Abstract
The enterolignans, enterolactone and enterodiol, the main metabolites produced from plant lignans by the gut microbiota, have enhanced bioavailability and activity compared to their precursors, with beneficial effects on metabolic and cardiovascular health. Although extensively studied, the biosynthesis, cardiometabolic effects, and other therapeutic implications of mammalian lignans are still incompletely understood. The aim of this review is to provide a comprehensive overview of these phytoestrogen metabolites based on up-to-date information reported in studies from a wide range of disciplines. Established and novel synthetic strategies are described, as are the various lignan precursors, their dietary sources, and a proposed metabolic pathway for their conversion to enterolignans. The methodologies used for enterolignan analysis and the available data on pharmacokinetics and bioavailability are summarized and their cardiometabolic bioactivity is explored in detail. The special focus given to research on the health benefits of microbial-derived lignan metabolites underscores the critical role of lignan-rich diets in promoting cardiovascular health.
Background
Lignans are phenolic secondary metabolites found in a wide range of plant-derived foods, including seeds, whole grains, beans, fruits, and vegetables, virgin olive oil, although they represent only a small fraction of the phenolic compounds consumed in the human diet (Adriouch et al. Citation2017; Landete Citation2012). Upon digestion by the gut microbiota, lignans are transformed into more active and bioavailable metabolites known as enterolignans. Inter- and intra-individual variations in enterolignan production have been observed and are attributed to individual dietary habits, food transit time, and gut microbial composition and abundance. Three different enterolignan-producing phenotypes have been defined (low, middle, and high), based on the metabolic capacity of the gut microbiota, the differences being influenced by age, sex, and genetics (Possemiers et al. Citation2007; Sawane et al. Citation2022; Senizza et al. Citation2020). The most extensively studied mammalian enterolignans are the primary end metabolites enterolactone (ENL) and enterodiol (END) (Baldi et al. Citation2023; Senizza et al. Citation2020). Dietary lignans in general, including ENL and END, have been linked to cardiovascular health benefits, and are therefore of great interest in the field of preventive nutrition (Peterson et al. Citation2010; Reger et al. Citation2016). Notably, non-communicable diseases, above all cardiovascular disease, account for more than half of the global burden of disease (Abbafati et al. Citation2020). Research suggests that dietary lignans may help to reduce cardiovascular risk by promoting endothelial function, improving the lipid profile, and reducing inflammation (Frankenfeld Citation2017; Peterson et al. Citation2010; Reger et al. Citation2016; Vanharanta et al. Citation2003). The effects of dietary lignan exposure depend on factors such as conversion rates into enterolignans and the metabolite profile and yield (Senizza et al. Citation2020).
Although some publications deal with mammalian enterolignans (Plaha et al. Citation2022), a comprehensive review encompassing their synthesis and cardiometabolic health effects has been lacking to date. Therefore, to fill this gap, the present work aims to summarize and discuss the latest developments in mammalian enterolignan research, focusing on various key aspects: (1) new synthetic strategies are outlined, and established routes summarized; (2) lignan precursors and their food sources are comprehensively described; (3) a tentative metabolic pathway of mammalian lignans is presented; (4) an updated summary of the current analytical approaches used in enterolignan research is provided; (5) the pharmacokinetics and bioavailability of ENL and END are addressed; and (6) the cardiometabolic bioactivity of enterolignans are analyzed in detail, outcome by outcome. Special emphasis has been given to research focused on the potential health benefits of microbiota-derived lignan metabolite exposure, which provides a more accurate approximation of the impact of consuming lignan-containing foods.
Synthesis of mammalian enterolignans
The structures of END and ENL () have been elucidated from mass spectrometric, infrared, ultraviolet, and nuclear magnetic resonance (NMR) data and validated by chemical synthesis. The simple spectra afforded by the structurally symmetrical END are easily interpreted (Setchell, Bull, and Adlercreutz Citation1980), unlike the asymmetrical ENL, for which the 1H-NMR spectrum in the high-field region resembles that of matairesinol and its demethylether (Nishibe et al. Citation1980). It was not until 2002 that full 1H- and 13C-NMR data were first reported for both compounds (Wang Citation2002). ENL has been found in urine as a racemic mixture resulting from its isomerization by the exchange of the hydrogen vicinal to the lactone carbonyl group. The optical rotation of synthetic (–)-ENL is [α]19D = −40.3° (c = 0.55, CHCl3) and (–)- END is [α]23D = −13.2° (c = 1 in EtOH) (van Oeveren, Jansen, and Feringa Citation1994).
As END and ENL are produced by animals in small quantities and their extraction is unviable, their total synthesis has been critical, initially for structural validation and then to produce standards for research on metabolism, pharmacokinetics, and bioactivity. Since the 2018 review of ENL total synthesis (Waulters et al. Citation2018), three novel and innovative synthetic strategies have been reported. A summary of the main synthetic routes reported before 2018 are included in the supporting information (Tables S1 and S2, Figures S1–S4).
Latest enantiopure syntheses of enterolactone
Since 2018, three new syntheses providing enantiomerically pure ENL, taking advantage of metal- or organocatalytic processes as the key step, have been reported.
Zhang’s synthesis (2021)
Zhang and coworkers (Zhang et al. Citation2021) developed a powerful procedure for the synthesis of enantiomerically enriched α-borylzinc reagents by nickel-catalyzed carbozincation of vinylboronic esters. Its synthetic value was demonstrated by the successful synthesis of (−)-ENL in six steps and overall yield of 20% ().
Figure 2. A nickel-catalyzed carbozincation approach to enterolignan synthesis (THF: tetrahydrofuran; DMSO: dimethyl sulfoxide; DMF: dimethylformamide; DCM: dichloromethane; NMO: N-methylmorpholine N-oxide; PCC: pyridinium chlorochromate; LDA: lithium diisopropylamide; HMPA: hexamethylphosphoramide; rt: room temperature; er: enantiomeric ratio.
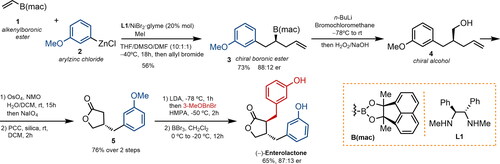
From vinylboronic ester 1, the synthesis began with the preparation of an enantioenriched organozinc intermediate, which was trapped with allyl bromide to obtain boronic ester 3 in moderate yield and enantioselectivity. Boronic ester 3 was then converted to chiral alcohol 4 through a Matteson homologation, and this intermediate underwent oxidative lactonization to provide lactone 5 in 76% yield. The final diastereoselective alkylation and subsequent demethylation with BBr3 afforded (−)-ENL.
Jiang’s synthesis (2022)
Jiang et al. reported the synthesis of both (−)-ENL and its enantiomer by enantioselective oxidative lactonization of meso-diols () (Jiang et al. Citation2022). The required meso-aldehyde 7 was prepared in three steps from cis-3-cyclobutene-1,2-dimethanol (6): protection, dihydroxylation, and oxidative cleavage with silica gel-supported NaIO4. The enantioselective intramolecular Tishchenko reaction using a chiral Ir complex afforded lactone 8 in 78% yield and 91% ee, which was then obtained in optically pure form by recrystallization. The acetal moiety in lactone 8 was cleaved under acidic conditions and the intermediate unexpectedly underwent a beta-epimerization process to afford the corresponding trans-diol 9a as the major product in 96% yield. A subsequent Appel reaction followed by double Negishi coupling of the resulting diiodide provided (−)-ENL. This synthetic route was completed in six steps and 29% overall yield.
Figure 3. Asymmetric synthesis of both enantiomers of enterolactone via selective epimerization at the α- or β-position. NMO: N-methylmorpholine N-oxide; DBU: 1,8-diazabicyclo(5.4.0)undec-7-ene; DCE: 1,2-dichloroethane; MPM: methoxybenzyl, PPTS: pyridinium p-toluenesulfonate, ee: enantiomeric excess)
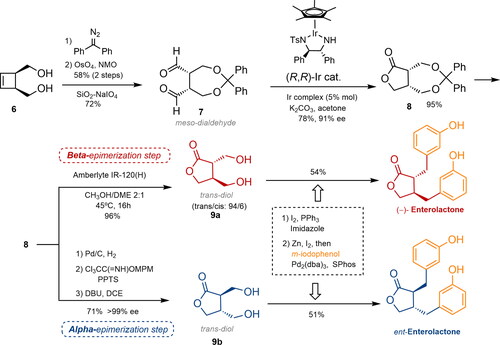
The synthesis of ent-ENL via a controlled alpha-epimerization step was also completed by hydrogenolysis of lactone 8, protection, and subsequent treatment under basic conditions with DBU to furnish trans-diol 9b as the unique product. The ent-ENL was produced in seven steps with an overall yield of 20%.
Yetra’s synthesis (2022)
Recently, a catalytic photochemical enantioselective alpha-alkylation of aldehydes with amino acid-derived pyridinium, using the McMillan amine catalyst A, led to the enantioselective total synthesis of ENL and END () (Yetra, Schmitt, and Tambar Citation2023). Aldehyde 10 and the pyridinium salt of racemic m-tyrosine reacted in a stereoconvergent process to form an alpha-alkylated product, which was subjected to reductive conditions to obtain lactones 11 in 46% yield and a low diastereomeric ratio but high enantiomeric excess. Nevertheless, after treatment with LiHMDS and TMSCl, the natural product was obtained with a 12:1 diastereomeric ratio and 97% ee. Finally, reduction with LiAlH4 afforded the natural precursor (−)-ENL in 70% yield as a single diastereomer.
Figure 4. Yetra’s synthetic approach to enterolignans via enantioselective alpha-alkylation of carbonylic compounds with pyridinium salts. DMA, TMSCl: trimethylsilyl chloride; LiHMDS: lithium bis(trimethylsilyl)amide; THF: tetrahydrofuran; DCM: dichloromethane; dr: diastereomeric ratio; ee: enantiomeric excess.
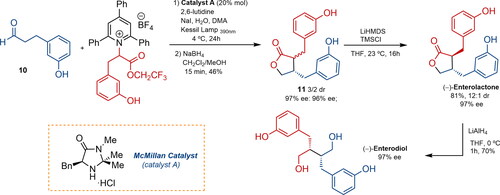
Enterolignan precursors and food sources
END and ENL are phenolic metabolites produced by the intestinal microbiota from dietary lignans. The first plant lignans to be identified in foods, and thus the focus of early research in this field, were secoisolariciresinol (SECO) and matairesinol (MAT) (Horn-Ross et al. Citation2000; Mazur et al. Citation2000; Valsta et al. Citation2003). Subsequent lignans analyzed in food include pinoresinol (PIN) and lariciresinol (LAR) (Milder et al. Citation2005; Thompson et al. Citation2006), and more recently, medioresinol (MED), and syringaresinol (SYR) (Peñalvo et al. Citation2008). The lignan 7′-hydroxyMAT, which is predominant in Norway spruce knots (Holmbom et al. Citation2003), has been reported in cereals such as wheat and rye (Peñalvo et al. Citation2005), as well as in sesame seeds in significant amounts (Smeds et al. Citation2007). Sesamin (SES) has been quantified in sesame seeds (Peñalvo et al. Citation2005), but is generally not included in routine analytical studies due to its scarce detection in other foods.
Lignans are present in a wide variety of plant foods, including seeds (flax, sunflower, sesame), whole grains (rye, oats, barley), bran (wheat, oat, rye), beans, fruits (particularly berries), cruciferous vegetables (broccoli, cabbage), and beverages (tea, coffee), as well as olive oil, grapes, and wine. Although high quantities of lignans are found in flax and sesame seeds, daily intake of seeds is low in some populations, where the main dietary sources of lignans are whole grain cereals, vegetables, beverages and vegetable oils. The predominant lignan in flaxseeds is SECO diglucoside (Landete Citation2012). Lignans are absent in most seed oils, and their content in whole or ground seeds varies according to the region, environment, crop maturity, and storage time. Despite the numerous food sources of lignans, they represent less than 10% of total dietary phenolic intake (Adriouch et al. Citation2017; Tresserra-Rimbau et al. Citation2014). With variations across geographical regions and populations, the mean intake of lignans ranges from 0.2 to 6.4 mg/day (Rizzolo-Brime et al. Citation2022; Tresserra-Rimbau et al. Citation2014).
Detailed tables showing the contents of SECO, MAT, PIN, LAR, MED, SYR and SES in food are included in the Supporting Information using the classification of the Phenol-Explorer (version 3.6) database. However, as some mistakes have been detected in the database, the contents in the tables (mg per 100 g of fresh weight (FW)) have been retrieved from the original articles. Here, we discuss the primary food sources of each specific lignan.
Secoisolariciresinol
SECO, the most extensively studied lignan, is primarily found in seeds, nuts, cereals, vegetables, and certain fruits, as listed in Table S3. Flaxseed is the richest source of SECO, with concentrations ranging from 690.75 to 81.70 mg/100 g FW, according to several studies (Mazur et al. Citation1996; Milder et al. Citation2005; Obermeyer et al. Citation1995; Peñalvo et al. Citation2005; Smeds et al. Citation2007; Smeds, Eklund, and Willför Citation2012; Thompson et al. Citation2006). Bread made with whole-grain flaxseed, multi-grains, or rye is also a good source of SECO (Mazur et al. Citation1996; Milder et al. Citation2005; Thompson et al. Citation2006). Other notable sources are berries (blackberries, lingonberries, and cranberries) (Smeds, Eklund, and Willför Citation2012), certain nuts (Brazil nuts, cashews, and chestnuts) (Kuhnle et al. Citation2008, Citation2009; Smeds et al. Citation2007), seeds (sunflower, pumpkin, and sesame seeds) (Kuhnle et al. Citation2008; W. Mazur et al. Citation1996; Smeds, Eklund, and Willför Citation2012), and dried fruits (dates, apricots, and prunes) (Kuhnle et al. Citation2009). In addition, vegetables such as asparagus, soybeans, mung beans, and sweet potatoes contain significant amounts of this lignan.
Matairesinol
MAT is found in foods in lower amounts compared to SECO and is primarily present in seeds and cereals, with minor quantities in other foods like vegetables and fruits (Table S4). The highest concentrations of this lignan have been reported in whole grain flaxseed (Mazur et al. Citation1996; Peñalvo et al. Citation2005) and sesame seeds (Smeds et al. Citation2007; Smeds, Eklund, and Willför Citation2012) (5.20 mg/100 g FW and 1.16 mg/100 g FW, respectively), followed by cereals such as rye, oat, and wheat (0.72–0.41 mg/100 g FW) (Smeds et al. Citation2007). Among vegetables, only sweet potatoes are notable sources of MAT (Kuhnle et al. Citation2007, Citation2009). Generally, fruits have low levels of this lignan, with raisins and pineapple containing the most (Kuhnle et al. Citation2009).
