22 (1):e133731.
- Morrison, D. K. 2012. MAP kinase pathways. Cold Spring Harbor Perspectives in Biology 4 (11):a011254. doi:10.1101/cshperspect.a011254.
- Mozafari, M. R. 2010. Nanoliposomes: Preparation and analysis. In Liposomes: Methods and protocols, volume 1: Pharmaceutical nanocarriers, 29–50. Weissig, V., Ed., Human Press, Totowa, NJ, USA.
- Muhammad, K., J. Zhao, I. Ullah, J. Guo, X-k Ren, and Y. Feng. 2019. Ligand targeting and peptide functionalized polymers as non-viral carriers for gene therapy. Biomaterials Science 8 (1):64–83. doi:10.1039/c9bm01112a.
- Murray, M., A. L. Dordevic, K. Cox, A. Scholey, L. Ryan, and M. P. Bonham. 2021. Twelve weeks’ treatment with a polyphenol-rich seaweed extract increased HDL cholesterol with no change in other biomarkers of chronic disease risk in overweight adults: A placebo-controlled randomized trial. The Journal of Nutritional Biochemistry 96:108777. doi:10.1016/j.jnutbio.2021.108777.
- Murray, M., A. L. Dordevic, L. Ryan, and M. P. Bonham. 2019. A single-dose of a polyphenol-rich Fucus vesiculosus extract is insufficient to blunt the elevated postprandial blood glucose responses exhibited by healthy adults in the evening: A randomised crossover trial. Antioxidants 8 (2):49. doi:10.3390/antiox8020049.
- Nakamura, T., S. Maeda, K. Horiguchi, T. Maehara, K. Aritake, B-i Choi, Y. Iwakura, Y. Urade, and T. Murata. 2015. PGD2 deficiency exacerbates food antigen-induced mast cell hyperplasia. Nature Communications 6 (1):7514. doi:10.1038/ncomms8514.
- Nazzaro, F., F. Fratianni, A. d’Acierno, V. De Feo, F. J. Ayala-Zavala, A. Gomes-Cruz, D. Granato, and R. Coppola. 2019. Effect of polyphenols on microbial cell-cell communications. In Quorum sensing, 195–223. Tommonaro, G., Ed., Academic Press, Netherlands.
- Obluchinskaya, E. D., A. Daurtseva, O. Pozharitskaya, E. Flisyuk, and A. Shikov. 2019. Natural deep eutectic solvents as alternatives for extracting phlorotannins from brown algae. Pharmaceutical Chemistry Journal 53 (3):243–7. doi:10.1007/s11094-019-01987-0.
- Obluchinskaya, E. D., O. N. Pozharitskaya, D. V. Zakharov, E. V. Flisyuk, I. I. Terninko, Y. E. Generalova, I. E. Smekhova, and A. N. Shikov. 2022. The biochemical composition and antioxidant properties of Fucus vesiculosus from the Arctic region. Marine Drugs 20 (3):193. doi:10.3390/md20030193.
- Park, E., H. Yu, J.-H. Lim, J. H. Choi, K.-J. Park, and J. Lee. 2023. Seaweed metabolomics: A review on its nutrients, bioactive compounds and changes in climate change. Food Research International 163:112221. doi:10.1016/j.foodres.2022.112221.
- Park, J.-Y., J. H. Kim, J. M. Kwon, H.-J. Kwon, H. J. Jeong, Y. M. Kim, D. Kim, W. S. Lee, and Y. B. Ryu. 2013. Dieckol, a SARS-CoV 3CLpro inhibitor, isolated from the edible brown algae Ecklonia cava. Bioorganic & Medicinal Chemistry 21 (13):3730–7. doi:10.1016/j.bmc.2013.04.026.
- Patel, A. K., A. P. Vadrale, R. R. Singhania, P. Michaud, A. Pandey, S.-J. Chen, C.-W. Chen, and C.-D. Dong. 2023. Algal polysaccharides: Current status and future prospects. Phytochemistry Reviews 22 (4):1167–96. doi:10.1007/s11101-021-09799-5.