Pinoresinol
Sesame seeds are the most significant source of PIN, with concentrations ranging from 47.13 to 6.81 mg/100g FW (Milder et al. Citation2005; Smeds et al. Citation2007; Smeds, Eklund, and Willför Citation2012; Thompson et al. Citation2006), followed by flaxseed (3.32–2.46 mg/100 g FW) (Milder et al. Citation2005; Peñalvo et al. Citation2005), with lower amounts found in almonds and sunflower seeds (Table S5). Vegetables, cereals, and fruits are also notable sources of PIN. Among vegetables, the highest content is found in curly kale and common cabbage (Milder et al. Citation2005), and among cereals, in rye, oat, and spelt wheat (Smeds et al. Citation2007), as well as bread made with whole grain flaxseed, multi-grains, or rye (Milder et al. Citation2005). Berries such as strawberries, blackberries, and lingonberries have notable amounts of PIN (1.40–1.07 mg/100 g FW) (Smeds, Eklund, and Willför Citation2012). Other fruits containing this lignan include apricots, lemons, peaches, and nectarines. In addition, extra virgin olive oil has a significant content of PIN, ranging from 0.24 to 0.14 mg/100 g FW) (Milder et al. Citation2005; Thompson et al. Citation2006).
Lariciresinol
LAR, similar to PIN, is primarily found in seeds, nuts, and cereals, followed by certain vegetables and fruits, as listed in Table S6. Sesame seeds are the most important source of LAR, with contents ranging from 15.94 to 9.47 mg/100 g FW (Milder et al. Citation2005; Smeds et al. Citation2007; Smeds, Eklund, and Willför Citation2012). Other seeds and nuts rich in LAR are flaxseeds, with contents of 3.67 − 2.81 mg/100 g FW (Milder et al. Citation2005; Peñalvo et al. Citation2005; Thompson et al. Citation2006), sunflower seeds and cashew nuts (Milder et al. Citation2005). Notable amounts are also found in cereals such as rye, oats, wheat, and spelt wheat (Peñalvo et al. Citation2005; Smeds et al. Citation2007; Smeds, Eklund, and Willför Citation2012).
Additionally, a range of fruits and vegetables have significant amounts of PIN, particularly cloudberries (5.00 mg/100 g FW)(Smeds, Eklund, and Willför Citation2012), broccoli, curly kale, Brussels sprouts, and cabbage (Milder et al. Citation2005; Thompson et al. Citation2006).
Medioresinol
Primarily found in seeds and fruits, MED is usually much less abundant in food than other lignans (see Table S7). The food source richest in MED is sesame seeds, with contents ranging from 9.47 to 4.15 mg/100g (FW) (Smeds et al. Citation2007; Smeds, Eklund, and Willför Citation2012). Berries, including cloudberries, lingonberries, strawberries, and blackberries, are another excellent source, with contents varying from 3.75 to 0.47 mg per 100 g (FW) (Smeds, Eklund, and Willför Citation2012), as are cereals such as rye, spelt wheat, and wheat (Peñalvo et al. Citation2005; Smeds et al. Citation2007; Smeds, Eklund, and Willför Citation2012). Vegetables, on the other hand, are generally low in MED, with only avocados containing >0.2 mg/100 g (FW) of MED (Peñalvo et al. Citation2005, Citation2008).
Syringaresinol
Hemp seeds are the richest source of SYR but their intake in the general population has not been estimated (Smeds, Eklund, and Willför Citation2012), whereas other seeds and nuts do not contain significant amounts of this lignan (Table S8). Among cereals, high concentrations of SYR are found in rye, wheat, and spelt wheat, as well as rye bread and some flours (Peñalvo et al. Citation2005; Smeds et al. Citation2007; Smeds, Eklund, and Willför Citation2012). Berries such as cloudberries, cranberries, and blackberries are again notable for their high levels of SYR (Smeds, Eklund, and Willför Citation2012). Similar to MED, avocados contain 0.44 mg/100 g FW of SYR (Peñalvo et al. Citation2005, Citation2008), whereas the contribution of other vegetables to SYR intake is insignificant.
Sesamin
Sesame seeds, which have been utilized as an oilseed since antiquity, are an exceptional source of SES (for contents, see Table S9). In fact, the notable resistance of sesame oil to oxidative deterioration in comparison with other vegetable oils is attributed to its lignan content (Hemalatha and Ghafoorunissa Citation2007). However, the amounts of SES and sesamolin in sesame seeds differ greatly. Most of the research published so far has focused on determining the SES profile according to Sesamum species (Kamal-Eldin and Appelqvist Citation1994), the origin of the sesame cultivars (India (Hemalatha and Ghafoorunissa Citation2004) or Taiwan (Wu Citation2007)), or the impact of processing (Moazzami and Kamal-Eldin Citation2006; Yoshida et al. Citation1995; Yoshida and Takagi Citation1997) and storage conditions (Shahidi et al. Citation1997). However, the richest source of SES is flour made from whole sesame seeds, which contains 538.68 mg of SES per 100 g (FW) (Liu, Saarinen, and Thompson Citation2006).
Metabolism of lignans to enterolignans
Lignans are dietary diphenolic chemicals. The majority of lignans found in plants are glycosides, and after consumption, the sugar moieties are hydrolyzed to produce aglycones. Bacterial β-glucosidases and β-glucosidases in the mucosal brush border of the human gut are capable of hydrolyzing substances. The intestinal bacteria can absorb the aglycones or further convert them to enterolignans (Lampe, Atkinson, and Hullar Citation2006).
The mammalian enterolignans END and ENL are formed from plant lignan precursors by the activity of the gut microbiota in the proximal colon. A tentative metabolic pathway of enterolignans and their absorption in the gut are depicted in . Although enterolignans can passively diffuse across the enterocyte membrane, a portion of these molecules undergo phase II metabolism by UDP-glucuronosyltransferases (UGT) and sulfotransferases (ST) in the enterocyte, resulting in the formation of polar and soluble conjugated metabolites (glucuronide and sulfate). These metabolites require active transport to cross the basolateral intestinal membrane for systemic distribution via the portal vein. In the hepatocyte, enterolignans can undergo phase II metabolism by UGT and ST enzymes prior to active bile transport, resulting in enterohepatic recirculation. Once in the systemic circulation, enterolignans can reach various tissues and promote biological responses. Finally, enterolignans can be eliminated in the feces (most predominant) or urine (De Silva and Alcorn Citation2019).
Figure 5. Enterolignan absorption and metabolism. Dietary lignans are metabolized by the gut microbiota to enterolignans (END and ENL), which can passively diffuse across the enterocyte membrane. However, a portion of these molecules undergo phase II metabolism by UDP-glucuronosyltransferases (UGT) and sulfotransferases (ST) in the enterocyte, resulting in the formation of polar and soluble conjugated metabolites (glucuronide and sulfate). These metabolites require active transport to cross the basolateral intestinal membrane for systemic distribution via the portal vein. In the hepatocyte, enterolignans can undergo phase II metabolism by UGT and ST enzymes prior to active bile transport, resulting in enterohepatic recirculation. Once in the systemic circulation, enterolignans can reach various tissues and promote biological responses. Finally, enterolignans can be eliminated in the feces (predominantly) or urine (De Silva and Alcorn Citation2019).
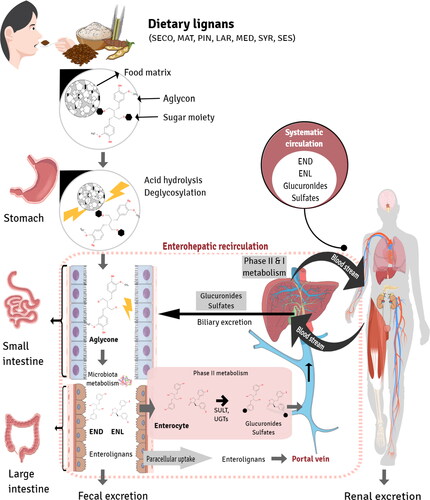
The transformation of plant lignans such as SECO diglucoside, PIN diglucoside, arctiin, and MAT to ENL and END by intestinal bacteria involves a series of reactions such as deglucosylation, ring cleavage, demethylation, dehydroxylation, and oxidation. PIN diglucoside undergoes two sequential deglycosylations to produce the corresponding monoglycoside and aglycone. PIN is converted to LAR by reductive cleavage of its furofuran bicyclic ring and further metabolized by elimination of the furan ring to SECO, which alternatively can be generated by deglycosylation of the non-absorbed dietary SECO diglucoside (Quartieri et al. Citation2016; Setchell et al. Citation2014; Xie et al. Citation2003). The oxidation of SECO generates MAT (Setchell et al. Citation2014). Finally, the demethylation and dehydroxylation of both SECO and MAT may be carried out by intestinal microbiota, leading to END and ENL, respectively (Niemeyer et al. Citation2003). Alternatively, ENL can be generated by oxidation of END (Adlercreutz Citation2007).
Although END and ENL were detected when SES was fermented with human fecal inoculum, the amount was relatively small compared with SECO diglucoside. SES can be classified as a furofuran lignan with methylenedioxyphenyls, whose oxidation results in the formation of the corresponding catechol (Liu, Saarinen, and Thompson Citation2006). On the other hand, the lignan phillyrin is metabolized by intestinal bacteria to ENL; the estimated metabolic pathway is also presented in (Nishibe et al. Citation2021).
In addition, two extra methoxy groups at meta positions in both phenolic rings distinguish the structure of SYR from that of PIN, its substitution pattern being especially complicated. Several SYR metabolites have been produced in in vitro studies. The majority of the tentatively identified metabolites have an oxydiarylbutane structure, which suggests that SYR breaks down similarly to PIN and involves the equivalent LAR and SECO intermediates (Heinonen et al. Citation2001). Since the first identification of two plant enterolignan precursors, SECO and MAT (Axelson et al. Citation1982), evidence has emerged indicating that 7-hydroxyMAT and arctigenin, both present in sesame seeds (7.20 and 0.01 mg/100 g FW, respectively) (Smeds et al. Citation2007), are converted to enterolignans by the colon microbiota (Nose et al. Citation1992; Saarinen et al. Citation2000). 7-hydroxyMAT has been transformed to ENL and, in small amounts, to END both in vivo (rats) and in vitro (Saarinen et al. Citation2000). According to Smeds et al. (Citation2005), the reported in vitro oxidation of 7-hydroxyMAT to 7-oxoMAT suggests that lignans possessing a hydroxyl group at C-7 are likely to also be oxidated in vivo, a hypothesis supported by the presence of 7-oxoMAT in human plasma. On the other hand, the metabolic processes undergone by the lignan artiin include deglucosylation as well as several demethylations and dehydroxylations catalyzed by intestinal bacteria (Wang et al. Citation2013). The two adjacent methoxy groups of arctigenin are rather resistant to demethylation and the methoxy group adjacent to a hydroxy group is easily demethylated in the initial steps of the metabolic pathway (Nose et al. Citation1992). Although isoLAR has been detected in in vitro studies, it is thought to be generated from LAR when samples are treated with acid, and its intestinal conversion to enterolignans appears not to take place. A thorough study of the gut bacteria responsible for the synthesis of ENL from SECO diglucoside (SDG) was published in 2006 (Clavel et al. Citation2006).
Extraction and analysis of the mammalian phytoestrogens enterodiol and enterolactone
To understand the biological activity of END and ENL and the factors that influence their production, their structural analysis is necessary. A range of approaches have been employed, using different extraction methods such as mass spectrometry (MS) (Fotsis et al. Citation1982) or immunoassays (Shinkaruk et al. Citation2014). The compounds have been analyzed alone, or together with other phytoestrogens (Gaya et al. Citation2016) or lignans (Nørskov et al. Citation2015) and more recently with multiple other microbial metabolites (González-Domínguez, Urpi-Sarda, et al. Citation2020; Laveriano-Santos, Marhuenda-Muñoz et al. Citation2022). Due to their biological importance, an official method has been developed for the analysis of urinary END and ENL along with other phytoestrogens using high-performance liquid chromatography (HPLC) coupled to mass spectrometry in tandem (MS/MS) (CDC-Division of Laboratory Sciences Citation2004). Before analysis, some sample pretreatment is usually necessary. The first step is the deconjugation of ENL and END from sulfate and glucuronide groups, followed by a separation of phenolic compounds. Finally, after the samples are filtered, the phenolic compounds are derivatized prior to the analysis. For each pretreatment step, different methodological approaches have been proposed, including a range of solvents, reactants, and materials, depending on the analyzer and the other analytes. All these steps are optional, depending on the type of analysis, and some (or even all) can be avoided. The different procedures are discussed below, together with their possible applications, and all the methods are summarized in .
Figure 7. Flow chart depicting the different extraction and analytical methods used to study enterolignans. SPE: solid phase extraction; LLE: liquid-liquid extraction; GC: gas chromatography; MS: mass spectrometry; LC: liquid chromatography; CAD: colourimetric array detector; DAD: diode array detector.
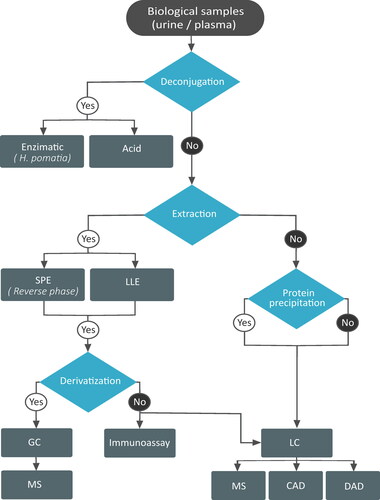
The deconjugation step is most frequently performed enzymatically, using the digestive juice from the edible snail (Helix pomatia), which contains both β-glucosidase and sulphatase (Nørskov et al. Citation2015; Shinkaruk et al. Citation2014; Smeds et al. Citation2006). Alternatively, deconjugation can be carried out with chlorohydric acid (S. H. Lee et al. Citation2004). Some studies have eliminated the deconjugation step, analyzing the sulfate and glucuronide forms and aglycones separately (Laveriano-Santos, Marhuenda-Muñoz et al. Citation2022; Nørskov et al. Citation2016) to elucidate which chemical forms, that have different biological activity, are really present in the biological samples. A study using feces reached the conclusion that the sulfate and glucuronide content was too low in this kind of sample (less than 10%) to make deconjugation worthwhile (Adlercreutz et al. Citation1995).
For the extraction and purification, two main approaches have been proposed: a solid phase extraction (SPE) (Wyns et al. Citation2010) and liquid-liquid extraction (LLE) (Prasain et al. Citation2010), using either ethyl acetate or diethyl ether (Gaya et al. Citation2016). It is not clear which method is more effective for enterolignan extraction; for example, when LLE with diethyl ether was used, the recoveries in serum were 87% (END) and 81% (ENL) compared with 88% (END) and 74% (ENL) obtained with an SPE C18 (Laveriano-Santos, Marhuenda-Muñoz et al. Citation2022). Higher extractions have been achieved with other more complex methods, such as supported liquid extraction using a mixture of ethyl acetate and diethyl ether (30:70), which has resulted in recoveries of 96% for both END and ENL. SPE arguably offers more advantages than other methods, including simplicity, speed, and low reactant consumption; it also allows several samples to be processed at the same time (OASIS HLB 96-well plate) and facilitates the purification step. If deconjugation is included, the separation step is necessary to remove the enzymes and buffer salts from the samples. Otherwise, the sample is usually cleaned by the SPE (Laveriano-Santos, Marhuenda-Muñoz et al. Citation2022). Recently, González-Domínguez et al. (Citation2020) (González-Domínguez, Jáuregui, et al. Citation2020; González-Domínguez, Urpi-Sarda, et al. Citation2020) proposed using SPE for urine samples and only protein precipitation for plasma.
Finally, when using gas chromatography (GC), a derivatization step is needed to volatilize the analytes, which is done by adding trimethylsilyl (TMS) to the ENL and END molecules. Two different methods have been proposed: an offline derivatization reacting END and ENL with TMS ether (Fotsis et al. Citation1982) or N-methyl-N-trifluorotrimethylsilyl acetamide (Lee et al. Citation2004), or an on-line procedure using a solid-phase microextraction and an on-fiber derivatization with N,O-bis(trimethylsilyl)trifluoroacetamide) (Aresta, Cotugno, and Zambonin Citation2019).
After the sample pretreatment, the enterolignans are analyzed by different methods: immunoassays (Adlercreutz et al. Citation1998), GC-MS (Fotsis et al. Citation1982), high-performance liquid chromatography (HPLC)-MS (Franke et al. Citation2002), HPLC-fluorescence (FL) (Mukker et al. Citation2010), HPLC-photodiode array detection (DAD) (Gaya et al. Citation2016) and HPLC-colorimetric array detection (CAD) (Gamache and Acworth Citation1998). Ultra-high performance liquid chromatography (UHPLC)-MS has also been employed (Socas-Rodríguez et al. Citation2017).
The first method developed and validated was GC-MS (Fotsis et al. Citation1982), and received extensive use, giving good limits of detection (LOD) and quantification (LOQ): 0.3 µg/L and 1 µg/L, respectively, for both ENL and END (Choi et al. Citation2002). In healthy adults, concentrations measured in urine were 1.2–25.9 µg/L (ENL) and 1.4–17 µg/L (END), and in plasma 5.6–11.9 µg/L (ENL) and 3.7–19.4 µg/L (END) (Choi et al. Citation2002). However, GC requires a derivatization step and considerable processing time for each sample: 22 min of chromatography plus the refrigeration time (Choi et al. Citation2002). Recently, the derivatization step has been avoided by using on-fiber derivatization, although the time required for chromatography is still high at 50 min (Aresta, Cotugno, and Zambonin Citation2019).
In contrast, in HPLC methods, chromatography time is reduced to as little as two minutes (Prasain et al. Citation2010), whereas the LODs and LOQs differ according to the type of detector. When using CAD, the LOD for both END and ENL in plasma and urine is 1.32 µg/L (Gamache and Acworth Citation1998) and 0.28 µg/L for ENL in blood (Melby et al. Citation2005); when using DAD, the LOQs are 0.53 µg/L (END) and 0.84 µg/L (ENL) in fecal samples (Gaya et al. Citation2016). Regarding MS, limit values also vary according to whether single quadrupole MS, MS/MS or high-resolution MS (HRMS) are used. For example, the LOQs reported in urine are 0.133 µg/L (END) and 0.003 µg/L (ENL) for single quadrupole MS (Palma-Duran et al. Citation2020), 0.037 µg/L (END) and 0.066 µg/L (ENL) for MS/MS (González-Domínguez, Urpi-Sarda, et al. Citation2020), and 0.18 µg/L (END) and 0.06 µg/L (ENL) for HRMS (Laveriano-Santos, Marhuenda-Muñoz et al. Citation2022). Besides better LODs, MS also offers improved selectivity compared to the other detectors, giving more confidence in the results (Wilson et al. Citation2014).
Finally, the immunoassays used can be specific, designed for only one molecule (Adlercreutz et al. Citation1998), or nonspecific. A nonspecific assay has been used to analyze estrogenic activity, the test reacting to more than one molecule, but only those with estrogenic effects (Dusza et al. Citation2019). Regarding the specific immunoassays, four have been reported for ENL, two validated for plasma (Adlercreutz et al. Citation1998; Shinkaruk et al. Citation2014) and two for urine (Bensaada et al. Citation2023; Uehara et al. Citation2000), but none for END; the reported LOQs are low, varying from 0.1 to 0.5 µg/L. These specific tests allow rapid screening of a high number of samples, but the required synthesis and preparation of antibodies and haptens represents an analytical bottleneck. In contrast, nonspecific tests have the advantage of readily available commercial reactants (Dusza et al. Citation2019), but they are limited by lack of specificity, allowing the estrogenic activity of a sample to be quantified, but without identifying the compounds responsible. As a solution, Nielen et al. (Citation2004) designed a combined HPLC-bioassay-HRMS method, in which the flow was divided after chromatography, one part analyzed in a bioassay for estrogenic activity and the rest injected to an HRMS instrument to identify and quantify the active compounds.
In summary, the most optimal approach for quantifying ENL and END in biological samples is through UHPLC-MS methods that require minimal sample preparation, can quantitate the aglycones separately from the sulfates and glucuronides, and can quantitate a large number of compounds (González-Domínguez, Urpi-Sarda, et al. Citation2020; González-Domínguez, Jáuregui, et al. Citation2020; Laveriano-Santos, Marhuenda-Muñoz et al. Citation2022). These techniques combine a rapid chromatographic analysis and few preparation steps with a high selectivity and sensitivity, allowing large numbers of samples to be analyzed in relatively little time.
Pharmacokinetics and bioavailability of enterolignans
Several studies have investigated the pharmacokinetics and bioavailability of lignans and their metabolic products END and ENL in subjects administered whole, ground, or milled flaxseed (also known as linseed) or isolated lignan extracts. In a study of 12 postmenopausal women who received a single dose of 25 mg/day of 7-hydroxymatairesinol aglycone (7-HMR), levels of 7-HMR and its metabolite ENL were measured in blood samples over 72 h. It was found that 7-HMR was rapidly absorbed into the bloodstream and effectively metabolized to ENL, reaching a maximum concentration (Cmax) (mean + standard deviation) of 4.8 ± 1.64 ng/mL at 24 h (Udani et al. Citation2013). In a similar study by Kuijsten, Arts, Van’t Veer, et al. (Citation2005), this time using a single dose of a purified secoisolariciresinal diglucoside (SDG), the Cmax for END was reached at 14.8 ± 5.1 h and for ENL at 19.7 ± 6.2 h, and the mean area under the curve of ENL was double that of END (Kuijsten, Arts, Tom B., et al. Citation2005). In another study, seven subjects ingesting 500 g of lignan-rich strawberry in a single dose had the highest mean blood and urinary ENL at 24 h and 25–36 h, respectively, as measured by a fluoroimmunoassay, with considerable inter-individual variation (Mazur et al. Citation2000).
In a randomized, cross-over study with healthy women aged 20–40 years receiving daily doses of raw ground flaxseed (5, 15, or 25 g) for 7 or 8 days, a time- and dose-response was observed in urinary lignan excretion. The study found that it takes more than 24 h and possibly as long as a week for urinary enterolignans to reach their peak and level off with a once-daily supplementation of 25 g of flaxseeds, while 24 h is insufficient to see plasma END and ENL return to baseline values (Nesbitt, Lam, and Thompson Citation1999). Similar results were found by Setchell et al. (Citation2014) in a dose-response study in postmenopausal women receiving a single-dose of SDG. The serum concentration of SDG reached a peak at 7.2 ± 1.0 h after exposure, thereafter decreasing rapidly, some of it being biotransformed to END and then ENL in a time-dependent sequence. The time required to reach the Cmax (Tmax) of END in serum was between 12 and 24 h with a half-life of 9.4 h, compared to 24–36 h and a half-life of 13.2 h for ENL. The delayed appearance of END and ENL in serum relative to SDG is consistent with their production in the distal intestine and colon and the known precursor-product relationship between the two enterolignans. Urinary excretion of END and ENL was also delayed, little appearing in the first 12 h and still detectable after 5 days. It was also concluded that a steady-state concentration of lignans would be attained after 5–7 days of daily SDG administration (Setchell et al. Citation2014).
The bioavailability of lignans has been investigated in randomized, controlled intervention trials of 4–8 wk. Knust et al. (Citation2006) reported a 2.6-fold increase in total urinary lignans after 8 wk of supplementation with 20 g flaxseed (9.13 mg SDG/g) in pre- and perimenopausal women. In another study, 31 postmenopausal women underwent a 7-week flaxseed supplementation, which led to a significant linear, dose-response increase in the excretion of END and ENL (Hutchins et al. Citation2000). Pharmacokinetics studies also indicate a significant correlation between urine and serum concentrations (Knust et al. Citation2006; Nesbitt, Lam, and Thompson Citation1999; Setchell et al. Citation2014), making urinary enterolignan concentration a surrogate marker of lignan bioavailability, given that renal clearance and elimination in urine are the predominant route by which lignans are removed from the body.
The END:ENL ratio has also been investigated. After 8 days of lignan supplementation, ratios of END: ENL >1 were found (Nesbitt, Lam, and Thompson Citation1999). However, after 8 wk of ground flaxseed supplementation, the mean END:ENL ratio was 0.9, suggesting a high induction of bacterial enzymes and therefore an almost complete metabolism of the terminal end-product ENL prior to absorption (Knust et al. Citation2006). The rate of ENL formation from END may be influenced by exposure time but can also be explained by the composition of the microbiota, transit time, and the redox level of the large intestine of each subject.
In a comparative study of younger (18–29 years old) and older (45–69 years old) populations consuming 30 g/d of milled flaxseed for 4 wk, differences were observed in END:ENL ratios and inter-group coefficients of variation. The younger group exhibited a higher inter-individual variability in total enterolignans, which could be attributed to the small sample size, age-related differences between groups, gut microbiota, or the lower liver volume of elderly people (Edel, Pierce, and Aliani Citation2015). The differences in enterolignan pharmacokinetics between men and women found in another study were attributed to the smaller blood volume of women, even when adjusted for body weight, which resulted in earlier and higher Cmax values (Kuijsten, Arts, Tom B., et al. Citation2005). A study comparing the metabolism of raw and processed flaxseed in healthy women found that its consumption as an ingredient of muffins or bread did not affect the quantity of lignan excretion (Nesbitt, Lam, and Thompson Citation1999). As the absorption of enterolignans occurs in the colon, the food matrix might not play an important role in enterolignan release, but the crushing and milling of flaxseed was found to improve their bioavailability (Kuijsten, Arts, Van’t Vree et al. Citation2005).
In summary, enterolignans are produced to a greater or lesser extent by the entire population, but wide ranges of concentrations in biofluids have been found. The high interindividual variability can be explained by differences in the composition of human microbiota, transit time, the redox state of the large intestine, and habitual diet. It has also been hypothesized that the variability of lignan excretion in humans is due to the existence of several alternative metabolic pathways or mammalian lignan metabolites that are not routinely measured. Additionally, a 24h-study seems insufficient for accurately determining the pharmacokinetics of END and ENL due to their delayed appearance in serum and urine and slow elimination. Indeed, these factors make the metabolites suitable for use as long-term biomarkers of lignan exposure. Furthermore, dose-response studies have shown that END and ENL concentrations in biofluids reflect the amount of lignans consumed, discriminating between different levels of consumption in a healthy population and allowing quantitative determination of exposure to dietary lignans and related health effects. Finally, interindividual differences in END:ENL ratios have been found, with unknown physiological implications.
Cardiometabolic bioactivity of enterolignans: studies in humans, in vivo and in vitro
Potential effects of enterolignan exposure on cardiometabolic health, including a reduced cardiovascular disease (CVD) risk and mortality, have been reported in several studies (Adlercreutz Citation2007; Adolphe et al. Citation2010; Frankenfeld Citation2017; Peterson et al. Citation2010). This relationship might be influenced by the interplay between lignan intake, gut microbial diversity, and host-microorganism interaction, as shown in . However, the mechanisms of action underlying the impact of enterolignans on cardiometabolic parameters such as inflammation, blood pressure, adiposity, blood lipid, and glucose levels have not been well established. Table S10 includes information on in vivo cardiometabolic studies of enterolignans: study design, subjects, metabolite, findings, and in the case of randomized clinical trials, dose of lignan or lignan source.
Inflammation and oxidative stress
The immune system is a coordinated response of innate and adaptive immunity. Data from several human studies support that END and ENL can scavenge reactive oxygen species (ROS) and exert anti-inflammatory and antioxidative activity (Adolphe et al. Citation2010).
Miles et al. (Citation2021) found that ENL was inversely associated with proteins involved in both innate and adaptive immunity in healthy adults: Fc receptor-like protein 5, which promotes the development and signaling of B cells; GRB2-associated binding protein 1, a regulator of cytokine production; lysosomal-associated protein transmembrane 5, which is involved in cytokine production and autophagy in immune cells; and carcinoembryogenic antigen-related cell adhesion molecule-1, involved in cell adhesion and intracellular signaling. In a study conducted in 6,009 adults aged 40 years or older, higher urinary levels of END and ENL were associated with lower concentrations of serum C-reactive protein (CRP), a biomarker of inflammation. These results remained robust after controlling for confounders such as age, sex, race, education, body mass index (BMI), smoking status, alcohol consumption, and urinary creatinine. Thus, each unit increase in END and ENL was associated with a decrease in CRP of 0.07 and 0.15 units, respectively. Moreover, participants with the highest urinary excretion of ENL (median of 1.630 ng/mL) had a lower risk (odds ratio [OR] = 0.59) of high CRP levels (≥ 3.0 mg/L) compared to those with the lowest excretion of ENL (median of 30 ng/mL) (Reger et al. Citation2017). Shivappa et al. (Citation2019) reported that a pro-inflammatory diet may has a negative effect on microbial diversity, reduces urinary enterolignan levels, and increases the risk of elevated CRP levels (≥ 3.0 mg/L) in adults aged >19 years. Eichholzer et al. (Citation2014) observed that increases in urinary ENL were associated with lower CRP levels. Additionally, higher urinary END and ENL values were associated with 2.1% and 1.3% lower white blood cell counts, respectively (a marker of systemic inflammation) and with lower TXA2:PGI2 ratio and TXA2 levels, these two molecules are important regulator of the interaction between platelets and vessel walls in vivo, and crucial for vascular health (Parilli-Moser et al. Citation2023). Holma et al. (Citation2014) showed that consuming a mixture of probiotics (Lactobacillus, Bifidobacterium, and Propionibacterium strains) and galactooligosaccharides increased serum ENL concentration in human males, but had no significant effect on CRP levels. In contrast with the results from the observational studies described above, a randomized clinical trial with 32 older healthy adults aged between 60 to 80 years found that a daily oral supplementation of 600 mg secoisalariciresinol diglucoside for six months was correlated with plasma concentrations of enterolignans, but did not have a significant effect on tumor necrosis factor-alpha (TNF- α), interleukin 6 (IL-6), or CRP levels (Di et al. Citation2017).