- Pelletreau, K. N. 2008. The application of molecular tools towards the study of brown algal chemical ecology and the production of phlorotannins. University of Delaware, USA.
- Pereira, L. 2016. Edible seaweeds of the world, CRC Press, Boca Raton, USA.
- Petchidurai, G., J. A. Nagoth, M. S. John, K. Sahayaraj, N. Murugesan, and S. Pucciarelli. 2019. Standardization and quantification of total tannins, condensed tannin and soluble phlorotannins extracted from thirty-two drifted coastal macroalgae using high performance liquid chromatography. Bioresource Technology Reports 7:100273. doi:10.1016/j.biteb.2019.100273.
- Phang, S. J., H. X. Teh, M. L. Looi, B. Arumugam, M. B. Fauzi, and U. R. Kuppusamy. 2023. Phlorotannins from brown algae: A review on their antioxidant mechanisms and applications in oxidative stress-mediated diseases. Journal of Applied Phycology 35 (2):867–92. doi:10.1007/s10811-023-02913-4.
- Phang-Lyn, S., and V. A. Llerena. 2019. Biochemistry, biotransformation, StatPearls, Treasure Island, FL.
- Phasanasophon, K., and S. M. Kim. 2019. Anti-inflammatory activity of the phlorotannin trifuhalol A using LPS-stimulated RAW264. 7 cells through NF-κB and MAPK main signaling pathways. Natural Product Communications 14 (5):1934578X19849798. doi:10.1177/1934578X19849798.
- Pradhan, B., R. Nayak, P. P. Bhuyan, S. Patra, C. Behera, S. Sahoo, J.-S. Ki, A. Quarta, A. Ragusa, and M. Jena. 2022. Algal phlorotannins as novel antibacterial agents with reference to the antioxidant modulation: Current advances and future directions. Marine Drugs 20 (6):403. doi:10.3390/md20060403.
- Price, G., and D. A. Patel. 2021. Drug bioavailability. In StatPearls [Internet]. Statpearls Publishing, Treasure Island, FL.
- Priya, S., V. M. Desai, and G. Singhvi. 2023. Surface modification of lipid-based nanocarriers: A potential approach to enhance targeted drug delivery. ACS Omega 8 (1):74–86. doi:10.1021/acsomega.2c05976.
- Raja, R., S. Hemaiswarya, K. Arunkumar, N. Mathiyazhagan, S. Kandasamy, A. Arun, and P. Ramasamy. 2023. Efficacy of Eisenia bicyclis phlorotannins in the treatment of diabetes and reducing inflammation. Food Bioscience 52:102381. doi:10.1016/j.fbio.2023.102381.
- Rajan, D. K., K. Mohan, S. Zhang, and A. R. Ganesan. 2021. Dieckol: A brown algal phlorotannin with biological potential. Biomedicine & Pharmacotherapy = Biomedecine & Pharmacotherapie 142:111988. doi:10.1016/j.biopha.2021.111988.
- Ramya, S., S. Thiruvenkataswamy, K. Kavithaa, S. Preethi, H. Winster, V. Balachander, M. Paulpandi, and A. Narayanasamy. 2021. pH dependent drug release of Silibinin, a polyphenol conjugated with magnetic nanoparticle against the human colon cancer cell. Journal of Cluster Science 32 (2):305–17. doi:10.1007/s10876-020-01789-5.
- Romagnoli, C., F., D’Asta, and M. L. Brandi. 2013. Drug delivery using composite scaffolds in the context of bone tissue engineering. Clinical Cases in Mineral and Bone Metabolism 10 (3):155.
- Sailler, B., and K. W. Glombitza. 1999. Halogenated phlorethols and fucophlorethols from the brown alga Cystophora retroflexa. Natural Toxins 7 (2):57–62. doi:10.1002/(SICI)1522-7189(199903/04)7:2<57::AID-NT42>3.0.CO;2-F.