Findings from in vitro studies confirm the anti-inflammatory and antioxidant properties of enterolignans and reveal plausible mechanisms for their effects. As mentioned, enterolignans act as general antioxidants by scavenging harmful ROS, but they also interact directly with enzymes, including those associated with the nitric oxide (NO) system, such as superoxide dismutase (SOD) (Almousa et al. Citation2018). Enterolignans may enhance NO bioactivity by stimulation of endothelial eNOS via the p38 mitogen-activated protein kinase (p38 MAPK) pathway. A study carried out in endothelial cells showed that 50 µM of ENL, produced by ex vivo incubation of human gut microbiota with arctiin and phillyrin from a Forsythia sp. extract, reduced TNF-α and IL-1β production, as well as neutrophil adhesion and the expression of E-selectin, intercellular adhesion molecule-1 (ICAM-1), and β2 integrins DC11a/CD18, CD11b/CD18. These results suggest that lignans, and probably ENL, can attenuate the phosphorylation of proteins such as p38 MAPK and extracellular signal-regulated kinase (ERK) and contribute to reducing inflammation and oxidative stress (Michalak et al. Citation2018). Activation of p38 MAPK in neutrophils has been identified as a key factor in the synthesis of pro-inflammatory cytokines, although the process is unknown (Moens, Kostenko, and Sveinbjørnsson Citation2013; Zu et al. Citation1998). Similarly, activation of the ERK pathway has been shown to result in the expression of pro-inflammatory cytokines (Simard et al. Citation2015). A study by Almousa et al. revealed that the administration of ENL in different concentrations (200 nM and 20,000 nM) increased SOD activity and reduced ROS levels as well as malondialdehyde (MDA) production in macrophages. MDA is a well-established marker of lipid peroxidation closely related to oxidative stress damage. It was also suggested that ENL, along with other bioactive compounds present in flax, may regulate the expression of TNF-α and PPAR-γ, thus contributing to its protective effect against oxidative stress (Almousa et al. Citation2018). A study by Kitts et al. (Citation1999) reported that 10 µM of END and ENL suppressed lipid peroxidation by 20.8% and 31.6%, respectively. This effect can be attributed to the ability of enterolignans (10 and 1,000 µM) to protect DNA from damage, which was demonstrated by a reduction in deoxyribose oxidation and DNA site-specific •OH breakage, even at low concentrations. Another study concluded that physiological concentrations (50 and 100 µM) of END and ENL have a protective effect against DNA damage although to a lesser extent than SDG and SECO (C. Hu, Yuan, and Kitts Citation2007). Other authors found that 2.5 mg/ml of END and ENL added directly to blood reduced the activity of polymorphonuclear leukocytes by 94.2% and 81.6%, respectively, exhibiting higher antioxidant potency than SDG (Prasad Citation2011). However, the concentration of END and ENL in plasma was higher compared to the levels observed in human clinical studies.
Vascular function
Contradictory findings have been reported in the literature regarding the effect of enterolignans on vascular function. A randomized clinical trial conducted in healthy postmenopausal women aged 61 ± 7 years, who were administered 500 mg of SDG daily for six weeks, found no effect on flow-mediated dilation, a biomarker of endothelial function and cardiovascular health (Hallund, Tetens, et al. Citation2006). A case-control study, including 608 case-control pairs, assessed the association between the gut-derived metabolites ENL and trimethylamine N-oxide (TMAO) in plasma and the risk of coronary artery disease (CAD). The results revealed that a higher ENL:TMAO ratio, indicative of a greater intake of plant-based foods and a lower intake of animal-based foods, was associated with a significantly lower CAD risk, with a multivariable-adjusted odds ratio of 0.58. Neither ENL nor TMAO alone were correlated with CAD risk, although higher plasma levels of ENL in women were associated with a 54% lower risk (G. Liu et al. Citation2021). Studies performed with middle-aged men in eastern Finland showed that individuals with higher serum ENL levels had a 65.3% lower risk of acute coronary events (Vanharanta et al. Citation1999). This was supported by a 56% lower incidence of mortality related to coronary heart disease and a 45% reduction in CVD-related mortality (Vanharanta et al. Citation1999, Citation2003).
Platelet aggregation
P-selectin, a cell adhesion molecule, is commonly employed as a biomarker of platelet aggregation (Merten and Thiagarajan Citation2000). Another biomarker linked to platelet function is the Von Willebrand Factor (VWF), a large glycoprotein involved in thrombus formation (Ruggeri Citation2007). A study showed that rats fed a specific-pathogen-free diet had higher serum levels of ENL compared to rats on a germ-free diet, the latter group also developing more pronounced endothelium plaque lesions (VWF-positive endothelial cells) in their aorta and coronary arteries from weeks 13 to 52. These differences were related to a higher accumulation of macrophages and higher expression of TNF-α and IL-6 in cells in the arterial walls of the rats on the germ-free diet (Chuang et al. Citation2022). In humans, ENL and END have been associated. with lower TXA2:PGI2 ratio and TXA2 levels, these two molecules are important regulator of the interaction between platelets and vessel walls in vivo, and crucial for vascular health (Parilli-Moser et al. Citation2023). It was further concluded that the gut microbiota may modulate the production of ENL and END, which exhibits anti-inflammatory properties and prevents plaque formation in blood vessels, although the underlying molecular mechanisms are still not fully understood (Chuang et al. Citation2022).
Blood pressure
The literature about the effect of enterolignans on blood pressure (BP) is controversial. A nested case-control study showed that individuals with a minimum of 76.4 nmol/L of plasma ENL had a significantly lower risk of prehypertension and hypertension compared with those with less than 31.6 nmol/L, especially in participants with obesity (J. Lee et al. Citation2021). In contrast, results from a large survey found no association between urinary ENL and high BP in a sample of 2,260 adults (≥20 years) from the 2003–2010 US National Health and Nutrition Examination Surveys (NHANES) (Frankenfeld Citation2014). Similarly, urinary ENL and ED were not associated with BP parameters in two cross-sectional studies with 200 participants at high risk for CVD or in 560 young adolescents (Domínguez-López et al. Citation2023; Laveriano-Santos, Quifer-Rada, et al. Citation2022). A prospective case-control study also observed no association between serum ENL and BP in 760 males aged 50–69 years (Kilkkinen et al. Citation2006). However, a cross-sectional analysis of 2,429 children and adolescents from the 2009–2010 NHANES found that urinary ENL, but not END, was inversely associated with systolic blood pressure (SBP). Nevertheless, when participants were categorized by high SBP (greater than or equal to 140 mm Hg), no significant association was found (Jeng, Kantaria, and Beydoun Citation2015). In contrast, results from a Spanish adolescent cohort (n = 560) revealed no association between urinary enterolignans and BP parameters (Laveriano-Santos, Quifer-Rada, et al. Citation2022). Different results have been obtained in an adult population, as Struja et al. (Citation2014) found that 1,748 US adults with the highest excretion of enterolignans (more than 794 µg/g creatinine) had a lower risk of hypertension compared to those with the lowest excretion (less than 153 µg/g creatinine), although the mean BP did not differ between lignan excretion categories.
In addition, supplementation with 30 g of milled flaxseed for 6 months led to a reduction of 10 mmHg of SBP and 7 mmHg of DBP compared with the control group (Rodriguez-Leyva et al. Citation2013). Similar results were found in a study by Di et al. (Citation2017), where older healthy adults with high SBP (greater than or equal to 140 mmHg) receiving a daily dose of 600 mg SDG from a flaxseed lignan complex for 24 wk had increased plasma levels of enterolignans. After the intervention, their SBP values were 15 mmHg lower compared to the placebo group. In line with these findings, a study found that participants who consumed 32% carbohydrates and 60 g of flaxseed powder daily for 42 days had higher serum levels of END and ENL, as well as urinary levels of ENL, compared to baseline.
Plasma or serum lipid levels
Studies have shown that the consumption of high levels of lignans can have a positive effect on the lipid profile, i.e., the levels of total cholesterol (TC), low-density lipoprotein cholesterol (LDL-c), high-density lipoprotein cholesterol (HDL-c), and triacylglycerols (TAG). In a cross-over study of 40 men with metabolic syndrome, LDL-c and TC concentrations were lower after 4 wk on a whole-grain rye diet (30% of daily energy intake) compared with a wheat diet (Eriksen et al. Citation2020). The authors showed that the rye diet improved the lipid profile in adults, and additional supplementation with lignan capsules containing 280 mg SDG resulted in the highest plasma and urinary concentrations of END and ENL (Eriksen et al. Citation2020). In addition, a daily supplementation with 300 or 600 mg of SDG from a flaxseed extract for 8 wk reduced TC, HDL-c, and LDL-c in adults with hypercholesterolemia compared to baseline, and the observed lower lipid levels (TC, LDL-c, HDL-c, and TAG) were correlated with plasma END (W. Zhang et al. Citation2008). Another study showed that after supplementation with 0.3 g/kg/day of flaxseed for 7 days, serum ED and ENL levels increased approximately 10-fold (143 nM after intervention). Furthermore, flaxseed supplementation regulated intestinal microflora such as Ruminococcaceae, which was positively associated with ENL production and LDL-c levels (Lagkouvardos et al. Citation2015). In contrast with these previous studies, other study reported a significantly increased concentration of enterolignans in the blood and urine of healthy postmenopausal women who consumed low-fat muffins enriched with a lignan complex every day for 6 wk, but no effect on the plasma lipid profile was observed (Hallund, Ravn-Haren, et al. Citation2006). Controversial results have also been found in observational studies. Frankenfeld (Citation2014) reported that ENL was inversely associated with lower HDL-c levels (<40 mg/dL). In another cohort, US adults with the highest quartile of urinary enterolignan levels had significantly lower plasma TAG levels and higher HDL-c levels than those in the lowest quartile (Struja et al. Citation2014). Similar results were found in a cross-sectional analysis of 1,492 adults from the 1999–2004 NHANES, with a mean urinary enterolignan concentration of 1.9 µmol/L. The participants with the highest excretion of enterolignans had higher levels of HDL-c and lower levels of TAG than those in the lowest tertile (Peñalvo and López-Romero Citation2012). Meanwhile, Domínguez-López et al. (Citation2023) and Liu et al. (Citation2018) found no association between enterolignans and blood lipids.
Results from in vitro and in vivo studies support some of the findings described above. Owen et al. described the phytoestrogenic role of END in the macrophage uptake of atherogenic lipoproteins such as LDL-c and lipoprotein(a) (Lp(a)) (Owen and Abbey Citation2004). Although similar in structure, Lp(a) differs from LDL-c in that it contains a specific protein, apo(a), which is an important risk factor for CVD. However, unlike LDL, Lp(a) levels are less sensitive to dietary changes because they are not easily altered by HMG-CoA reductase inhibitors (Enkhmaa et al. Citation2020). A study found that END (10 μM) reduced the uptake of Lp(a) into macrophages, but had no effect on the LDL-c uptake (Owen and Abbey Citation2004).
The effect of ENL on the lipid profile can be attributed to its potential antioxidant capacity, as proposed by Fortin et al. (Citation2017). They found that ENL prevented oxidative liver damage induced by a diet high in polyunsaturated fatty acids in early lactating cows. However, ENL increased the expression of genes encoding enzymes involved in lipid metabolism, such as MLX interacting protein-like, fatty acid (FA) synthase and stearoyl-CoA desaturase, suggesting that flax lignan supplementation alone may increase hepatic TAG accumulation in dairy cows. In addition, Biasiotto et al. (Citation2018) suggested that ENL and END, gut microbiota metabolites of 7-hydroxymatairesinol, may inhibit adipogenesis genes such as PPARγ, C/EBPα and aP2, and reduce TAG uptake in 3T3-L1 cells. Drygalski et al. (Citation2017) showed that 50 µM of ENL protects against liver steatosis in the HepG2 human liver cell line by stimulating the extracellular free FA and TAG efflux into the medium, inhibiting β-oxidation, reducing the expression of enzymes involved in the regulation of lipid metabolism such as carnitine palmitoyltransferase 1 (CPT1), AMP-activated protein kinase (pAMPK), and peroxisome proliferator-activated receptor alpha (PPARα), and increasing sterol regulatory element-binding protein 1c (SREBP1c) expression. CPT1 is an enzyme implicated in the transport of FA into the mitochondria membrane for β-oxidation, and defects in its activity have been linked to metabolic disorders, such as insulin resistance, obesity, fatty liver disease, and cancer (Fang et al. Citation2022; M. Wang et al. Citation2021). AMPK controls cellular metabolism and promotes cell proliferation by regulating the oxidation and synthesis of FA and TAG (Shen et al. Citation2019). PPARα is a transcription factor that promotes the transcription of genes involved in FA uptake, transport, and β-oxidation to control lipid homeostasis (Lin, Wang, and Li Citation2022). SREBP1c is also a transcription factor implicated in the transcription of genes related to lipid synthesis in the liver, such as fatty acid synthase, stearoyl-CoA desaturase, acetyl-CoA carboxylase, and diacylglycerol acyltransferase (Yang et al. Citation2022).
Adiposity
A cross-sectional study with 6,806 participants evaluated the association of urinary enterolignan concentration with adiposity. Those individuals with higher END excretion had a lower likelihood of being obese or overweight or having a high-risk waist circumference. Regarding ENL, higher concentrations in urine were associated with a lower risk of obesity and overweight. In this study, age and sex did not modify the associations (Frankenfeld Citation2013). Y. Hu et al. (Citation2015) analyzed the associations between urinary ENL and END in a prospective study with women. Women with a higher excretion of total lignans were associated with a lower BMI, and those with a higher END excretion gained less weight in the following 10 years. These results suggest that lignans, specially END, contribute to slower weight gain. X. Wu et al. (Citation2012) examined urinary excretion of phytoestrogens in a population-based cohort study that included 2,165 adult women. A weak inverse association was found between END and ENL and BMI. Surprisingly, ENL excretion was associated with a higher fat intake. A large cross-sectional study assessed the levels of urinary phytoestrogens in both adults and children. Interestingly, a stronger negative association was found for ENL with obesity and waist circumference in adult males aged 20–60 years compared to children and older adults (C. Xu et al. Citation2015). The effects of lignans have also been studied in post-menopausal women. A cross-sectional study measuring dietary lignans and plasma ENL found that women who consumed more lignans, and therefore had higher concentrations of plasma ENL, had lower BMI and total body fat mass (Morisset et al. Citation2009). In a longitudinal study assessing the effect of phenolic compound exposure during childhood, Deierlein et al. found that ENL was inversely associated with BMI, waist circumference, and percent of body fat (Deierlein et al. Citation2017). The effects of phytoestrogen exposure on pubertal stages in girls were evaluated by Wolff et al. (Citation2010) in a multi-ethnic longitudinal study. They found that the association between BMI and breast development was modified by ENL. In girls with low exposure to ENL, breast development differed depending on the BMI, whereas with high exposure, there was no significant association (Wolff et al. Citation2010).
The benefits of END and ENL on adiposity may be related to their ability to modulate gene expression. It has been observed that phytoestrogens can inhibit adipose cell differentiation in vitro (Taxvig et al. Citation2013). Another in vitro study showed that in adipocyte-like phenotype cells, END and ENL inhibited the expression of PPARγ, C/EBPα and aP2, proteins that modulate fat metabolism and play a key role in the final phases of adipocyte differentiation. In addition, they also prevented triglyceride uptake in hepatoma cells (Biasiotto et al. Citation2018). In an in vivo study in mice, Fukumitsu et al. (Citation2008) found that END induces the mRNA expression of two genes related to adiposity, adiponectin and PPAR-γ.