- Sanadgol, N., and J. Wackerlig. 2020. Developments of smart drug-delivery systems based on magnetic molecularly imprinted polymers for targeted cancer therapy: A short review. Pharmaceutics 12 (9):831. doi:10.3390/pharmaceutics12090831.
- Sancini, G., M. Gregori, E. Salvati, I. Cambianica, F. Re, F. Ornaghi, M. Canovi, C. Fracasso, A. Cagnotto, and M. Colombo. 2013. Functionalization with TAT-peptide enhances blood-brain barrier crossing in vitro of nanoliposomes carrying a curcumin-derivative to bind amyloid-β peptide. Journal of Nanomedicine & Nanotechnology 04 (03):1–8. doi:10.4172/2157-7439.1000171.
- Sathya, R., N. Kanaga, P. Sankar, and S. Jeeva. 2017. Antioxidant properties of phlorotannins from brown seaweed Cystoseira trinodis (Forsskål) C. Agardh. Arabian Journal of Chemistry 10:S2608–S2614. doi:10.1016/j.arabjc.2013.09.039.
- Savaghebi, D., M. Barzegar, and M. R. Mozafari. 2020. Manufacturing of nanoliposomal extract from Sargassum boveanum algae and investigating its release behavior and antioxidant activity. Food Science & Nutrition 8 (1):299–310. doi:10.1002/fsn3.1306.
- Savaghebi, D., M. Ghaderi-Ghahfarokhi, and M. Barzegar. 2021. Encapsulation of Sargassum boveanum algae extract in nano-liposomes: Application in functional mayonnaise production. Food and Bioprocess Technology 14 (7):1311–25. doi:10.1007/s11947-021-02638-7.
- Schaffenburg, W. C., B. N. Lockshin, and C. M. C. DeKlotz. 2021. 3 - Polymorphisms: Why individual drug responses vary. In Comprehensive dermatologic drug therapy, ed. Stephen E. Wolverton, 21–33.e2. 4th ed. Elsevier, Netherlands.
- Schoenwaelder, M. E., and M. N. Clayton. 2000. Physode formation in embryos of Phyllospora comosa and Hormosira banksii (Phaeophyceae). Phycologia 39 (1):1–9. doi:10.2216/i0031-8884-39-1-1.1.
- Shahidi, F., and H. Peng. 2018. Bioaccessibility and bioavailability of phenolic compounds. Journal of Food Bioactives 4:11–68. doi:10.31665/JFB.2018.4162.
- Shannon, E., and N. Abu-Ghannam. 2019. Seaweeds as nutraceuticals for health and nutrition. Phycologia 58 (5):563–77. doi:10.1080/00318884.2019.1640533.
- Shen, Z., H. Ye, M. Kröger, and Y. Li. 2018. Aggregation of polyethylene glycol polymers suppresses receptor-mediated endocytosis of PEGylated liposomes. Nanoscale 10 (9):4545–60. doi:10.1039/c7nr09011k.
- Shim, S. Y., M. Lee, and K. D. Lee. 2016. Achyranthes japonica Nakai water extract suppresses binding of IgE antibody to cell surface FcɛRI. Preventive Nutrition and Food Science 21 (4):323–9. doi:10.3746/pnf.2016.21.4.323.
- Shrestha, S., W. Zhang, and S. Smid. 2021. Phlorotannins: A review on biosynthesis, chemistry and bioactivity. Food Bioscience 39:100832. doi:10.1016/j.fbio.2020.100832.
- Shrivastava, A. K., and A. Pandey. 2013. Inflammation and rheumatoid arthritis. Journal of Physiology and Biochemistry 69 (2):335–47. doi:10.1007/s13105-012-0216-5.
- Singh, I. P., and J. Sidana. 2013. 5 - Phlorotannins. In Functional ingredients from algae for foods and nutraceuticals, ed. H. Domínguez, 181–204. Woodhead Publishing, Cambridge, UK.