Glucose metabolism
Evidence for the effect of END and ENL on glucose metabolism is contradictory. A cross-sectional study conducted in children and adolescents found a direct association between fasting glucose and urinary END (Jeng, Kantaria, and Beydoun Citation2015). Similarly, a positive association between blood glucose and urinary END was observed in adults (Frankenfeld Citation2014). In contrast, other studies have found no association between these metabolites in plasma or urine and glucose or type-2 diabetes (J. Liu et al. Citation2018; Marhuenda-Muñoz et al. Citation2022; Talaei et al. Citation2016).
Nevertheless, most research in this field has reported beneficial effects of enterolignans on glucose metabolism. A cross-over study in which 29 adults consumed whole pea flour and fractionated pea flour detected an increase in urinary ENL, although without any changes in fasting insulin or insulin resistance (Marinangeli and Jones Citation2011). The effects of a flaxseed lignan extract were evaluated in a randomized clinical trial with 55 hypercholesterolemic subjects. As expected, plasma END and ENL increased in the group that consumed the dietary supplement, the participants also experiencing a reduction in fasting glucose (W. Zhang et al. Citation2008).
Another cross-over study administering dietary flaxseed lignans in patients with type-2 diabetes found an improvement in glycaemic control after consumption of the supplement, but fasting glucose and insulin sensitivity were not affected (Pan et al. Citation2007). In post-menopausal women, Morisset et al. (Citation2009) reported that participants with higher concentrations of plasma ENL showed better insulin sensitivity, as they had lower insulin resistance and 2h postload glycemia, and a higher glucose disposal rate.
A few mechanisms have been proposed to explain the effects of enterolignans on glucose metabolism. In vitro, it has been demonstrated that ENL can suppress insulin signaling protein phosphorylation in hepatic cells, which reduces insulin sensitivity and therefore insulin resistance in the liver (Charytoniuk et al. Citation2019). ENL also promoted glucose uptake through translocation of the GLUT4 transporter to plasma membrane in L6 myoblasts. These results were corroborated in vivo in a db/db mouse model of type-2 diabetes, as it improved fasting blood glucose and the index of insulin resistance (Zhou et al. Citation2017).
Conclusions and future challenges
This review of state-of-the-art research on enterolignans has focused above all on their effects on cardiometabolic health, but also includes other aspects to provide a comprehensive overview of these gut metabolites. Strategies to synthesize ENL and END have been developed since the early 1980s, centered on novel metal or organocatalytic reactions, achieving their stereoselective synthesis, and providing pure standards for analytical investigation. To understand their health effects, the quantification of ENL and END is crucial, especially in biological samples. Among the different methods used, UHPLC-MS stands out for its high selectivity and sensitivity, rapid analysis with minimal sample preparation, and capacity to handle large sample volumes. Technical advances now allow END and ENL to be quantified with a huge amount of other bioactive compounds. With information about the concentration of each compound and inter-individual differences in concentration, it is possible to discern which compounds are associated with any perceived health effects.
After the ingestion of lignan precursors, whose main food sources are whole cereals and seeds, they undergo bacterial transformation to enterolignans. Concentrations of the metabolites ENL and END in biofluids vary between individuals, due to differences in gut microbiota, transit time, redox state, and dietary patterns. Other factors such as alternative pathways or unmeasured metabolites may also contribute to the variability in enterolignan excretion. Due to their delayed appearance and slow elimination, END and ENL serve as long-term biomarkers of dietary lignan exposure in dose-response studies.
Regarding the cardiometabolic effects of enterolignans, most available data are based on observational studies, which provide conflicting results. Interpretation of these findings should take into account the potentially confounding variables like a low consumption of animal foods and the high fiber content of lignan-rich foods. Human trials have revealed positive effects of ligan intake on parameters related to inflammation, oxidative stress, blood pressure, lipid profile, and glucose metabolism, which have been associated with higher enterolignan levels in urine and/or plasma.
Future research on enterolignans should include mechanistic investigations, larger studies with comprehensive and variable controls, the use of enterolignans as long-term biomarkers, and comparative studies to understand the differential effects of dietary lignans and enterolignans, with a focus on inter-individual variability. Taking on these research challenges will contribute to a deeper understanding of the role of enterolignans in improved cardiometabolic health and their potential therapeutic implications.
Author contributions
Conceptualization: AVQ, RMLR, MP; Data curation: EPLS, CLC, MTS, JLC, IDL, AVQ, MP; Visualization: EPLS, MP; Writing – original draft: EPLS, CLC, MTS, JLC, IDL, S.C.-B, AVQ, MP; Writing – review & editing: EPLS, RMLR, MP, S.C.-B. All authors read and approved the final manuscript.
Abbreviations | ||
AUC | = | area under the curve |
BMI | = | body mass index |
BP | = | blood pressure |
CAD | = | colorimetric array detector |
Cmax | = | maximum concentration |
CVD | = | cardiovascular disease |
CHD | = | coronary heart disease |
CPT1 | = | palmitoyltransferase 1 |
CRP | = | serum C-reactive protein |
DAD | = | diode array detector |
DBU | = | 1,8-Diazabicyclo(5.4.0)undec-7-ene |
END | = | Enterodiol |
ENL | = | Enterolactone |
eNOS | = | endothelial nitric oxide Synthase |
ERK | = | extracellular signal-regulated kinase |
EtOH | = | ethanol |
FA | = | fatty acid |
FMD | = | flow-mediated dilation |
HDL-c | = | high-density lipoprotein cholesterobioactive compoundsl |
HPLC | = | high performance liquid chromatography |
HRMS | = | high-resolution mass spectrometry |
ICAM-1 | = | intercellular adhesion molecule-1 |
LAR | = | lariciresinol |
LDL-c | = | low-density lipoprotein cholesterol |
LiHMDS | = | Lithium bis(trimethylsilyl)amide |
LLE | = | liquid-liquid extraction |
LOD | = | limit of detection |
LOQ | = | limit of quantification |
Lp(a) | = | lipoprotein(a) |
MAPK | = | mitogen-activated protein kinase |
MDA | = | malondialdehyde |
MAT | = | matairesinol |
MED | = | medioresinol |
MS | = | Mass spectrometry |
MS/MS | = | mass spectrometry in tandem |
NO | = | nitric oxide |
pAMPK | = | AMP-activated protein kinase |
PIN | = | pinoresinol |
PMNLs | = | polymorphonuclear leukocytes |
PUFAs | = | polyunsaturated fatty acids |
PPARα | = | peroxisome proliferator-activated receptor alpha |
OR | = | odds ratio |
RCT | = | randomized clinical trial |
ROS | = | reactive oxygen species |
SBP | = | systolic blood pressure |
SDG | = | secoisolariciresinal diglucoside |
SECO | = | Secoisolariciresinol |
SES | = | Sesamin |
SOD | = | superoxide dismutase |
SPE | = | solid phase extraction |
SREBP1c | = | sterol regulatory element-binding protein 1c |
SYR | = | syringaresino |
TAG | = | triacylglycerols |
TC | = | total cholesterol |
TMSCl | = | Trimethylsilyl chloride |
TMAO | = | N-óxido trimetilamina |
UHPLC | = | ultra-high performance liquid chromatography. |
Supplemental Material
Download MS Word (1.2 MB)Disclosure statement
No potential conflict of interest was reported by the authors.
Additional information
Funding
References
- Abbafati, C., M. Kaja, M. Abbas, F. Abbasi-Kangevari, A. Abd-Allah, M. Abdelalim, I. Abdollahi, K. Abdollahpour, A. Hussein, A. Hassan, et al. 2020. Global burden of 369 diseases and injuries in 204 countries and territories, 1990–2019: A systematic analysis for the Global Burden of Disease Study 2019. Lancet 396 (10258):1204–22. doi: 10.1016/S0140-6736(20)30925-9.
- Adlercreutz, H., T. Fotsis, M. S. Kurzer, K. Wähälä, T. Mäkelä, and T. Hase. 1995. Isotope dilution gas chromatographic-mass spectrometric method for the determination of unconjugated lignans and isoflavonoids in human feces, with preliminary results in omnivorous and vegetarian women. Analytical Biochemistry 225 (1):101–8. doi: 10.1006/abio.1995.1114.
- Adlercreutz, H. 2007. Lignans and human health. Critical Reviews in Clinical Laboratory Sciences 44 (5–6):483–525. doi: 10.1080/10408360701612942.
- Adlercreutz, H., G. J. Wang, O. Lapcík, R. Hampl, K. Wähälä, T. Mäkelä, K. Lusa, M. Talme, and H. Mikola. 1998. Time-resolved fluoroimmunoassay for plasma enterolactone. Analytical Biochemistry 265 (2):208–15. doi: 10.1006/abio.1998.2886.
- Adolphe, J. L., S. J. Whiting, B. H. J. Juurlink, L. U. Thorpe, and J. Alcorn. 2010. Health effects with consumption of the flax lignan secoisolariciresinol diglucoside. The British Journal of Nutrition 103 (7):929–38. doi: 10.1017/S0007114509992753.
- Adriouch, S., E. Kesse-Guyot, T. Feuillet, M. Touvier, V. Olié, V. Andreeva, S. Hercberg, P. Galan, and L. K. Fezeu. 2017. Total and specific dietary polyphenol intakes and 6-year anthropometric changes in a middle-aged general population cohort. International Journal of Obesity 342 (3):310–7. doi: 10.1038/ijo.2017.227.
- Almousa, A. A., F. Meurens, E. S. Krol, and J. Alcorn. 2018. Linoorbitides and enterolactone mitigate inflammation-induced oxidative stress and loss of intestinal epithelial barrier integrity. International Immunopharmacology 64 (November):42–51. doi: 10.1016/j.intimp.2018.08.012.
- Aresta, A., P. Cotugno, and C. Zambonin. 2019. Solid-phase microextraction and on-fiber derivatization for assessment of mammalian and vegetable milks with emphasis on the content of major phytoestrogens. Scientific Reports 9 (1):6398. doi: 10.1038/s41598-019-42883-7.
- Axelson, M., J. Sjövall, B. E. Gustafsson, and K. D. R. Setchell. 1982. Origin of lignans in mammals and identification of a precursor from plants. Nature 298 (5875):659–60. doi: 10.1038/298659a0.
- Baldi, S., M. Tristán Asensi, M. Pallecchi, F. Sofi, G. Bartolucci, and A. Amedei. 2023. Interplay between lignans and gut microbiota: Nutritional, functional and methodological aspects. Molecules 28 (1):343. doi: 10.3390/molecules28010343.
- Bensaada, S., I. Raymond, I. Pellegrin, J.-F. Viallard, and C. Bennetau-Pelissero. 2023. Validation of ELISAs for isoflavones and enterolactone for phytoestrogen intake assessment in the French population. Nutrients 15 (4):967. doi: 10.3390/NU15040967/S1.
- Biasiotto, G., I. Zanella, F. Predolini, I. Archetti, M. Cadei, E. Monti, M. Luzzani, B. Pacchetti, P. Mozzoni, R. Andreoli, et al. 2018. 7-Hydroxymatairesinol improves body weight, fat and sugar metabolism in C57BJ/6 mice on a high-fat diet. The British Journal of Nutrition 120 (7):751–62. doi: 10.1017/S0007114518001824.
- CDC-Division of Laboratory Sciences. 2004. Phytoestrogens: Genistein, daidzein, equol, enterodiol, enterolactone, O-desmethylangolensin. https://www.cdc.gov/nchs/data/nhanes/nhanes_03_04/l06phy_c_met.pdf.
- Charytoniuk, T., N. Iłowska, K. Berk, K. Drygalski, A. Chabowski, and K. Konstantynowicz-Nowicka. 2019. The effect of enterolactone on sphingolipid pathway and hepatic insulin resistance development in HepG2 cells. Life Sciences 217 (January):1–7. doi: 10.1016/J.LFS.2018.11.044.
- Choi, M. H., K.-R. Kim, J. K. Hong, S. J. Park, and B. C. Chung. 2002. Determination of non-steroidal estrogens in breast milk, plasma, urine and hair by gas chromatography/mass spectrometry. Rapid Communications in Mass Spectrometry 16 (24):2221–8. doi: 10.1002/rcm.845.
- Chuang, H.-L., C.-C. Chiu, C. Lo, C.-C. Hsu, J.-Y. Liu, S.-W. Hung, S.-C. Tsai, H.-H. Sung, C.-K. L. Wang, and Y.-T. Huang. 2022. Circulating gut microbiota-related metabolites influence endothelium plaque lesion formation in ApoE knockout rats. PLOS One 17 (5):e0264934. doi: 10.1371/journal.pone.0264934.
- Clavel, T., G. Henderson, W. Engst, J. Doré, and M. Blaut. 2006. Phylogeny of human intestinal bacteria that activate the dietary lignan secoisolariciresinol diglucoside. FEMS Microbiology Ecology 55 (3):471–8. doi: 10.1111/j.1574-6941.2005.00057.x.
- De Silva, S. F., and J. Alcorn. 2019. Flaxseed lignans as important dietary polyphenols for cancer prevention and treatment: Chemistry, pharmacokinetics, and molecular targets. Pharmaceuticals 12 (2):68. doi: 10.3390/ph12020068.
- Deierlein, A. L., M. S. Wolff, A. Pajak, S. M. Pinney, G. C. Windham, M. P. Galvez, M. Rybak, A. M. Calafat, L. H. Kushi, F. M. Biro, et al. 2017. Phenol concentrations during childhood and subsequent measures of adiposity among young girls. American Journal of Epidemiology 186 (5):581–92. doi: 10.1093/aje/kwx136.
- Di, Y., J. Jones, K. Mansell, S. Whiting, S. Fowler, L. Thorpe, J. Billinsky, N. Viveky, P. C. Cheng, A. Almousa, et al. 2017. Influence of flaxseed lignan supplementation to older adults on biochemical and functional outcome measures of inflammation. Journal of the American College of Nutrition 36 (8):646–53. doi: 10.1080/07315724.2017.1342213.
- Domínguez-López, I., C. Arancibia-Riveros, M. Marhuenda-Muñoz, A. Tresserra-Rimbau, E. Toledo, M. Fitó, E. Ros, R. Estruch, and R. M. Lamuela-Raventós. 2023. Association of microbiota polyphenols with cardiovascular health in the context of a Mediterranean diet. Food Research International 165 (March):112499. doi: 10.1016/j.foodres.2023.112499.
- Drygalski, K., K. Berk, T. Charytoniuk, N. Iłowska, B. Łukaszuk, A. Chabowski, and K. Konstantynowicz-Nowicka. 2017. Does the enterolactone (ENL) affect fatty acid transporters and lipid metabolism in liver? Nutrition & Metabolism 14 (1):69. doi: 10.1186/s12986-017-0223-1.
- Dusza, H. M., E. Janssen, R. Kanda, and J. Legler. 2019. Method development for effect-directed analysis of endocrine disrupting compounds in human amniotic fluid. Environmental Science & Technology 53 (24):14649–59. doi: 10.1021/acs.est.9b04255.