- Sinha, A., and S. PK. 2019. Enhanced induction of apoptosis in HaCaT cells by luteolin encapsulated in PEGylated liposomes—role of caspase-3/caspase-14. Applied Biochemistry and Biotechnology 188 (1):147–64. doi:10.1007/s12010-018-2907-z.
- Siriwardhana, N., K.-W. Lee, and Y.-J. Jeon. 2005. Radical scavenging potential of hydrophilic phlorotannins of Hizikia fusiformis. Algae 20 (1):69–75. doi:10.4490/ALGAE.2005.20.1.069.
- Son, M., S. Oh, H. S. Lee, D.-M. Chung, J. T. Jang, Y.-J. Jeon, C. H. Choi, K. Y. Park, K. H. Son, and K. Byun. 2019. Ecklonia cava extract attenuates endothelial cell dysfunction by modulation of inflammation and brown adipocyte function in perivascular fat tissue. Nutrients 11 (11):2795. doi:10.3390/nu11112795.
- Spiller, F., R. O. Formiga, J. F. da Silva Coimbra, J. C. Alves-Filho, T. M. Cunha, and F. Q. Cunha. 2019. Targeting nitric oxide as a key modulator of sepsis, arthritis and pain. Nitric Oxide: Biology and Chemistry 89:32–40. doi:10.1016/j.niox.2019.04.011.
- Subbiah, V., X. Duan, O. T. Agar, F. R. Dunshea, C. J. Barrow, and H. A. Suleria. 2023. Comparative study on the effect of different drying techniques on phenolic compounds in Australian beach-cast brown seaweeds. Algal Research 72:103140. doi:10.1016/j.algal.2023.103140.
- Subbiah, V., F. Ebrahimi, O. T. Agar, F. R. Dunshea, C. J. Barrow, and H. A. Suleria. 2023. Comparative study on the effect of phenolics and their antioxidant potential of freeze-dried Australian beach-cast seaweed species upon different extraction methodologies. Pharmaceuticals 16 (5):773. doi:10.3390/ph16050773.
- Subbiah, V., C. Xie, F. R. Dunshea, C. J. Barrow, and H. A. Suleria. 2023. The quest for phenolic compounds from seaweed: Nutrition, biological activities and applications. Food Reviews International 39 (8):5786–813. doi:10.1080/87559129.2022.2094406.
- Sugiura, Y., M. Usui, H. Katsuzaki, K. Imai, M. Kakinuma, H. Amano, and M. Miyata. 2018. Orally administered phlorotannins from Eisenia arborea suppress chemical mediator release and cyclooxygenase-2 signaling to alleviate mouse ear swelling. Marine Drugs 16 (8):267. doi:10.3390/md16080267.
- Sugiura, Y., M. Usui, H. Katsuzaki, K. Imai, and M. Miyata. 2017. Anti-inflammatory effects of 6, 6′-bieckol and 6, 8′-bieckol from Eisenia arborea on mouse ear swelling. Food Science and Technology Research 23 (3):475–80. doi:10.3136/fstr.23.475.
- Sugiura, Y., M. Usui, H. Katsuzaki, K. Imai, R. Tanaka, T. Matsushita, and M. Miyata. 2021. Dieckol isolated from a brown alga, Eisenia nipponica, suppresses ear swelling from allergic inflammation in mouse. Journal of Food Biochemistry 45 (4):e13659. doi:10.1111/jfbc.13659.
- Sun, X., Y. Luo, L. Huang, B.-Y. Yu, and J. Tian. 2017. A peptide-decorated and curcumin-loaded mesoporous silica nanomedicine for effectively overcoming multidrug resistance in cancer cells. RSC Advances 7 (27):16401–9. doi:10.1039/C7RA01128H.
- Surendhiran, D., H. Cui, and L. Lin. 2019. Encapsulation of phlorotannin in alginate/PEO blended nanofibers to preserve chicken meat from Salmonella contaminations. Food Packaging and Shelf Life 21:100346. doi:10.1016/j.fpsl.2019.100346.