- Edel, A. L., G. N. Pierce, and M. Aliani. 2015. Age-dependency in the metabolism of flaxseed lignans by healthy adults. Journal of Functional Foods 17 (August):948–57. doi: 10.1016/j.jff.2015.06.042.
- Eichholzer, M., A. Richard, H. L. Nicastro, E. A. Platz, J. Linseisen, and S. Rohrmann. 2014. Urinary lignans and inflammatory markers in the US National Health and Nutrition Examination Survey (NHANES) 1999–2004 and 2005–2008. Cancer Causes & Control: CCC 25 (3):395–403. doi: 10.1007/s10552-014-0340-3.
- Enkhmaa, B., K. S. Petersen, P. M. Kris-Etherton, and L. Berglund. 2020. Diet and Lp(a): Does dietary change modify residual cardiovascular risk conferred by Lp(a)? Nutrients 12 (7):2024. doi: 10.3390/nu12072024.
- Eriksen, A. K., C. Brunius, M. Mazidi, P. M. Hellström, U. Risérus, K. N. Iversen, R. Fristedt, L. Sun, Y. Huang, N. P. Nørskov, et al. 2020. Effects of whole-grain wheat, rye, and lignan supplementation on cardiometabolic risk factors in men with metabolic syndrome: A randomized crossover trial. The American Journal of Clinical Nutrition 111 (4):864–76. doi: 10.1093/ajcn/nqaa026.
- Fang, C., J. Pan, N. Qu, Y. Lei, J. Han, J. Zhang, and D. Han. 2022. The AMPK pathway in fatty liver disease. Frontiers in Physiology 13 (August):970292. doi: 10.3389/fphys.2022.970292.
- Fortin, É., R. Blouin, J. Lapointe, H. V. Petit, and M.-F. Palin. 2017. Linoleic acid, α-linolenic acid and enterolactone affect lipid oxidation and expression of lipid metabolism and antioxidant-related genes in hepatic tissue of dairy cows. The British Journal of Nutrition 117 (9):1199–211. doi: 10.1017/S0007114517000976.
- Fotsis, T., R. Heikkinen, H. Adlercreutz, M. Axelson, and K. D. Setchell. 1982. Capillary gas chromatographie method for the analysis of lignans in human urine. Clinica Chimica Acta; International Journal of Clinical Chemistry 121 (3):361–71. doi: 10.1016/0009-8981(82)90245-5.
- Franke, A. A., L. J. Custer, L. R. Wilkens, L. L. Le Marchand, A. M. Y. Nomura, M. T. Goodman, and L. N. Kolonel. 2002. Liquid chromatographic–photodiode array mass spectrometric analysis of dietary phytoestrogens from human urine and blood. Journal of Chromatography. B, Analytical Technologies in the Biomedical and Life Sciences 777 (1–2):45–59. doi: 10.1016/S1570-0232(02)00216-7.
- Frankenfeld, C. L. 2013. Relationship of obesity and high urinary enterolignan concentrations in 6806 children and adults: Analysis of National Health and Nutrition Examination Survey Data. European Journal of Clinical Nutrition 67 (8):887–9. doi: 10.1038/ejcn.2013.107.
- Frankenfeld, C. L. 2014. Cardiometabolic risk factors are associated with high urinary enterolactone concentration, independent of urinary enterodiol concentration and dietary fiber intake in adults. The Journal of Nutrition 144 (9):1445–53. doi: 10.3945/jn.114.190512.
- Frankenfeld, C. L. 2017. Cardiometabolic risk and gut microbial phytoestrogen metabolite phenotypes. Molecular Nutrition & Food Research 61 (1):1500900. doi: 10.1002/mnfr.201500900.
- Fukumitsu, S., K. Aida, N. Ueno, S. Ozawa, Y. Takahashi, and M. Kobori. 2008. Flaxseed lignan attenuates high-fat diet-induced fat accumulation and induces adiponectin expression in mice. The British Journal of Nutrition 100 (3):669–76. doi: 10.1017/S0007114508911570.
- Gamache, P. H., and I. N. Acworth. 1998. Analysis of phytoestrogens and polyphenols in plasma, tissue, and urine using HPLC with coulometric array detection. Proceedings of the Society for Experimental Biology and Medicine 217 (3):274–80. doi: 10.3181/00379727-217-44232.
- Gaya, P., J. L. Arqués, M. Medina, I. Álvarez, and J. M. Landete. 2016. A new HPLC-PAD/HPLC-ESI-MS method for the analysis of phytoestrogens produced by bacterial metabolism. Food Analytical Methods 9 (2):537–47. doi: 10.1007/s12161-015-0226-3.
- González-Domínguez, R., O. Jáuregui, P. Mena, K. Hanhineva, F. J. Tinahones, D. Angelino, and C. Andrés-Lacueva. 2020. Quantifying the human diet in the crosstalk between nutrition and health by multi-targeted metabolomics of food and microbiota-derived metabolites. International Journal of Obesity 44 (12):2372–81. doi: 10.1038/s41366-020-0628-1.
- González-Domínguez, R., M. Urpi-Sarda, O. Jáuregui, P. W. Needs, P. A. Kroon, and C. Andrés-Lacueva. 2020. Quantitative dietary fingerprinting (QDF)—a novel tool for comprehensive dietary assessment based on urinary nutrimetabolomics. Journal of Agricultural and Food Chemistry 68 (7):1851–61. doi: 10.1021/acs.jafc.8b07023.
- Hallund, J., G. Ravn-Haren, S. Bügel, T. Tholstrup, and I. Tetens. 2006. A lignan complex isolated from flaxseed does not affect plasma lipid concentrations or antioxidant capacity in healthy postmenopausal women. The Journal of Nutrition 136 (1):112–6. doi: 10.1093/jn/136.1.112.
- Hallund, J., I. Tetens, S. Bügel, T. Tholstrup, M. Ferrari, T. Teerlink, A. Kjaer, and N. Wiinberg. 2006. Daily consumption for six weeks of a lignan complex isolated from flaxseed does not affect endothelial function in healthy postmenopausal women. The Journal of Nutrition 136 (9):2314–8. doi: 10.1093/jn/136.9.2314.
- Heinonen, S., T. Nurmi, K. Liukkonen, K. Poutanen, K. Wähälä, T. Deyama, S. Nishibe, and H. Adlercreutz. 2001. In vitro metabolism of plant lignans: New precursors of mammalian lignans enterolactone and enterodiol. Journal of Agricultural and Food Chemistry 49 (7):3178–86. doi: 10.1021/jf010038a.
- Hemalatha, S., and Ghafoorunissa. 2004. Lignans and tocopherols in Indian sesame cultivars. Journal of the American Oil Chemists’ Society 81 (5):467. doi: 10.1007/s11746-004-0924-5.
- Hemalatha, S., and Ghafoorunissa. 2007. Sesame lignans enhance the thermal stability of edible vegetable oils. Food Chemistry 105 (3):1076–1085. doi: 10.1016/j.foodchem.2007.05.023.
- Holma, R., R. A. Kekkonen, K. Hatakka, T. Poussa, H. Vapaatalo, H. Adlercreutz, and R. Korpela. 2014. Low serum enterolactone concentration is associated with low colonic Lactobacillus – Enterococcus counts in men but is not affected by a synbiotic mixture in a randomised, placebo-controlled, double-blind, cross-over intervention study. The British Journal of Nutrition 111 (2):301–9. doi: 10.1017/S0007114513002420.
- Holmbom, B., C. Eckerman, P. Eklund, J. Hemming, L. Nisula, M. Reunanen, R. Sjöholm, A. Sundberg, K. Sundberg, and S. Willför. 2003. Knots in trees – a new rich source of lignans. Phytochemistry Reviews 2 (3):331–40. doi: 10.1023/B:PHYT.0000045493.95074.a8.
- Horn-Ross, P. L., S. Barnes, M. Lee, L. Coward, J. E. Mandel, J. Koo, E. M. John, and M. Smith. 2000. Assessing phytoestrogen exposure in epidemiologic studies: Development of a database (United States). Cancer Causes & Control 11 (4):289–98. doi: 10.1023/a:1008995606699.
- Hu, C., Y. V. Yuan, and D. D. Kitts. 2007. Antioxidant activities of the flaxseed lignan secoisolariciresinol diglucoside, its aglycone secoisolariciresinol and the mammalian lignans enterodiol and enterolactone in vitro. Food and Chemical Toxicology 45 (11):2219–27. doi: 10.1016/j.fct.2007.05.017.
- Hu, Y., Y. Song, A. A. Franke, F. B. Hu, R. M. van Dam, and Q. Sun. 2015. A prospective investigation of the association between urinary excretion of dietary lignan metabolites and weight change in US women. American Journal of Epidemiology 182 (6):503–11. doi: 10.1093/aje/kwv091.
- Hutchins, A. M., M. C. Martini, B. A. Olson, W. Thomas, and J. L. Slavin. 2000. Flaxseed influences urinary lignan excretion in a dose-dependent manner in postmenopausal women. Cancer Epidemiology, Biomarkers & Prevention 9 (10):1113–8.
- Jeng, H. A. C., K. Kantaria, and H. A. Beydoun. 2015. Urinary phytoestrogens in relation to metabolic disturbances among children and adolescents. Journal of Environmental Science and Health. Part. B, Pesticides, Food Contaminants, and Agricultural Wastes 50 (2):121–7. doi: 10.1080/03601234.2015.975618.
- Jiang, R., T. Abe, D.-Y. Zhou, K. Asano, T. Suzuki, H. Sasai, T. , Suzuki, and Ismiyarto. 2022. Using α- and β-epimerizations of Cis -2,3-bis(hydroxymethyl)-γ-butyrolactone for the synthesis of both enantiomers of enterolactone. The Journal of Organic chemistry 87 (8):5051–5056. doi: 10.1021/acs.joc.1c02801.
- Kamal-Eldin, A., and L. Å. Appelqvist. 1994. Variations in the composition of sterols, tocopherols and lignans in seed oils from four Sesamum species. Journal of the American Oil Chemists’ Society 71 (2):149–56. doi: 10.1007/BF02541549.
- Kilkkinen, A., I. Erlund, M. J. Virtanen, G. Alfthan, K. Ariniemi, and J. Virtamo. 2006. Serum enterolactone concentration and the risk of coronary heart disease in a case-cohort study of Finnish male smokers. American Journal of Epidemiology 163 (8):687–93. doi: 10.1093/aje/kwj080.
- Kitts, D. D., Y. V. Yuan, A. N Wijewickreme, and L. U. Thompson. 1999. Antioxidant activity of the flaxseed lignan secoisolariciresinol diglycoside and its mammalian lignan metabolites enterodiol and enterolactone. Molecular and Cellular Biochemistry 202 (1–2):91–100. doi: 10.1023/a:1007022329660.
- Knust, U., B. Spiegelhalder, T. Strowitzki, and R. W. Owen. 2006. Contribution of linseed intake to urine and serum enterolignan levels in German females: A randomised controlled intervention trial. Food and Chemical Toxicology 44 (7):1057–64. doi: 10.1016/j.fct.2005.12.009.
- Kuhnle, G. G. C., C. Dell’Aquila, S. M. Aspinall, S. A. Runswick, A. A. Mulligan, and S. A. Bingham. 2008. Phytoestrogen content of beverages, nuts, seeds, and oils. Journal of Agricultural and Food Chemistry 56 (16):7311–5. doi: 10.1021/jf801534g.
- Kuhnle, G. G. C., C. Dell’aquila, Y.-L. Low, M. Kussmaul, and S. A. Bingham. 2007. Extraction and quantification of phytoestrogens in foods using automated solid-phase extraction and LC/MS/MS. Analytical Chemistry 79 (23):9234–9. doi: 10.1021/ac701732r.
- Kuhnle, G. G. C., C. Dell’Aquila, S. M. Aspinall, S. A. Runswick, A. M. Joosen, A. A. Mulligan, and S. A. Bingham. 2009. Phytoestrogen content of fruits and vegetables commonly consumed in the UK based on LC–MS and 13C-labelled standards. Food Chemistry 116 (2):542–54. doi: 10.1016/j.foodchem.2009.03.002.
- Kuijsten, A., I. C. W. Arts, P. Van’t Veer, and P. C. H. Hollman. 2005. The relative bioavailability of enterolignans in humans is enhanced by milling and crushing of flaxseed. The Journal of Nutrition 135 (12):2812–6. doi: 10.1093/jn/135.12.2812.
- Kuijsten, A., I. C. W. Arts, V. Tom B., and P. C. H. Hollman. 2005. Pharmacokinetics of enterolignans in healthy men and women consuming a single dose of secoisolariciresinol diglucoside. the Journal of Nutrition 135 (4):795–801. doi: 10.1093/jn/135.4.795.
- Lagkouvardos, I., K. Kläring, S. S. Heinzmann, S. Platz, B. Scholz, K.-H. Engel, P. Schmitt-Kopplin, D. Haller, S. Rohn, T. Skurk, et al. 2015. Gut metabolites and bacterial community networks during a pilot intervention study with flaxseeds in healthy adult men. Molecular Nutrition & Food Research 59 (8):1614–28. doi: 10.1002/mnfr.201500125.
- Lampe, J. W., C. Atkinson, and M. A. J. Hullar. 2006. Assessing exposure to lignans and their metabolites in humans. Journal of AOAC International 89 (4):1174–81. doi: 10.1093/jaoac/89.4.1174.
- Landete, J. M. 2012. Plant and mammalian lignans: a review of source, intake, metabolism, intestinal bacteria and health. Food Research International 46 (1):410–24. doi: 10.1016/j.foodres.2011.12.023.
- Laveriano-Santos, E. P., M. Marhuenda-Muñoz, A. Vallverdú-Queralt, M. Martínez-Huélamo, A. Tresserra-Rimbau, E. Miliarakis, C. Arancibia-Riveros, O. Jáuregui, A. M. Ruiz-León, S. Castro-Baquero, et al. 2022. Identification and quantification of urinary microbial phenolic metabolites by HPLC-ESI-LTQ-Orbitrap-HRMS and their relationship with dietary polyphenols in adolescents. Antioxidants 11 (6):1167. doi: 10.3390/antiox11061167.
- Laveriano-Santos, E. P., P. Quifer-Rada, M. Marhuenda-Muñoz, C. Arancibia-Riveros, A. Vallverdú-Queralt, A. Tresserra-Rimbau, A. M. Ruiz-León, R. Casas, R. Estruch, P. Bodega, et al. 2022. Microbial phenolic metabolites in urine are inversely linked to certain features of metabolic syndrome in Spanish adolescents. Antioxidants 11 (11):2191. doi: 10.3390/antiox11112191.
- Lee, J., J.-Y. Kang, K.-P. Ko, and S.-K. Park. 2021. The association between plasma concentration of phytoestrogens and hypertension within the Korean Multicenter Cancer Cohort. Nutrients 13 (12):4366. doi: 10.3390/nu13124366.
- Lee, S. H., B. H. Jung, S. Y. Kim, and B. C. Chung. 2004. Determination of phytoestrogens in traditional medicinal herbs using gas chromatography–mass spectrometry. The Journal of Nutritional Biochemistry 15 (8):452–60. doi: 10.1016/j.jnutbio.2004.01.007.
- Lin, Y., Y. Wang, and P-f Li. 2022. PPARα: An emerging target of metabolic syndrome, neurodegenerative and cardiovascular diseases. Frontiers in Endocrinology 13 (December):1074911. doi: 10.3389/fendo.2022.1074911.
- Liu, G., J. Li, Y. Li, Y. Hu, A. A. Franke, L. Liang, F. B. Hu, A. T. Chan, K. J. Mukamal, E. B. Rimm, et al. 2021. Gut microbiota–derived metabolites and risk of coronary artery disease: a prospective study among US men and women. The American Journal of Clinical Nutrition 114 (1):238–47. doi: 10.1093/ajcn/nqab053.
- Liu, J., S. Mi, L. Du, X. Li, P. Li, K. Jia, J. Zhao, H. Zhang, W. Zhao, and Y. Gao. 2018. The associations between plasma phytoestrogens concentration and metabolic syndrome risks in Chinese Population. PLOS One 13 (3):e0194639. doi: 10.1371/journal.pone.0194639.
- Liu, Z., N. M. Saarinen, and L. U. Thompson. 2006. Sesamin is one of the major precursors of mammalian lignans in sesame seed (Sesamum Indicum) as observed in vitro and in rats. The Journal of Nutrition 136 (4):906–12. doi: 10.1093/jn/136.4.906.
- Marhuenda-Muñoz, M., I. Domínguez-López, E. P. Laveriano-Santos, I. Parilli-Moser, C. Razquin, M. Ruiz-Canela, F. J. Basterra-Gortari, D. Corella, J. Salas-Salvadó, M. Fitó, et al. 2022. One-year changes in urinary microbial phenolic metabolites and the risk of type 2 diabetes—a case-control study. Antioxidants 11 (8):1540. doi: 10.3390/ANTIOX11081540/S1.
- Marinangeli, C. P. F., and P. J. H. Jones. 2011. Whole and fractionated yellow pea flours reduce fasting insulin and insulin resistance in hypercholesterolaemic and overweight human subjects. The British Journal of Nutrition 105 (1):110–7. doi: 10.1017/S0007114510003156.
- Mazur, W. M., M. Uehara, K. Wähälä, and H. Adlercreutz. 2000. Phyto-oestrogen content of berries, and plasma concentrationsand urinary excretion of enterolactone after a single strawberry-meal in human subjects. British Journal of Nutrition 83 (4):381–7.
- Mazur, W., T. Fotsis, K. Wähälä, S. Ojala, A. Salakka, and H. Adlercreutz. 1996. Isotope dilution gas chromatographic–mass spectrometric method for the determination of isoflavonoids, coumestrol, and lignans in food samples. Analytical Biochemistry 233 (2):169–80. doi: 10.1006/ABIO.1996.0025.
- Melby, M. K., S. Watanabe, P. L. Whitten, and C. M. Worthman. 2005. Sensitive high-performance liquid chromatographic method using coulometric electrode array detection for measurement of phytoestrogens in dried blood spots. Journal of Chromatography. B, Analytical Technologies in the Biomedical and Life Sciences 826 (1–2):81–90. Elsevier doi: 10.1016/j.jchromb.2005.08.009.
- Merten, M., and P. Thiagarajan. 2000. P-selectin expression on platelets determines size and stability of platelet aggregates. Circulation 102 (16):1931–6. doi: 10.1161/01.CIR.102.16.1931.
- Michalak, B., A. Filipek, P. Chomicki, M. Pyza, M. Woźniak, B. Żyżyńska-Granica, J. P. Piwowarski, A. Kicel, M. A. Olszewska, and A. K. Kiss. 2018. Lignans from forsythia × intermedia leaves and flowers attenuate the pro-inflammatory function of leukocytes and their interaction with endothelial cells. Frontiers in Pharmacology 9 (APR):401. doi: 10.3389/fphar.2018.00401.
- Milder, I. E. J., I. C. W. Arts, B. van de Putte, D. P. Venema, and P. C. H. Hollman. 2005. Lignan contents of Dutch plant foods: A database including lariciresinol, pinoresinol, secoisolariciresinol and matairesinol. The British Journal of Nutrition 93 (3):393–402. doi: 10.1079/BJN20051371.
- Miles, F. L., S. L. Navarro, C. B. Garrison, T. W. Randolph, Y. Zhang, A. Shojaie, M. Kratz, M. A. J. Hullar, D. Raftery, M. L. Neuhouser, et al. 2021. Urinary enterolactone is associated with plasma proteins related to immunity and cancer development in healthy participants on controlled diets. Human Nutrition & Metabolism 25 (September):200128. doi: 10.1016/j.hnm.2021.200128.
- Moazzami, A. A., and A. Kamal-Eldin. 2006. Sesame seed is a rich source of dietary lignans. Journal of the American Oil Chemists’ Society 83 (8):719–23. doi: 10.1007/s11746-006-5029-7.
- Moens, U., S. Kostenko, and B. Sveinbjørnsson. 2013. The role of mitogen-activated protein kinase-activated protein kinases (MAPKAPKs) in inflammation. Genes 4 (2):101–33. doi: 10.3390/genes4020101.
- Morisset, A.-S., S. Lemieux, A. Veilleux, J. Bergeron, S. John Weisnagel, and A. Tchernof. 2009. Impact of a lignan-rich diet on adiposity and insulin sensitivity in post-menopausal women. The British Journal of Nutrition 102 (2):195–200. doi: 10.1017/S0007114508162092.
- Mukker, J. K., V. Kotlyarova, R. S. P. Singh, and J. Alcorn. 2010. HPLC method with fluorescence detection for the quantitative determination of flaxseed lignans. Journal of Chromatography. B, Analytical Technologies in the Biomedical and Life Sciences 878 (30):3076–82. doi: 10.1016/j.jchromb.2010.09.012.
- Nesbitt, P. D., Y. Lam, and L. U. Thompson. 1999. Human metabolism of mammalian lignan precursors in raw and processed flaxseed. The American Journal of Clinical Nutrition 69 (3):549–55. doi: 10.1093/ajcn/69.3.549.
- Nielen, M. W. F., E. O. van Bennekom, H. H. Heskamp, J. H. A. van Rhijn, T. F. H. Bovee, and L. A. P. Hoogenboom. 2004. Bioassay-directed identification of estrogen residues in urine by liquid chromatography electrospray quadrupole time-of-flight mass spectrometry. Analytical Chemistry 76 (22):6600–8. doi: 10.1021/ac0490705.
- Niemeyer, H. B., D. M. Honig, S. E. Kulling, and M. Metzler. 2003. Studies on the metabolism of the plant lignans secoisolariciresinol and matairesinol. Journal of Agricultural and Food Chemistry 51 (21):6317–25. doi: 10.1021/jf030263n.
- Nishibe, S., M. Chiba, A. Sakushima, S. Hisada, S. Yamanouchi, M. Takido, U. Sankawa, and A. Sakakibara. 1980. Introduction of an alcoholic hydroxyl group into 2,3-dibenzylbutyrolactone lignans with oxidizing agents and carbon-13 nuclear magnetic resonance spectra of the oxidation products. Chemical and Pharmaceutical Bulletin 28 (3):850–60. doi: 10.1248/cpb.28.850.
- Nishibe, S., K. Mitsui-Saitoh, J. Sakai, and T. Fujikawa. 2021. The biological effects of forsythia leaves containing the cyclic AMP phosphodiesterase 4 inhibitor phillyrin. Molecules 26 (8):2362. doi: 10.3390/molecules26082362.
- Nørskov, N. P., C. Kyrø, A. Olsen, A. Tjønneland, and K. E. B. Knudsen. 2016. High-throughput LC–MS/MS method for direct quantification of glucuronidated, sulfated, and free enterolactone in human plasma. Journal of Proteome Research 15 (3):1051–8. doi: 10.1021/acs.jproteome.5b01117.
- Nørskov, N. P., A. Olsen, A. Tjønneland, A. K. Bolvig, H. N. Lærke, and K. E. B. Knudsen. 2015. Targeted LC-MS/MS method for the quantitation of plant lignans and enterolignans in biofluids from humans and pigs. Journal of Agricultural and Food Chemistry 63 (27):6283–92. doi: 10.1021/acs.jafc.5b01275.
- Nose, M., T. Fujimoto, T. Takeda, S. Nishibe, and Y. Ogihara. 1992. Structural transformation of lignan compounds in rat gastrointestinal tract. Planta Medica 58 (6):520–3. doi: 10.1055/s-2006-961540.
- Obermeyer, W. R., S. M. Musser, J. M. Betz, R. E. Casey, A. E. Pohland, and S. W. Page. 1995. Chemical studies of phytoestrogens and related compounds in dietary supplements: Flax and chaparral. Proceedings of the Society for Experimental Biology and Medicine 208 (1):6–12. doi: 10.3181/00379727-208-43824.
- Owen, A. J., and M. Abbey. 2004. The effect of estrogens and phytoestrogenic lignans on macrophage uptake of atherogenic lipoproteins. BioFactors 20 (3):119–27. doi: 10.1002/biof.5520200301.
- Palma-Duran, S. A., G. Caire-Juvera, M. M. Campa-Siqueiros, K. M. Chávez-Suárez, M. d R. Robles-Burgueño, M. L. Gutiérrez-Coronado, M. d C. Bermúdez-Almada, M. d S. Saucedo-Tamayo, P. Grajeda-Cota, and A. I. Valenzuela-Quintanar. 2020. A comprehensive HPLC-DAD-ESI-MS validated method for the quantification of 16 phytoestrogens in food, serum and urine. Applied Sciences 10 (22):8147. doi: 10.3390/app10228147.
- Pan, A., J. Sun, Y. Chen, X. Ye, H. Li, Z. Yu, Y. Wang, W. Gu, X. Zhang, X. Chen, et al. 2007. Effects of a flaxseed-derived lignan supplement in type 2 diabetic patients: A randomized, double-blind, cross-over trial. PLOS One 2 (11):e1148. doi: 10.1371/journal.pone.0001148.
- Parilli-Moser, I., I. Domínguez-López, A. Vallverdú-Queralt, S. Hurtado-Barroso, and R. M. Lamuela-Raventós. 2023. Urinary phenolic metabolites associated with peanut consumption may have a beneficial impact on vascular health biomarkers. Antioxidants 12 (3):698. doi: 10.3390/ANTIOX12030698.
- Peñalvo, J. L., H. Adlercreutz, M. Uehara, A. Ristimaki, and S. Watanabe. 2008. Lignan content of selected foods from Japan. Journal of Agricultural and Food Chemistry 56 (2):401–9. doi: 10.1021/jf072695u.
- Peñalvo, J. L., K. M. Haajanen, N. Botting, and H. Adlercreutz. 2005. Quantification of lignans in food using isotope dilution gas chromatography/mass spectrometry. Journal of Agricultural and Food Chemistry 53 (24):9342–7. doi: 10.1021/jf051488w.
- Peñalvo, J. L., and P. López-Romero. 2012. Urinary enterolignan concentrations are positively associated with serum HDL cholesterol and negatively associated with serum triglycerides in U.S. The Journal of Nutrition 142 (4):751–6. doi: 10.3945/jn.111.150516.
- Peterson, J., J. Dwyer, H. Adlercreutz, A. Scalbert, P. Jacques, and M. L. McCullough. 2010. Dietary lignans: Physiology and potential for cardiovascular disease risk reduction. Nutrition Reviews 68 (10):571–603. doi: 10.1111/j.1753-4887.2010.00319.x.
- Plaha, N. S., S. Awasthi, A. Sharma, and N. Kaushik. 2022. Distribution, biosynthesis and therapeutic potential of lignans. 3 Biotech 12 (10):255. doi: 10.1007/s13205-022-03318-9.
- Possemiers, S., S. Bolca, E. Eeckhaut, H. Depypere, and W. Verstraete. 2007. Metabolism of isoflavones, lignans and prenylflavonoids by intestinal bacteria: Producer phenotyping and relation with intestinal community. FEMS Microbiology Ecology 61 (2):372–83. doi: 10.1111/j.1574-6941.2007.00330.x.
- Prasad, K. 2011. Antioxidant activity of secoisolariciresinol diglucoside-derived metabolites, secoisolariciresinol, enterodiol, and enterolactone. The International Journal of Angiology 9 (4):220–5. doi: 10.1007/BF01623898.
- Prasain, J. K., A. Arabshahi, D. Ray Moore, G. A. Greendale, J. Michael Wyss, and S. Barnes. 2010. Simultaneous determination of 11 phytoestrogens in human serum using a 2min liquid chromatography/tandem mass spectrometry method. Journal of Chromatography. B, Analytical Technologies in the Biomedical and Life Sciences 878 (13–14):994–1002. doi: 10.1016/j.jchromb.2010.02.032.
- Quartieri, A., R. García-Villalba, A. Amaretti, S. Raimondi, A. Leonardi, M. Rossi, and F. Tomàs-Barberàn. 2016. Detection of novel metabolites of flaxseed lignans in vitro and in vivo. Molecular Nutrition & Food Research 60 (7):1590–601. doi: 10.1002/mnfr.201500773.
- Reger, M. K., T. W. Zollinger, Z. Liu, J. Jones, and J. Zhang. 2016. Urinary phytoestrogens and cancer, cardiovascular, and all-cause mortality in the continuous national health and nutrition examination survey. European Journal of Nutrition 55 (3):1029–40. doi: 10.1007/s00394-015-0917-y.
- Reger, M. K., T. W. Zollinger, Z. Liu, J. Jones, and J. Zhang. 2017. Association between urinary phytoestrogens and c-reactive protein in the continuous national health and nutrition examination survey. Journal of the American College of Nutrition 36 (6):434–41. doi: 10.1080/07315724.2017.1318722.
- Rizzolo-Brime, L., E. M. Caro-Garcia, C. A. Alegre-Miranda, M. Felez-Nobrega, and R. Zamora-Ros. 2022. Lignan exposure: A worldwide perspective. European Journal of Nutrition 61 (3):1143–65. doi: 10.1007/s00394-021-02736-4.
- Rodriguez-Leyva, D., W. Weighell, A. L. Edel, R. LaVallee, E. Dibrov, R. Pinneker, T. G. Maddaford, B. Ramjiawan, M. Aliani, R. Guzman, et al. 2013. Potent antihypertensive action of dietary flaxseed in hypertensive patients. Hypertension 62 (6):1081–9. doi: 10.1161/HYPERTENSIONAHA.113.02094.
- Ruggeri, Z. M. 2007. The role of von Willebrand factor in thrombus formation. Thrombosis Research 120 (Suppl 1):S5–S9. doi: 10.1016/j.thromres.2007.03.011.
- Saarinen, N. M., A. Wärri, S. I. Mäkelä, C. Eckerman, M. Reunanen, M. Ahotupa, S. M. Salmi, A. A. Franke, L. Kangas, and R. Santti. 2000. Hydroxymatairesinol, a novel enterolactone precursor with antitumor properties from coniferous tree (Picea Abies). Nutrition and Cancer 36 (2):207–16. doi: 10.1207/S15327914NC3602_10.