- Tanaka, K., M. Ohno, and D. B. Largo. 2020. An update on the seaweed resources of Japan. Botanica Marina 63 (1):105–17. doi:10.1515/bot-2018-0100.
- Tang, J., W. Wang, and W. Chu. 2020. Antimicrobial and anti-quorum sensing activities of phlorotannins from seaweed (Hizikia fusiforme). Frontiers in Cellular and Infection Microbiology 10:586750. doi:10.3389/fcimb.2020.586750.
- Tong, T., X. Liu, and C. Yu. 2021. Extraction and nano-sized delivery systems for phlorotannins to improve its bioavailability and bioactivity. Marine Drugs 19 (11):625. doi:10.3390/md19110625.
- Turck, D., J.-L. Bresson, B. Burlingame, T. Dean, S. Fairweather-Tait, M. Heinonen, K. I. Hirsch-Ernst, I. Mangelsdorf, H. J. McArdle, A. Naska, et al. 2017. Safety of Ecklonia cava phlorotannins as a novel food pursuant to Regulation (EC) No 258/97. EFSA Journal. European Food Safety Authority 15 (10):e05003. doi:10.2903/j.efsa.2017.5003.
- Um, M. Y., J. Y. Kim, J. K. Han, J. Kim, H. Yang, M. Yoon, J. Kim, S. W. Kang, and S. Cho. 2018. Phlorotannin supplement decreases wake after sleep onset in adults with self-reported sleep disturbance: A randomized, controlled, double-blind clinical and polysomnographic study. Phytotherapy Research: PTR 32 (4):698–704. doi:10.1002/ptr.6019.
- Ummat, V., B. K. Tiwari, A. K. Jaiswal, K. Condon, M. Garcia-Vaquero, J. O’Doherty, C. O’Donnell, and G. Rajauria. 2020. Optimisation of ultrasound frequency, extraction time and solvent for the recovery of polyphenols, phlorotannins and associated antioxidant activity from brown seaweeds. Marine Drugs 18 (5):250. doi:10.3390/md18050250.
- Verhoef, J. J., and T. J. Anchordoquy. 2013. Questioning the use of PEGylation for drug delivery. Drug Delivery and Translational Research 3 (6):499–503. doi:10.1007/s13346-013-0176-5.
- Victor, S. P., and C. P. Sharma. 2015. Chapter 9 - Anti-inflammatory drug delivery systems using marine products. In Functional Marine Biomaterials, ed. S.-K. Kim, 137–47. Woodhead Publishing, Cambridge, UK.
- Vijay, N. K. G., and C. Vellapandian. 2023. Ameliorative effects of phlorotannin-rich fraction of Sargassum tenerrimum in high-fat diet and low dose streptozotocin-induced metabolic changes and oxidative stress in diabetic rats. Journal of Herbmed Pharmacology 12 (3):367–79.
- Vissers, A. M., A. Caligiani, S. Sforza, J. P. Vincken, and H. Gruppen. 2017. Phlorotannin composition of Laminaria digitata. Phytochemical Analysis 28 (6):487–95. doi:10.1002/pca.2697.
- Wang, T., R. Jonsdottir, and G. Ólafsdóttir. 2009. Total phenolic compounds, radical scavenging and metal chelation of extracts from Icelandic seaweeds. Food Chemistry 116 (1):240–8. doi:10.1016/j.foodchem.2009.02.041.
- Wei, P., E. J. Cornel, and J. Du. 2021. Ultrasound-responsive polymer-based drug delivery systems. Drug Delivery and Translational Research 11 (4):1323–39. doi:10.1007/s13346-021-00963-0.
- Wekre, M. E., S. Hellesen Brunvoll, and M. Jordheim. 2022. Advancing quantification methods for polyphenols in brown seaweeds—Applying a selective qNMR method compared with the TPC assay. Phytochemical Analysis 33 (7):1099–110. doi:10.1002/pca.3162.