- Sawane, K., K. Hosomi, J. Park, K. Ookoshi, H. Nanri, T. Nakagata, Y.-A. Chen, A. Mohsen, H. Kawashima, K. Mizuguchi, et al. 2022. Identification of human gut microbiome associated with enterolignan production. Microorganisms 10 (11):2169. doi: 10.3390/microorganisms10112169.
- Senizza, A., G. Rocchetti, J. I. Mosele, V. Patrone, M. L. Callegari, L. Morelli, and L. Lucini. 2020. Lignans and gut microbiota: An interplay revealing potential health implications. Molecules 25 (23):5709. doi: 10.3390/molecules25235709.
- Setchell, K. D. R., R. Bull, and H. Adlercreutz. 1980. Steroid excretion during the reproductive cycle and in pregnancy of the vervet monkey (Cercopithecus Aethiopus Pygerythrus). Journal of Steroid Biochemistry 12 (C):375–84. doi: 10.1016/0022-4731(80)90296-4.
- Setchell, K. D. R., N. M. Brown, L. Zimmer-Nechemias, B. Wolfe, P. Jha, and J. E. Heubi. 2014. Metabolism of secoisolariciresinol-diglycoside the dietary precursor to the intestinally derived lignan enterolactone in humans. Food & Function 5 (3):491–501. doi: 10.1039/c3fo60402k.
- Shahidi, F., R. Amarowicz, H. A. Abou-Gharbia, and A. A. Y. Shehata. 1997. Endogenous antioxidants and stability of sesame oil as affected by processing and storage. Journal of the American Oil Chemists’ Society 74 (2):143–8. doi: 10.1007/s11746-997-0159-0.
- Shen, B., C. Zhao, Y. Wang, Y. Peng, J. Cheng, Z. Li, L. Wu, M. Jin, and H. Feng. 2019. Aucubin inhibited lipid accumulation and oxidative stress via Nrf2/HO-1 and AMPK signalling pathways. Journal of Cellular and Molecular Medicine 23 (6):4063–75. doi: 10.1111/jcmm.14293.
- Shinkaruk, S., E. Pinot, V. Lamothe, J.-M. Schmitter, L. Baguenard, B. Bennetau, and C. Bennetau – Pelissero. 2014. Design and validation of a novel immunological test for enterolactone. Talanta 119 (February):116–24. doi: 10.1016/j.talanta.2013.10.034.
- Shivappa, N., M. D. Wirth, E. A. Murphy, T. G. Hurley, and J. R. Hébert. 2019. Association between the Dietary Inflammatory Index (DII) and urinary enterolignans and C-reactive protein from the National Health and Nutrition Examination Survey-2003–2008. European Journal of Nutrition 58 (2):797–805. doi: 10.1007/s00394-018-1690-5.
- Simard, F. A., A. Cloutier, T. Ear, H. Vardhan, and P. P. McDonald. 2015. MEK-independent ERK activation in human neutrophils and its impact on functional responses. Journal of Leukocyte Biology 98 (4):565–73. doi: 10.1189/jlb.2MA1214-599R.
- Smeds, A. I., P. C. Eklund, R. E. Sjöholm, S. M. Willför, S. Nishibe, T. Deyama, and B. R. Holmbom. 2007. Quantification of a broad spectrum of lignans in cereals, oilseeds, and nuts. Journal of Agricultural and Food Chemistry 55 (4):1337–46. doi: 10.1021/jf0629134.
- Smeds, A. I., P. C. Eklund, and S. M. Willför. 2012. Content, composition, and stereochemical characterisation of lignans in berries and seeds. Food Chemistry 134 (4):1991–8. doi: 10.1016/j.foodchem.2012.03.133.
- Smeds, A. I., K. Hakala, T. T. Hurmerinta, L. Kortela, N. M. Saarinen, and S. I. Mäkelä. 2006. Determination of plant and enterolignans in human serum by high-performance liquid chromatography with tandem mass spectrometric detection. Journal of Pharmaceutical and Biomedical Analysis 41 (3):898–905. doi: 10.1016/j.jpba.2005.12.036.
- Smeds, A. I., N. M. Saarinen, P. C. Eklund, R. E. Sjöholm, and S. I. Mäkelä. 2005. New lignan metabolites in rat urine. Journal of Chromatography. B, Analytical Technologies in the Biomedical and Life Sciences 816 (1–2):87–97. doi: 10.1016/j.jchromb.2004.11.017.
- Socas-Rodríguez, B., A. V. Herrera-Herrera, J. Hernández-Borges, and M. Á. Rodríguez-Delgado. 2017. Multiresidue determination of estrogens in different dairy products by ultra-high-performance liquid chromatography triple quadrupole mass spectrometry. Journal of Chromatography. A 1496 (May):58–67. doi: 10.1016/j.chroma.2017.03.034.
- Struja, T., A. Richard, J. Linseisen, M. Eichholzer, and S. Rohrmann. 2014. The association between urinary phytoestrogen excretion and components of the metabolic syndrome in NHANES. European Journal of Nutrition 53 (6):1371–81. doi: 10.1007/s00394-013-0639-y.
- Talaei, M., B. L. Lee, C. N. Ong, R. M. van Dam, J. M. Yuan, W. P. Koh, and A. Pan. 2016. Urine phyto-oestrogen metabolites are not significantly associated with risk of type 2 diabetes: The Singapore Chinese Health Study. The British Journal of Nutrition 115 (9):1607–15. doi: 10.1017/S0007114516000581.
- Taxvig, C., I. O. Specht, J. Boberg, A. M. Vinggaard, and C. Nellemann. 2013. Dietary relevant mixtures of phytoestrogens inhibit adipocyte differentiation in vitro. Food and Chemical Toxicology 55 (May):265–71. doi: 10.1016/j.fct.2012.12.060.
- Thompson, L. U., B. A. Boucher, Z. Liu, M. Cotterchio, and N. Kreiger. 2006. Phytoestrogen content of foods consumed in Canada, including isoflavones, lignans, and coumestan. Nutrition and Cancer 54 (2):184–201. doi: 10.1207/s15327914nc5402_5.
- Tresserra-Rimbau, A., E. B. Rimm, A. Medina-Remón, M. A. Martínez-González, M. C. López-Sabater, M. I. Covas, D. Corella, J. Salas-Salvadó, E. Gómez-Gracia, J. Lapetra, et al. 2014. Polyphenol intake and mortality risk: A re-analysis of the PREDIMED trial. BMC Medicine 12 (1):77. doi: 10.1186/1741-7015-12-77.
- Udani, J. K., D. J. Brown, M. O. C. Tan, and M. Hardy. 2013. Pharmacokinetics and bioavailability of plant lignan 7-hydroxymatairesinol and effects on serum enterolactone and clinical symptoms in postmenopausal women: A single-blinded, parallel, dose - comparison study. Journal of the American College of Nutrition 32 (6):428–35. doi: 10.1080/07315724.2013.849578.
- Uehara, M., O. Lapcík, R. Hampl, N. Al-Maharik, T. Mäkelä, K. Wähälä, H. Mikola, and H. Adlercreutz. 2000. Rapid analysis of phytoestrogens in human urine by time-resolved fluoroimmunoassay. The Journal of Steroid Biochemistry and Molecular Biology 72 (5):273–82. doi: 10.1016/S0960-0760(00)00045-5.
- Valsta, L. M., A. Kilkkinen, W. Mazur, T. Nurmi, A.-M. Lampi, M.-L. Ovaskainen, T. Korhonen, H. Adlercreutz, and P. Pietinen. 2003. Phyto-oestrogen database of foods and average intake in Finland. The British Journal of Nutrition 89 (S1):S31–S38. doi: 10.1079/BJN2002794.
- van Oeveren, A., J. F. G. A. Jansen, and B. L. Feringa. 1994. Enantioselective synthesis of natural dibenzylbutyrolactone lignans (−)-enterolactone, (−)-hinokinin, (−)-pluviatolide, (−)-enterodiol, and furofuran lignan (−)-eudesmin via tandem conjugate addition to.gamma.-alkoxybutenolides. The Journal of Organic Chemistry 59 (20):5999–6007. doi: 10.1021/jo00099a033.
- Vanharanta, M., S. Voutilainen, T. A. Lakka, M. van der Lee, H. Adlercreutz, and J. T. Salonen. 1999. Risk of acute coronary events according to serum concentrations of enterolactone: A prospective population-based case-control study. Lancet 354 (9196):2112–5. doi: 10.1016/S0140-6736(99)05031-X.
- Vanharanta, M., S. Voutilainen, T. H. Rissanen, H. Adlercreutz, and J. T. Salonen. 2003. Risk of cardiovascular disease-related and all-cause death according to serum concentrations of enterolactone: Kuopio ischaemic heart disease risk factor study. Archives of Internal Medicine 163 (9):1099–104. doi: 10.1001/archinte.163.9.1099.
- Wang, L.-Q. 2002. Mammalian phytoestrogens: Enterodiol and enterolactone. Journal of Chromatography. B, Analytical Technologies in the Biomedical and Life Sciences 777 (1–2):289–309. doi: 10.1016/S1570-0232(02)00281-7.
- Wang, M., K. Wang, X. Liao, H. Hu, L. Chen, L. Meng, W. Gao, and Q. Li. 2021. Carnitine palmitoyltransferase system: A new target for anti-inflammatory and anticancer therapy? Frontiers in Pharmacology 12 (October):760581. doi: 10.3389/fphar.2021.760581.
- Wang, W., Q. Pan, X.-Y. Han, J. Wang, R.-Q. Tan, F. He, D.-Q. Dou, and T.-G. Kang. 2013. Simultaneous determination of arctiin and its metabolites in rat urine and feces by HPLC. Fitoterapia 86 (1):6–12. doi: 10.1016/j.fitote.2013.01.016.
- Waulters, Q. T., W. Bio-Sawe, R. Hofmann, and M. P. Sibi. 2018. Overview of enterolactone biological properties and total synthesis. Arkivoc 2018 (4):76–93. doi: 10.24820/ark.5550190.p010.451.
- Wilson, L., A. Arabshahi, B. Simons, J. K. Prasain, and S. Barnes. 2014. Improved high sensitivity analysis of polyphenols and their metabolites by nano-liquid chromatography–mass spectrometry. Archives of Biochemistry and Biophysics 559 (October):3–11. doi: 10.1016/j.abb.2014.06.014.
- Wolff, M. S., S. L. Teitelbaum, S. M. Pinney, G. Windham, L. Liao, F. Biro, L. H. Kushi, C. Erdmann, R. A. Hiatt, M. E. Rybak, et al. 2010. Investigation of relationships between urinary biomarkers of phytoestrogens, phthalates, and phenols and pubertal stages in girls. Environmental Health Perspectives 118 (7):1039–46. doi: 10.1289/ehp.0901690.
- Wu, W.-H. 2007. The contents of lignans in commercial sesame oils of Taiwan and their changes during heating. Food Chemistry 104 (1):341–4. doi: 10.1016/j.foodchem.2006.11.055.h]
- Wu, X., H. Cai, Y.-T. Gao, Q. Dai, H. Li, Q. Cai, G. Yang, A. A. Franke, W. Zheng, and X. O. Shu. 2012. Correlations of urinary phytoestrogen excretion with lifestyle factors and dietary intakes among middle-aged and elderly Chinese women. International Journal of Molecular Epidemiology and Genetics 3 (1):18–29.
- Wyns, C., S. Bolca, D. De Keukeleire, and A. Heyerick. 2010. Development of a high-throughput LC/APCI-MS method for the determination of thirteen phytoestrogens including gut microbial metabolites in human urine and serum. Journal of Chromatography. B, Analytical Technologies in the Biomedical and Life Sciences 878 (13-14):949–56. doi: 10.1016/j.jchromb.2010.02.022.
- Xie, L.-H., T. Akao, K. Hamasaki, T. Deyama, and M. Hattori. 2003. Biotransformation of pinoresinol diglucoside to mammalian lignans by human intestinal microflora, and isolation of Enterococcus Faecalis strain PDG-1 Responsible for the Transformation of (+)-Pinoresinol to (+)-Lariciresinol. Chemical & Pharmaceutical Bulletin 51 (5):508–15. doi: 10.1248/cpb.51.508.
- Xu, C., Q. Liu, Q. Zhang, A. Gu, and Z.-Y. Jiang. 2015. Urinary enterolactone is associated with obesity and metabolic alteration in men in the US National Health and Nutrition Examination Survey 2001–10. The British Journal of Nutrition 113 (4):683–90. doi: 10.1017/S0007114514004115.
- Yang, Z., G-l Yu, X. Zhu, T-h Peng, and Y-c Lv. 2022. Critical roles of FTO-mediated MRNA M6A demethylation in regulating adipogenesis and lipid metabolism: Implications in lipid metabolic disorders. Genes & Diseases 9 (1):51–61. doi: 10.1016/j.gendis.2021.01.005.
- Yetra, S. R., N. Schmitt, and U. K. Tambar. 2023. Catalytic photochemical enantioselective α-alkylation with pyridinium salts. Chemical Science 14 (3):586–92. doi: 10.1039/D2SC05654B.
- Yoshida, H., J. Shigezaki, S. Takagi, and G. Kajimoto. 1995. Variations in the composition of various acyl lipids, tocopherols and lignans in sesame seed oils roasted in a microwave oven. Journal of the Science of Food and Agriculture 68 (4):407–15. doi: 10.1002/jsfa.2740680403.
- Yoshida, H., and S. Takagi. 1997. Effects of seed roasting temperature and time on the quality characteristics of sesame (Sesamum Indicum) oil. Journal of the Science of Food and Agriculture 75 (1):19–26. doi: 10.1002/(SICI)1097-0010(199709)75:1<19::AID-JSFA830>3.3.CO;2-3.
- Zhang, C., W. Hu, G. J. Lovinger, J. Jin, J. Chen, and J. P. Morken. 2021. Enantiomerically enriched α-borylzinc reagents by nickel-catalyzed carbozincation of vinylboronic esters. Journal of the American Chemical Society 143 (35):14189–95. doi: 10.1021/jacs.1c05274.
- Zhang, W., X. Wang, Y. Liu, H. Tian, B. Flickinger, M. W. Empie, and S. Z. Sun. 2008. Dietary flaxseed lignan extract lowers plasma cholesterol and glucose concentrations in hypercholesterolaemic subjects. The British Journal of Nutrition 99 (6):1301–9. doi: 10.1017/S0007114507871649.
- Zhou, F., K. Furuhashi, M. J. Son, M. Toyozaki, F. Yoshizawa, Y. Miura, and K. Yagasaki. 2017. Antidiabetic effect of enterolactone in cultured muscle cells and in type 2 diabetic model Db/Db mice. Cytotechnology 69 (3):493–502. doi: 10.1007/s10616-016-9965-2.
- Zu, Y.-L., J. Qi, A. Gilchrist, G. A. Fernandez, D. Vazquez-Abad, D. L. Kreutzer, C.-K. Huang, and R. I. Sha’afi. 1998. P38 mitogen-activated protein kinase activation is required for human neutrophil function triggered by TNF-α or FMLP stimulation. The Journal of Immunology 160 (4):1982–1989. doi: 10.4049/jimmunol.160.4.1982.