- Xie, C., Z. J. Lee, S. Ye, C. J. Barrow, F. R. Dunshea, and H. A. Suleria. 2023. A review on seaweeds and seaweed-derived polysaccharides: Nutrition, chemistry, bioactivities, and applications. Food Reviews International 1–36. doi:10.1080/87559129.2023.2212055.
- Yadav, D., and H. K. Dewangan. 2021. PEGYLATION: An important approach for novel drug delivery system. Journal of Biomaterials Science. Polymer Edition 32 (2):266–80. doi:10.1080/09205063.2020.1825304.
- Yang, H., M. Yoon, J. Kim, and S. Cho. 2014. Acute oral toxicity of phlorotannins in beagle dogs. Korean Journal of Fisheries and Aquatic Sciences 47 (4):356–62. doi:10.5657/KFAS.2014.0356.
- Yao, D., M. Dong, C. Dai, and S. Wu. 2019. Inflammation and inflammatory cytokine contribute to the initiation and development of ulcerative colitis and its associated cancer. Inflammatory Bowel Diseases 25 (10):1595–602. doi:10.1093/ibd/izz149.
- Yeo, M., W.-K. Jung, and G. Kim. 2012. Fabrication, characterisation and biological activity of phlorotannin-conjugated PCL/β-TCP composite scaffolds for bone tissue regeneration. Journal of Materials Chemistry 22 (8):3568–77. doi:10.1039/c2jm14725d.
- Yingying, M., L. Xiu-Xia, C. Luyun, and L. Jianrong. 2022. pH-Sensitive ε-polylysine/polyaspartic acid/zein nanofiber membranes for the targeted release of polyphenols. Food & Function 13 (12):6792–801. doi:10.1039/d1fo03051e.
- Yoshioka, H., M. Ishida, K. Nishi, H. Oda, H. Toyohara, and T. Sugahara. 2014. Studies on anti-allergic activity of Sargassum horneri extract. Journal of Functional Foods 10:154–60. doi:10.1016/j.jff.2014.06.002.
- Zhang, J., A. Hassane Hamadou, C. Chen, and B. Xu. 2023. Encapsulation of phenolic compounds within food-grade carriers and delivery systems by pH-driven method: A systematic review. Critical Reviews in Food Science and Nutrition 63 (19):4153–74. doi:10.1080/10408398.2021.1998761.
- Zhang, J., L. Zhang, C. Lai, Y. Liang, L. Gao, K. Kaliaperumal, and Y. Jiang. 2022. Nutraceutical potential of navel orange peel in diabetes management: The chemical profile, antioxidant, α-glucosidase inhibitory and antiglycation effects of its flavonoids. Food Bioscience 49:101943. doi:10.1016/j.fbio.2022.101943.
- Zhang, S., A. Taehwan Kim, X. Liu, L. Yan, and S. Moo Kim. 2020. Antioxidant and antidiabetic activities of vanadium-binding protein and trifuhalol A. Journal of Food Biochemistry 44 (12):e13540. doi:10.1111/jfbc.13540.
- Zhao, W., V. Subbiah, C. Xie, Z. Yang, L. Shi, C. Barrow, F. Dunshea, and H. A. Suleria. 2023. Bioaccessibility and bioavailability of phenolic compounds in seaweed. Food Reviews International 39 (8):5729–60. doi:10.1080/87559129.2022.2094404.
- Znad, H., M. R. Awual, and S. Martini. 2022. The utilization of algae and seaweed biomass for bioremediation of heavy metal-contaminated wastewater. Molecules 27 (4):1275. doi:10.3390/molecules27041275.
- Zou, L-q., W. Liu, W-l Liu, R-h Liang, T. Li, C-m Liu, Y-l Cao, J. Niu, and Z. Liu. 2014. Characterization and bioavailability of tea polyphenol nanoliposome prepared by combining an ethanol injection method with dynamic high-pressure microfluidization. Journal of Agricultural and Food Chemistry 62 (4):934–41. doi:10.1021/jf402886s.